A simple model indicates that there are sufficient water supply points for fighting forest fires in the Czech Republic
Jaroslav Holuša A E , Milan Koreň B , Roman Berčák A , Karolina Resnerová A , Jiří Trombik A , Jan Vaněk A , Ryszard Szczygieł C and Ivan Chromek DA Faculty of Forestry and Wood Sciences, Czech University of Life Sciences, Kamýcká 129, 16500 Praha 6 Suchdol, Czech Republic.
B Faculty of Forestry, Technical University in Zvolen, T. G. Masaryka 24, 960 53 Zvolen, Slovak Republic.
C Forest Research Institute, Laboratory of Forest Fire Protection, Braci Leśnej 3, 05-090 Raszyn, Poland.
D Faculty of Wood Sciences and Technology, Technical University in Zvolen, T. G. Masaryka 2117/24, 960 53 Zvolen, Slovak Republic.
E Corresponding author. Email: holusaj@seznam.cz
International Journal of Wildland Fire 30(6) 428-439 https://doi.org/10.1071/WF20103
Submitted: 6 July 2020 Accepted: 25 March 2021 Published: 23 April 2021
Journal Compilation © IAWF 2021 Open Access CC BY-NC-ND
Abstract
Long periods of drought and mild winters have significantly affected the forests of the Czech Republic in recent years. If this trend continues, forest stands will be weakened and dehydrated, which will increase the probability that forest fires will occur and spread. It is essential that fire managers prepare for this possibility. A main requirement for extinguishing large forest fires is the availability of water supply points. In this study, we determined if the Czech Republic has enough water to fight forest fires and whether these water supply points are distributed so that all forest stands will have enough water nearby to fight fires. We analysed forests, water supply points and forest roads in three study areas. One reservoir is sufficient to cover tens to hundreds of hectares of forest. We found that there are currently enough water supply points in the studied areas of the Czech Republic to extinguish forest fires. The results indicate that any shortage in water availability can be eliminated by using water supply points that are useable but that are not currently part of the database used by Fire Rescue Service of the Czech Republic. A thorough and regular update of the database of water supply points is therefore essential.
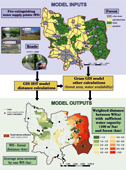
Keywords: central Europe, forest road, support decision system, volume of water required, water availability, water supply point.
Introduction
Forest fires are severe ecological and environmental disturbances (Jaiswal et al. 2002; Böhm et al. 2011) and are major challenges to forestry worldwide (Kandya et al. 1998; Tuia et al. 2008). The increasing effects of climate change, such as long periods of drought and mild winters, are leading to moisture loss in forest stands, which significantly increases the flammability of the forest environment (Xiao and Zhuang 2007; Xanthopoulos et al. 2012).
Interest in forest disturbances has increased in recent years, partly as a result of climate change and of the growing awareness of the potential for mega-disturbances that might result (Millar and Stephenson 2015). Forest disturbance regimes are influenced by climate (e.g. Westerling et al. 2006; Bentz et al. 2010) as well as by stand conditions (Fettig et al. 2007), and projected future changes in climate are expected to increase the probability of both wildfire frequency and severity as well as insect pest outbreaks (Bentz et al. 2010; Pechony and Shindell 2010).
With the increased number of dead trees and the accumulation of biomass in forests as a result of recent bark beetle outbreaks in central Europe (e.g. Vanická et al. 2020), the flammability of the forest environment has increased. When a forest stands is attacked by bark beetles, the stand loses its canopy; as a consequence, sunlight reaches the soil surface and supports the growth of grasses, and over time, small branches fall from the dry trees. These changes significantly increase the flammable fuel in the stand (Hicke et al. 2012; Hart et al. 2015). For example, a bark beetle outbreak in the Białowieża Forest caused the average fuel load of dead wood to increase from 16 t ha−1 in 2011 to 109 t ha−1 in 2017. The fuel load of grass cover, which developed after the decomposition of stands covering almost 14 000 ha, increased from ~0.5 kg m−2 before the outbreak to ~2.5 kg m−2 in 2017 (Szczygieł et al. 2018).
The number of forest fires in most of southern European countries (but also e.g. in Latvia) has recently been declining probably because intensive prevention measures (Turco et al. 2016; Donis et al. 2017). A decline in the number of small forest fires increases the probability of catastrophic forest fires, such as those that occurred in Portugal, Spain, Croatia and Greece in the summers of 2017 and 2018 (San-Miguel-Ayanz et al. 2019). Similarly, large-scale forest fires also severely affected northern European countries (Scotland, Norway and Sweden) in 2019 (International Association of Fire and Rescue Services 2019), although no temporal trend in average area of forest fire was evident in Sweden (Drobyshev et al. 2012). These natural disasters have burned a huge area of forest and also resulted in human casualties. In central Europe, forest fires do not threaten human populations but may cause great economic and environmental losses (De Rigo et al. 2017). Small fires, on the other hand, increase ecosystem diversity (Delarze et al. 1992; Hofmann et al. 1998; Tinner et al. 1999; Granström 2001).
Successful forest firefighting involves a combination of important decisions that affect fire development and the efficient use of available resources (Rodríguez-Veiga et al. 2018). Unlike industrial fires, in which the specifics of the burning area and the need for firefighting forces and supplies are relatively well known in advance, a forest fire represents a unique environment with many unknown variables. The assessment of individual forest fires is usually done by the commanding intervention officer, who is responsible for the correct risk assessment. Any mistakes in the risk assessment by firefighters can jeopardise firefighter safety and reduce the effectiveness of the fire suppression (Clancy 2011).
The decisive factor determining the time required to extinguish a forest fire is the fire’s place of origin. Each fire’s location is a certain distance from fire brigade stations and has varying terrain accessibility for fire engines. These factors influence the start time of forest fire extinguishing by fire brigades and the final size of the burnt area. In the case of bigger forest fires, water availability does not have to be sufficient. Then, the distance of the fire from water supply points becomes another important factor. Transport of water, which generally occurs over difficult terrain in central Europe, represents one of the most important factors in successful fire extinguishing (Dermek 2017). The further the water supply point is from the fire, the more forces and supplies are needed to ensure a continuous supply of water to fight the fire (Kozičová et al. 2015; Dermek 2017). The importance of both these factors (fire location and distance to a water supply point) depends on the density and quality of the forest road network, as well as on the availability of trained personnel (Dennison et al. 2012).
To ensure both quick and effective access to water supply points, firefighters require sufficient documentation about water reservoirs and other water sources in the area (Davis 2000). To be prepared to fight forest fires and to reduce the risk of such fires, individual territories and entire states should possess detailed maps of water supply points (Gill et al. 2008; Cerbu et al. 2013) and forest roads (Burnett 2001; Marchi et al. 2010; Cerbu et al. 2013). The water supply point for fighting fires must satisfy several basic requirements and in particular adequate accessibility for vehicles (Cerbu et al. 2013). With the development of firefighting mechanisation, however, these requirements have been significantly reduced such that information on all water sources and roads in forested areas is becoming increasingly important.
Forest fires are less common in central than in southern Europe because forest landscapes in central Europe are usually fragmented and the forests are usually temperate; the average number of forest fires per 10 000 ha per year is only 3 in the Czech Republic, 1–2 in Slovakia, and <1 in Austria, Germany and Switzerland (San-Miguel-Ayanz et al. 2019). The exception is Poland, where the number of forest fires per 10 000 ha per year is almost 8; furthermore, there are far fewer forest fires in Scandinavia (e.g. almost 2 per 10 000 in Sweden and <1 in Norway and Finland). While the average size of the burned area is >1 ha in Slovakia and Switzerland, it is <0.5 ha in Poland and other central European and Scandinavian countries. This is the result of sophisticated forest fire management in Poland, which includes the distribution of water supply points. Each location in a forest in Poland must be located within 3–5 km (depending on the forest fire risk category) of a water supply point with a minimum volume of 50 m3 (Szczygiel et al. 2020). Austria and Germany, in contrast, have no regulations regarding the availability of water sources in forests; the construction and mapping of water sources is not regulated and is done only by local management. In the Czech Republic, it is obligatory to have one water reservoir in each cadastral territory (i.e. a relatively homogeneous land area of ~600 ha on average).
The current study had two objectives: to determine whether there are enough water sources for forest fire management in the typical fragmented landscape of central Europe (the Czech Republic) (i.e. to determine whether it is necessary to build additional sources of water); and to determine how the volume of water available will affect fire interventions. In support of these objectives, we tested the following hypotheses: (i) there are several small water reservoirs and larger streams in the forests and their immediate surroundings that are currently usable as a water source or that would become usable after minor modifications that are missing from the records of the Fire Rescue Service of the Czech Republic and are therefore not used for firefighting; and (ii) the use of these unrecorded sources would reduce the distance of the water source from a possible fire and thus make fire intervention more efficient. To accomplish these objectives and to test these hypotheses, we used a model that included water supply point properties (location, suitability for firefighting and water volume), road properties (location and suitability for firefighting) and forest properties.
Methods
Study area
The Czech Republic (78 866 km2) is located in central Europe and in the middle of the temperate zone of the northern hemisphere (Fig. 1). Its climate is mild and includes four seasons. The climate is transitional between oceanic and continental, and is characterised by prevailing western winds, intensive cyclonal activity, and fairly high precipitation. The average temperature ranges from 3°C (January) to 17°C (July). The average annual precipitation across more than 60% of the country ranges from 600 to 800 mm (Tolasz 2007). The climate is, however, considerably influenced by the quite rapid changes in elevation and relief. The elevation ranges from 115 to 1603 m above sea level (asl) with a median of 430 m asl. The prevailing relief types are hills and highlands.
![]() |
The average population density is 133 people km−2. The naturally dominant plant communities in the Czech Republic are mixed beech–fir forests transitioning towards broad-leaved oak-dominated forests in the lowlands and towards coniferous spruce-dominated forests at higher elevations (Chytrý 2012). However, as a result of intensive forestry management since the 19th century, the present forest composition differs significantly from the natural state. Forests at present cover 33.9% of the country and are mainly composed of Picea abies (52%), Pinus sylvestris (17%), Fagus sylvatica (7%), Quercus spp. (7%), Larix decidua (4%), Betula pendula (3%) and Abies alba (1%). Other broad-leaved species (e.g. Carpinus betulus, Acer spp., Fraxinus spp., Populus spp., Salix spp. and Tilia spp.) occupy ~8% of the forested area (Ministry of Agriculture of the Czech Republic 2018).
Approximately 90% of the area of the Czech forests is represented by commercial forests that are intensively managed, usually via a clear-cutting system. More than 60% of the forested area is state-owned (Ministry of Agriculture of the Czech Republic 2018). The main forest disturbance agents in the Czech Republic are abiotic factors in the following order of importance: wind storms (which account for 50% of disturbances), droughts (5–13%), snow (2–12%) and frost (1–12%). The biotic disturbance factors are dominated by insect outbreaks (which account for 10–27% of disturbances). Wildfire plays a relatively minor role, is responsible for 1–7% of the volume of salvage logging (Zahradník Zahradníková 2019), and does not have substantial socioeconomic or ecologic effects. The minor effect of fire results from a fairly efficient system of fire detection and suppression.
Between 2006 and 2015, an average of 726 forest fires were recorded annually in the Czech Republic. The total burned area across these years was 25.8 km2, and the average was 2.58 km2 per year. Forest fires >10 ha are rare. The dominant type of forest fire was ground fire (Holuša et al. 2018). A monthly assessment of forest fire occurrence reveals two clear peaks, i.e. a spring peak and a summer peak. The spring peak is probably caused by human activity such as the burning of harvest residues and spring tourism. The spring peak is also triggered by the abundance of old grass, which is highly flammable. The summer peak is mostly influenced by weather conditions (high temperatures and low precipitation), but is also caused by human activity, i.e. summer tourism.
The Czech Republic is divided into three categories of forest fire risk (low, medium and high). Three study areas were selected to represent each category (Holuša et al. 2017). Our study area A surrounds Rakovník town (50.10N, 13.72E) (municipalities of Lužná, Líšany, Chrášťany, Olešná and Rakovník) and has a high risk of forest fire. Study area B surrounds Liberec town (50.76N, 15.06E) (municipalities Mníšek and Oldřichov v Hájích) and has a medium risk of forest fire. Study area C surrounds Kostelec nad Černými lesy town (49.99N, 14.85E) (municipalities of Kostelec nad Černými Lesy, Předhvozdí, Tuchoraz, and Přistoupim) and has a low risk of forest fire (Table 1, Fig. 1).
![]() |
Water supply points
All water supply points in the study areas were identified and evaluated with current field surveys. First, the list of water supply points of study areas was based on database water supply points used by the Fire Rescue Service of the Czech Republic and other available databases of water sources. Other water sources were identified using satellite and map images. The size (m2), volume (m3) and exact GIS (geographic information system) coordinates of the water supply points were applied from existing databases or established with GIS software and field surveys. The practical possibilities of fire brigades using water supply points for firefighting were also assessed with field surveys based on three criteria:
-
Road access to the water supply and a turning point for firefighting vehicles are available.
-
A secure point for transferring water from the supply to tanker equipment is available.
-
The water depth of water supply points cannot be lower than 1 m in the long term. In the case of watercourses, small dams are used to create these water supply points (Czech National Standard 75 2411 (Czech National Standard 2004); Czech National Standard 73 0834 (Czech National Standard 2011)).
We also determined whether each water supply point is currently used by the Fire Rescue Service of the Czech Republic, i.e. if the water supply point is included in Fire Rescue Service water supply points database. Based on these criteria, the water supply points in the three study areas were divided into four categories (Table 2). Water supply points 1 (WS1) satisfied all three criteria and are currently included in the database used by the Fire Rescue Service; WS2 also satisfied all three criteria but are not currently included in that database, because the obligation of municipalities is to manage one water source in the cadastral area; if modified, WS3 (Fig. 1) could satisfy the criteria (roads very often do not lead to WS3 water supply points); and WS4 could never satisfy the criteria.
![]() |
The locations of the water supply points in the study areas are indicated in Fig. 1, and their numbers and volumes are indicated in Table 3.
![]() |
Forest roads
Forest roads are either paved, or unpaved but stabilised and are 3.5 to 4.0 m wide (Fig. 2); the width is sufficient for firefighting vehicles (Fig. 3). They are used by forest workers throughout the growing season. A longitudinal gradient <8% is preferred, but gradients up to 10% occur and are acceptable for fighting fires. Traffic lanes must be fully drained and passing places must be provided (Fig. 2).
![]() |
The Fire Rescue Service of the Czech Republic, although professionalised, has almost no special vehicles for forest firefighting. Firefighting vehicles in the Czech Republic are divided into three categories: road, off-road, and mixed (Fig. 3). In the event of a forest fire, vehicles with increased off-road ability are generally given priority. All fires are reported to the Fire Rescue Service of the Czech Republic, which ensures their suppression. If necessary, support is provided by volunteer firefighters (Fire Rescue Service of the Czech Republic 2018).
After the forest roads were evaluated in the field, the forest road network was vectorised in the ArcMap environment based on the topographic map of the Czech Republic (1:10 000 and 1:5000) and aerial photographs of the study areas (Fig. 1).
Calculation of water supply distance
Distances to water supply points were optimised so as to minimise the distance travelled by firefighting vehicles.
In the first step, the shortest distances from the water supply points to the roads (WS3) were identified and then calculated if the roads did not lead to the water supply points (this was not case for WS1 and WS2). In the second step, the distances along the road network from the connection points to the water supply points were calculated. In the last step, the shortest distances from forest stands to roads were then calculated. The resulting distance of the grid cell (forest – 25 × 25 m raster) from the water supply point is the sum of the shortest distance from the cell to the road, the road distance to the nearest connection point, and the distance from the connection point to the water supply point (Fig. 4).
For each group of water supply points, the output is a set of raster layers. These calculations enabled us to compare the changes that occur with different groups of water supply points (Table 4). The average distances of individual forest stand from groups of water supply points were also calculated (Table 5). By overlapping the output raster layers with the layer of forest stands, we determined the area of the nearest forest according to the group of water supply points (Table 6) and also determined the weighted distance of forest stands from water supply points (Table 7; Fig. 5).
![]() |
![]() |
![]() |
![]() |
![]() |
Availability of water for firefighting
The amount of water in one water supply point is sufficient to extinguish forest fires only in a limited area. The area that is covered by one water supply point depends on the distance from the water supply point and its volume. In the next step of the calculations, we therefore considered the volume of water required to extinguish a forest fire, the distance from the water supply points, and the volume of the water supply points.
The volume of water required to extinguish a forest fire was determined based on data for the real volume of water used in forest firefighting in the Liberec Region (northern Czech Republic) in 2011–2015. For this region with an area of 3162.93 km2, we performed a detailed analysis of all 304 forest fires included in database of the Fire Rescue Service of the Czech Republic. The final burned areas varied between 1 m2 and 4 ha. The majority of fires are represented by small burned area not exceeding 0.5 ha. The region has a very rugged terrain, and water supply points are mostly located in valleys (Fig. 1). There is a close relationship between the size of the burned area and the amount of water used (which varied between 0.1 and 490 m3) to extinguish the fire (r = 0.83; P < 0.001):

where A is a burned area in hectares, and V is the amount of water used to extinguish a forest fire in cubic metres (m3).
The volume of water required to extinguish a forest fire varies over space and time. The volume of water is significantly affected by fuel volume and pattern, precipitation, weather, terrain complexity, the experience of firefighters and other factors (Keane et al. 2004). A suitable spatio-temporal model of the volume of water required to extinguish a forest fire was not available in the project period; therefore an average volume of water required to extinguish a forest fire per hectare Vha = 100.0 m3 ha−1 estimated by experienced forest firefighters (J. Vaněk, Dr I. Chromek, unpubl. data) was used in our study.
The volume of water required is in a separate raster layer. The layer was deduced from the layer of forest stands. The volume of water required was calculated in proportion to the cell size (25-m grid).

where Acell is the area of the raster cell (ha), and Vha is the volume of water required to extinguish a forest fire ha−1 (m3 ha−1).
Calculating the availability of water for forest firefighting is an iterative process. At every step, the distances to the water supply points in which water is still available and the coverage of the nearest forest area with water from these water supply points are calculated.
Separate input and output layers are created for every step of the iterative calculation. First, the distances to the water supply points with available water are calculated. The calculated distances from the forest (cell of forest) to the water supply points change at every step according to availability of water in each water supply point. The zones (areas) of the closest forest to the individual water supply points also change.
In the next step, the distribution of water to the nearest forest is calculated for each water supply point. The volume of water required to extinguish a fire is first calculated and assigned to cells of the nearest forest and is then calculated and assigned to cells of increasingly distant forests. The distribution of water from the water supply point is terminated when the requirements for water for all cells in the zone of the closest forest are satisfied, or when the entire volume of the water supply point is depleted.
The total volume of water available for extinguishing a forest fire is calculated as the sum of the volumes of available water from all water supply points during all steps of the simulation. The percentage of water demand satisfied (V%) is calculated based on the volume of water available and the volume of water required in the study:

where Vavailable is the volume of available water (m3), and Vrequired is the volume of water required to extinguish a forest fire (m3).
In some cases, water from several water supply points is required to extinguish a forest fire. In this case, the distance to the water supply points (dW) is calculated as the average of the distances to the water supply points (di) weighted by the volume of water obtained (Vi):

where n is the number of needed water supply points.
The weighted arithmetic mean distance thus considers the distance to the water supply points as well as the amount of water. If water is obtained from only one water supply point, the weighted distance is equal to the distance to the water supply point.
Results
Distance to water supply points
As the water supply points used increases from WS1 alone to include WS1 + WS2 (WS12) and then WS1 + WS2 + WS3 (WS123), the average distance that firefighting vehicles travel to the forest stand during the transport of water decreases in all three study areas (Table 5).
When multiple groups of water supply points are required (WS12 or WS123) rather than only WS1, the forest area covered by one water supply point (whether WS1, WS2, or WS3) decreases (Table 6).
Availability of water for fighting forest fires
The analysis also calculated the weighted distance to the water supply points from the individual raster cells of the forest assuming a water consumption of 100 m3 ha−1 of forest (Table 7). The water supply point is used only for the nearest raster cells of the forest, and if the supply point is depleted, it can no longer be used elsewhere.
The results in Table 7 differ from those in Table 5, because the algorithm used in Table 5 calculates distances to individual raster cells without considering water consumption, i.e. without considering that a water supply point can be exhausted.
The analysis of study area A shows that in its south-eastern part there is an area of 578.9 ha lacking water for extinguishing. The only water supply point is in the middle of this area, and its volume of water is very small. If the entire forest stand in area A were on fire and if only group WS1 water supply points are considered, there would not be enough water in this area to extinguish the fire (WS1). If other water supply points are included in the algorithm (WS12, WS123), the distance to the water supply point is reduced, and the problem of water shortage is eliminated (Fig. 5).
Discussion
The present study shows that there are currently sufficient water supply points in the studied areas of the Czech Republic to extinguish forest fires. One reservoir is sufficient to cover tens to hundreds of hectares of forest. No data are available on the situation in the surrounding central European countries, except for Poland, where at least one water supply point must be built or secured for every 3000 ha of forest (Szczygiel et al. 2020). If the currently used water supply points (WS1) are not sufficient, it is possible to increase the total water supply point of the area only by adding other suitable supply points (WS2) to the database of the Fire Rescue Service of the Czech Republic; group WS2 water supply points are not currently included in the database. Inclusion of the WS2 water supply points does not incur any cost and depends only on the arrangement between the fire departments of individual regions and the owners of the WS2 reservoirs. Proper documentation and optimisation of water supply points for fighting fires in European forests have been largely neglected. For example, we could not find any other research that performed an optimisation similar to the current one except for a study concerning the use of aviation technology in Spain (Rodríguez-Veiga et al. 2018).
When other types of reservoirs (WS2 and WS3) are used in addition to WS1 water supply points, the transport of water from the supply point to the forest fire is shortened. The reduction in distance is hundreds of metres for study areas A and C and ~1 km for study area B (Table 5). At the average speed of commonly used firefighting trucks, which is 56 km h−1 or ~15.5 m s−1 (Klaene and Sanders 2007), the reductions in distance would reduce travel times by 30 to 120 s. For comparison, the maximum speed of ground fire spread is 3 m min−1 (Rothermel 1983).
Shortening the time for intervention even by 60 s can be very important, especially if the fire is being spread from a focal point by wind. In this case, circumferential extinguishing is considered (Klaene and Sanders 2007). Consider, for example, a fire front that is 100 m long and advancing at 2 m min−1; if water delivery is slowed by 1 min, the fire will have spread over a 200-m2 area in that minute.
In practice, every fire, especially a forest fire, is unique. Unlike industrial fires, whose area is usually bounded by the object in which they burn, the limits of the spread of a forest fire can vary significantly based on both landscape and wind conditions (Thomas et al. 2010). In current models of forest fires, these factors are usually greatly simplified, and the actual fire as well as intervention measures may be different. From this point of view, even a 1-min reduction in intervention time can significantly affect the success of the intervention. It is not always possible to ensure the fulfillment of basic conditions that minimise the size of the fire, e.g. rapid fire detection and reporting, easy access to the area, sufficient firefighting forces, and sufficient water supply points. These are the basic factors that affect not only the time of fire development before it is reached by firefighters (Klaene and Sanders 2007), but also its location and extinguishing. In addition, the deployment of forces and water supply points can be complicated by the need to deploy forces and resources from one fire station to more than one event (traffic accident + forest fire, multiple forest fires, etc.) at the same time.
The majority of forest fires in the Czech Republic (3/4 of the total number) involve an area <0.1 ha (Holuša et al. 2018). Given that the average water consumption per hectare of fire is 100 m3, the volume of extinguishing water commonly sent in the initial deployment (i.e. 12 m3 for two fire trucks) is sufficient for these small-scale fires. If the fire is larger than 0.35 ha (i.e. an average fire; see Holuša et al. (2018)), three fire trucks (providing 2 × 4 m3 and 1 × 8 m3 of water = 16 m3 of water in total) are deployed according to the emergency plans for forest firefighting, and the commander can request a fourth fire truck if a shortage of water is expected. Therefore, with a basic volume of 16 m3 and timely reporting of a forest fire, sufficient access to the forest, and the availability of fire brigades, the initial water supply is usually sufficient and a shuttle service for water delivery is usually not required. A shuttle service is used only for fires larger than 0.35 ha, and this applies to <15% of the fires in the Czech Republic. Even when shuttle transport is needed (and cannot be replaced by a continuous hose line), three or four vehicles are sufficient to ensure a continuous supply of water. Only in the event of large fires will the intervention commander request additional firefighting equipment in order to maintain a continuous water supply.
Another factor that could reduce travel times is the density of the forest road network (Stergiadou 2014; Yakubu et al. 2015; Laschi et al. 2019), which is currently low (~16 m ha−1) in the Czech Republic. In Switzerland, the average density of the forest road network is 26.2 m ha−1, although this density ranges from 7.8 to 58.1 m ha−1 depending on the region. The road density is high in Austria (~35.4 m ha−1; Winkler and Hauk 1997) and is also high in Germany. Among central European countries, only Slovakia has a lower density than the Czech Republic. In the Czech Republic, it would be necessary to increase the density of the forest road network by ~10 m ha−1 in order to reach the density in Switzerland. This would mean building more than 27 000 km of new roads and increasing the total length of the forest road network by more than 60%. These values can be considered as a maximal goal for the forest infrastructure of the Czech Republic. The forest road networks in the areas of the current study are quite dense, ranging between 70 and 80% of the desired values of road density (Bystrický and Sirota 2013).
In the study areas of the current research, the existing forest road networks are sufficient in terms of density and in terms of suitability for the firefighting equipment used (Fig. 2). Distances to water supply points were calculated to maximise road movement and minimise off-road movement. The approach used in the current study assumes that water transport is more efficient on paved roads than on non-paved roads. On non-paved roads, the movement of vehicles can be significantly hampered by obstacles, wet soils, trees and other factors (Monoši and Dermek 2016).
With the use of a shuttle service to move needed water to forest fire areas, the vehicles must stop on paved roads if they encounter impassable areas; as a consequence, the distance that the vehicles must travel to the fire can increase. To account for this, the model could consider the maximum distance of the fire from the vehicle, so that the availability of water would not have to be calculated for the whole forest stand. The use of portable hose lines, however, can increase the total reach of a hose line to 80–100 m when dispensing water (Corbett 2009).
Depending on the groups of water supply points considered in the analyses, the area of forest (km2) optimally distant from the individual water reservoirs changes (Table 6). This means that as the number of water supply points used for firefighting increases, the area of forest covered by individual water supply points decreases (the forest area is divided among a larger number of reservoirs). This would reduce the amount of water pumped out of individual sources, so that potential fires in other parts of the forest would be covered by other water supply points. Increasing the number of water sources can therefore affect the viability of a water source, i.e. it would prevent a water source from drying out or being damaged owing to lack of water. In this regard, regular updating of water supply points, and also of roads, is critical.
The model searches only for the nearest water supply point. If the capacity of the tanks is limited, its water capacity may not always be sufficient to extinguish a fire in the nearest area. Because the availability of water in the studied areas ranges from hundreds to thousands of metres cubed per hectare when supply points are among those included in database of water supply points for extinguishing fires, the water sources in the studied areas would be able to provide enough water for forest fires with burned areas of 10 ha. If it were possible to estimate the volume of water needed to extinguish a unit of forest area, the model could search for the several nearest water sources that could together provide a sufficient volume of water. Also, the model as used in the current analysis kept the required water capacity for forest fire extinguishing constant for the entire forest area. The model makes it possible, however, to set different water volume requirements for each raster cell. Different requirements for the volume of water needed to extinguish a forest fire may be set according to the species composition and age of the forest stand. When analysing the availability of water, the calculation of the water required for extinguishing should be performed on the basis of recent data in the studied area (we used a value of 100 m3 ha−1).
The total capacity of each water source was used when simulating the distribution of water to forest fires. Given operational and ecological limits, it would be more appropriate to consider the usable capacity of the water supply point, for example 2/3 of the total volume. Part of the water in a source may not be available owing to lowering of the water level.
The simulation of the availability of water for fighting forest fires was done for the whole area at the same time. In the event of a real fire in part of the area, the availability of water and the distance to the nearest supply may be different. The calculation could be modified so that the availability of water and the distance to the water supply point were calculated only for a limited part of a forest stand. The model could be easily modified to account for this and could be easily provided to and accessed by the local fire department units for optimal planning of interventions. Model simulations may also quickly provide information about nearest water supply points and the shortest routes to them. To simulate the real situation, the model could also be modified to account for restrictive conditions on the roads, such as the closure of a section of the road due to a forest fire.
Conclusions
A distance-based model was developed for calculating optimal shuttle transport of water and the use of different types of water supply points for fighting forest fires. Shuttle transport, however, is currently needed in only a small number of cases because the currently used firefighting machinery carries sufficient water to extinguish average fires (which are small). If all available water supply points in the study areas (i.e. WS123) were considered rather than only the WS1 water supply points (which are the water supply points currently used by the Fire Rescue Service of the Czech Republic), the time saved to transport water to a fire would range from 30 to 180 s.
Although the database of water supply points currently used by firefighters (i.e. WS1 water supply points) is probably sufficient in most parts of the Czech Republic, each area clearly contains other supply points (WS2 and WS3 supplies) that meet the requirements for the collection of water to be used for fighting fires; these other supplies could be immediately included in the database used by firefighters. The optimisation model developed in this study can detect areas that would not be optimally supplied by water in the event of complex forest fires. We therefore suggest that each forest owner use the model to perform a simple analysis that would reveal any potential shortcomings in current fire protection plans. We also recommend that the model be used to study those areas that local fire departments consider to be at high danger in forest fires, i.e. areas with large and complex forests, with sparse forest road networks, and with limited water supply points.
Before the model is used, however, comprehensive documentation of potential water supply points should be conducted throughout the Czech Republic. Although the current legislation on regional forest development requires an overview of natural or artificial sources of water sources that are available and accessible to firefighting equipment for pumping water for forest firefighting, these data are usually used only by local fire departments and no nationwide database of water supply points that can be used to fight fires currently exists. The database should be updated every 5 years.
Conflicts of interest
The authors declare no conflicts of interest.
Declaration of funding
This work was supported by projects of the Czech University of Life Sciences No. IGA A_20_11 and IGA A_20_08.
Acknowledgements
The authors thank Dr Bruce Jaffee (USA) for checking the English.
References
Bentz BJ, Régnière J, Fettig CJ, Hansen EM, Hayes JL, Hicke JA, Seybold SJ (2010) Climate change and bark beetles of the western United States and Canada: direct and indirect effects. Bioscience 60, 602–613.| Climate change and bark beetles of the western United States and Canada: direct and indirect effects.Crossref | GoogleScholarGoogle Scholar |
Böhm CH, Quinkenstein A, Freese D (2011) Yield prediction of young black locust (Robinia pseudoacacia L.) plantations for woody biomass production using allometric relations. Annals of Forest Research 54, 215–227.
Burnett HS (2001) Forest roads: Benefits for wildlife management, fire suppression, and water quality. Water Resources Impact 3, 5–7.
Bystrický R, Sirota I (2013) Lesní dopravní síť v ČR stav a budoucnost. [Forest roads in the Czech Republic, state and future]. Lesnická práce 92, 17–19.
Cerbu G, Hanewinkel M, Berger F, Schüler S, Gerosa G, Jandl R (2013) ‘Management strategies to adapt Alpine Space forests to climate change risks.’ (BoD – Books on Demand).
Chytrý M (2012) Vegetation of the Czech Republic: diversity, ecology, history and dynamics. Preslia 84, 427–504.
Clancy D (2011) Can acceptable risk be defined in wildland firefighting? In ‘Proceedings of the Second Conference on the Human Dimensions of Wildland Fire’, Newtown Square, PA. USDA Forest Service, Northern Research Station.
Corbett G (2009) ‘Fire Engineering’s Handbook for Firefighter I and II.’ (Fire Engineering Books: Oklahoma, USA).
Czech National Standard (2004) ČSN 75 2411 - Zdroje požární vody. [Czech National Standard 75 2411. Water supply points for firefighting]. Czech Republic.
Czech National Standard (2011) ČSN 73 0834 Požární bezpečnost staveb. [Czech National Standard 73 0834 – Fire safety of buildings]. Czech Republic.
Davis S (2000) ‘Fire Fighting Water: A Review of Fire Fighting Water Requirements. A New Zealand Perspective.’ (University of Canterbury: New Zealand).
De Rigo D, Libertà G, Houston Durrant T, Artés Vivancos T, San-Miguel-Ayanz J (2017) ‘Forest fire danger extremes in Europe under climate change: variability and uncertainty.’ (Publications Office of the European Union: Luxembourg).
Delarze R, Caldelari D, Hainnard P (1992) Effects of fire on forest dynamics in southern Switzerland. Journal of Vegetation Science 3, 55–60.
| Effects of fire on forest dynamics in southern Switzerland.Crossref | GoogleScholarGoogle Scholar |
Dennison KJ, Mullineaux DR, Yates JW, Abel MG (2012) The effect of fatigue and training status on firefighter performance. Journal of Strength and Conditioning Research 26, 1101–1109.
| The effect of fatigue and training status on firefighter performance.Crossref | GoogleScholarGoogle Scholar | 22446677PubMed |
Dermek M (2017) The parameters of the optimal method of water transport to forest fires. Procedia Engineering 192, 96–100.
| The parameters of the optimal method of water transport to forest fires.Crossref | GoogleScholarGoogle Scholar |
Donis J, Kitenberga M, Šņepsts G, Matisons R, Zariņš J, Jansons A (2017) The forest fire regime in Latvia during 1922–2014. Silva Fennica 51, 7746
| The forest fire regime in Latvia during 1922–2014.Crossref | GoogleScholarGoogle Scholar |
Drobyshev I, Niklasson M, Linderholm HW (2012) Forest fire activity in Sweden: Climatic controls and geographical patterns in 20th century. Agricultural and Forest Meteorology 154–155, 174–186.
| Forest fire activity in Sweden: Climatic controls and geographical patterns in 20th century.Crossref | GoogleScholarGoogle Scholar |
Fettig CJ, Klepzig KD, Billings RF, Munson AS, Nebeker TE, Negrón JF, Nowak JT (2007) The effectiveness of vegetation management practices for prevention and control of bark beetle infestations in coniferous forests of the western and southern United States. Forest Ecology and Management 238, 24–53.
| The effectiveness of vegetation management practices for prevention and control of bark beetle infestations in coniferous forests of the western and southern United States.Crossref | GoogleScholarGoogle Scholar |
Fire Rescue Service of the Czech Republic (2018) Fire Rescue Service of the Czech Republic. MoI-Directorate General of the FRS CR. (Prague, Czech Republic).
Gill A, Worthy M, Wade A, Mueller N, White I (2008) Fire and Water: Framing Fire and Fuel Management of Water-supply Catchments. In ‘Proceedings of Water Down Under 2008: Incorporating 31st Hydrology and Water Resources Symposium and 4th International Conference on Water Resources and Environment Research’, 14–17 April, 2008, Adelaide, SA, Australia. (Eds M Lambert, T Daniell, M Leonard). pp. 1463–1471. (Engineers Australia and Causal Productions: Modbury, SA)
Granström A (2001) Fire management for biodiversity in the European boreal forest. Scandinavian Journal of Forest Research 16, 62–69.
| Fire management for biodiversity in the European boreal forest.Crossref | GoogleScholarGoogle Scholar |
Hart SJ, Schoennagel T, Veblen TT, Chapman TB (2015) Area burned in the western United States is unaffected by recent mountain pine beetle outbreaks. Proceedings of the National Academy of Sciences of the United States of America 112, 4375–4380.
| Area burned in the western United States is unaffected by recent mountain pine beetle outbreaks.Crossref | GoogleScholarGoogle Scholar | 25831541PubMed |
Hicke JA, Johnson MC, Hayes JL, Preisler HK (2012) Effects of bark beetle-caused tree mortality on wildfire. Forest Ecology and Management 271, 81–90.
| Effects of bark beetle-caused tree mortality on wildfire.Crossref | GoogleScholarGoogle Scholar |
Hofmann C, Conedera M, Delarze R, Carraro G, Giorgetti P (1998) Effets des incendies de forêt sur la végétation au sud des Alpes suisses. Mitteilungen der Eidgenössischen Forschungsanstalt fur Wald, Schnee und Landschaft 73, 1–90.
Holuša J, Lukášová K, Berčák R, Trombik J (2017) Set of maps: distribution of forests in the Czech Republic based on the threat of forest fires. Czech University of Life Sciences. (Prague, Czech Republic).
Holuša J, Berčák R, Lukášová K, Hanuška Z, Agh P, Vaněk J, Kula E, Chromek I (2018) Forest fires in the Czech Republic – definition and classification: review. Zprávy Lesnického Vyzkumu 63, 20–27.
International Association of Fire and Rescue Services (2019) Scotland, Norway and Sweden already severely affected by forest fires due to the dry weather in the north. Available at https://www.ctif.org/news/scotland-norway-and-sweden-already-severely-effected-forest-fires-due-dry-weather-north [Verified 24 April 2019]
Jaiswal RK, Mukherjee S, Raju KD, Saxena R (2002) Forest fire risk zone mapping from satellite imagery and GIS. International Journal of Applied Earth Observation and Geoinformation 4, 1–10.
| Forest fire risk zone mapping from satellite imagery and GIS.Crossref | GoogleScholarGoogle Scholar |
Kandya AK, Kimothi MM, Jadhav RN, Agrawal JP (1998) Application of GIS in identification of fire prone areas – a feasibility study in parts of Junagarh (Gujrat, India). Indian Forester 124, 531–535.
Keane RE, Cary GJ, Davies ID, Flannigan MD, Gardner RH, Lavorel S, Lenihan JM, Li C, Rupp TS (2004) A classification of landscape fire succession models: spatial simulations of fire and vegetation dynamics. Ecological Modelling 179, 3–27.
| A classification of landscape fire succession models: spatial simulations of fire and vegetation dynamics.Crossref | GoogleScholarGoogle Scholar |
Klaene B, Sanders R (2007) ‘Structural firefighting: strategy and tactics.’ (Jones & Bartlett Learning).
Kozičová B, Dermek M, Monoši M (2015) Risks fires in the natural environment. In ‘Management of Environment 2015’, XV. Medzinárodnej vedeckej konferencie: Bratislava, 17–18 Apríl 2015, Strix, Žilina (Eds M Rusko, J Harangozó).
Laschi A, Foderi C, Fabiano F, Neri F, Cambi M, Mariotti B, Marchi E (2019) Forest road planning, construction and maintenance to improve forest fire fighting: a review. Croatian Journal of Forest Engineering 40, 207–219.
Marchi E, Montorselli NB, Neri F (2010) The role of forest road network in forest fire prevention and suppression: a case study in Italy. In ‘FORMEC – Forest Engineering: Meeting the Needs of the Society and the Environment’, 43rd International Symposium on Forestry Mechanization, 11–14 July, Padua, Italy. (Eds R Cavalli, S Grigolato) pp. 11–14. (University of Padua, Legnaro, Italy)
Millar CI, Stephenson NL (2015) Temperate forest health in an era of emerging megadisturbance. Science 349, 823–826.
| Temperate forest health in an era of emerging megadisturbance.Crossref | GoogleScholarGoogle Scholar | 26293954PubMed |
Ministry of Agriculture of the Czech Republic (2018) Information on forests and forestry in the Czech Republic by 2017. Ministry of Agriculture of the Czech Republic. (Prague, Czech Republic).
Monoši M, Dermek M (2016) The purpose of fire engines at fires in environment. Journal of Environmental Protection 7, 1–4.
Pechony O, Shindell DT (2010) Driving forces of global wildfires over the past millennium and the forthcoming century. Proceedings of the National Academy of Sciences of the United States of America 107, 19167–19170.
| Driving forces of global wildfires over the past millennium and the forthcoming century.Crossref | GoogleScholarGoogle Scholar | 20974914PubMed |
Rodríguez-Veiga J, Costa IG, Villamayor MJG, Méndez BC, Díaz JL (2018) Assignment problems in wildfire suppression: models for optimization of aerial resource logistics. Forest Science 64, 504–514.
| Assignment problems in wildfire suppression: models for optimization of aerial resource logistics.Crossref | GoogleScholarGoogle Scholar |
Rothermel RC (1983) How to predict the spread and intensity of forest and range fires. USDA Forest Service, Intermountain Forest and Range Experiment Station, General Technical Report INT-143. (Ogden, UT, USA)
San-Miguel-Ayanz J, Durrant T, Boca R, Liberta G, Branco A, De Rigo D, Ferrari D, Maianti P, Artes Vivancos T, Pfeiffer H, Loffler P, Nuijten D, Leray T, Jacome Felix Oom D (2019) ‘Forest Fires in Europe, Middle East and North Africa 2018.’ EUR 29856 EN (Publications Office of the European Union: Luxembourg)
Stergiadou A (2014) Prevention and suppression of forest-fires by using the road network and water tanks. Fresenius Environmental Bulletin 23, 2755–2761.
Szczygieł R, Kwiatkowski M, Kołakowski B (2018) Influence of bark beetle infestation on the forest fire risk in the Białowieża Forest. Sylwan 162, 955–964.
Szczygiel R, Kwiatkowski M, Piwnicki J, Kołakowski B (2020)’ Instrukcja ochrony przeciwpożarowej lasu.’ (Centrum Informacyjne Lasów Państowowych: Warszawa).
Thomas PA, McAlpine RS, Hirsch K, Hobson P (2010) ‘Fire in the forest.’ (Cambridge University Press: Cambridge, UK).
Tinner W, Hubschmid P, Wehrli M, Ammann B, Conedera M (1999) Long-term forest-fire ecology and dynamics in southern Switzerland. Journal of Ecology 87, 273–289.
| Long-term forest-fire ecology and dynamics in southern Switzerland.Crossref | GoogleScholarGoogle Scholar |
Tolasz R (2007) ‘Atlas podnebí Česka.’ [Climate atlas of Czechia] (Czech Hydrometeorological Institute and Palacký University Olomouc: Olomouc, Czech Republic).
Tuia D, Ratle F, Lasaponara R, Telesca L, Kanevski M (2008) Scan statistics analysis of forest fire clusters. Communications in Nonlinear Science and Numerical Simulation 13, 1689–1694.
| Scan statistics analysis of forest fire clusters.Crossref | GoogleScholarGoogle Scholar |
Turco M, Bedia J, Di Liberto F, Fiorucci P, von Hardenberg J, Koutsias N, Llasat MC, Xystrakis F, Provenzale A (2016) Decreasing fires in Mediterranean Europe. PLoS One 11, e0150663
| Decreasing fires in Mediterranean Europe.Crossref | GoogleScholarGoogle Scholar | 26982584PubMed |
Vanická H, Holuša J, Resnerová K, Ferenčík J, Potterf M, Véle A, Grodzki W (2020) Interventions have limited effects on the population dynamics of Ips typographus and its natural enemies in the Western Carpathians (central Europe). Forest Ecology and Management 470–471, 118209
| Interventions have limited effects on the population dynamics of Ips typographus and its natural enemies in the Western Carpathians (central Europe).Crossref | GoogleScholarGoogle Scholar |
Westerling AL, Hidalgo HG, Cayan DR, Swetnam TW (2006) Warming and earlier spring increase western US forest wildfire activity. Science 313, 940–943.
| Warming and earlier spring increase western US forest wildfire activity.Crossref | GoogleScholarGoogle Scholar | 16825536PubMed |
Winkler H, Hauk E (1997) ‘Gute Walderschließung in Österreich.’ (Forstliche Bundesversuchasanstalt –Österreichisches Waldforschungszentrum)
Xanthopoulos G, Calfapietra C, Fernandes P (2012) Fire hazard and flammability of European forest types. In ‘Post-fire management and restoration of Southern European forests.’ (Ed. F. Moreira, M. Arianoutsou, P. Corona, J. De las Heras) Managing Forest Ecosystems Series Vol. 24, pp. 79–92. (Springer: Dordrecht).
Xiao J, Zhuang Q (2007) Drought effects on large fire activity in Canadian and Alaskan forests. Environmental Research Letters 2, 044003
| Drought effects on large fire activity in Canadian and Alaskan forests.Crossref | GoogleScholarGoogle Scholar |
Yakubu I, Mireku-Gyimah D, Duker AA (2015) Review of methods for modelling forest fire risk and hazard. African Journal of Environmental Science and Technology 9, 155–165.
| Review of methods for modelling forest fire risk and hazard.Crossref | GoogleScholarGoogle Scholar |
Zahradník P, Zahradníková M (2019) Salvage felling in the Czech Republic’s forests during the last twenty years. Central European Forestry Journal 65, 12–20.