Patterns of wildfire risk in the United States from systematic operational risk assessments: how risk is characterised by land managers
Erin Noonan-Wright A C * and Carl A. Seielstad B *A US Forest Service, Rocky Mountain Research Station, Wildland Fire Management Research Development and Application, 5775 W. Broadway St., Missoula, MT 59808, USA.
B National Center for Landscape Fire Analysis, University of Montana, CHCB 441, 32 Campus Drive, Missoula, MT 59812, USA.
C Corresponding author. Email: erin.noonan@usda.gov
International Journal of Wildland Fire 30(8) 569-584 https://doi.org/10.1071/WF21020
Submitted: 12 February 2021 Accepted: 3 May 2021 Published: 10 June 2021
Journal Compilation © IAWF 2021 Open Access CC BY-NC-ND
Abstract
Risk management is a significant part of federal wildland fire management in the USA because policy encourages the use of fire to maintain and restore ecosystems while protecting life and property. In this study, patterns of wildfire risk were explored from operational relative risk assessments (RRA) completed by land managers on 5087 wildfires from 2010 to 2017 in every geographic area of the USA. The RRA is the formal risk assessment used by land managers to develop strategies on emerging wildfires when concerns and issues related to wildfire management are in real-time. Only 38% of these wildfires were rated as high risk and 28% had high ratings for values at risk. Large regional variations were evident, with the West Coast regions selecting high risk and the South-west and Eastern regions selecting low risk. There were finer-scale influences on perceived risk when summarised on a jurisdictional level. Finally, risk summarised by USA agencies showed that the National Park Service and USDA Forest Service selected high risk less frequently compared with other agencies. By illuminating patterns of risk, this research intends to stimulate examination of the social, cultural, and physiographic factors influencing conceptions of risk.
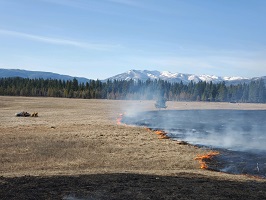
Keywords: geographic area, federal, fire management, relative risk assessment, Wildland Fire Decision Support System.
Introduction
Wildland fire, climate variability, people and vegetation have interacted over long time periods to produce vast fire-dependent ecosystems in the USA (Stewart 1951; Vale 2002; Whitlock et al. 2010; Marlon et al. 2012). Early in the 20th century, policy makers focused on the extraction of forest resources to fuel westward migration and implemented a fire policy that directed all fire ignitions to be extinguished by 10 a.m. the next morning (Loveridge 1944). By the 1960s, momentum was building to restore fire to some affected ecosystems, primarily in the National Park Service (NPS) lands followed later in USDA Forest Service (USFS) wilderness areas (van Wagtendonk 2007; Smith 2014). Iterations of the USA fire policy have since evolved to recognise fire as a ‘critical, natural process’, with the use of wildland fire as an important component of land management (Zimmerman and Bunnell 2000). In practice, using and suppressing fire together is complex and it has become more important to place fire management decision-making within a risk framework to ensure success and accountability.
Risk is the expectation of loss or benefit based on the probability and consequence of uncertain future events (Finney 2005; Ager et al. 2010; Calkin et al. 2010; Yoe 2011; Miller and Ager 2013; Scott et al. 2013; Thompson et al. 2016a). Although its definition is clear, risk is more difficult to articulate consistently when establishing strategies, goals and objectives for emerging wildfires, which can require quick decisions in chaotic environments. Consequently, it is not unusual for differences in the perception of risk and disparate risk management practices to exist among land managers, even for the same incidents (Thompson et al. 2016b).
Risk perceptions inherent in operational risk assessments are influenced by a multitude of factors, including assumptions, recent memories, quality, skill and bias associated with professional judgements, perceived affect and real risk (Tversky and Kahneman 1973; Alhakami and Slovic 1994; Sjöberg 2000; Kahneman and Klein 2009; Johnson-Laird 2010). These factors can lead to risk aversion in fire management decision-making, attributed to mental shortcuts developed during uncertain and conflicting decision environments (Maguire and Albright 2005). In one study, costly and risk-intolerant management strategies were favoured by fire managers given social and political constraints simulated in hypothetical scenarios (Calkin et al. 2013). Other studies have shown that risk-accepting behaviour is also present during wildfires. For instance, managers with extensive experience were more likely to identify long-term considerations as important and tended to support the use of wildfires in wilderness areas when selecting fire management strategies from hypothetical scenarios (Wilson et al. 2011), suggesting that some combination of experience, perspective and individual risk tolerance plays an important role in deciding whether to use wildland fire for ecological benefit. Similarly, a commitment to return fire to fire-adapted landscapes was the most significant factor influencing fire managers to use wildfire in a study by Williamson (2007).
Overcoming the potential constraints imposed by risk perception has manifested as solutions such as those proposed by Marcot et al. (2012), with formal procedures encompassing the four stages of structured decision-making, which can be used in an operational wildfire context (Taber et al. 2013) to facilitate strategic decisions that promote the use of fire. Ultimately, when the risk of fires interacting negatively with values at risk is recognised and mitigated consistently, it becomes possible to implement more strategies that allow fire to do its work, as sought in federal fire policy (Young et al. 2020).
In part to promote consistency in risk assessment in the USA, the Wildland Fire Decision Support System (WFDSS) incorporates a systematic operational risk assessment tool called the relative risk assessment (RRA) for emerging fires on federal lands (NIFC 2021). The RRA belongs to a collection of data, models and tools for evaluating expected fire behaviour, cost, damage and ecological benefit, among other things, in the context of guidance from legally binding land management plans (Calkin et al. 2011; Noonan-Wright et al. 2011; Pence and Zimmerman 2011; Zimmerman 2011; Zimmerman 2012). Development of the WFDSS and its RRA capitalised on the emergence of national-scale cadastral and critical infrastructure data in the USA, which together with spatial fire models, allowed managers to better quantify threats and hazards to values at risk. The WFDSS includes a formal decision process mandated for federal wildland fires that have exceeded the period of time considered to be the initial response (NIFC 2021). Some managers have used the WFDSS primarily to document decisions already made and others have found it useful for facilitating strategic, collaborative decision-making (Noble and Paveglio 2020; Rapp et al. 2020). Regardless of its perceived utility, the WFDSS has been an important step in advancing risk assessment of wildland fires in the USA (Finney 2005).
The RRA is a semiquantitative process enabling fire managers to assign relative rankings to risk elements using predetermined categories and terminology (Philpot et al. 1995; Thompson et al. 2016b). Each RRA includes high, moderate and low ratings for three elements (Values, Hazard and Probability) which are collectively integrated into an overall Relative Risk rating. The RRA elements are derived subjectively through deliberation by small groups of local decision-makers informed by observation, models and data. RRAs are real-time assessments of risk that guide planning and management of incidents and are one of three components used to evaluate incident complexity, in addition to identifying fire fighter safety issues and the appropriate incident management organisation (NWCG 2014). As such, they provide snapshots of how land managers, administrators and fire specialists with access to state-of-art data, models and analysis tools assess the risk of thousands of wildfires while they are burning. Over the life of an incident, many RRAs may be produced to reflect the risk of a dynamic fire environment. These assessments inform the fire management strategies outlined in the WFDSS decision signed by an agency administrator and because they document largely initial, subjective risk specific to an individual fire, they are termed ‘relative’ risk assessments (T. Zimmerman pers. comm.).
In this study, RRAs from completed assessments in the USA from 2010 to 2017 were examined to gain insights into the risk profiles shaping fire management decisions. The purpose of this research was to identify patterns in wildfire risk perceptions; specifically, risk evaluated at the national, regional and unit scales, as well as among the different land management agencies. The research focused exclusively on the RRA in the WFDSS and sought to answer the following questions:
-
What is the wildfire risk profile for long-duration, federal fires in the USA?
-
Does risk and the factors leading to it vary by geographic area (GA)?
-
Are differences in risk evident at scales finer than GA?
-
Do the federal land management agencies differ in selection of risk?
Methods
Data sources
The data used in this analysis was obtained from the WFDSS. In addition, the Fire Occurrence Database (FOD) was used to contextualise fires reported in the WFDSS relative to total fire load (Short 2017). Information regarding the incident management teams was summarised from the National Interagency Fire Center (NIFC) (NIFC 2017). The RRA consists of categorical data, with users selecting high, moderate, and low ratings for nine sub-elements to produce ratings for the main elements of Values (at risk), Hazard, Probability, and ultimately Relative Risk (Fig. 1, Table 1). Users also write qualitative justifications for each specific element. The ratings of each sub-element are integrated in graphical tables to assign ratings to each risk element (Values, Hazard, Probability). The final Relative Risk rating is then derived from the three main elements in a similar graphical table (Fig. 1).
![]() |
![]() |
The RRA is used for wildfires occurring in federal jurisdictions and by some states (New Mexico, Arizona and Alaska) and is targeted for emerging incidents that are expected to cause containment problems or burn for a long duration. For context, there were 616 032 wildfires in the USA from 2010 to 2017 (Short 2017). Of these, ~17% were fires on federal lands. Among federal fires, 4.9% resulted in publication of a risk assessment in the WFDSS. This analysis, then, provides insights into risk on mostly federal lands for wildfires that were expected to pose management challenges.
Data collection
The WFDSS is a J2EE, java server faces web application that integrates technologies to store, create, query and display geospatial and tabular data through the application server and other services (Calkin et al. 2011; Noonan-Wright et al. 2011). Data stored in the relational data stream management system were queried though the use of structured query language to link data tables and to extract information.
Duplicates and other anomalies with the data were remedied using CRAN – R (R Core Team 2019) and various packages to compute time (Grolemund and Wickham 2011), create and append data tables (Wickham 2007, 2014, 2016) and expedite processes (Bache and Wickham 2016). A total of 36 variables were extracted from the WFDSS, including numeric (mostly discrete) and categorical (a mixture of ordinal and nominal) data types. Qualitative notes were included in the RRA dataset as ‘_notes’ variables (Supplementary Table S1, available online), but not used explicitly for this analysis.
Because numerous RRAs can be completed during an incident, the first instance of the most frequently occurring Relative Risk rating was selected to represent the Relative Risk for that incident. In the case of incidents having only one high, moderate or low RRA, the first rating was chosen to represent that incident’s Relative Risk. This resulted in 5087 unique RRAs representing individual wildfires from 1 January 2010 to 31 December 2017. RRAs are published at inconsistent time intervals for a variety of reasons, such as a need to annotate the existing semiquantitative ratings, to polish a RRA for inclusion into the authoritative WFDSS decision or to reflect real changes in risk such as an increase in fire behaviour. Although the method of selection used in this research excluded some of the incident RRA data, it allowed for each incident to contribute equally, without giving preference to those fires with many published RRAs. For context, there were 12 324 risk assessments on the 5087 fires used in this analysis. There were 4.6% more high ratings, 3.6% fewer low ratings and a negligible difference in moderate ratings (<1%) among all risk assessments compared with the incident-specific selection used in this study.
Scales of analysis
Risk was first summarised nationally, then regionally (referred to as GA) and finally at the unit scale (e.g. individual national forests, field offices, refuges, reservations and national parks in the western USA). GA is a codified institutional level of organisation in which wildfires are prioritised and resources allocated (Fig. 2). An additional analysis was also completed at the agency-level to examine differences among the five major federal agencies responsible for managing land in the USA, including the Bureau of Land Management (BLM), USDA Forest Service (USFS), U.S. Fish and Wildlife Service (USFWS), the Bureau of Indian Affairs (BIA) and the National Park Service (NPS).
![]() |
Frequency analysis
Computing observed frequencies
The observed frequencies of high, moderate and low risk (expressed as percentages) were computed from counts of individual ratings for Relative Risk, the risk elements and the sub-elements. Counts were produced for 10 GAs and for the USA as a whole. Each tally was divided by the total for each respective GA to produce frequencies (expressed as percentages).
Computing expected frequencies from chance
The expected frequencies of high, moderate and low risk (expressed as percentages) were computed from all possible combinations of sub-element ratings (n = 32 805) for Hazard, Values, Probability and Relative Risk based on a random selection of risk ratings for each sub-element. Expected frequencies for sub-elements were one in three (33.3%) except for seasonal severity, which was one in five (20%). These frequencies are referred to as ‘% expected’ in the proceeding analyses and graphs.
Relative frequencies
Observed frequencies (% observed) were normalised to USA frequencies in order to express risk relative to the national picture (% observedU.S.). The selection metrics were computed to show the propensity to select specific ratings compared with the USA value:

where: = unit-less index showing the selection of ratings for each of the (i) GA compared with the percent observed frequencies for the collective USA;
. = percent observed frequencies for each (j) rating (high, moderate or low) by (k) Relative Risk, element, or sub-element;
= percent observed frequencies of the USA for each (j) rating (high, moderate or low) by (k) Relative Risk, element or sub-element.
In sum, the selection metric revealed a propensity for GAs to select specific ratings more or less relative to the USA. In the interest of simplifying the interpretation, 1 is subtracted from the relative frequency such that resulting negative values become the actual percentage difference between observed and expected; for example, –0.20 means that particular rating was chosen 20% less than the national percentage. For positive values, 1 is added back to the value and the interpretation is that a region used a particular risk level more than what was expected for the USA; for instance, a value of 2.5 indicates a 350% higher usage than expected.
Analysis techniques
Cluster analysis
Agglomerative hierarchical cluster analysis was performed on observed frequencies of risk for each GA and the USA to explore how GAs share or isolate selection of risk and its elements, with no scaling because the data were already scaled to percentages. Distance was measured as squared Euclidean distance using Ward’s method for a similarity metric, which evaluates an increase in the sum of squares as group membership changes. The ‘hclust’ function in CRAN – R was used to produce the dissimilarity matrices and clustering.
The observed frequencies of all 27 possible combinations of Values, Hazard and Probability were used as inputs. In addition, the frequencies of each sub-element combination were used to assess how GAs grouped based on the individual elements of Values, Hazard and Probability. For example, to cluster GAs for the Values element, observed frequencies for all combinations of high, moderate and low resources, threat and concern were used.
Location quotient
A spatial metric called the location quotient (Unwin 1981) was used to highlight concentrations of high Relative Risk by jurisdictional unit in the western USA to examine fine-scale patterns of Relative Risk. Unit refers to geographic management areas within agencies; for example, individual national forests, national parks, reservations and wildlife refuges. The location quotient shows geographic concentrations (e.g. Shaw and Wheeler 1985) and measures the extent to which different GAs depart from some norm (LQ > 1.0 depicts concentrations and vice versa). For this analysis, it depicted concentrations of high Relative Risk relative to the national occurrence of high Relative Risk. Each unit needed at least three incidents to be included in the analysis: 24 incidents on average were used to calculate the LQ at the unit level. LQ was defined as:
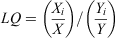
where Xi = frequency of high Relative Risk ratings, i, in any given jurisdiction or unit; X = sum of all high Relative Risk ratings in a GA; Yi = frequency of high Relative Risk ratings, i, at the national level; Y = sum of all the high Relative Risk ratings for the USA.
Results
What is the wildfire risk profile for long-duration, federal fires in the USA?
Nationally, a slight majority of fires (n = 1915, 38%) received a moderate Relative Risk rating, followed by high (n = 1913, 37.6%) and low (n = 1239, 24.4%). Land managers favoured moderate (52.1%) and high (30.2%) for the Probability element. They selected low (37.3%) and moderate (35.9%) for Values at risk. Hazard was the most symmetrical element, with a preference for moderate (41.6%) and roughly equal proportions of high and low (Fig. 3).
![]() |
Approximately 40% of fires were expected to have little effect on natural, cultural resources and infrastructure (resources) or few socioeconomic (concerns), while 25% were close to Values at risk (threat) and expected to reach them without mitigation (Fig. 4). Observed and expected fire behaviour was low to moderate for 84% of fires and most (78%) were expected to experience little to moderate fire growth and provide low to moderate resistance to fire control (potential). Barriers to fire spread were absent for 19% of fires, numerous for 31%, with ~80% having at least some barriers limiting fire spread. Individually, the sub-element frequencies illustrated moderate to low ratings roughly 80% of the time (on a per-sub-element basis).
![]() |
Does risk and the factors leading to it vary by GA?
General patterns emerged when risk was summarised by GA. The South-west and Eastern GAs selected low Relative Risk; the North-west, Northern California and to a lesser extent Southern California used high Relative Risk and the other regions fell in between (Fig. 5). These patterns were pronounced relative to the risk frequencies of the USA as a whole (Fig. 6). The Great Basin risk profile was essentially identical to the National (average) profile, followed closely by the Southern, Northern Rockies, and Rocky Mountain GAs. All of the regions used low Relative Risk more than expected (Table 2). The North-west and Northern California stood out as the only regions using high Relative Risk more than expected (Table 2, Fig. 5).
![]() |
![]() |
A dendrogram created by hierarchical clustering of the main risk elements produced four general risk groups consistent with the patterns described above (Fig. 7). GAs on the West Coast made up a high-risk group; South-west and Eastern formed a low-risk group; Rocky Mountain, Great Basin and Southern were a National Average group; and Alaska and Northern Rockies made up a National Average subgroup that tended to perceive somewhat lower Values at risk and higher Probabilities than the National Average group.
Clustering individually on risk elements revealed other differences. The Northern Rockies, Alaska and Eastern formed a low Value cluster. Northern California, the North-west and Southern California clustered separately as high Value. The rest of the GAs clustered in the middle (Fig. 7). Southern joined the high-risk group of Northern and Southern California and the North-west for Hazard. The remaining GAs clustered together in unique patterns: Rocky Mountain and Great Basin; USA and Alaska; Northern Rockies and South-west. Probability produced the familiar low-risk group of Eastern and South-west, a moderate probability group (Rocky Mountain, Great Basin, Southern, Alaska and the USA), and the Northern Rockies joined the high-risk group of the North-west, Northern and Southern California.
Generally, moderate to low ratings were selected by all GAs for all sub-elements (Fig. 8). Overall, natural-cultural-infrastructure (Values), socioeconomic concerns (Values), threat to (Values) and fire behaviour (Hazard) trended low compared with the other sub-elements. Some specific regional differences were also evident when the GAs were compared with the USA. For example, fuel condition (Hazard) posed an elevated risk in Northern California, Southern California, the North-west and Southern relative to the other GAs and fire was more proximate to (Values) in the two California regions and the North-west. Barriers limiting fire spread (Probability) were more prevalent in the South-west and Eastern and less so for about one-quarter of the fires in the North-west. Seasonal severity (Probability) was more often extreme and very high in Southern California, Northern California, Northern Rockies, North-west and arguably for the Eastern Region, which selected extreme seasonal severity more than any other GA. Almost half of South-west fires occurred late in the season (Probability), which is rarely the case for Alaska, and can occur throughout the fire season in the Southern and Eastern GAs. Potential for fire spread (Hazard) was comparatively low in Eastern, South-west and to a lesser extent Rocky Mountain and was high in North-west, Northern California, Alaska and the Northern Rockies. Socioeconomic concerns (Values) were lower in the South-west and Alaska and higher in Southern California and the North-west, while resource and infrastructure (Values) were lower in Alaska, Northern Rockies and Eastern and relatively high in Northern California and North-west. Southern California had fewer natural resource/infrastructure values and more socioeconomic concerns relative to Northern California.
Specific patterns belonging to individual GAs emerged from federal fire information contained in the WFDSS and provided context for the selection of risk ratings. Formal use of the risk assessment was highly variable among the GAs. Alaska and the Great Basin published risk assessments on 12.6% and 4.6% of wildfires, respectively, while half of the GAs produced risk assessments less than 1% of the time, suggesting that most wildfires were either not federal or easily extinguished. Wildfires with published risk assessments were generally longer in duration, with ~22 days between the start and containment. Alaska, the Northern Rockies and the North-west GAs had the longest duration fires, with the Eastern, Southern, Rocky Mountain and the Great Basin having the shortest (Table 3).
Utilisation of incident management teams (IMT) also varied by region. IMTs are generally used to manage longer duration fires that have a high probability of affecting values at risk, with a Type 1 IMT used for the most complex incidents (Table 3). Alaska and the Eastern GAs used the fewest number of Type 1 IMTs (n = 6 and 5, respectively), while the North-west and South-west used the most (n = 60 and 43, respectively). The North-west also used the most Type 2 IMTs (n = 228). A normalised look at IMT usages revealed that Eastern, Northern California, North-west, Southern California and Southern all exceeded 3 teams per 10 WFDSS fires (range 3.1–5.2). Many of these same GAs also demonstrated high resource capacity in addition to high IMT usage (Hand et al. 2017), which may be influenced by risk preference (Katuwal et al. 2017).
Are differences in wildfire risk evident at scales finer than the GA?
There is a geography to wildfire risk in the USA at the unit-scale that crosses GA boundaries (Fig. 9). The LQ depicts higher concentrations of the use of high Relative Risk, especially in the Great Basin and adjacent areas dominated by rangeland vegetation types. Higher risk was also evident for BLM and BIA/Tribal lands in the South-west (also dominated by rangeland fuels) relative to USFS forested lands. High risk was less prevalent (LQ < 1.0) in forested units dominated by the USFS (especially wilderness areas) and in NPS jurisdictions in all GAs.
![]() |
Do the federal land management agencies differ in risk selection?
The NPS used high Relative Risk infrequently compared with other agencies (Fig. 10) and the USFS also favoured low and moderate Relative Risk compared with other agencies. County and local, state and ‘other’ (primarily federal) jurisdictions invoked high Relative Risk and strongly limited low Relative Risk. Of the federal agencies, the BIA and the BLM used high Relative Risk most frequently. With the exception of the USFS and NPS, all agencies selected low Relative Risk less than the national value.
![]() |
Discussion
The concept of risk pervades fire decision-making in the USA, in large part because federal policy mandates consideration of the use of fire to maintain and restore ecosystems while simultaneously protecting values at risk, including life and property. Although life and private property are salient to all wildfire objectives, a patchwork of priorities for fire management exist, because the various missions of the federal agencies differentially emphasise protection versus ecosystem values. Almost by default, wildland fire management is set up to be complex in the USA, where many wildfires involve multiple ownerships or jurisdictions.
Despite this complexity, fire managers used the federally mandated the WFDSS risk assessment process on < 1% of wildfires occurring between 2010 and 2017 (5087 of 616 032), indicating that formal decision support is not used or needed for most wildfires. The remaining 99% of wildfires in the period were either not federal, were suppressed or went out on their own before a formal assessment was necessary. WFDSS fires lasted 20–30 days on average and more than 30% were shared between more than one jurisdiction, suggesting a degree of administrative complexity. WFDSS fires were also dominated by one agency, the USFS (~60%), indicating a strong influence of this agency on the data.
Notwithstanding the generally complicated nature of these extended attack wildfires represented in the WFDSS, most of the USA favoured low and moderate Relative Risk. The fact that a majority of these long-duration federal wildfires were not considered high risk may be due in part to an absence of values at risk in the areas where the fires occurred (e.g. Value ratings were consistently rated lower than other elements) (Fig. 3) or federal land managers were able to mitigate adverse wildfire effects. Less than 25% of these fires had a high rating for the Value sub-element (i.e. ‘proximity and threat of fire to values’), suggesting that the remaining wildfires either had multiple burn periods for a fire to reach values at risk or posed little threat at all (Fig. 4).
Patterns of risk
Nationally, 38% of long-duration, federal wildfires were rated as high risk, but, at finer scales, the GAs on the West Coast used high risk more than other GAs. These areas are at the epicentre of biophysical risk, with large populations living adjacent to highly flammable landscapes (Ager et al. 2013, 2019). In the North-west GA, close to 60% of WFDSS fires were high Relative Risk and > 30% of its fires involved multiple agencies. The region also used high Values, Hazard and Probability more than any other GA. In contrast, the South-west favoured low for fire behaviour (Hazard), resources, threat and concern (Values) and time of season (Probability), which translated into low ratings for all of the main risk elements, especially Values, and resulted in low and moderate risk for 76% of its fires. The South-west likely benefits from the occurrence of the North American Monsoon, which provides a semi-predictable end to its primary fire season during the month of July (Sheppard et al. 2002), and its flammable mountain ranges are usually isolated by inflammable deserts in all but the wettest of years. The scales of these temporal and spatial ‘barriers’ are fundamentally different from what occurs in the North-west GA, perhaps giving managers at least the perception of more certainty (and less risk) in the future of evolving wildfires, which is a topic for future exploration.
Patterns in wildfire risk were also evident at finer scales (Fig. 9) across GA boundaries. The LQ showed how some jurisdictions selected high risk more than the national value, along shared GA boundaries, such as between the Great Basin and North-west GAs. The role of more complex fire management considerations in these areas, such as rangeland vegetation, cattle grazing, fast-moving fires and the expansion of invasive annual grasses, managed primarily by the BLM, are worthy of further exploration. These traditional multi-use areas are dissected with range allotments for cattle grazing or private lands interspersed among federal lands, especially along historic railroad corridors where land was provided to corporations and states through land grants to encourage development and westward migration in the middle of the 19th century (Pacific Railroad Act 1862 – an act to aid in the construction of a railroad and telegraph line from the Missouri river to the Pacific ocean, and to secure to the government the use of the same for postal, military, and other purposes). Ecological and social challenges related to federal land use juxtaposed with the preservation of highly flammable sage grouse rangeland habitat, an indicator species of the sagebrush rangelands endemic to the Great Basin, may also be a factor (Wisdom and Chambers 2009; Shinneman et al. 2018).
The BIA lands are often associated with high Relative Risk. Many reservations are located in rangelands and drier forests, property is widely dispersed within them and there is often a reliance on timber and grazing assets to support local economies. Among all agencies, jurisdictions associated with dry forest types used high Relative Risk more than the national value, notably in the Eastern Cascade mountain range in Washington and the Southern Cascades in Oregon and California. These tendencies were different for the dry forests in the South-west, which used high Relative Risk less than the national average and are managed primarily by the USFS. Concentrations of high risk also occurred in national forests adjacent to communities, as seen in the Rocky Mountain and Northern Rockies GAs, where lower use of high risk was evident in backcountry areas and wilderness.
The different missions of land management agencies may also be contributing to patterns of risk. The NPS mission emphasises conservation, which perhaps favours greater risk tolerance in achieving natural outcomes. For instance, Crater Lake National Park located in the southern portion of the North-west GA and Lassen National Park in the north-east portion of the Northern California GA are islands of lower risk in relation to the national forests surrounding them (LQ < 0.50). Past fires in these areas may also contribute to perceptions of lower risk in the form of natural barriers to fire spread. Similar patterns emerged in the central Sierra Nevada mountains in California, especially the NPS jurisdictions of Sequoia-Kings Canyon and Yosemite National Parks, two of the original locations where prescribed natural fire was introduced in the late 1960s (van Wagtendonk 1995). Physiographic characteristics amenable to low risk (high-elevation, rocky terrain, moist forest types etc.) for some NPS units may also be a contributing factor to the less frequent use of high Relative Risk.
Similarly, USFS lands with a strong wilderness presence tended to use high risk less frequently. The Wilderness Act of 1964 designated wilderness as lands for protection and preservation in their natural condition, similar to the conservation emphasis of the NPS mission. The traditional use of wildfire to maintain natural conditions in wilderness areas may be contributing to the selection of less high risk, as seen in the Selway-Bitterroot and Frank Church wilderness (Great Basin GA) and the Bob Marshall, Great Bear and Scapegoat wilderness areas in the Northern Rockies (Dale 2006; Collins and Stephens 2007; Collins et al. 2009; Miller et al. 2012; Larson et al. 2013; Hunter et al. 2014; Parks et al. 2014; Boisramé et al. 2017).
Overall, the patterns of risk identified in this research were likely caused by a complex mix of biophysical factors, infrastructure and community development patterns, agency missions and regional fire culture. Direct causal explanations are obscured by the variability of these factors within GAs. Although it is likely that the fire environments of the North-west and Northern California tend to produce more higher risk fires than the South-west, due to higher density of Values at risk, proximity of fire to Values at risk, less certainty in future weather, longer duration events, higher fuel loads and more continuous fuels, it is also likely that cultural differences among fire managers play a role. In a previous study of USFS decision-makers (Cortner et al. 1990), patterns of risk tolerance were reported by GA, matching patterns found in our study. In this study, the South-west and Great Basin GAs were higher risk-takers while California and the Pacific North-west were more risk averse, consistently selecting low-risk/high-expense options from a range of hypothetical planning scenarios. Risk avoidance was influenced most strongly by safety, values/resources at risk, public opinion and the reliability of information. The commonality in patterns between those of Cortner et al. (1990) and ours suggests that some of the regional differences in risk perception identified almost 30 years ago may still persist.
A tendency when examining patterns of risk from operational assessments is to wonder what the real risk is. This tendency implies the existence of objective risk that managers might not know or do not use. We argue that the RRA is real risk because it is driving strategic responses on wildfires. We suspect that disparities exist between risk from the RRAs and the various quantitative wildfire risk assessments (QWRAs) used for land management planning and this is the subject of future research. Although risk has been assessed systematically at the national scale in the USA (Calkin et al. 2010; Scott et al. 2013; Ager et al. 2019), the QWRA is applicable at spatial and temporal scales that may be incompatible with the RRA and most useful during pre-fire planning phases when actual ignition locations are unknown. The QWRA applies burn probabilities simulated from random or historical ignition locations to estimate ranges of fire behaviour from historical weather and wind data; however, these estimates become less useful to address the finer-scale temporal or spatial fuels, weather and fire behaviour once an ignition location is known. The QWRA may also miss the breadth of Values at risk for a specific fire as discerned by a land manager in favour of spatially consistent cadastral records (Hollingsworth and Panunto 2018). Applying QWRA pre-fire planning information to evaluate real-time, operational wildfire risk in the RRA presents a challenge for future work and additional efforts to leverage risk management assistance products such as exceedance probability curves to help prioritise wildfires for multi-agency coordination groups during periods of heavy wildfire occurrence is a start to bridging quantitative and operational risk (Scott and Thompson 2015; Schultz et al. 2021). Ultimately, this equates to responding to wildfire ignitions using the best available information and tools that allow fire to be part of ecosystems while protecting life and property.
Conclusion
This research explored patterns of wildfire risk at different scales and among land management agencies. High risk was more prevalent in the western USA than any other region when incident-specific RRAs from the WFDSS were summarised from 2010 through 2017. High risk was also more common for the BIA, BLM, State, County/Local and other non-land management agencies (i.e. Department of Energy or Defence). In many locations across the USA there was a disparate use of high risk between adjacent jurisdictions, which warrants further exploration of the biophysical, cultural and other factors driving these differences between seemingly similar geographic locations.
The drivers of risk perceptions that manifest during wildfire events is a topic of further study and may help policy makers better allocate resources to address local-level fire management concerns. Some locations may benefit from increased support for working with communities to better prepare for fire (e.g. locations where both social concerns and resources and infrastructure are rated high). Other locations may benefit from closer examination of landscape barriers and how they spatially connect to create contingency lines (e.g. locations where barriers are rated as numerous or low). The influence of risk perceptions on the selection of fire management strategies also warrants further exploration to understand why some jurisdictions use a range of fire management strategies compared with a preference for suppression strategies. Managing naturally ignited wildfires to achieve ecological and restoration objectives is allowed for some federal lands and is part of the larger federal wildland fire management goal of increasing the resiliency of communities from wildfire (USDI/USDA 2014; Christiansen 2018).
By illuminating patterns of risk by GA, agency and unit, we sought to encourage discussion regarding how risk is characterised across the USA and where additional investments in tools and training might be targeted. This is the first formal summary of risk data housed in the WFDSS and although numerous DSS exist globally, the ability to summarise patterns of risk over a reasonably long period of time is unique to the WFDSS. Risk patterns can provide insights into the factors that are influencing local decision-making and may provide opportunities to directing resources and research to where it is needed most.
Conflict of interest
The authors declare no conflicts of interest.
Declaration of funding
Funding for this project provided by the Wildland Fire Management Research, Development and Application Group, Rocky Mountain Research Station, USA Forest Service and the National Center for Landscape Fire Analysis, W.A. Franke College of Forestry, University of Montana.
References
Ager AA, Finney MA, McMahan A, Cathcart J (2010) Measuring the effect of fuel treatments on forest carbon using landscape risk analysis. Natural Hazards and Earth System Sciences 10, 2515–2526.| Measuring the effect of fuel treatments on forest carbon using landscape risk analysis.Crossref | GoogleScholarGoogle Scholar |
Ager AA, Buonopane M, Reger A, Finney MA (2013) Wildfire exposure analysis on the national forests in the Pacific Northwest, USA. Risk Analysis 33, 1000–1020.
| Wildfire exposure analysis on the national forests in the Pacific Northwest, USA.Crossref | GoogleScholarGoogle Scholar | 23078351PubMed |
Ager AA, Day MA, Palaiologou P, Houtman RM, Ringo C, Evers CR (2019) Cross-boundary wildfire and community exposure: A framework and application in the western U.S. USDA Forest Service, Rocky Mountain Research Station, General Technical Report RMRS-GTR-392. (Fort Collins, CO)
Alhakami AS, Slovic P (1994) A Psychological Study of the Inverse Relationship Between Perceived Risk and Perceived Benefit. Risk Analysis 14, 1085–1096.
| A Psychological Study of the Inverse Relationship Between Perceived Risk and Perceived Benefit.Crossref | GoogleScholarGoogle Scholar | 7846317PubMed |
Bache, S, Wickham, H (2016) ‘Package “magrittr”.’ Available at https://CRAN.R-project.org/package=magrittr
Boisramé G, Thompson S, Collins B, Stephens S (2017) Managed Wildfire Effects on Forest Resilience and Water in the Sierra Nevada. Ecosystems 20, 717–732.
| Managed Wildfire Effects on Forest Resilience and Water in the Sierra Nevada.Crossref | GoogleScholarGoogle Scholar |
Calkin DE, Ager AA, Gilbertson-Day J (Eds) (2010) Wildfire risk and hazard: procedures for the first approximation. USDA Forest Service, Rocky Mountain Research Station, General Technical Report RMRS-GTR-235. (Fort Collins, CO)
Calkin DE, Thompson MP, Finney MA, Hyde KD (2011) A Real-Time Risk Assessment Tool Supporting Wildland Fire Decisionmaking. Journal of Forestry 109, 274–280.
Calkin DE, Venn T, Wibbenmeyer M, Thompson MP (2013) Estimating US federal wildland fire managers? preferences toward competing strategic suppression objectives. International Journal of Wildland Fire 22, 212–222.
| Estimating US federal wildland fire managers? preferences toward competing strategic suppression objectives.Crossref | GoogleScholarGoogle Scholar |
Christiansen V (2018) Collaboration Across Boundaries: A Policy Perspective on the State of Wildland Fire. Fire Management Today 76, 38–43.
Collins BM, Stephens SL (2007) Managing natural wildfires in Sierra Nevada wilderness areas. Frontiers in Ecology and the Environment 5, 523–527.
| Managing natural wildfires in Sierra Nevada wilderness areas.Crossref | GoogleScholarGoogle Scholar |
Collins BM, Miller JD, Thode AE, Kelly M, van Wagtendonk JW, Stephens SL (2009) Interactions Among Wildland Fires in a Long-Established Sierra Nevada Natural Fire Area. Ecosystems 12, 114–128.
| Interactions Among Wildland Fires in a Long-Established Sierra Nevada Natural Fire Area.Crossref | GoogleScholarGoogle Scholar |
Cortner HJ, Taylor JG, Carpenter EH, Cleaves DA (1990) Factors Influencing Forest Service Fire Managers’ Risk Behavior. Forest Science 36, 531–548.
Dale L (2006) Wildfire Policy and Fire Use on Public Lands in the United States. Society & Natural Resources 19, 275–284.
| Wildfire Policy and Fire Use on Public Lands in the United States.Crossref | GoogleScholarGoogle Scholar |
Finney MA (2005) The challenge of quantitative risk analysis for wildland fire. Forest Ecology and Management 211, 97–108.
| The challenge of quantitative risk analysis for wildland fire.Crossref | GoogleScholarGoogle Scholar |
Grolemund G, Wickham H (2011) Dates and Times Made Easy with lubridate. Journal of Statistical Software 1, 2–25.
Hand M, Katuwal H, Calkin DE, Thompson MP (2017) The influence of incident management teams on the deployment of wildfire suppression resources. International Journal of Wildland Fire 26, 615–629.
| The influence of incident management teams on the deployment of wildfire suppression resources.Crossref | GoogleScholarGoogle Scholar |
Hollingsworth LT, Panunto MH (2018) Assessing wildfire risk in real time on the 2017 Frye Fire. In ‘The Fire Continuum Conference: Preparing for the Future of Wildland Fire; 21–24 May 2018. Missoula, MT’. (Ed. R Keane) Volume Proceedings RMRS-P-78. pp. 358 (USDA Forest Service, Rocky Mountain Research Station: Fort Collins, CO)
Hunter M, Iniguez J, Farris C (2014) Historical and Current Fire Management Practices in Two Wilderness Areas in the Southwestern United States: The Saguaro Wilderness Area and the Gila-Aldo Leopold Wilderness Complex. USDA Forest Service, Rocky Mountain Research Station, General Technical Report RMRS-GTR. (Fort Collins, CO)
Johnson-Laird PN (2010) Mental models and human reasoning. Proceedings of the National Academy of Sciences of the United States of America 107, 18243–18250.
| Mental models and human reasoning.Crossref | GoogleScholarGoogle Scholar | 20956326PubMed |
Kahneman D, Klein G (2009) Conditions for intuitive expertise: a failure to disagree. The American Psychologist 64, 515–526.
| Conditions for intuitive expertise: a failure to disagree.Crossref | GoogleScholarGoogle Scholar | 19739881PubMed |
Katuwal H, Dunn CJ, Calkin DE (2017) Characterising resource use and potential inefficiencies during large-fire suppression in the western US. International Journal of Wildland Fire 26, 604–614.
| Characterising resource use and potential inefficiencies during large-fire suppression in the western US.Crossref | GoogleScholarGoogle Scholar |
Larson AJ, Belote RT, Cansler CA, Parks SA, Dietz MS (2013) Latent resilience in ponderosa pine forest: effects of resumed frequent fire. Ecological Applications 23, 1243–1249.
| Latent resilience in ponderosa pine forest: effects of resumed frequent fire.Crossref | GoogleScholarGoogle Scholar | 24147398PubMed |
Loveridge EW (1944) The Fire Suppression Policy of the U.S. Forest Service. Journal of Forestry 42, 549–554.
Maguire LA, Albright EA (2005) Can behavioral decision theory explain risk-averse fire management decisions? Forest Ecology and Management 211, 47–58.
| Can behavioral decision theory explain risk-averse fire management decisions?Crossref | GoogleScholarGoogle Scholar |
Marcot BG, Thompson MP, Runge MC, Thompson FR, McNulty S, Cleaves D, Tomosy M, Fisher LA, Bliss A (2012) Recent advances in applying decision science to managing national forests. Forest Ecology and Management 285, 123–132.
| Recent advances in applying decision science to managing national forests.Crossref | GoogleScholarGoogle Scholar |
Marlon JR, Bartlein PJ, Gavin DG, Long CJ, Anderson RS, Briles CE, Brown KJ, Colombaroli D, Hallett DJ, Power MJ, Scharf EA, Walsh MK (2012) Long-term perspective on wildfires in the western USA. Proceedings of the National Academy of Sciences of the United States of America 109, E535–E543.
| Long-term perspective on wildfires in the western USA.Crossref | GoogleScholarGoogle Scholar | 22334650PubMed |
Miller C, Ager AA (2013) A review of recent advances in risk analysis for wildfire management. International Journal of Wildland Fire 22, 1–14.
| A review of recent advances in risk analysis for wildfire management.Crossref | GoogleScholarGoogle Scholar |
Miller JD, Collins BM, Lutz JA, Stephens SL, van Wagtendonk JW, Yasuda DA (2012) Differences in wildfires among ecoregions and land management agencies in the Sierra Nevada region, California, USA. Ecosphere 3, 80
| Differences in wildfires among ecoregions and land management agencies in the Sierra Nevada region, California, USA.Crossref | GoogleScholarGoogle Scholar |
NIFC (2000) ‘Cerro Grande Prescribed Fire Investigation Report’. Available at https://www.nwcg.gov/sites/default/files/wfldp/docs/sr-cg-cerro-grande-investigation-report-may-2000.pdf [Accessed 28 May 2021].
NIFC (2017) ‘Wildland Fire Summaries.’ National Interagency Fire Center. Available at https://www.predictiveservices.nifc.gov/intelligence/2017_statssumm/2017Stats&Summ.html [Accessed 28 May 2021].
NIFC (2021) ‘Red Book 2021: Interagency Standards for Fire and Fire Aviation Operations.’ National Interagency Fire Center. Available at https://www.nifc.gov/standards/guides/red-book [Accessed 28 May 2021].
Noble P, Paveglio TB (2020) Exploring Adoption of the Wildland Fire Decision Support System: End User Perspectives. Journal of Forestry 118, 154–171.
| Exploring Adoption of the Wildland Fire Decision Support System: End User Perspectives.Crossref | GoogleScholarGoogle Scholar |
Noonan-Wright EK, Opperman TS, Finney MA, Zimmerman GT, Seli RC, Elenz LM, Calkin DE, Fiedler JR (2011) Developing the US Wildland Fire Decision Support System. Journal of Combustion 2011, 1–14.
| Developing the US Wildland Fire Decision Support System.Crossref | GoogleScholarGoogle Scholar |
NWCG (2014) ‘Risk and Complexity Analysis, PMS-210.’ National Wildfire Coordinating Group. Available at https://www.nwcg.gov/sites/default/files/publications/pms210_rca.pdf [Accessed 28 May 2021].
Parks SA, Miller C, Nelson CR, Holden ZA (2014) Previous Fires Moderate Burn Severity of Subsequent Wildland Fires in Two Large Western US Wilderness Areas. Ecosystems 17, 29–42.
| Previous Fires Moderate Burn Severity of Subsequent Wildland Fires in Two Large Western US Wilderness Areas.Crossref | GoogleScholarGoogle Scholar |
Pence M, Zimmerman GT (2011) The Wildland Fire Decision Support System: Integrating Science, Technology, and Fire Management. Fire Management Today 71, 18–22.
Philpot C, Schechter C, Bartuska A, Beartusk K, Bosworth D, Coloff S, Douglas J, Edrington M, Gale R, Lavin MJ, Rosenkrance LK, Streeter R, van Wagtendonk J (1995) Federal Wildland Fire Management Policy & Program Review. Report (U.S. Dept. of the Interior; U.S. Dept. of Agriculture) Available at http://pubs.er.usgs.gov/publication/96587.
R Core Team (2019) ‘R: A Language and Environment for Statistical Computing.’ (R Foundation for Statistical Computing: Vienna, Austria)
Rapp C, Rabung E, Wilson R, Toman E (2020) Wildfire decision support tools: an exploratory study of use in the United States. International Journal of Wildland Fire 29, 581–594.
| Wildfire decision support tools: an exploratory study of use in the United States.Crossref | GoogleScholarGoogle Scholar |
Schultz CA, Miller LF, Greiner SM, Kooistra C (2021) A Qualitative Study on the US Forest Service’s Risk Management Assistance Efforts to Improve Wildfire Decision-Making. Forests 12, 344
| A Qualitative Study on the US Forest Service’s Risk Management Assistance Efforts to Improve Wildfire Decision-Making.Crossref | GoogleScholarGoogle Scholar |
Scott JH, Thompson MH (2015) Emerging Concepts in Wildfire Risk Assessment and Management. In ‘Proceedings of the large wildland fires conference, May 19–23, 2014. Missoula, MT’. (Eds RE Keane, M Jolly, R Parsons, K Riley) Volume RMRS-P-73, pp. 196–206. (USDA Forest Service, Rocky Mountain Research Station: Fort Collins, CO)
Scott JH, Thompson MP, Calkin DE (2013) A wildfire risk assessment framework for land and resource management. USDA Forest Service, Rocky Mountain Research Station, General Technical Report RMRS-GTR-315. (Fort Collins, CO)
Shaw, G, Wheeler, D (1985) ‘Statistical Techniques in Geographical Analysis.’ (John Wiley & Sons: New York, NY)
Sheppard PR, Comrie AC, Packin GD, Angersbach K, Hughes MK (2002) The climate of the US Southwest. Climate Research 21, 219–238.
| The climate of the US Southwest.Crossref | GoogleScholarGoogle Scholar |
Shinneman DJ, Aldridge CL, Coates PS, Germino MJ, Pilliod DS, Vaillant NM (2018) A Conservation Paradox in the Great Basin—Altering Sagebrush Landscapes with Fuel Breaks to Reduce Habitat Loss from Wildfire. No. Open-File Report 2018-1034. (US Geological Survey, Reston, VA)
Short KC (2017) ‘Spatial wildfire occurrence data for the United States, 1992–2015 [FPA_FOD_20170508].’ (Forest Service Research Data Archive, Fort Collins, CO)
Sjöberg L (2000) Factors in Risk Perception. Risk Analysis 20, 1–11.
| Factors in Risk Perception.Crossref | GoogleScholarGoogle Scholar | 10841699PubMed |
Smith, D (2014) From Research to Policy: The White Cap Wilderness Fire Study. Forest History Today Spring/Fall, 4–12.
Stewart OC (1951) Burning and Natural Vegetation in the United States. Geographical Review 41, 317–320.
| Burning and Natural Vegetation in the United States.Crossref | GoogleScholarGoogle Scholar |
Taber MA, Elenz LM, Langowski PG (2013) Decision Making for Wildfires: A Guide for Applying a Risk Management Process at the Incident Level. USDA Forest Service, Rocky Mountain Research Station, General Technical Report RMRS-GTR-298WWW. (Fort Collins, CO)
Thompson MP, Gilbertson-Day JW, Scott JH (2016a) Integrating Pixel- and Polygon-Based Approaches to Wildfire Risk Assessment: Application to a High-Value Watershed on the Pike and San Isabel National Forests, Colorado, USA. Environmental Modeling and Assessment 21, 1–15.
| Integrating Pixel- and Polygon-Based Approaches to Wildfire Risk Assessment: Application to a High-Value Watershed on the Pike and San Isabel National Forests, Colorado, USA.Crossref | GoogleScholarGoogle Scholar |
Thompson MP, Zimmerman TG, Mindar D, Taber M (2016b) Risk terminology primer: Basic principles and a glossary for the wildland fire management community. USDA Forest Service, Rocky Mountain Research Station, General Technical Report RMRS-GTR-349. (Fort Collins, CO)
Tversky A, Kahneman D (1973) Availability: A heuristic for judging frequency and probability. Cognitive Psychology 5, 207–232.
| Availability: A heuristic for judging frequency and probability.Crossref | GoogleScholarGoogle Scholar |
Unwin, DJ (1981) ‘Introductory spatial analysis.’ (Methuen: London)
USDI/USDA (1998) Wildland and prescribed fire management policy, implementation procedures reference guide. National Park Service, USDA Forest Service, Bureau of Indian Affairs, U.S. Fish and Wildlife Service, and Bureau of Land Management, Boise, ID. 190 p.
USDI/USDA (2014) ‘The National Strategy: The final phase in the development of the National Cohesive Wildland Fire Management Strategy.’ (US Department of Agriculture and US Department of Interior: Washington, D.C., USA).
Vale T (2002) ‘Fire, Native Peoples, and the Natural Landscape.’ (Island Press: Washington, United States)
van Wagtendonk JW (1995) ‘Dr. Biswell’s Influence on the Development of Prescribed Burning in California, The Biswell Symposium: Fire Issues and Solutions in Urban Interface and Wildland Ecosystems.’ Walnut Creek, CA, February 15–17, 1994. (USDA Forest Service)
van Wagtendonk JW (2007) The History and Evolution of Wildland Fire Use. Fire Ecology 3, 3–17.
| The History and Evolution of Wildland Fire Use.Crossref | GoogleScholarGoogle Scholar |
Whitlock C, Higuera P, McWethy D, Briles C (2010) Paleoecological Perspectives on Fire Ecology: Revisiting the Fire-Regime Concept. The Open Ecology Journal 3, 6–23.
| Paleoecological Perspectives on Fire Ecology: Revisiting the Fire-Regime Concept.Crossref | GoogleScholarGoogle Scholar |
Wickham H (2007) Reshaping data with the reshape package. Journal of Statistical Software 21, 1–20.
| Reshaping data with the reshape package.Crossref | GoogleScholarGoogle Scholar |
Wickham H (2014) Tidy data. Journal of Statistical Software 59, 1–24.
| Tidy data.Crossref | GoogleScholarGoogle Scholar |
Wickham H (2016) ‘ggplot2: Elegant Graphics for Data Analysis.’ (Springer-Verlag: New York, NY)
Williamson MA (2007) Factors in United States Forest Service district rangers’ decision to manage a fire for resource benefit. International Journal of Wildland Fire 16, 755–762.
| Factors in United States Forest Service district rangers’ decision to manage a fire for resource benefit.Crossref | GoogleScholarGoogle Scholar |
Wilson RS, Winter PL, Maguire LA, Ascher T (2011) Managing wildfire events: risk-based decision making among a group of federal fire managers. Risk Analysis 31, 805–818.
| Managing wildfire events: risk-based decision making among a group of federal fire managers.Crossref | GoogleScholarGoogle Scholar | 21143258PubMed |
Wisdom MJ, Chambers JC (2009) A Landscape Approach for Ecologically Based Management of Great Basin Shrublands. Restoration Ecology 17, 740–749.
| A Landscape Approach for Ecologically Based Management of Great Basin Shrublands.Crossref | GoogleScholarGoogle Scholar |
Yoe C (2011) ‘Primer on risk analysis: Decision making under uncertainty.’ (CRC Press: Boca Raton, FL)
Young JD, Evans AM, Iniguez JM, Thode A, Meyer MD, Hedwall SJ, McCaffrey S, Shin P, Huang C-H (2020) Effects of policy change on wildland fire management strategies: evidence for a paradigm shift in the western US? International Journal of Wildland Fire 29, 857–877.
| Effects of policy change on wildland fire management strategies: evidence for a paradigm shift in the western US?Crossref | GoogleScholarGoogle Scholar |
Zimmerman GT (2011) Fire Science Application and Integration in Support of Decision Making. In ‘Proceedings of the 5th International Wildland Fire Conference’, Sun City, South Africa, 9–13 May 2011. Available at https://www.fs.usda.gov/treesearch/pubs/39278
Zimmerman GT (2012) Wildland Fire Management Decision Making. Journal of Agricultural Science and Technology B 2, 169–178.
Zimmerman GT, Bunnell DL (2000) The Federal Wildland Fire Policy: Opportunities for Wilderness Fire Management. In ‘Wilderness Science in a Time of Change.’ Missoula, MT. (Eds DN Cole, SF McCool, WT Borrie, J O’Laughlin) pp. 288–297. (USDA Forest Service, Rocky Mountain Research Station: Ogden, UT)
* Contributed equally to this paper.