When soil becomes fuel: identifying a safe window for prescribed burning of Tasmanian vegetation growing on organic soils
Lynda D. Prior


A
B
Abstract
Flammable vegetation in Tasmania, Australia often grows on organic soils that can burn if sufficiently dry.
To develop an approach to identify a safe window for prescribed burning of vegetation on Tasmanian organic soils, when vegetation is dry enough to be combustible, yet organic soils are unlikely to burn.
We compiled a dataset of when organic soils did and did not burn when exposed to vegetation fires. Focussing on moorland, we used binomial modelling to estimate the probability of organic soil burning in relation to soil dryness index (SDI) computed from climate data. Vegetation combustibility was inferred from fuel moisture content estimated from climate data and records of area burnt.
Risk of soil fire varied with vegetation. In moorland, modelling predicted a 17% risk when SDI was 10, a conservative estimate because our dataset was biased towards positive records of soil fire. Using an SDI threshold of 10, the average annual number of ‘safe combustible’ days varied across Tasmania from 26 to 53.
This approach can be used to refine safe burning guidelines on organic soil.
This approach, when applied to an improved dataset, will assist fire management on organic soils.
Keywords: climate change, fine fuel moisture content, fire risk, organic soils, peat, prescribed fire, soil dryness index, soil fire, Tasmanian Wilderness World Heritage Area, treeless vegetation.
Introduction
Organic soils are globally important because they contain an immense store of carbon and can play a critical role in regional hydrology, acting as both a ‘sponge’ and a filter to maintain water quality and quantity (Joosten 2009; Turetsky et al. 2015; Goldstein et al. 2020; Wilson et al. 2022). Organic soils are defined as soils containing more than 12–18% carbon depending on clay content (Isbell 2002). Organic soils are rare in mainland Australia (Whinam and Hope 2005; Joosten 2009), but in the island state of Tasmania, 15% (approximately 1 million ha) has been mapped as having organosols (Cotching et al. 2009). These occur mostly in western Tasmania, and include the most extensive blanket bogs in the southern hemisphere (Bridle et al. 2003; Whinam and Hope 2005; di Folco 2007). The accumulation of organic matter that forms organic soils and various peat landforms is recognised as part of the Outstanding Universal Values of the Tasmanian Wilderness World Heritage Area. Tasmanian organic soils present a challenge for fire managers because of their embodied conservation values, the sharply contrasting fire ecology of vegetation types that grow on them and their vulnerability to fire damage; soil fire can cause significant and permanent ecosystem change in a single event (Hill 1982; Bowman et al. 2021; Prior et al. 2023). Organic soils form so slowly (0.4–4.2 mm year−1) that if burnt, it can take decades to centuries for them to recover (Wood et al. 2011). In addition to the ecological and human health damage caused by soil fires (Hill 1982; Bridle et al. 2003; Johnston et al. 2012), organic soils are a troublesome fuel. Once ignited they can be extremely difficult to extinguish, and can smoulder for months, flaring up to ignite surface fuels when weather becomes more favourable for vegetation fires (Lin et al. 2019, Santoso et al. 2019). Unfortunately, extinguishing hotspots can be time consuming, expensive, and often impractical.
Understanding the drivers of combustibility is key to predicting when organic soils are vulnerable to ignition. Such information is needed for fire managers to assess risks when planning fuel reduction burns, and to triage suppression efforts when there are multiple fires. It is widely accepted that soil moisture is a major control on the combustibility of organic soils (Frandsen 1987; Garlough and Keyes 2011; Prat-Guitart et al. 2016; Prior et al. 2020). Laboratory studies have been invaluable in determining how the risk of soil ignition varies with individual factors such as soil moisture content, soil bulk density and organic matter content (Frandsen 1997; Garlough and Keyes 2011; Prat-Guitart et al. 2016). However, scaling up the results of laboratory studies to make predictions at the landscape level is fraught with difficulty, because landscapes are topographically heterogeneous, such that soils and soil moisture can vary over fine spatial scales (di Folco and Kirkpatrick 2011; Lukenbach et al. 2015; Hokanson et al. 2016). One example where scaling up was attempted was when Prior et al. (2020) derived soil moisture thresholds from their laboratory studies, and then estimated the volumetric water content of Tasmanian organic soils using a geographic information system and a high-resolution climate database. This showed that most Tasmanian organic soils are likely to be combustible at some time almost every summer, but there was no field validation of this inference.
Thus, while laboratory studies can indicate the factors controlling soil combustibility, they need to be complemented with field observations of when organic soils are likely to ignite. Direct field observations are challenging given the intractable terrains that support organic soils, the stochastic occurrence of wildfires, and practical difficulties in collecting data during fire management operations.
Based on a preliminary study of fire behaviour, the potential for peat fires in flat buttongrass moorland has been considered to be low, because the soil moisture content is typically high throughout the year (Marsden-Smedley 1993). Nonetheless, peat fires do occur in buttongrass moorland, possibly initiated at the base of large shrubs and copses of small trees (Marsden-Smedley 1993; French et al. 2016). The occurrence of organic soil fires has become an important management issue with guidelines recommending that planned burning in buttongrass occurs in spring and autumn, under mild fire weather conditions and when soils are wet, although predictions of fire risk based on soil dryness index (SDI) have been described as unreliable for buttongrass soils (Marsden-Smedley 2009). This study is motivated to advance understanding of the fire risk to organic soils. We investigated when it is safe to burn Tasmanian vegetation growing on organic soils by assembling a dataset based on field records of vegetation fires (both management fires and wildfires), some of which resulted in soil fires, while others did not. Our dataset has good spatial coverage (Fig. 1), and spans two decades (May 2001 to August 2021). We note that despite best efforts and close engagement with fire managers, practical constraints made this is a very incomplete record of fires within that period, and there are likely biases in the data that we discuss and propose ways of overcoming in the future. The incidence of soil fire is likely to be over-represented in the data, because it is easier to observe presence than absence of soil fire, and occurrence is also more likely to be reported. We discuss these problems and develop recommendations for future work.
(a) Locations of study sites (99 burnt, 43 unburnt) and extent of organic soil in Tasmania, Australia. The boundary of the Tasmanian Wilderness World Heritage Area is shown in blue. (b) Terrain map of Tasmania, with the 1000-mm isohyet shown in red (higher rainfall to the west and mountains in the north-east of the island).
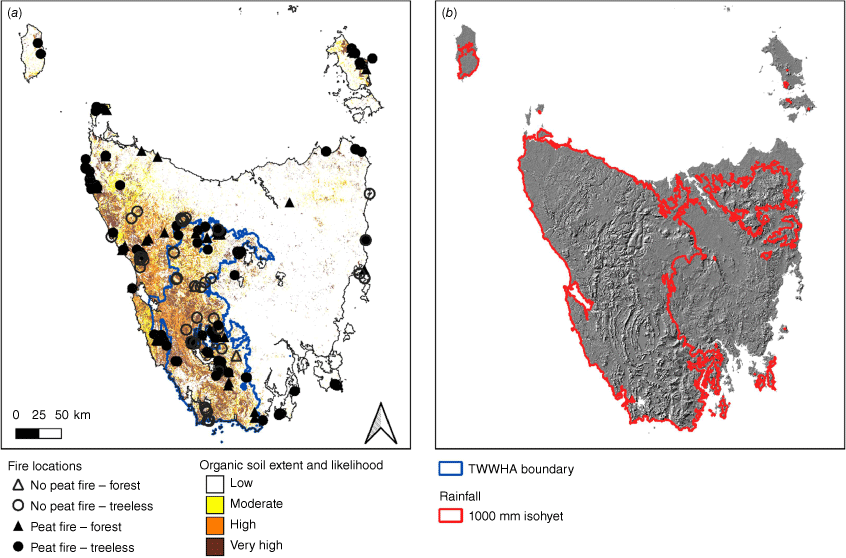
When land managers are planning fuel reduction burns, they are constrained not only by the risk of soil fires occurring, but also by whether the vegetation will carry a surface fire (Fig. 2). Globally, empirical research has found that the combustibility of forest vegetation can be predicted from the moisture content of dead fine fuels, which are composed of surface litter and other fine matter, and are readily ignitable (Ellis et al. 2022). The cumulative frequency of total area burnt in relation to fine fuel moisture content has been used to derive critical thresholds indicating high or extreme fire potential (Nolan et al. 2016; Ellis et al. 2022). While these thresholds are not appropriate to use for fuel reduction burns, we applied a similar approach to determine maximum fuel moisture thresholds indicating when vegetation is able to carry surface fire using available fire history data and associated estimates of fine fuel moisture using gridded climate data for Tasmania.
To identify the window for prescribed burning on organic soils across Tasmania, we: (1) investigated the probability of a vegetation fire igniting organic soils, in relation to modelled soil dryness, focussing on moorland sites. From this relationship, we proposed a ‘safe’ threshold, below which soils are unlikely to burn; (2) identified when vegetation is likely to be dry enough to carry fire, and examined the relationship between area burnt and estimated fine fuel moisture content; (3) produced maps of Tasmania showing the estimated number of days when vegetation fires were possible, and organic soils were unlikely to burn based on our selected ‘safe’ thresholds; and (4) discuss the assumptions and limitations associated with our results, and make suggestions for future improvements. In particular, we highlight the importance of comprehensively collecting and recording data regarding organic soil fires, including non-occurrence when these soils are exposed to vegetation fire. This is a vital step for adaptive management of fire on Tasmania’s organic soils.
Conceptual model showing probability of a flame starting a fire in organic soil (red) or vegetation (green) in relation to dryness. Management fires are usually planned to minimise the risk of organic soils catching fire (represented by red left-pointing arrow), yet must also be scheduled for when vegetation can carry fire (green right-pointing arrow), and are thus restricted to the narrow window (black double-headed arrow) when these conditions intersect, assuming the indicated probabilities for vegetation and soil fire. We assume a more conservative (lower) threshold for organic soil fire because the consequences of burning an organic soil are more serious than those of a management fire failing to ignite.
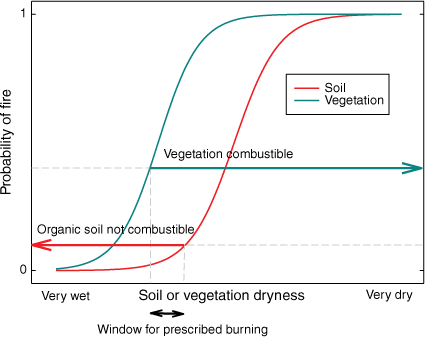
Materials and methods
Soil fire dataset
A soil fire history dataset was constructed based on areas known to have organic soils and to have carried vegetation fires (both prescribed fires and wildfires) (Fig. 1). We used a binary response variable to record whether a soil fire occurred (1) or not (0) after exposure to the vegetation fire. The fire data were based on field observations of soil fire by KS, reports from Parks and Wildlife Service (PWS) staff involved in fire management, and from aerial hotspot mapping where photographs of the hotspot clearly indicated soil was burning. Positive observations came from both prescribed burns and wildfires. Negative observations came largely from prescribed burns, where the generally smaller scale and active management of the fireground meant the absence of soil fire could be declared with some confidence. We acknowledge that this has likely led to biases in the dataset (discussed below) that have exaggerated soil fire risk in vegetation types subject only to wildfire, such as forest and scrub, and we therefore focus our analysis on the moorland sites.
The location of each fire was mapped and assigned a start date when the area was exposed to vegetation fire. This was derived from PWS records, or from fire boundary progression records collated by the Tasmanian Fire Service during bushfires. Where possible, it was cross checked with Sentinel satellite imagery (Sentinel Playground, https://apps.sentinel-hub.com/sentinel-playground, Sinergise Ltd.). Note that a single surface fire can be associated with numerous soil fires, sometimes starting on different days. These different soil fires were each recorded in the dataset if they were located in different grid cells used in the soil moisture models or started on different dates. The final soil fire dataset included 99 sites with burnt soil and 39 sites with unburnt soil (Fig. 1).
We classified the vegetation at all sites using remotely sensed imagery (see Supplementary Table S1), with our initial analyses comparing: (1) buttongrass moorland sites dominated by the sedge Gymnoschoenus sphaerocephalus; (2) scrub sites; and (3) forest sites including rainforest, wet eucalypt forest and woodland, dry eucalypt forest and woodland. Given that the most comprehensive recording of results from prescribed burns came from moorland sites, and that this is the vegetation type for which guidelines are most needed, our analyses and guidelines focus on the moorland sites.
Soil dryness index (SDI)
SDI was developed by Mount (1972) to estimate soil moisture depletion in the duff and upper soil layers and thereby predict fire danger under Tasmanian conditions (Kumar and Dharssi 2017). SDI ranges between 0 and 200, and indicates the quantity of rain (in mm) required to saturate the soil, so the higher the value, the dryer the soil. SDI considers interception and runoff separately as a function of vegetation categories that are based on forest and understorey densities (Kumar and Dharssi 2017). SDI was found to be superior to the widely-used Keech–Byram Dryness Index (KBDI) in capturing the soil water dynamics in southern Australia (Kumar and Dharssi 2017). SDI is used by Tasmanian fire management agencies to predict fire behaviour (Marsden-Smedley 2009), and is published daily by the Tasmanian Fire Service (https://www.fire.tas.gov.au/fire-danger-rating/sdi-map/).
The SDI data were supplied to us by the Australian Bureau of Meteorology, and covered the 6064 days between May 2001 and August 2021 inclusive, calculated on a 0.10° × 0.10° (approx. 10 km × 10 km) spatial grid. We used the data for the 138 grid cells in which organic soils were exposed to vegetation fire and where the soil response was known (soil fire present or absent).
Fine fuel moisture content and area burnt
Fine fuel moisture code (FFMC) is a component of the Canadian Fire Weather Index system (van Wagner 1987) that acts as a proxy for fuel moisture content percentage (FMC%), and can be transformed into the physical water content percentage (Wotton 2009):
Note that FMC%, like SDI, is predicted from weather observations, and is not a directly measured variable.
FFMC was calculated daily from the BARRA-TA downscaled reanalysis dataset (Su et al. 2021) for Tasmania at a 0.0135° resolution, which was available from 1990 through to 2018. We used 15:00 hours local time values for 2 m relative humidity, 2 m temperature, 10 m windspeed and 24-h precipitation accumulation in the calculation of FFMC, which was then converted to FMC%. Daily downscaled SDI values were also calculated from the BARRA-TA reanalysis grids for use in constructing state-wide maps of threshold exceedances, but these downscaled SDI values were not used to attribute individual peat fire records due to the lack of data post-2018, when most of the fires in our dataset occurred.
Burnt area data for Tasmania was obtained from the Tasmanian Fire Service and comprised primarily manually-mapped polygons of fire boundaries across Tasmania for the period covered by the BARRA-TA reanalysis data, with each polygon attributed with the date of fire ignition.
Probability of soil fire igniting when exposed to vegetation fire
To determine the probability of an organic soil burning if exposed to fire, we used binomial generalised linear modelling and the base package of the statistical software R (R Core Team 2023). We modelled the proportion of sites that burnt in relation to SDI for the entire dataset, using a binomial generalised linear model (logit link). We then compared buttongrass moorland sites with scrub and forest sites. A candidate set was constructed containing binomial generalised linear models (logit link) with all four combinations of SDI and VEG, excluding the interaction. AICc (Akaike’s Information Criterion modified for small sample size), which balances model fit with parsimony, was used to rank the models (Burnham and Anderson 2001). We focus on the results for the buttongrass moorland, which is most relevant for prescribed burning. Recognising the bias towards recording positive instances of organic soil fire, we also explored the relative likelihood of organic soil fire based only on positive observations of soil fire and the estimated SDI for their day and location, as well as the known distribution of SDI in our space-time domain. Details of this odds ratio analysis are presented in Supplementary Material S1.
Selection of ‘safe’ SDI threshold and combustible fuel moisture threshold
Selection of an SDI threshold for ‘safe’ burning on organic soils is arbitrary, but needs to balance the risk that soil fire will occur against the practicalities of being able to conduct prescribed burning.
We considered reasonable starting point was an SDI corresponding to a 5–20% probability of an organic soil fire occurring if exposed to vegetation fire. This range of probabilities was found for the model based on the buttongrass sites. For other vegetation types and for all sites combined, even at SDI of zero, the probability of soil fire exceeded this. We also evaluated the suitability of the SDI range (0–10, with an optimum of 5) recommended by Marsden-Smedley (2009) for prescribed burns in buttongrass moorland on organic soils.
We determined a threshold value of FMC% below which vegetation fire is possible by aggregating area of vegetation burnt across Tasmania, and the 25th percentile of FMC%, at monthly intervals between 1990 and 2018, within each 0.0135° meteorological grid cell. The data were limited to treeless vegetation, based on the findings reported above. A monthly aggregation period was selected to focus on the association of seasonal-scale fuel moisture trends with fire activity, and to account for the lack of completely precise fire start and end dates in the fire history dataset. The 25th percentile FMC% within each month for each cell was used to summarise each month as it reflects the drier end of conditions within the month, the period when fire is most likely to be burn. Cells were sorted by FMC% value, and the FMC% value below which 90% of total burnt area occurred (28.9%) was selected as a threshold value to define when vegetation fire is possible.
Map production
Across the available downscaled BARRA-TA reanalysis years (1990–2018), we calculated and plotted mean number of days per year below the identified SDI threshold, when peat fire is not likely.
For each spatial cell, we then calculated the number of days that were simultaneously below the identified ‘safe’ threshold SDI and drier than the threshold FMC% (vegetation combustible). From this we produced a map of mean number of days per year when vegetation can carry fire, yet organic soils are unlikely to ignite (‘safe, combustible days’). To explore the temporal variability in SDI, we also calculated the number of safe combustible days each year during the period 1990–2018 using downscaled grids for two sites representing the wet and dry extremes of sedgeland (treeless) vegetation in our dataset.
Results
Probability of soil fire and threshold selection
Our dataset showed organic soil fires were possible over the entire range of SDI values (Fig. 3), but soils were more likely to burn when at the drier end of their range (Fig. 4). The average SDI was 60 when soils ignited, compared to 15 when they did not, although there was substantial variation in SDI for both when organic soils ignited (1.5–141) or did not (0–88). Overall, 21% of vegetation fires on organic soil, but only 7% of soil fires, occurred on the 45% of days with SDI less than or equal to 5 (Fig. 5a). Similarly, only 12% of the soil fires and 28% of the vegetation fires occurred on the 55% of days when SDI <10. However, even at an SDI of zero, across all sites the probability of soil burning when exposed to vegetation fire was higher than our proposed 20% probability threshold.
Range in soil dryness index (SDI) at each site for all days between July 2010 and June 2015 (blue is minimum, grey maximum), compared to Day 0 of each vegetation fire where the soil (a) burned (red) or (b) did not burn (green). Data are sorted by minimum Day 0 SDI. Squares show moorland sites, circles other sites. Note that although there is a definite bias towards fire starting at high SDI, there are many exceptions. Conversely, most unburnt sites had low SDI, but there were sites with moderate SDI where soil fire did not occur.
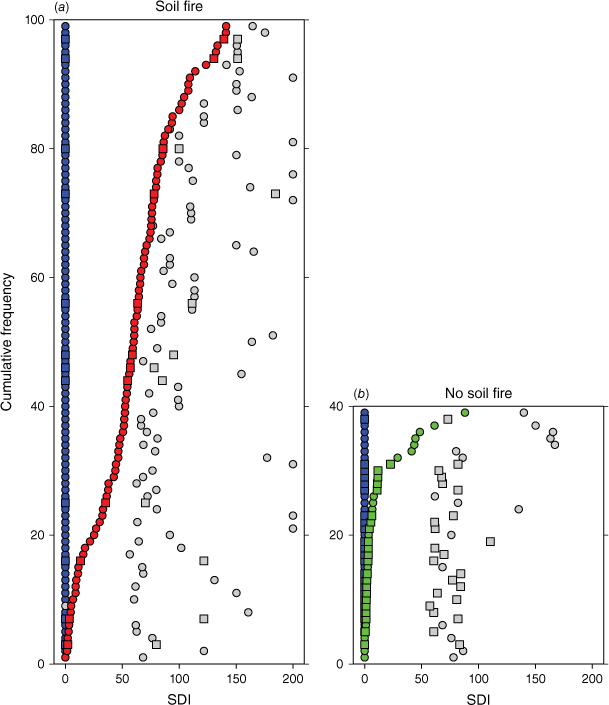
Count of sites exposed to vegetation fire according to soil dryness index (SDI), showing the counts of when fires did and did not occur (left hand panels). The corresponding proportion of those sites in which soil fires occurred is shown in the right-hand panels, with the lines indicating model predictions. Top row of panels show all sites, second row of panels show moorland sites, third row of panels show scrub sites, and bottom row of panels show forest sites. Note the different scales on the y-axes. For presentation, SDI was binned into 10-unit classes, with the average of each class used here, but actual data were used in the modelling.
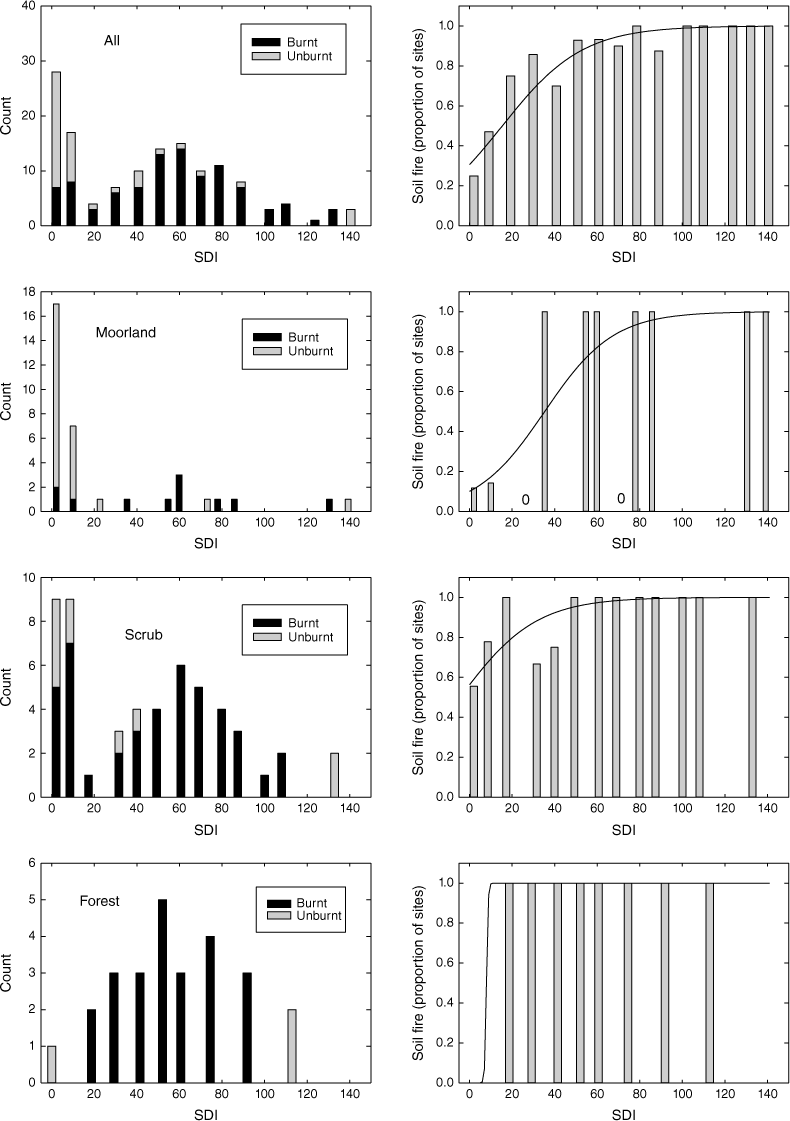
Cumulative relative frequency distribution of soil dryness index (SDI) for all days, vegetation fire days and soil fire days (a) across all sites, and (b) in moorland sites. Vegetation fire days, and especially soil fire days, are skewed towards higher SDI (drier soils).
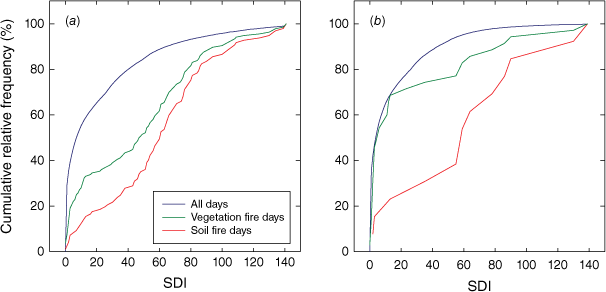
Twelve of 35 buttongrass moorland sites had soil fires, compared with 45 of 53 scrub sites and 25 of 26 forest sites. Modelling showed that in addition to SDI, vegetation (buttongrass moorland, scrub or forest) affected the probability of soil igniting if exposed to a vegetation fire (Table 1, Fig. 4, Fig. S1). The model that included both SDI and vegetation indicated soil fire was significantly more likely in scrub or forest than in buttongrass moorland when exposed to vegetation fire (Fig. 4). For buttongrass moorland (for which our dataset is most comprehensive), the probability of soil burning if exposed to vegetation fire was 10% (95% CI of 3–29%) at SDI of zero, 13% at SDI of 5 and 17% at SDI of 10. A 20% risk of soil fire was associated with an SDI of 12.9. At buttongrass moorland sites, SDI was below the existing recommended ‘optimum’ value of 5 for 54% of days, which included 49% of vegetation fires but only 9.6% of soil fires (Fig. 5b). Similarly, 65% of all days at our moorland sites have an SDI below 10, and 55% of vegetation fires but only 14% of soil fires occurred when SDI was below 10. Using the SDI value of 10 as the threshold, 80% of soil fire outcomes (positive and negative) were successfully predicted: soil fires occurred at two sites where it was predicted not to occur, and there was no soil fire at five sites where it was predicted (Table S2). We consider these results support the existing recommended SDI range of 0–10, with an optimum of 5, for planned burning in buttongrass moorlands.
Model | k | AICc | ΔAICc | w i | Explained deviance (%) | |
---|---|---|---|---|---|---|
SDI + VEG | 4 | 74.3 | 0.0 | 0.998 | 52.8 | |
SDI | 2 | 87.0 | 12.7 | 0.002 | 40.3 | |
VEG | 3 | 106.8 | 32.5 | 0.000 | 27.3 | |
Intercept only | 1 | 139.4 | 65.1 | 0.000 | NA |
k, number of parameters in the model; AICc, Akaike’s information criterion modified for small sample size; ΔAICc; difference in AICc relative to the best model; wi, Akaike weight for model i in the candidate set (these sum to 1), and deviance explained is relative to the intercept-only model.
Vegetation combustibility
The vegetation combustibility analysis showed that the area burnt was negligible until FMC% fell below 50%. It then increased gradually as FMC% declined further to about 25% (Fig. 6), and more rapidly below 25%. We considered vegetation to be combustible when FMC% fell below 28.9%, which corresponded to 90% of the total area burned.
Cumulative area burnt in relation to fine fuel moisture content (FMC%) for Tasmania between 1990 and 2022. The green vertical line indicates the value of fine fuel moisture content below which 90% of the total burnt area was burned; we chose to use this threshold of 28.9% to indicate when vegetation is combustible.
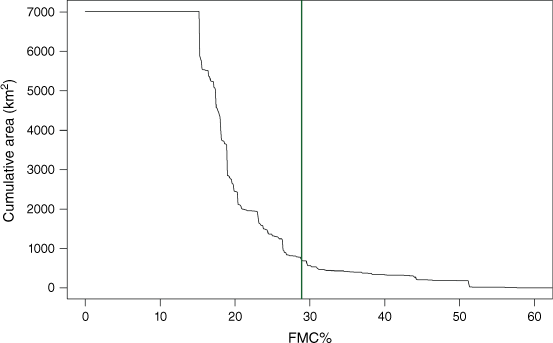
Maps of ‘safe’ and ‘safe combustible’ days
There was a strong east-west division in the average number of days per year below a ‘safe’ SDI threshold, with most of the west of the state having at least 200 days per year below an SDI of 5, compared with fewer than 64 days per year in much of the east (Fig. 7, Fig. S2). There was only a modest difference between a threshold of SDI 5 and 10 on the number of ‘safe’ days per year (Fig. 7, Fig. S3). However, screening out days when vegetation was not combustible altered this spatial pattern and greatly reduced the days suitable for prescribed burning (Figs. 7b, c). With an SDI threshold of 5, only a small area had more than nine suitable days on average each year, and mountainous areas in the west of the state, with shallow soils and high runoff, were rarely suitable. Safe prescribed burning was much more feasible with a threshold SDI of 10, with much of the state having more than 36 suitable days per year (Fig. 7, Fig. S3). The window for ‘safe’ prescribed burning varied considerably between years (Fig. 8). At one of the wetter buttongrass moorland sites, with an SDI threshold of 10, there were on average 53 safe combustible days per year, but this varied from 5 to 90 during the period 1990–2018 (Fig. 8b). By comparison, at one of the drier moorland sites, there were on average 26 safe combustible days per year, ranging from 14 to 39 days per year during this period.
Number of ‘safe’ days and ‘safe combustible’ days per year corresponding to soil dryness index (SDI) thresholds of 5 (considered optimal; top panels) and 10 (upper limit; lower panels). The risk of soil burning, if exposed to vegetation fire, was predicted to be 13% for SDI of 5, and 17% for SDI of 10. The corresponding ‘safe combustible’ days are those that are below the SDI threshold but fine fuels are dry enough to combust (considered to be FMC% <28.9%). Supplementary Figs S2 and S3 show the sensitivity of counts of ‘safe days’ and ‘safe combustible days’ to the selected SDI threshold.
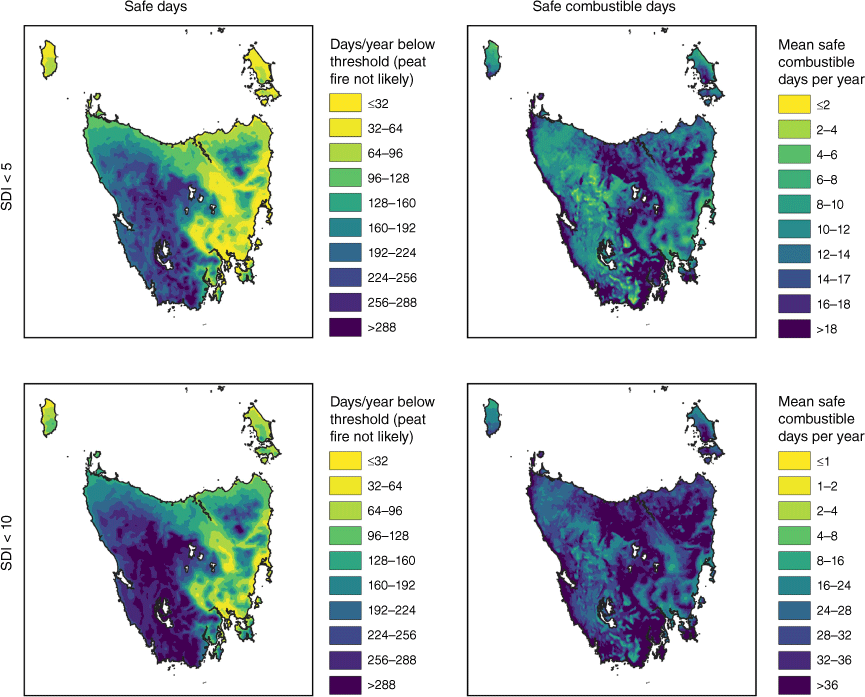
The number of ‘safe combustible’ days each calendar year between 1990 and 2018, for sedgeland at a dry site (Surv-1, in eastern Tasmania), and a wet site (Serp-2, in western Tasmania). The upper panel corresponds to a threshold soil dryness index (SDI) of 5, the lower panel to SDI of 10. Note the different scales on the y-axis.
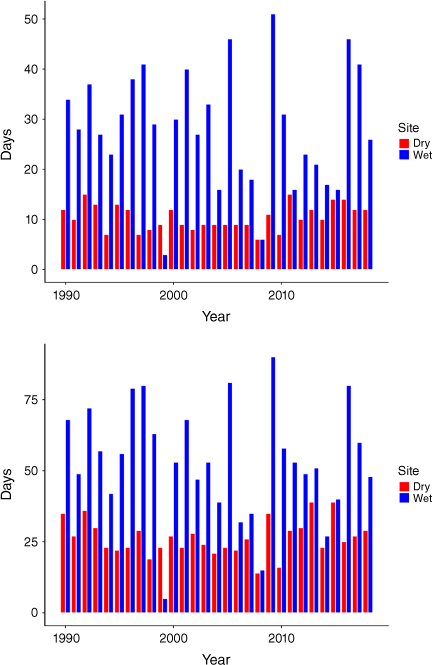
Discussion
Our overall aim was to develop a method to identify the window for prescribed burning on vegetation growing organic soils across Tasmania when these organic soils are unlikely to ignite. The most important finding of this study is that the safe window for prescribed burning on organic soils is limited. Our analysis highlights that burning vegetation on organic soils involves a difficult balancing act between vegetation combustibility and protecting organic soil from combustion. As we discuss below, there are caveats related to the quantity of, and probable bias in, the soil fire data and inaccuracies in the estimates of soil dryness and fine fuel moisture content at a coarse spatial scale. Nonetheless, our inference that burning vegetation on organic soils carries substantial risk is very likely to hold. Moreover, this risk is likely to increase due to anthropogenic climate change. Broadly, our findings harmonise with those of the laboratory-based study of Tasmanian organic soil combustion conducted by Prior et al. (2020).
Our work supports using the existing recommended guidelines that prescribed burning in buttongrass moorland vegetation should occur when SDI is between 0 and 10, and ideally around 5 (Marsden-Smedley 2009). At an SDI of 5, our binomial modelling showed that the risk of organic soil igniting was 13%, but this is likely an overestimate because of the dataset bias towards positive observations. We note that the number of ‘safe’ days per year was moderately sensitive to the specified SDI threshold (Fig. S2), but the number of ‘safe, combustible’ days was very sensitive to it (Fig. S3).
In buttongrass moorland, the estimated ‘safe combustible’ window ranged spatially from 8 to 35 days per year on average for an SDI threshold of 5, compared with 25–54 days for an SDI threshold of 10. While using an upper threshold of 10 offers more flexibility for conducting prescribed burns, it is associated with a slightly higher (17% vs 13%) risk of soil fire. We stress that these suggested SDI thresholds represent provisional guidelines only; managers should use their own experience of burning in this landscape, as well as prevailing weather conditions and direct measurement of soil moisture to make the final decision as to when and where to burn.
The vegetation combustibility constraint considerably narrowed the window for prescribed burning on organic soils. To indicate vegetation combustibility we selected an upper threshold of 28.9%, which is supported by Marsden-Smedley’s (1993) work showing a large increase in rate of spread of fire in buttongrass moorlands when moisture content of dead fuel dropped from 40 to 20%. The value of 28.9% is near the upper end of the 22–28% range considered to be very difficult to burn for eucalypt litter, and above that for grass to become flammable (Sullivan et al. 2012). It is therefore unlikely that the fine fuel moisture threshold could be substantially increased to widen the window for safe prescribed burning. The effects of climate change also need also be recognised; in western Tasmania there is a pronounced warming and drying trend apparent in the instrumental record with climate projections suggesting it is likely to increase the number of days when vegetation is combustible, but reduce the period when burning is safe (Grose et al. 2010; Fox-Hughes et al. 2014; Love et al. 2016). Additionally, marked interannual variation in ‘safe, combustible days’ means that prescribed burning should be avoided completely in particularly dry years. Rather than setting annual targets, fire management should be flexible, and focus on periods when conditions are safe for prescribed burning.
We acknowledge that the fires in our dataset are a very incomplete record of fires on organic soils in the last 20 years. They are necessarily biased towards prescribed burns and more accessible areas, but this is an advantage given the data will predominantly be used for planning management fires. Of more concern is that there is a likely bias towards vegetation fires that caused soil fires because it is easier to detect a soil fire than establish none occurred, and more likely that such a detection will be recalled and recorded. We stress that it is crucial to record negative observations: without them, predictions will always be biased towards higher probability of soil fire. For this reason, we advocate recording this information for every prescribed burn on organic soil.
There are many opportunities to refine the predictions presented here. First, we need more data on when soil fires do or do not occur to establish more reliable thresholds. While it is clear that when SDI is above about 40, soil fire is very likely, there is much less certainty for moister soil, when prescribed burns are scheduled. Therefore, more observations are required from days when SDI is in the low to moderate range. Second, higher spatial resolution of fires and burned soils will provide more accurate estimates of soil moisture at a given location, and potentially lead to more reliable predictions (Table S3). Fine scale spatial variation in soil moisture is likely responsible for apparently anomalous instances of soil fire occurring when SDI is 0; SDI is a coarse scale estimate and a value of 0 does not mean that soil is saturated across the entire landscape. Third, predictions are likely to be improved using a soil moisture index that better deals with the complex influences on soil hydrology. Selecting the optimal soil depth to use for moisture estimates is also likely to substantially improve predictions. Fourth, incorporating factors such as fire weather, landform, terrain and more detailed vegetation classes into the model will almost certainly improve predictions when these data are available, as they often will be in advance of prescribed burning. Compiling and analysing data from field measurements of soil (Prior et al. 2020) should complement the broad scale approach suggested here, Finally, the choice of threshold is ultimately arbitrary, although constrained by reality. In general, a conservatively low soil moisture threshold should be adopted to minimise risk of soil fire, while the choice of the FMC% threshold could be more flexible, because the consequences of vegetation not carrying fire are less serious.
Despite the limitations of our approach, this study, based on two decades of observations, represents a vital step for adaptive management of fire on Tasmania’s organic soils. We have demonstrated how simple records of date and location of vegetation fires can be used to estimate the risk of soil fire occurring. Refining the procedure described here will allow land managers to objectively plan the timing of prescribed burns on combustible organic soils, even in remote areas where access is difficult or expensive. By being aware of the associated risks, they can take precautions such as having adequate personnel and equipment available to quickly extinguish soil fires when they start. Additional data can be incorporated into the dataset and allow predictions to be more targeted to the conditions to which they will be applied.
Declaration of funding
This study received funding from the Tasmanian and Australian Governments through the Tasmanian Wilderness World Heritage Area funding arrangements.
Acknowledgements
Many highly knowledgeable staff from Parks and Wildlife Service and Tasmanian Fire Service provided data on dates and locations of soil fires they had fought. Steve Leonard had the idea of investigating the relationship between actual soil fires and modelled soil moisture. Paul Fox-Hughes, Vinod Kumar and Greg Kier of the Bureau of Meteorology assisted with the climate data. Jason Bradbury assisted greatly with data collation.
References
Bowman DMJS, Rodriguez-Cubillo D, Prior LD (2021) The 2016 Tasmanian Wilderness Fires: Fire Regime Shifts and Climate Change in a Gondwanan Biogeographic Refugium. In ‘Ecosystem Collapse and Climate Change’. (Eds JG Canadell, RB Jackson) pp. 133–153. (Springer International Publishing: Cham, Switzerland)
Burnham KP, Anderson DR (2001) Kullback-Leibler information as a basis for strong inference in ecological studies. Wildlife Research 28, 111-119.
| Crossref | Google Scholar |
Cotching WE, Lynch S, Kidd DB (2009) Dominant soil orders in Tasmania: distribution and selected properties. Australian Journal of Soil Research 47, 537-548.
| Crossref | Google Scholar |
di Folco M-B, Kirkpatrick JB (2011) Topographic variation in burning-induced loss of carbon from organic soils in Tasmanian moorlands. Catena 87, 216-225.
| Crossref | Google Scholar |
Ellis TM, Bowman DMJS, Jain P, Flannigan MD, Williamson GJ (2022) Global increase in wildfire risk due to climate-driven declines in fuel moisture. Global Change Biology 28, 1544-1559.
| Crossref | Google Scholar | PubMed |
Fox-Hughes P, Harris R, Lee G, Grose M, Bindoff N (2014) Future fire danger climatology for Tasmania, Australia, using a dynamically downscaled regional climate model. International Journal of Wildland Fire 23, 309-321.
| Crossref | Google Scholar |
Frandsen WH (1987) The influence of moisture and mineral soil on the combustion limits of smoldering forest duff. Canadian Journal of Forest Research 17, 1540-1544.
| Crossref | Google Scholar |
Frandsen WH (1997) Ignition probability of organic soils. Canadian Journal of Forest Research 27, 1471-1477.
| Crossref | Google Scholar |
French BJ, Prior LD, Williamson GJ, Bowman DMJS (2016) Cause and effects of a megafire in sedge-heathland in the Tasmanian temperate wilderness. Australian Journal of Botany 64, 513-525.
| Crossref | Google Scholar |
Garlough EC, Keyes CR (2011) Influences of moisture content, mineral content and bulk density on smouldering combustion of ponderosa pine duff mounds. International Journal of Wildland Fire 20, 589-596.
| Crossref | Google Scholar |
Goldstein A, Turner WR, Spawn SA, et al. (2020) Protecting irrecoverable carbon in Earth’s ecosystems. Nature Climate Change 10, 287-295.
| Crossref | Google Scholar |
Grose MR, Barnes-Keoghan I, Corney SP, White CJ, Holz GK, Bennett JB, Gaynor SM, Bindoff NL (2010) Climate futures for Tasmania: general climate impacts technical report. The Antarctic Climate & Ecosystems Cooperative Research Centre, Hobart, Tas. https://climatefutures.org.au/wp-content/uploads/2023/05/Climate-Futures-General-Climate-Impacts-Full-Report.pdf
Hill R (1982) Rainforest fire in western Tasmania. Australian Journal of Botany 30, 583-589.
| Crossref | Google Scholar |
Hokanson KJ, Lukenbach MC, Devito KJ, Kettridge N, Petrone RM, Waddington JM (2016) Groundwater connectivity controls peat burn severity in the boreal plains. Ecohydrology 9, 574-584.
| Crossref | Google Scholar |
Johnston FH, Henderson SB, Chen Y, Randerson JT, Marlier M, DeFries RS, Kinney P, Bowman DM, Brauer M (2012) Estimated global mortality attributable to smoke from landscape fires. Environmental Health Perspectives 120, 695-701.
| Crossref | Google Scholar | PubMed |
Lin SR, Sun PY, Huang XY (2019) Can peat soil support a flaming wildfire? International Journal of Wildland Fire 28, 601-613.
| Crossref | Google Scholar |
Lukenbach MC, Hokanson KJ, Moore PA, Devito KJ, Kettridge N, Thompson DK, Wotton BM, Petrone RM, Waddington JM (2015) Hydrological controls on deep burning in a northern forested peatland. Hydrological Processes 29, 4114-4124.
| Crossref | Google Scholar |
Nolan RH, Boer MM, de Dios VR, Caccamo G, Bradstock RA (2016) Large-scale, dynamic transformations in fuel moisture drive wildfire activity across southeastern Australia. Geophysical Research Letters 43, 4229-4238.
| Crossref | Google Scholar |
Prat-Guitart N, Rein G, Hadden RM, Belcher CM, Yearsley JM (2016) Propagation probability and spread rates of self-sustained smouldering fires under controlled moisture content and bulk density conditions. International Journal of Wildland Fire 25, 456-465.
| Crossref | Google Scholar |
Prior LD, French BJ, Storey K, Williamson GJ, Bowman DMJS (2020) Soil moisture thresholds for combustion of organic soils in western Tasmania. International Journal of Wildland Fire 29, 637-647.
| Crossref | Google Scholar |
Prior LD, Nichols SC, Williamson GJ, Bowman DMJS (2023) Post-fire restoration of Sphagnum bogs in the Tasmanian Wilderness World Heritage Area, Australia. Restoration Ecology 31, e13797.
| Crossref | Google Scholar |
Santoso MA, Christensen EG, Yang J, Rein G (2019) Review of the transition from smouldering to flaming combustion in wildfires. Frontiers in Mechanical Engineering 5, 49.
| Crossref | Google Scholar |
Su CH, Eizenberg N, Jakob D, Fox-Hughes P, Steinle P, White CJ, Franklin C (2021) BARRA v1.0: kilometre-scale downscaling of an Australian regional atmospheric reanalysis over four midlatitude domains. Geoscientific Model Development 14, 4357-4378.
| Crossref | Google Scholar |
Sullivan AL, McCaw WL, Cruz MG, Matthews S, Ellis PF (2012) Fuel, fire weather and fire behaviour in Australian ecosystems. In ‘Flammable Australia. Fire regimes, biodiversity and ecosystems in a changing world’. (Eds RA Bradstock, AM Gill, RJ Williams) pp. 51–78. (CSIRO Publishing: Melbourne, Vic., Australia)
Turetsky MR, Benscoter B, Page S, Rein G, van der Werf GR, Watts A (2015) Global vulnerability of peatlands to fire and carbon loss. Nature Geoscience 8, 11-14.
| Crossref | Google Scholar |
Whinam J, Hope G (2005) The peatlands of the Australasian region. Stapfia 85, 397-433.
| Google Scholar |
Wilson BR, Tulau M, Kuginis L, McInnes‐Clarke S, Grover S, Milford H, Jenkins BR (2022) Distribution, nature and threats to soils of the Australian Alps: a review. Austral Ecology 47, 166-188.
| Crossref | Google Scholar |
Wood SW, Hua Q, Bowman DMJS (2011) Fire-patterned vegetation and the development of organic soils in the lowland vegetation mosaics of south-west Tasmania. Australian Journal of Botany 59, 126-136.
| Crossref | Google Scholar |
Wotton BM (2009) Interpreting and using outputs from the Canadian Forest Fire Danger Rating System in research applications. Environmental and Ecological Statistics 16, 107-131.
| Crossref | Google Scholar |