Organic amendments improved soil properties and native plants’ performance in an Australian degraded land
Jonas Larsen A , Mehran Rezaei Rashti

A
B
C
D
Abstract
Land degradation poses a substantial threat to both the sustainable environment and human health. Efforts towards rehabilitation and remediation often require addition of soil amendments and careful selection of plant species.
We assessed the effect of recycled organic amendments on improvement of soil physicochemical properties and performance of native plant species in an Australian degraded soil.
A glasshouse pot experiment investigated the effects of compost (CO), biochar (BC), and compost-biochar (COBC) mixture on performance of three native Australian plant species (Eucalyptus tereticornis (EU), Acacia leiocalyx (AC), and Banksia integrifolia (BA)) in a degraded soil.
Application of CO, BC, and COBC organic amendments increased soil dissolved organic carbon and microbial biomass carbon contents compared to the control treatment. COBC amendment increased nutrient retention and reduced CO2 emissions compared to CO amendment. BC amendment also resulted in low CO2 emissions similar to the control treatment, where no significant differences were observed. AC outperformed the EU and BA species in biomass production due to its leguminous nature, with amendment application had an insignificant effect on AC performance. Within the EU treatments, the COBC:EU demonstrated the highest biomass production, followed by CO:EU, BC:EU, and CK:EU, respectively.
All amendments exhibited overall improvements in soil and plant parameters, with more significant outcomes observed with COBC application. However, the observed improvements from biochar application were minimal in this short-term experiment, which may not have allowed for the manifestation of long-term benefits.
Further research is warranted to investigate the effects of compost and biochar amendments on diverse soil types and native plant species.
Keywords: Acacia leiocalyx, Australian native plants, Banksia integrifolia, biochar, compost, degraded land, Eucalyptus tereticornis, organic amendments.
Introduction
Terrestrial life is intricately interconnected with the abundant resources provided by land, such as soil, water, and various ecosystem services. However, anthropogenic activities have led to significant land degradation, compromising its capacity to support life (Prăvălie 2021). Once initiated, land degradation can have detrimental effects on environmental processes, the functionality of surrounding ecosystems, economies, and human wellbeing (Conacher 2009). Land degradation can take many forms, including land clearing, aridity, biological invasions, coastal erosion, land erosion, land pollution, land subsidence, landslides, permafrost thawing, soil acidification, soil biodiversity loss, soil compaction, soil sealing and waterlogging (Bateman and Muñoz-Rojas 2019; Prăvălie 2021). A common form of land degradation in Australia is the clearance of forests for agricultural development, resulting from unsustainable farming practices (Bradshaw 2012; Evans 2016; Rashti et al. 2024). Historical data indicates that approximately 30% of Australia’s land surface was once covered by forests, whereas current forests only cover 19% of Australia’s land surface, representing a 38% loss of old-growth forests since the 1800s (Barson et al. 2000; Bradshaw 2012). Most of Australia’s remaining native vegetation has been extensively modified or fragmented. Considering the cumulative impact of land clearing and anthropogenic influences on forests, around 50% of Australia’s forests have either been completely cleared or partially degraded (Bradshaw 2012).
Ecological restoration efforts in Australia have shown some success, particularly in the central north region where vegetation has become greener. However, overall land degradation remains an ongoing issue (Bradshaw 2012; Liu et al. 2015). To enhance the effectiveness of reforestation projects, soil amendments such as chemical and organic fertilisers have been widely utilised. These amendments have been shown to increase soil nutrient concentrations (e.g. N, P, C, K, Mg) and improve soil properties (e.g. pH, cation exchange capacity, water retention) associated with plant growth (Bellino et al. 2015; Cesarano et al. 2017; Liu et al. 2017). However, the conventional application of chemical fertilisers has proven to be unsustainable, contributing to approximately 2.1% of global greenhouse gas (GHG) emissions (Menegat et al. 2022). Therefore, the use of organic amendments such as compost and biochar is considered a more sustainable approach since they are derived from recycled organic materials. Compost, for instance, is produced by purposefully decomposing organic waste for use as a soil amendment (Alvarenga et al. 2017). In contrast, biochar is the char derived from heating of various organic feedstocks in an anaerobic environment through pyrolysis process, and is considered a relatively new field of research for producing novel soil amendments (Sohi et al. 2009; Rezaei Rashti et al. 2019).
The majority of research on organic amendment application has focused on its use in agriculture, as it is the most common application for such amendments (Rezaei Rashti et al. 2016; Liu et al. 2022). Studies conducted within agricultural contexts have consistently identified the agricultural benefits of utilising organic amendments. For instance, the use of compost in agricultural lands has been shown to enhance overall soil quality and promote healthier plant growth (Bellino et al. 2015). Similarly, the application of biochar in agricultural systems has demonstrated comparable (though less consistent) benefits to compost, while also offering the added advantage of enhanced carbon sequestration potential (Schmidt et al. 2021). In some instances, the utilisation of compost and biochar as a combined amendment for agricultural lands has shown significant benefits, such as increased microbial activities, reduced emissions of greenhouse gases and ammonia (NH3), and the immobilisation of heavy metals and organic pollutants (Guo et al. 2020).
The beneficial effects of compost and biochar application in improving soil quality and promoting plant growth have been extensively studied in agricultural systems. However, it is reasonable to consider that some of these benefits may also extend to non-agricultural systems, such as degraded land remediation. Nonetheless, it is important to acknowledge that these benefits may vary in non-agricultural systems due to the significant differences in environmental conditions and desired outcomes (Thomas and Gale 2015). Therefore, a significant research gap exists regarding the impact of compost and, specifically, biochar on degraded soils and native woody plants. Considering the benefits of applying recycled organic amendments to enhance soil and plant health, and the ongoing global issue of land degradation, finding novel ways to rehabilitate degraded lands using local and recycled organic amendments could be a crucial strategy for promoting sustainable practices. Therefore, this study aimed to explore the effect of recycled organic amendments, in the form of compost and biochar, on improvement of the physicochemical properties of a degraded soil and performance of three native Australian plant species. We hypothesised that: (1) application of either biochar or compost as single amendments would improve plant performance compared to an unamended soil; and (2) the combination of compost and biochar would result in greater plant performance when compared to the use of either amendment alone. Additionally, we explored the differences in soil microbial activities in response to the amendments and discussed the feasibility of employing a compost-biochar amendment for degraded lands.
Materials and methods
Preparation of soil, organic amendments, and plant seeds
Soil used in the experiment was collected from the top 10 cm of an open cut coal mine (subsoil section of soil profile remained at the end of mining operation, and before rehabilitation of the site) located near Brisbane, Australia (27°40′9″S, 152°49′9″E). The soil was randomly collected from five points (~10 m from each other) within the sampling site and bulked together to make a composite sample. Soil preparation consisted of removing plant roots, rocks, and other foreign objects, after passing the soil from a 2-mm sieve. Any visible plant tissues were then removed manually. The soil was classified as Vertisol (FAO soil classification) with a clay texture (15% sand, 35% silt, and 50% clay), pH of 8.6 (1:5 water), EC of 152 μS cm−1, mineral N of 11.1 mg kg−1, total organic carbon of 3.2 g kg−1, and water holding capacity of 27%. Measuring the moisture content of soil consisted of oven drying in 105°C for 48 h and determining the percentage of soil water content. The soil was considered restrictive towards ill-adapted plants due to its low organic matter and nutrient contents, poor structure, susceptibility to waterlogging, and shrinking-swelling dynamics which may result in root tearing.
Recycled organic amendments used in this experiment were selected based on their local availability. The applied green waste compost was produced by locally sourced biomass from residential properties around Brisbane, Australia. The process of composting involved mixing the proportion of ‘green’ material (material containing high levels of moisture and considered fresh), and ‘dry’ material (material with low moisture levels) to promote decomposition. Compost piles were placed in head rows and were regularly mixed and watered. The applied biochar derived from locally sourced peanut shell biomass in a slow pyrolysis kiln reactor under the maximum temperature of 500°C with residence time of 60 min and heating rate of 25°C min−1. The properties of applied green waste compost and peanut shell biochar are in Table 1. The amendments along with the soil were mixed in plastic bags until homogeneous prior to placement in dedicated pots. Three native Australian plant species (Eucalyptus tereticornis, Acacia leiocalyx, and Banksia integrifolia) were used in this study. The seeds of A. leiocalyx required heat treatment prior to sowing. This process consisted of soaking seeds (which were wrapped in a cloth rag) in boiling water for 1 min to simulate the high temperature of a bushfire for which the species are adapted to and require for successful germination. The properly treated seeds were then soaked in cold water overnight before sowing.
Green waste compost | Peanut shell biochar | ||
---|---|---|---|
pH () | 7.8 | 7.5 | |
EC (μS cm−1) | 3.44 | 1.32 | |
TC (%) | 24.1 | 60.2 | |
TN (%) | 3.4 | 2.2 | |
C:N ratio | 7.1 | 27.4 | |
NH4+-N (mg kg−1) | 330 | 88 | |
NH3−-N (mg kg−1) | 50 | 8 | |
DOC (g kg−1) | 1.22 | 3.24 | |
DON (g kg−1) | 0.35 | 0.26 | |
WHC (%) | 21 | 127 | |
BD (g cm−3) | 0.37 | 0.31 |
The reported data are means of three replicates.
EC, electrical conductivity; TC, total carbon content; TN, total nitrogen content; DOC, dissolved organic carbon; DON, dissolved organic nitrogen; WHC, water holding capacity; BD, bulk density.
Experimental design
The pot experiment was conducted in a glasshouse for 280 days using a randomised block design, which contained three different species of native Australian plants and two soil amendments (green waste compost and peanut shell biochar). The four amendment variables (10 ton ha−1, which is equal to 4.2 g dry mass per pot) were: (1) compost (CO); (2) biochar (BC); (3) compost-biochar (COBC; 2.1 g dry mass each); and (4) control (CK; no amendment). The four plant variables were: (1) E. tereticornis (EU); (2) A. leiocalyx (AC); (3) B. integrifolia (BA); and (4) control, (CK, no plants). The pot-trial layout was positioned into four blocks (groups) based on the four plant variables, and within each block a column was created based on the four treatments given. The pots position was randomly changed every 30 days during the experiment. In total, 16 treatments were created by combing each amendment type with each plant species using 500 mL pots with 500 g soil (dry weight equivalent), in five replicates. The applied organic amendments were mixed with soil on an end-over-end shaker for 24 h before the start of experiment to achieve a homogeneous mixture. A total of 80 pots were used for the experiment, with each pot being fitted with a plastic mesh filter (0.70 mm) at its inner base to prevent the loss of fine soil particles from seeping through the drainage holes. Seeds of all species (five seeds per pot) were uniformly planted 2 cm below the soil surface in each pot excluding the control treatment (no plants). Following germination, three plants were left in each pot and plants were harvested after 280 days. The average temperature of glasshouse was 34°C during the experiment, with a maximum of 38°C and a minimum of 31°C. The pots were irrigated three times per week with 200 mL water (total of 600 mL per week) to avoid drought stress during the experiment. Drainage of experimental pots were poor which created waterlogged condition immediately after each irrigation for approximately 30 min.
Soil and plant sampling and analysis
All five replicates of each treatment were destructively sampled at the end of experiment (Day 280). Separation of plant material using sieves and tweezers was undertaken to weigh and analyse the plant biomass and soil properties appropriately. After properly homogenising the soil in each pot, 100 g subsample soil was collected for physicochemical analysis. Moisture content of each soil sample was determined by drying the soil samples at 105°C for 48 h. Soil pH and electrical conductivity (EC) were measured in a 1:5 (w/v) extract ratio of soil to distilled water using a glass electrode. Hydrometer method was used to determine soil texture (Rayment and Lyons 2011). Total dissolved organic carbon (DOC) and organic nitrogen (DON) contents of soil were determined using a Shimadzu TOC-V/NCSH/CSNTOC/N analyser (Rayment and Lyons 2011). Total carbon and nitrogen contents of soil and plant samples were determined by combustion method using a LECO Trumac C/N analyser (TruMac NO.830-300-400. USA). Water holding capacity (WHC) was determined by placing soil samples into a cylindrical plastic tube (5 cm in diameter and 7 cm high) that had a fine mesh fitted at its base. The soil samples were then left to soak in sitting water for roughly 2 h. The saturated samples were then removed from the water and left to drip for 24 h. The dripped samples were then weighed and placed into the oven for 48 h at a temperature of 105°C. Weighing of the oven dried samples were undertaken to calculate the differences in each samples water content. To determine dry weight of root and shoot biomass, plant samples were oven dried for 48 h at 65°C.
Soil microbial biomass carbon (MBC) and nitrogen (MBN) contents were determined by fumigation-extraction method using an Ec conversion factor of 2.64 (Vance et al. 1987) and an En conversion factor of 2.22 (Jenkinson 1988), as described by (Liu et al. 2018a). CO2 emissions from each treatment was measured from the gas sampling chambers’ headspace (cylindrical polyethylene chambers with 23.5 cm diameter and 12 cm high). At each gas sampling dates, two pots per treatment were randomly selected and placed into airtight gas chambers sealed with rubber strip and high vacuum silicon grease. The gas samples collected approximately 1 h after chamber closure using a 25-mL gastight syringe and immediately transferred to pre-evacuated 12-mL glass vials (Exetainer; Labco, High Wycombe, UK). The extracted gas samples were analysed for CO2 content using a Gas Chromatograph (Shimadzu GC-2010 Plus), as described by (Rashti et al. 2015). The microbial metabolic quotient (qCO2), defined as the carbon respired per unit of MBC per day, was calculated from the ratio of CO2-C emission rate (mg CO2-C kg−1 soil day−1) to MBC (mg C kg−1 soil) content of each treatment (Liu et al. 2018b).
Statistical analysis
Statistix (ver. 9.0) and R-studio (ver. 1.1.442) was used to run one-way ANOVA, Two-way ANOVA and Pairwise LSD tests. Two-way ANOVA was used for measuring the differences in plant parameters [organic amendment (4) × plant species (3 + 1 CK)], while One-way ANOVA was used to calculate differences in amendments biochemical properties. Pairwise LSD tests was used to measure differences between the means for each treatment. R-studio (ver. 1.1.442) was used to create graphs.
Results
Soil chemical and biological properties
Unamended soil samples (CK treatment) were slightly alkaline with an average pH of 8.5 (Table 2). This value was marginally reduced to 8.3 and 8.4 with the introduction of compost in CO and COBC treatments, respectively, regardless of introduced plant species. Significant differences (P < 0.05) were observed in pH values between CO and BC treatments, while no significant differences were observed among the other treatments. EC was significantly (P < 0.05) lower in CK than other treatments. DOC content significantly (P < 0.05) increased in all amended treatments compared to the control (Fig. 1). In addition, no significant differences were observed in WHC among treatments, but slight reductions were observed after amendment of compost and biochar, where WHC reduced from 26.7% to ~23.0%.
Treatments | pH | EC (μS cm−1) | MBN (mg kg−1) | MBC (mg kg−1) | qCO2 (mg kg−1) | WHC (%) | |
---|---|---|---|---|---|---|---|
CK | 8.5ab | 130a | 87.3a | 105.1a | 4.8a | 26.7a | |
CO | 8.3b | 201b | 59.9b | 129.7a | 8.0c | 23.0a | |
BC | 8.6a | 200b | 102.7c | 135.8a | 4.0a | 23.6a | |
COBC | 8.4b | 238b | 61.0bc | 134.3a | 5.8b | 23.0a |
Means followed by different letters within a column indicate significant differences between the treatments at P < 0.05. The reported data are means of 20 replicates (five replicates for each plant variable).
EC, electrical conductivity; MBN, microbial biomass nitrogen; MBC, microbial biomass carbon; qCO2, microbial metabolic quotient; WHC, water holding capacity; CK, no amendment; CO, compost amendment; BC, biochar amendment; COBC, compost-biochar amendment.
Total dissolved organic carbon contents of treatments at the end of experiment. CK, no amendment; CO, compost amendment; BC, biochar amendment; COBC, compost-biochar amendment. Significant differences were observed between CK and CO, CK and BC, CK and COBC treatments at P < 0.05. The reported data are means of 20 replicates (five replicates for each plant variable).
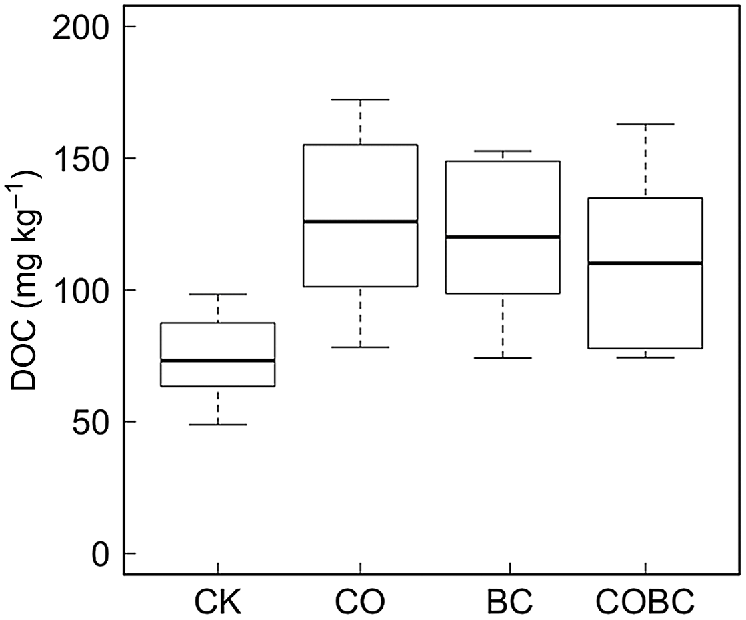
Soil MBC contents generally improved following application of amendments (CO, BC and COBC), while the observed differences between treatments were not statistically significant (Table 2). Soil MBC in CK treatment was lowest amongst the treatments. Soil MBN contents showed a significant difference between BC and CO treatments, and between COBC and BC treatments, with MBN content was lowest in the CO treatment (59.9 mg kg−1), and highest in the BC treatment (102.7 mg kg−1). The cumulative soil respiration in CK and BC treatments did not differ significantly as displayed in Fig. 2. Additionally, soil respiration in the CK and BC treatments were significantly (P < 0.05) lower than that of CO and COBC treatments. Microbial metabolic quotient (qCO2) was highest in the CO and COBC amended treatments, with CO being significantly (P < 0.05) higher than COBC. The qCO2 in COBC was also significantly (P < 0.05) higher than CK and BC treatments, with CK and BC treatments showed no significant differences.
Cumulative soil respiration of treatments at the end of experiment. CK, no amendment; CO, compost amendment; BC, biochar amendment; COBC, compost-biochar amendment. Significant differences were observed between CK and CO treatments at P < 0.05. The reported data are means of 20 replicates (five replicates for each plant variable).
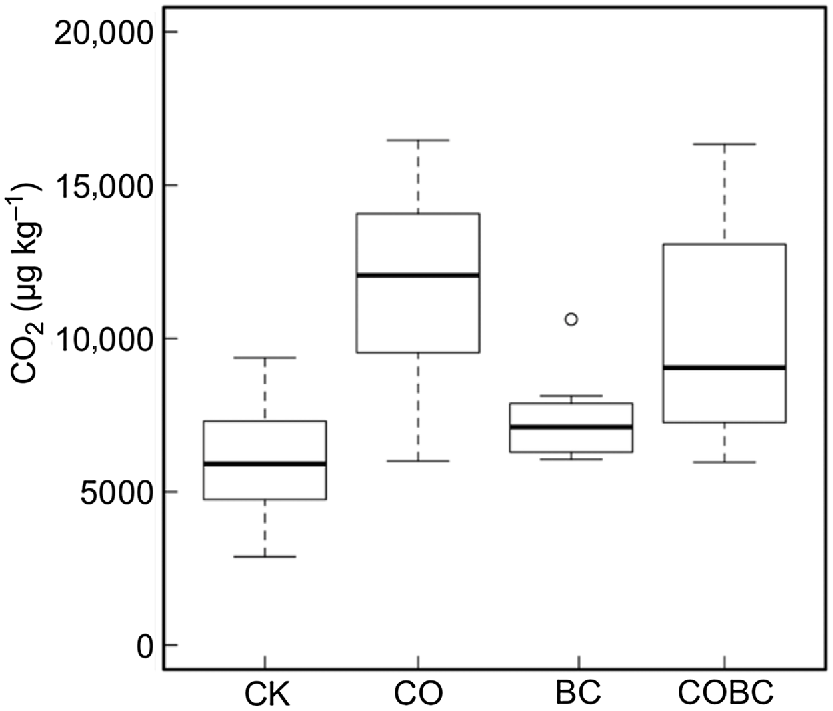
Plant properties
Plant carbon content in the CK:EU treatment was shown to be lowest compared to amended treatments (CO:EU, BC:EU, and COBC:EU), where significant (P < 0.05) differences observed between CK:EU (0.36 g pot−1), CO:EU (0.41 g pot−1), and BC:EU (0.40 g pot−1) treatments (Table 3). The carbon content in AC plans was relatively similar across all treatments irrespective of amendment application and was generally higher than that of the other plant species. Overall, plant carbon contents were higher in species amended with CO and BC. Total carbon sequestration in the CK:EU treatment was significantly (P < 0.05) lower than that of the other EU treatments. Relatively similar rates of carbon sequestration, irrespective of amendments, were observed among the AC treatments. Additionally, the AC treatments showed higher carbon sequestration than the EU and BA treatments. Only BA amended with CO (CO:BA) showed a notable carbon sequestration, while the remaining BA treatments performed relatively poorly.
Treatments | Species | Plant C content (g pot−1) A | Total sequestered C (g pot−1) | Plant N content (mg g−1) | Plant N uptake (mg pot−1) | Shoot/root biomass ratio | Cost (AUD per tonne) B | |
---|---|---|---|---|---|---|---|---|
CK | EU | 0.36c | 0.01f | 28.4b | 1.1d | 1.0e | $0 | |
AC | 0.37bc | 0.20ab | 20.4de | 10.9ab | 2.1de | |||
BA | 0.38abc | 0.09de | 16.5ef | 4.1cd | 5.4ab | |||
CO | EU | 0.41a | 0.13cde | 33.6a | 10.5ab | 2.6cde | $220 | |
AC | 0.39abc | 0.18abc | 22.1d | 10.3ab | 1.1e | |||
BA | 0.42a | 0.24a | 18.9def | 10.9ab | 4.7abc | |||
BC | EU | 0.40ab | 0.09de | 20.9de | 5.1cd | 2.1de | $1800 | |
AC | 0.39abc | 0.19abc | 27.1bc | 12.9a | 1.4e | |||
BA | 0.41a | 0.07ef | 14.7f | 2.3d | 6.1a | |||
COBC | EU | 0.38bc | 0.14bcd | 20.4de | 7.3bc | 4.2abcd | $1100 | |
AC | 0.41a | 0.23a | 23.5bcd | 13.6a | 3.3bcde | |||
BA | 0.02d | 0.01f | 23.4cd | 5.4cd | 2.7cde |
Means followed by different letters within a column indicate significant differences between the treatments at P < 0.05. The reported data are means of five replicates.
CK, no amendment; CO, compost amendment; BC, biochar amendment; COBC, compost-biochar amendment; EU, Eucalyptus tereticornis; AC, Acacia leiocalyx; BA, Banksia integrifolia.
Plant nitrogen concentration in the CK:EU treatment (28.4 mg g−1) was significantly (P < 0.05) lower than that of the CO:EU treatment (33.6 mg g−1). However, the lowest recorded nitrogen concentrations in plant samples were related to the BC:EU (20.9 mg g−1) and COBC:EU (20.4 mg g−1) treatments, which were significantly (P < 0.05) lower than CK:EU (28.4 mg g−1) and CO:EU (33.6 mg g−1) treatments. Relatively similar rates of nitrogen concentration were observed across the AC treatments ranging from 20.4 mg g−1 (CK:AC) to 27.1 mg g−1 (BC:AC). Nitrogen uptake in CK:EU (1.1 mg pot−1) and BC:EU (5.1 mg pot−1) were significantly (P < 0.05) lower than that of CO:EU (10.5 mg pot−1). However, nitrogen uptake across AC treatments were highest among all plant species, while there were no significant differences in nitrogen concentration among treatments with different amendments.
On average, plant biomass was highest within the AC treatments (Fig. 3). Additionally, AC treatments did not significantly differ from each other regardless of applied amendments. Plant biomass was significantly (P < 0.05) lower in the CK:EU compared to CO:EU, BC:EU and COBC:EU treatments. Eucalyptus amended with compost and biochar mixture (COBC:EU) showed the highest rate of plant biomass compared to other amended EU treatments. Banksia amended with compost (CO:BA) significantly (P < 0.05) outperformed CK:BA, BC:BA, and COBC:BA treatments in biomass production.
Plant biomass of treatments at the end of experiment. CK, no amendment; CO, compost amendment; BC, biochar amendment; COBC, compost-biochar amendment; EU, Eucalyptus tereticornis; AC, Acacia leiocalyx; BA, Banksia integrifolia. The reported data are means of five replicates. Means of the treatments by the same letter are not different at the 5% level of significance.
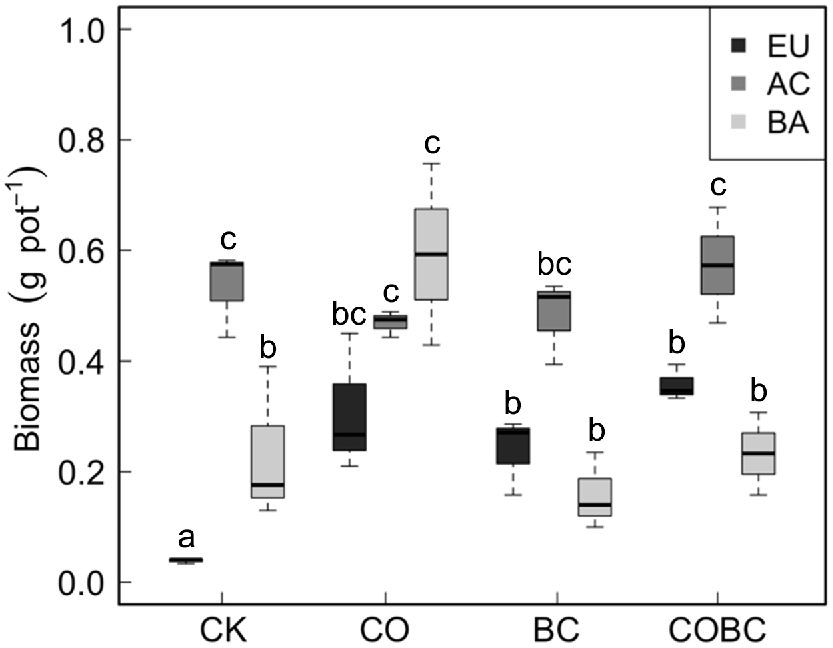
Discussion
Effects of applied organic amendments on soil properties
Since the experiment was conducted using a degraded clay-dominant soil, the physical and chemical stresses resulting from soil limitations may have significantly influenced its biological properties (Roy 2012). Throughout the experiment, it was observed that the soil was susceptible to shrinking, swelling, and waterlogging, likely due to the high percentage of 2:1 expanding clay particles. With the addition of CO and COBC as amendments the pH of treatments were decreased with a significant difference found between CK and CO (Table 2). The balancing of pH to more neutral levels after the addition of organic matter (i.e. compost) has been reported in several studies, which may explain the change in pH of compost containing treatments in this experiment. However, the BC amendment showed a slight increase in pH. This suggests that the pH buffering capacity of the applied biochar may not be equivalent to that of compost.
The degraded soil used in this experiment was nutrient-poor, alkaline, and dominated by clay particles, making it prone to waterlogging (Table 2). Previous studies by Agren et al. (2012) and Camenzind et al. (2018) have shown that nutrient limitations can negatively impact plant and soil biological activities. Additionally, Walter (2018) has described the effects of waterlogging on soil. These soil stress factors likely influenced soil microbial activity and plant growth in this experiment. To assess signs of soil stress, measurements of MBC, CO2 emissions, and microbial metabolic quotient (qCO2) were performed (Table 2, Fig. 2). qCO2 is considered a reliable bioindicator of a soil’s microbial population response to environmental stresses (Zhou and Ding 2007). Table 2 showed a significant increase in qCO2 following the addition of CO and COBC, compared to the CK and BC treatments, while no significant differences observed between CK and BC treatments. The higher rates of qCO2 in the CO and COBC treatments demonstrates that organic carbon was more readily utilised for microbial respiration rather than being converted into microbial biomass. The stimulation of microbial activity in both CO and COBC treatments was likely due to the high level of labile carbon in applied compost. The lack of significant difference in qCO2 between the CK and BC treatments may be attributed to biochar’s resistance to decomposition and limited labile carbon content (Thies and Rillig 2012). The low levels of MBC and MBN observed in our treatments, along with increased CO2 emissions in the CO and COBC treatments, align with the qCO2 results. These findings suggest that soil microbial populations experienced considerable stress despite the application of amendments, potentially impeding plant performance. The alkalinity of the soil and the occurrence of waterlogging could have further contributed to the hindered plant growth.
The application of organic amendments has been extensively shown to improve soil functionality and structure in degraded lands (Tejada et al. 2009; Diacono and Montemurro 2011; Carlson et al. 2015). The type of organic amendments can also significantly influence soil properties (Tejada and Gonzalez 2007; Masunga et al. 2016; Yazdanpanah et al. 2016). In our experiment, a significant increase in EC and DOC values observed in all amended treatments (CO, BC, COBC) compared to the control (CK). This aligns with the findings of Robin et al. (2018), who demonstrated the ability of organic amendments to increase soil carbon levels. Additionally, Carmo et al. (2016) showed that EC can be improved through the application of organic amendments. A significant increase in CO2 emissions from CO treatment was observed in Fig. 2. It further indicated that the BC and COBC treatments had no significant difference in CO2 emissions compared to the control (CK), despite the COBC treatment containing a labile carbon source from compost amendment. The COBC treatment also had the highest EC value in the experiment (Table 2). The COBC showed no increase in CO2 emissions compared to the control (CK) and had the highest EC in the experiment which can be attributed to the combined effects of compost and biochar in this treatment. Biochar is highly resistant to microbial decomposition and is known to be a greenhouse gas inhibitor (Agyarko-Mintah et al. 2017; Li et al. 2018a). Previous studies by Kammann et al. (2015) and Prost et al. (2013) have reported increased nutrient loading in soils amended with a combination of compost and biochar compared to compost-only or biochar-only treatments. This suggests that the interaction of compost and biochar in the COBC treatment may have led to improved nutrient loading and reduced soil CO2 emissions. Given that the EC values in the CO, BC, and COBC treatments were similarly enhanced, the reduction in CO2 emissions resulting from applied biochar makes the COBC and BC treatments more desirable for minimising soil CO2 emissions.
Effects of applied organic amendments on plant performance
Increasing soil organic carbon (SOC) pools in degraded lands, through the application of organic amendments or the bioaccumulation of carbon by plants, can contribute to the success of land rehabilitation efforts (Lal 2015). Plants play a crucial role in improving SOC stocks by bioaccumulation and decomposition of organic matter (Novara et al. 2015). Therefore, it is desirable to select plant species that excel at carbon storage for revegetation projects, as they can enhance the rate of carbon sequestration. This experiment highlights an effective carbon sequestration by AC plants. Fig. 3 showcases how AC species exhibited the highest biomass across all treatment groups. Balieiro et al. (2017) also reported high rates of carbon sequestration in leguminous species. The high rate of carbon sequestration and biomass accumulation in AC treatments (particularly in CK:AC) likely related to the leguminous nature of the Acacia species, removing any impediments toward nitrogen acquisition (Reverchon et al. 2015). The AC treatment group did not show any signs of nitrogen deficiencies (Table 3) and exhibited relatively high plant nitrogen concentrations compared to other species. Additionally, there were no significant differences in plant biomass between the AC treatments (Fig. 3), suggesting that organic amendments had limited impact on AC performance. Although the COBC:AC treatment recorded the highest biomass within the AC treatments, this difference was not statistically significant and could be attributed to improved nutrient loading in this specific treatment.
The application of amendments had a notable impact on the biomass content within the EU treatment group. In particular, significant differences were observed between the control group (CK:EU) and the amended EU treatments, with CK:EU exhibiting the lowest biomass content (Fig. 3). This suggests that the application of amendments, which resulted in increased nutrient availability and improved soil structure, played a crucial role in enhancing the performance of EU plants. The combination of compost and biochar in the COBC:EU treatment resulted in the highest biomass (statistically significant) and carbon sequestration (not statistically significant) within the EU treatment group (Fig. 3, Table 3). The higher rates of biomass and carbon sequestration in COBC:EU compared to the other EU treatments could be attributed to the enhanced nutrient availability in COBC amended soil (Table 2), and improved nutrient loading occurred in compost-biochar applied soil as described by Kammann et al. (2015) and Prost et al. (2013). Improved soil structure following the application of organic amendments, which reduced waterlogging conditions, likely contributed to the observed outcomes (Table 2). Breton et al. (2016) has demonstrated that plants produce higher rates of survival after organic amendment application in a revegetation setting. While studies by Donn et al. (2014), Jain et al. (2014) and Li et al. (2018b) have shown that plant performance can be improved after the application of organic amendments. The compost only amendment (CO:EU) performed relatively well within the EU treatment group, generally producing comparable but slightly lower results to COBC:EU (Fig. 3, Table 3). Therefore, to maximise carbon sequestration when growing eucalyptus species in this soil type, it is recommended to use amendments comprising compost or a mixture of compost and biochar, as they are likely to yield the most beneficial results.
The Banksia treatment group exhibited poor performance throughout the experiment, showing low biomass accumulation compared to the EU and AC treatment groups. This underperformance could be attributed to the fact that B. integrifolia are naturally adapted to thrive in sandy soils (Wilson 1999), rather than the clay soil utilised in this study. Sandy soils can stimulate seedling growth of native plants due to their better wetting properties, higher water infiltration rates, and proper physical characteristics for optimum development of plants’ tap root (Maestre and Cortina 2002; Hallett et al. 2014). However, the CO:BA treatment yielded high biomass rates, similar to the AC treatments. This suggests that if this particular Banksia species were to be planted with this soil type, higher rates of compost amendment might be necessary for successful establishment in a revegetation setting. It has been reported that compost application to degraded lands would not only improve soil physical conditions but also increase soil microbial activity and its macro- (e.g. N, P, K, Ca) and micro- (e.g. Zn, Fe, Mn) nutrient status, required for optimum performance of native plant species (Jones et al. 2012). It is important to note that the biomass obtained from the CO:BA treatment may not be reliable enough for accurate extrapolation in predicting the behaviour of this species during revegetation of a similar soil type to what was used in this study. Additional studies with different organic amendments and application rates required to confirm the findings of this experiment.
Recommendations for future application of compost-biochar amendments in degraded soils
Compost and biochar exhibit different behaviours in their resistance to decomposition. The labile and recalcitrant nature of organic carbon in these amendments should be taken into account when considering their future application in controlled or field environments. Depending on the application context, short-term strategies for soil amendment may benefit from utilising compost due to its labile carbon nature, which provides nutrients relatively quickly. However, long-term strategies may wish to utilise the properties of biochar to improve soil chemistry and structure over an extended period. When utilising short-term strategies (i.e. compost amendments), reapplication may be necessary to sustain the improvements observed from the initial application. As an example of long-term application strategies, the application of CO in this experiment had a similar effect on soil EC when compared to BC, with both equalling ~200 μS cm−1 (Table 2). Given biochar’s high recalcitrant carbon rating compared to compost, incorporating biochar can be an effective long-term approach to enhancing nutrient bioavailability within the soil profile. To obtain short- and long-term improvements in amended soil properties, the results of this experiment indicate that combining compost and biochar in an amendment, as demonstrated by the COBC treatment in this study, may provide more significant benefits compared to using compost or biochar alone. Such a combined approach could potentially lead to reduced soil respiration, improved soil structure, and enhanced nutrient loading, thus offering substantial improvements in amended soil properties in both the short- and long-term.
Consideration of soil microbial activity and performance is essential when applying organic amendments. While organic amendments can initially improve soil conditions, particularly those with high labile organic carbon, the effects can diminish over time due to soil microbial respiration and decomposition processes if no additional amendments are applied. To address this issue, including durable plants that generate high rates of organic matter can provide a continuous input of soil organic carbon. This could improve and maintain soil physicochemical properties without the need for additional amendment applications. In our experiment, Acacia demonstrated the highest biomass and carbon sequestration potential among the plant species, although the difference between amendments was not significant. Consequently, Acacia species would be a suitable option for maintaining and increasing organic matter after amendment application in similar soil conditions. Additionally, the COBC:AC treatment showed the highest rate of carbon sequestration (not statistically significant), suggesting that a combination of AC and COBC amendment could be a potentially effective strategy for maintaining soil organic carbon pools in future applications under similar soil types. However, further research is necessary to validate this approach in larger-scale studies beyond the scope of this experiment.
In order to assess the practicability of future applications and research involving compost-biochar mixtures, it is crucial to consider the financial constraints associated with each component. Table 3 presents the current market price differences between compost and biochar in Australia, revealing a significant price discrepancy per ton. For larger scale applications and research involving compost-biochar amendments, the effects of such a price discrepancy will be more pronounced. Therefore, cost-effectiveness and practicality should guide future research endeavours, particularly when the findings are intended to be implemented in industry settings. The results of this experiment indicate that the inclusion of biochar in combination with compost did provide some benefits. However, these benefits may not be significant enough to justify the inclusion of biochar in larger scale applications considering its cost. It is important to note that the duration of this experiment was relatively short and did not allow for the investigation of long-term benefits associated with the recalcitrant nature of biochar. Extending the duration of this experiment would likely reveal more apparent long-term effects of biochar within a compost-biochar amendment. Furthermore, it is important to acknowledge that the conclusions and recommendations drawn from this experiment are based on a controlled glasshouse experiment, which may not directly translate to larger scale scenarios in the future. Therefore, additional research should be approached with caution and conducted to further investigate the application of the methods and results discussed in this paper before considering broadscale implementation.
Conclusion
This study investigated the effects of organic amendments (CO, BC, and COBC) on improvement of soil properties and native plants performance in a degraded soil. Results showed a general increase in soil dissolved organic carbon content, microbial biomass content and microbial respiration in amended treatments compared to the control. The Acacia treatment group showed the highest biomass production and carbon sequestration potential among the plant species, making it a promising candidate for enhancing soil organic matter in degraded lands with similar soil conditions. While the application of compost and biochar demonstrated substantial improvements in soil properties and plant performance, it is essential to consider the financial aspects associated with these amendments, especially for large-scale remediation projects. Further research is warranted to validate the findings of this experiment in long-term field applications.
Conflicts of interest
No financial or non-financial (political, personal, professional) interests/relationships have influenced the manuscript and the research it contains. Author Chengrong Chen is an Associate Editor of Soil Research but played no role in the editorial handling or reviewing of this manuscript.
Acknowledgements
We acknowledge the contributions of Dennis Baker who assisted in organising soil samples, and in part for analysis undertaken during the experiment. We also acknowledge the contributions from members of the Soil Biogeochemistry Research Laboratory at Griffith University during the length of this experiment.
References
Agren GI, Wetterstedt JÅM, Billberger MFK (2012) Nutrient limitation on terrestrial plant growth–modeling the interaction between nitrogen and phosphorus. New Phytologist 194(4), 953-960.
| Crossref | Google Scholar | PubMed |
Agyarko-Mintah E, Cowie A, Singh BP, Joseph S, Van Zwieten L, Cowie A, Harden S, Smillie R (2017) Biochar increases nitrogen retention and lowers greenhouse gas emissions when added to composting poultry litter. Waste Management 61, 138-149.
| Crossref | Google Scholar | PubMed |
Alvarenga P, Palma P, Mourinha C, Farto M, Dores J, Patanita M, Cunha-Queda C, Natal-da-Luz T, Renaud M, Sousa JP (2017) Recycling organic wastes to agricultural land as a way to improve its quality: a field study to evaluate benefits and risks. Waste Management 61, 582-592.
| Crossref | Google Scholar | PubMed |
Balieiro FdC, Costa CA, Oliveira RBd, Oliveira Rd, Donagemma GK, Andrade AGd, Capeche CL (2017) Carbon stocks in mined area reclaimed by leguminous trees and sludge. Revista Árvore 41(6), e410610.
| Crossref | Google Scholar |
Bateman AM, Muñoz-Rojas M (2019) To whom the burden of soil degradation and management concerns. Advances in Chemical Pollution, Environmental Management and Protection 4, 1-22.
| Crossref | Google Scholar |
Bellino A, Baldantoni D, De Nicola F, Iovieno P, Zaccardelli M, Alfani A (2015) Compost amendments in agricultural ecosystems: confirmatory path analysis to clarify the effects on soil chemical and biological properties. The Journal of Agricultural Science 153(2), 282-295.
| Crossref | Google Scholar |
Bradshaw CJA (2012) Little left to lose: deforestation and forest degradation in Australia since European colonization. Journal of Plant Ecology 5(1), 109-120.
| Crossref | Google Scholar |
Breton V, Crosaz Y, Rey F (2016) Effects of wood chip amendments on the revegetation performance of plant species on eroded marly terrains in a Mediterranean mountainous climate (Southern Alps, France). Solid Earth 7(2), 599-610.
| Crossref | Google Scholar |
Camenzind T, Hättenschwiler S, Treseder KK, Lehmann A, Rillig MC (2018) Nutrient limitation of soil microbial processes in tropical forests. Ecological Monographs 88(1), 4-21.
| Crossref | Google Scholar |
Carlson J, Saxena J, Basta N, Hundal L, Busalacchi D, Dick RP (2015) Application of organic amendments to restore degraded soil: effects on soil microbial properties. Environmental Monitoring and Assessment 187(3), 109.
| Crossref | Google Scholar | PubMed |
Carmo DLd, Lima LBd, Silva CA (2016) Soil fertility and electrical conductivity affected by organic waste rates and nutrient inputs. Revista Brasileira de Ciência do Solo 40, e0150152.
| Crossref | Google Scholar |
Cesarano G, De Filippis F, La Storia A, Scala F, Bonanomi G (2017) Organic amendment type and application frequency affect crop yields, soil fertility and microbiome composition. Applied Soil Ecology 120, 254-264.
| Crossref | Google Scholar |
Conacher A (2009) Land degradation: a global perspective. New Zealand Geographer 65(2), 91-94.
| Crossref | Google Scholar |
Donn S, Wheatley RE, McKenzie BM, Loades KW, Hallett PD (2014) Improved soil fertility from compost amendment increases root growth and reinforcement of surface soil on slopes. Ecological Engineering 71, 458-465.
| Crossref | Google Scholar |
Evans MC (2016) Deforestation in Australia: drivers, trends and policy responses. Pacific Conservation Biology 22(2), 130-150.
| Crossref | Google Scholar |
Guo X-X, Liu H-T, Zhang J (2020) The role of biochar in organic waste composting and soil improvement: a review. Waste Management 102, 884-899.
| Crossref | Google Scholar | PubMed |
Hallett LM, Standish RJ, Jonson J, Hobbs RJ (2014) Seedling emergence and summer survival after direct seeding for woodland restoration on old fields in south-western Australia. Ecological Management & Restoration 15, 140-146.
| Crossref | Google Scholar |
Jain P, Sharma RC, Bhattacharyya P, Banik P (2014) Effect of new organic supplement (Panchgavya) on seed germination and soil quality. Environmental Monitoring and Assessment 186(4), 1999-2011.
| Crossref | Google Scholar |
Jones BEH, Haynes RJ, Phillips IR (2012) Addition of an organic amendment and/or residue mud to bauxite residue sand in order to improve its properties as a growth medium. Journal of Environmental Management 95, 29-38.
| Crossref | Google Scholar | PubMed |
Kammann CI, Schmidt H-P, Messerschmidt N, Linsel S, Steffens D, Muller C, Koyro H-W, Conte P, Joseph S (2015) Plant growth improvement mediated by nitrate capture in co-composted biochar. Scientific Reports 5, 11080.
| Crossref | Google Scholar | PubMed |
Lal R (2015) Restoring soil quality to mitigate soil degradation. Sustainability 7(5), 5875-5895.
| Crossref | Google Scholar |
Li Y, Hu S, Chen J, Müller K, Li Y, Fu W, Lin Z, Wang H (2018a) Effects of biochar application in forest ecosystems on soil properties and greenhouse gas emissions: a review. Journal of Soils and Sediments 18(2), 546-563.
| Crossref | Google Scholar |
Li Z, Schneider RL, Morreale SJ, Xie Y, Li C, Li J (2018b) Woody organic amendments for retaining soil water, improving soil properties and enhancing plant growth in desertified soils of Ningxia, China. Geoderma 310, 143-152.
| Crossref | Google Scholar |
Liu YY, van Dijk AIJM, De Jeu RAM, Canadell JG, McCabe MF, Evans JP, Wang G (2015) Recent reversal in loss of global terrestrial biomass. Nature Climate Change 5(5), 470-474.
| Crossref | Google Scholar |
Liu Z, Rong Q, Zhou W, Liang G (2017) Effects of inorganic and organic amendment on soil chemical properties, enzyme activities, microbial community and soil quality in yellow clayey soil. PLoS ONE 12(3), e0172767.
| Crossref | Google Scholar | PubMed |
Liu XY, Rashti MR, Esfandbod M, Powell B, Chen CR (2018a) Liming improves soil microbial growth, but trash blanket placement increases labile carbon and nitrogen availability in a sugarcane soil of subtropical Australia. Soil Research 56(3), 235-243.
| Crossref | Google Scholar |
Liu X, Rezaei Rashti M, Dougall A, Esfandbod M, Van Zwieten L, Chen C (2018b) Subsoil application of compost improved sugarcane yield through enhanced supply and cycling of soil labile organic carbon and nitrogen in an acidic soil at tropical Australia. Soil and Tillage Research 180, 73-81.
| Crossref | Google Scholar |
Liu X, Milla R, Granshaw T, Van Zwieten L, Rezaei Rashti M, Esfandbod M, Chen C (2022) Responses of soil nutrients and microbial activity to the mill-mud application in a compaction-affected sugarcane field. Soil Research 60(4), 385-398.
| Crossref | Google Scholar |
Maestre FT, Cortina J (2002) Spatial patterns of surface soil properties and vegetation in a Mediterranean semi-arid steppe. Plant and Soil 241, 279-291.
| Crossref | Google Scholar |
Masunga RH, Uzokwe VN, Mlay PD, Odeh I, Singh A, Buchan D, De Neve S (2016) Nitrogen mineralization dynamics of different valuable organic amendments commonly used in agriculture. Applied Soil Ecology 101, 185-193.
| Crossref | Google Scholar |
Menegat S, Ledo A, Tirado R (2022) Greenhouse gas emissions from global production and use of nitrogen synthetic fertilisers in agriculture. Scientific Reports 12(1), 14490.
| Crossref | Google Scholar |
Novara A, Rühl J, La Mantia T, Gristina L, La Bella S, Tuttolomondo T (2015) Litter contribution to soil organic carbon in the processes of agriculture abandon. Solid Earth 6(2), 425-432.
| Crossref | Google Scholar |
Prăvălie R (2021) Exploring the multiple land degradation pathways across the planet. Earth-Science Reviews 220, 103689.
| Crossref | Google Scholar |
Prost K, Borchard N, Siemens J, Kautz T, Séquaris J-M, Möller A, Amelung W (2013) Biochar affected by composting with farmyard manure. Journal of Environmental Quality 42(1), 164-172.
| Crossref | Google Scholar | PubMed |
Rashti MR, Wang WJ, Harper SM, Moody PW, Chen CR, Ghadiri H, Reeves SH (2015) Strategies to mitigate greenhouse gas emissions in intensively managed vegetable cropping systems in subtropical Australia. Soil Research 53(5), 475-484.
| Crossref | Google Scholar |
Rashti MR, Nelson PN, Lan Z, Su N, Esfandbod M, Liu X, Goloran J, Zhang H, Chen C (2024) Sugarcane cultivation altered soil nitrogen cycling microbial processes and decreased nitrogen bioavailability in tropical Australia. Journal of Soils and Sediments 24, 946-955.
| Crossref | Google Scholar |
Reverchon F, Yang H, Ho TY, Yan G, Wang J, Xu Z, Chen C, Zhang D (2015) A preliminary assessment of the potential of using an acacia – biochar system for spent mine site rehabilitation. Environmental Science and Pollution Research 22(3), 2138-2144.
| Crossref | Google Scholar | PubMed |
Rezaei Rashti M, Wang WJ, Reeves SH, Harper SM, Moody PW, Chen CR (2016) Linking chemical and biochemical composition of plant materials to their effects on N2O emissions from a vegetable soil. Soil Biology and Biochemistry 103, 502-511.
| Crossref | Google Scholar |
Rezaei Rashti M, Esfandbod M, Phillips IR, Chen CR (2019) Aged biochar alters nitrogen pathways in bauxite-processing residue sand: environmental impact and biogeochemical mechanisms. Environmental Pollution 247, 438-446.
| Crossref | Google Scholar |
Robin P, Morel C, Vial F, Landrain B, Toudic A, Li Y, Akkal-Corfini N (2018) Effect of three types of exogenous organic carbon on soil organic matter and physical properties of a sandy technosol. Sustainability 10(4), 1146.
| Crossref | Google Scholar |
Schmidt H-P, Kammann C, Hagemann N, Leifeld J, Bucheli TD, Sanchez Monedero MA, Cayuela ML (2021) Biochar in agriculture – a systematic review of 26 global meta-analyses. GCB Bioenergy 13(11), 1708-1730.
| Crossref | Google Scholar |
Tejada M, Gonzalez JL (2007) Influence of organic amendments on soil structure and soil loss under simulated rain. Soil and Tillage Research 93(1), 197-205.
| Crossref | Google Scholar |
Tejada M, Hernandez M, Garcia C (2009) Soil restoration using composted plant residues: effects on soil properties. Soil and Tillage Research 102(1), 109-117.
| Crossref | Google Scholar |
Thomas SC, Gale N (2015) Biochar and forest restoration: a review and meta-analysis of tree growth responses. New Forests 46(5–6), 931-946.
| Crossref | Google Scholar |
Vance ED, Brookes PC, Jenkinson DS (1987) An extraction method for measuring soil microbial biomass C. Soil Biology and Biochemistry 19(6), 703-707.
| Crossref | Google Scholar |
Walter J (2018) Effects of changes in soil moisture and precipitation patterns on plant-mediated biotic interactions in terrestrial ecosystems. Plant Ecology 219(12), 1449-1462.
| Crossref | Google Scholar |
Yazdanpanah N, Mahmoodabadi M, Cerdà A (2016) The impact of organic amendments on soil hydrology, structure and microbial respiration in semiarid lands. Geoderma 266, 58-65.
| Crossref | Google Scholar |
Zhou L, Ding M (2007) Soil microbial characteristics as bioindicators of soil health. Biodiversity Science 15(2), 162.
| Crossref | Google Scholar |