Modified fungal diversity in dense clay subsoils after deep-banding organic substrate
Joshua J. Vido A , Jian Jin B , Helen L. Hayden C F , Corinne Celestina

A
B
C
D
E
F Present address:
Abstract
Deep-banded nutrient rich amendments can overcome crop productivity constraints of Australian dense clay subsoils. However, knowledge on essential microbial community in field trials is limited.
We examined subsoils that had been deep-ripped 2 years earlier with various types of amendments (organic, a blend of organic and inorganic, and purely inorganic). Subsoil samples (15–25 cm) were collected encompassing the amendment band (0 cm), as well as at increasing distances from it (14 and 28 cm). Bacterial 16S rRNA, fungal ITS amplicon sequencing, and SOM/TOC measurements on amendment band samples were done to assess microbial communities.
While no variations were detected in bacterial communities across treatments, soils enriched with organic substrates diverged significantly in fungal diversity compared to the control, concentrated primarily within the amendment bands. Fungal response to these organic amendments was primarily dominated by an enrichment of filamentous saprotrophic fungi.
Changes in fungal diversity and the enrichment of saprotrophic fungi is primarily attributed to the introduction of organic substrates into the subsoil. However, despite the absence of SOM/TOC differences between treatments, SOM/TOC levels were initially expected to rise in response to organic amendments. Consequently, variations in fungal communities may have initially arisen from heightened SOM/TOC levels but persisted even as these levels returned to baseline, suggesting a lasting legacy effect.
A single application of deep-banded organic amendments was effective in enriching agriculturally significant fungi within dense clay subsoils after 2 years. This can further aid crop productivity by fostering soil structural improvements and optimising nutrient cycling, even after the organic amendments are undetectable.
Keywords: agricultural management, microbial communities, microbial diversity, organic amendments, saprotrophic fungi, soil bioindicators, soil ecology, subsoil amelioration.
Introduction
Soil microbial communities underpin the maintenance and function of terrestrial ecosystems through their relationship with soil fertility and plant productivity. This occurs primarily through ecological functions mediated by bacteria and fungi such as nutrient cycling, soil aggregation, pathogenesis, and rhizosphere interactions (Fierer et al. 2007; Thiele-Bruhn et al. 2012). Consequently, microorganisms contribute critically to the maintenance and productivity of agricultural systems. However, agricultural management practises aimed at enhancing plant growth via soil physicochemical modifications (i.e. tillage, deep-ripping, and the use of manures/fertilisers) strongly impact soil microbiology through direct (response to soil physicochemical change) and indirect (response to plant fitness change) mechanisms (Vido et al. 2024). As a result, these practices can enhance or constrain the proliferation of microorganisms, which conduct metabolic processes closely associated with crop productivity and soil fertility such as nutrient cycling (Kabir 2005; Ding et al. 2017; Hermans et al. 2020; Yarwood 2021) Therefore, examining the soil microbial dynamics is of paramount importance in assessing the benefits and consequences of a new management practice used in an agricultural system.
Deep-banding of nutrient-rich amendments (i.e. fertilisers, manures, plant residues) is an agricultural management practice designed to ameliorate the crop productivity constraints in dispersive clay subsoils found across south-eastern Australia. These subsoils restrict crop production though poor soil structure (i.e. high bulk density, minimal porosity, and aggregation), high sodicity, periodic waterlogging or limited plant-available water (MacEwan et al. 2010; Sale et al. 2021). However, past research has shown that the deep-banding of nutrient-rich organic amendments can effectively increase the productivity of crops growing in soils with dispersive clay subsoils (Sale et al. 2019; Uddin et al. 2020; Armstrong et al. 2021). Increased grain yield responses to deep-banded amendments are hypothesised to result from several factors including increased nutrient availability and soil organic matter (SOM), soil structural improvements and enhanced root proliferation (Gill et al. 2009; Celestina et al. 2018; Uddin et al. 2022). However, the response of bacterial and fungal communities to deep-banded amendments, particularly in field-based studies continues to be unclear.
Deep-banded organic amendments have been shown to impact microbial communities via direct and indirect mechanisms, and introduce macro- and micronutrients, including C and N sources, to the soil. Increased microbial abundance, activity (i.e. CO2 respiration) and community composition changes have been observed in response to deep-banded amendments (Clark et al. 2007; Celestina et al. 2019a, 2019b; Wang et al. 2020). However, as the amendments are banded in the soil and not mixed, a significant distance- dependent effect has been observed, with most of the microbial response within the amendments detritusphere (i.e. 1–2 cm surrounding the amendment) (Nkebiwe et al. 2017; Celestina et al. 2019a; Wang et al. 2020; Vido et al. 2024). This is facilitated by greater nutrient availability and soil physicochemical modifications within the soil immediately surrounding the amendments, which also contributes to localised enhanced root distribution and rhizodeposition (Wang et al. 2020). This leads to a highly localised plant-mediated bacterial (and possibly fungal) response within this expanded rhizosphere (Vido et al. 2024). Furthermore, Wang et al. (2020) determined fungi in conjunction with plant roots contributed to the formation of clay macroaggregates localised primarily within the subsoil 1–2 cm beside the amendment, and also 3–4 cm below the amendment. Whilst these studies demonstrate a significant microbial response to deep-banded amendments, they are primarily short-term (i.e. one crop cycle) controlled soil column experiments.
To date, only a study by Celestina et al. (2019c) has examined the long-term microbial response to a single application of deep-banded amendments in the field. Celestina et al. (2019c) observed a small but significant fungal community change in response to deep-banded amendments 3 years after placement. However, this study did not examine the impact of sampling position in relation to the amendment band (i.e. detritusphere) on the magnitude of microbial community changes, which would be a distance dependent effect. Therefore, it has not been ascertained whether the microbial response is heavily localised as observed in controlled column studies, or whether significant changes are observed over time at increased distances through gradual amendment induced soil modifications and root growth (Gill et al. 2009). Furthermore, this study (Celestina et al. 2019c) and other subsoil amendment microbial studies (Celestina et al. 2019a, 2019b; Wang et al. 2020) have focused mostly on abundance and community level changes. As a result, there is a need to obtain a more comprehensive understanding of microbial responses involving functional and ecologically relevant information in relation to the wider soil environment.
This study examined bacterial and fungal responses to deep-banded amendments 2 years after their placement in an Australian field site. We aimed to: (1) detect whether microbial community variations were associated with inorganic and/or organic amendments 2 years after placement; (2) examine the impact of sampling position, in relation to the amendment band on the microbial community response; and (3) determine the ecology of microbial taxa that were impacted by the amendments and whether they are indicative of extended soil environmental modifications. We hypothesise that microbial community variations detected after an extended period following deep-banded amendments could allude to: (1) sustained soil environment alterations; or (2) legacy effects on soil microbial ecology driven by amendment addition. Furthermore, we predict that agriculturally relevant insights can be uncovered through determining the specific microbial taxa shown to be strongly impacted by the amendments.
Materials and methods
Study site and experimental design
The study site was located within the high rainfall region of south-eastern Victoria, Australia (37°29′48.30″S 142°58′38.56″E) (Armstrong et al. 2021). The annual rainfall was 492 and 547 mm for 2019 and 2020, respectively (Jin et al. 2023). Before addition of amendment in 2018, the subsoil (15–25 cm) had a bulk density of 1.43 g cm−3, pH of 6.3, electrical conductivity of 0.22 dS m−1, cation exchange capacity of 21.9 cmol kg−1, exchangeable sodium percentage of 6.1%, Nephelometric Turbidity (dispersion) of 3200 NTU, and organic C of 0.76% (Jin et al. 2023).
The study was a randomised complete block design consisting of four replicates of 11 experimental treatments with a control treatment, as detailed by Jin et al. (2023). Soil sampling was conducted on both the control group and a subset comprising three specific treatments: (1) wheat straw enhanced with fluid inorganic nutrients (WS + FN); (2) chicken manure pellets (CMP); and (3) inorganic fluid nutrients (FN) (Fig. 1). CMP was added at 20 t ha−1 fresh weight and contained 10% moisture, and 34% C, 3.6% N, 1.48% P, 0.9% S and 2.3% Ca on a dry weight basis. Wheat straw was added at 20 t ha−1 fresh weight and comprised of 43.4% C, 0.6% N, 0.05% P, 0.08% S, 1.25% Ca and 0.63% K. Fluid nutrients were added to the WS + FN treatment and the FN treatment at 46.6 kg P ha−1 and 287.8 kg N ha−1 and comprised of a liquid mixture of urea, ammonium nitrate (Incitec Pivot Easy) and ammonium polyphosphate (Campbells Nitro-P).
Experimental design schematic of the GRDC field trial site located at Tatyoon, Victoria, Australia from an aerial perspective. The experiment was a randomised block design with a total of four replicate blocks each containing one replicate of 12 different treatments. A subset of four treatments from a total of 12 were used in the current study. Buffer zones of 16 m were established between the experimental plots to prevent confounding results. Each experimental treatment plot was 20 m in length and 3.6 m wide containing six riplines (i.e. amendment bands) spaced ∼0.6 m apart. Non-treatment zones separating plots were 2 m in width. Tramlines refer to a pair of parallel wheel tracks or pathways that are created and maintained in a field to provide a designated route for agricultural machinery.
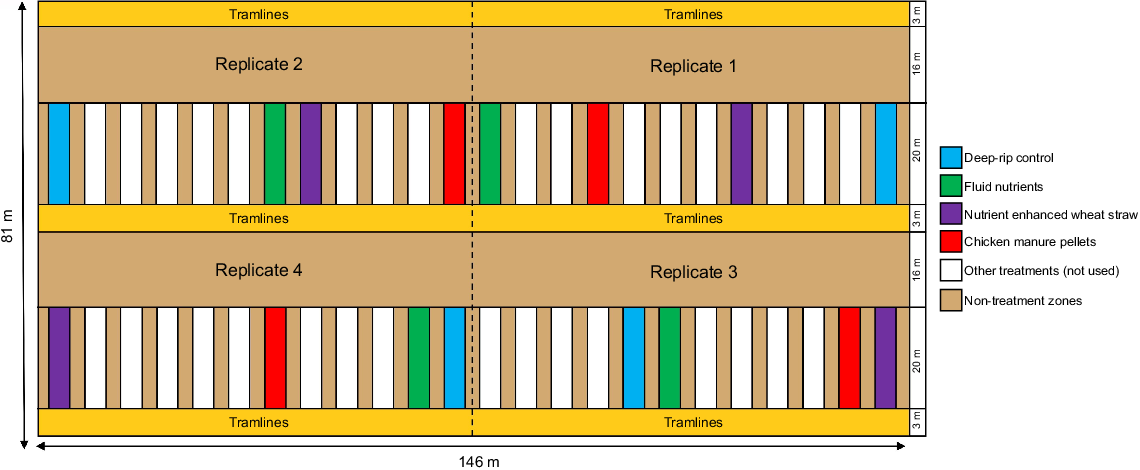
All amendments were deep-placed at the base of riplines within the subsoil at depth of 25 cm depth using a custom-built subsoil machine and hopper (i.e. deep-ripping). The bands of CMP and WS + N extended from the base of the rip-line to approximately 15 cm above the base; hence, amendments were located at 10–25 cm within the soil profile. The control was deep-ripped without any added amendment to examine the impact of deep-ripping alone. The experimental treatments were carried out once in April 2018 in plots that were 72 m2 (20 m long × 3.6 m wide) with 2 m between plots (Fig. 1). Each 3.6-m wide plot contained six amendment bands spaced 56 cm apart. Markers were placed in each plot to indicate the location of the centremost ripline. Wheat (Triticum aestivum) cv. Derrimut was sown in May 2018 and harvested in December 2018 and barley (Hordeum vulgare) cv. Planet was sown in May 2019 and harvested in December 2019.
Subsoil sample collection
Soil sampling was conducted during fallow in March 2020, 3 months following harvest. Prior to soil sampling, the deep-ripping direction and location of the centremost amendment band within each treatment plot were located utilising previously established markers. A hand-held soil auger (5 cm diameter × 10 cm height) was used to collect soil at a depth of 15–25 cm. Samples were collected at three distances relative to the amendment band at: (1) the centre the amendment band (0 cm ± 2.5 cm); 14 cm ± 2.5 cm away from the amendment band; and (3) 28 cm ± 2.5 cm away from the amendment band. Three samples for each distance were taken at 5-m intervals along the centre ripline of each replicate plot, beginning at 5 m and ending at 15 m. Buffer areas 5 m long at the ends of each plot were not sampled in order to avoid disturbed areas. This resulted in three replicate samples at each distance for each treatment plot and a total of 12 soil samples for each sampling distance for each of the four treatments. Each soil sample was thoroughly mixed and sieved to 10 mm to remove large non-soil material.
Bacterial and fungal taxonomic diversity measurements
DNA samples were obtained from the extraction of 0.30 g fresh soil using PowerSoil Pro DNA isolation kit (MoBio, Calsbad, USA) and stored at −20°C. Marker genes were sequenced with 10% phiX control on the Illumina MiSeq platform (2 × 300) to determine bacterial and fungal diversity. The V4 hypervariable region of the bacterial 16S rRNA gene was targeted with primers 515F (GTGYCAGCMGCCGCGGTAA)/806R (GGACTACNVGGGTWTCTAAT) (Apprill et al. 2015). Fungal ITS2 region was targeted with primers FITS7 (GTGARTCATCGAATCTTTG)/ITS4 (TCCTCCGCTTATTGATATGC) (Ihrmark et al. 2012; Lear et al. 2018).
Bacterial and fungal microbiome bioinformatics were performed via QIIME2 2020.2 (Bolyen et al. 2019). Raw single-end sequences were demultiplexed and trimmed of primers using the q2-demux plugin followed by ‘Cutadapt’ (Martin 2011) (via q2-cutadapt). Denoising, truncation to 250 bp and chimaera removal was completed on trimmed sequences using DADA2 (Callahan et al. 2016) (via q2-dada2). A total of 42,057 unique bacterial and 23,741 unique fungal amplicon sequence variants (ASVs) were identified. Taxonomy was assigned to bacterial and fungal ASVs using the q2-feature-classifier classify-sklearn (Bokulich et al. 2018) against SILVA_132 at 99% reference sequences (Quast et al. 2012; Yilmaz et al. 2014) and UNITE ver. 8.0 Dynamic classifier (i.e. either a 97% or 99% threshold based on which is more accurate for certain lineages of fungi, determined manually by experts in the field) (Nilsson et al. 2019; Kõljalg et al. 2020; Abarenkov et al. 2024).
The bacterial and fungal abundance tables were uploaded to R for community analysis (R-Core-Team 2022). Agglomeration to the genus level was completed via phyloseq (McMurdie and Holmes 2013), which concatenated the counts off 42,057 bacterial ASVs into 2468 genera and 23,741 fungal ASVs into 800 genera. Agglomeration was performed to examine variation in the community occurring at higher taxonomic levels (i.e. genus) due to greater ecological differences found between taxonomic groups at these levels. Additionally, agglomeration removed ASV level variation that can over exaggerate ecological differences between treatments by separating phylogenetically related taxa. Further abundance filtering was completed to remove genera with mean abundance counts <10 prior to normalisation via proportions (McKnight et al. 2019). Beta-diversity was assessed through Permutational Multivariate Analysis of Variance (PERMANOVA) and Permutational Multivariate Analysis of Dispersion (PERMDISP) based on Bray-Curtis distances using RStudio packages ‘adonis2’, ‘pairwiseadonis’ and ‘betadisper’ (Anderson et al. 2006; Anderson and Walsh 2013). Pairwise PERMANOVA and PERMDISP P-value obtained through 9999 permutations were adjusted for multiple-comparisons via the Benjamini-Hochberg (i.e. False discovery rate) method (Benjamini and Hochberg 1995). Principal coordinate analysis (PCoA) was completed based on Bray-Curtis distances via ampvis2 (Andersen et al. 2018).
Differential abundance analysis was conducted on bacterial and fungal abundance tables aggregated to the genus level using the R package DESeq2 (Love et al. 2014). This methodology is tailored to identify discrepancies in abundance levels of bacterial and fungal genera between two experimental groups. It utilises shrinkage estimation techniques to account for variations in dispersions and fold changes, enhancing the precision of the analysis and providing valuable insights into the differential abundance patterns. Prior to analysis, non-normalised abundance tables were filtered to remove bacterial and fungal genera present in <10% of samples. Additional filtering removed genera with a mean relative abundance of <100 to focus on the most ecologically relevant differences. P-values were adjusted via the Benjamini–Hochberg method for multiple comparisons (Benjamini and Hochberg 1995).
Analysis of the primary ecological lifestyles of the fungal community via the FungalTraits database
Fungal genera identified as differentially abundant through DESEq2 analysis were characterised with ecological attributes such as primary lifestyle and growth form, utilising data from FungalTraits (Põlme et al. 2020; Tanunchai et al. 2023). Given that FungalTraits operates on a genus-based classification system for ecological assignments, fungi identified as differentially abundant but lacking genus-level classification were designated as ‘unknown’. However, in cases where a taxon was identified as differentially abundant and classified to the family level, ecological attributes were inferred based on the collective information provided by the FungalTraits database for all genera within that particular family.
Furthermore, FungalTraits was employed to replace the taxonomic data in the abundance table with the primary lifestyle information of each genus, following the methodology detailed by Põlme et al. (2020). Out of 800 fungi analysed, 68% (n, 544) were successfully assigned a primary lifestyle, while the remaining 32% (n, 256) were excluded to establish a prevalence table reflecting the functional composition of each sample. Subsequently, the abundances were aggregated into 21 primary lifestyles through further consolidation. DESEq2 analysis was then conducted to ascertain whether treatments at various sampling distances exhibited variations in the overall prevalence of specific primary lifestyles.
Soil physicochemical measurements on amendment band samples
Soil organic matter (SOM), total organic carbon (TOC), and soil moisture levels were assessed on a subset of the total subsoil samples by CSBP laboratories (https://csbplab.com.au/). Specifically, samples extracted from the amendment bands of all treatments (CMP, control, FN, and WS + FN) were chosen for SOM, TOC, and moisture analysis. TOC measurements were conducted using a modified Walkey-Black method (Heanes 1984), employing a concentrated sulfuric acid and dichromate solution. SOM was measured using the Loss on Ignition (LOI) method. Initially, soil samples were dried overnight at 110°C, providing an additional measure of soil moisture percentage. Subsequently, the dried samples were weighed before being heated to 400°C, and the weight loss upon ignition was used to determine the percentage of SOM.
Results
Beta-diversity analysis of the fungal taxonomic composition between treatments
PERMANOVA indicated that treatment had a significant main effect on the overall fungal community composition (F (3139) = 2.73, R2 = 0.06, P = 0.0001). Pairwise analysis highlighted significant fungal community dissimilarity between CMP and the control (F = 4.55, R2 = 0.06, Adj. P = 0.0030), WS + FN and the control (F = 3.95, R2 = 0.05, Adj. P = 0.0030), CMP and FN (F = 2.77, R2 = 0.04, Adj. P = 0.0074) and between WS + FN and FN (F = 2.02, R2 = 0.03, Adj. P = 0.0291). No community dissimilarity was found between FN and the control or between CMP and WS + FN.
Further PERMANOVA analysis was conducted via subsetting the total dataset based on sampling distance from the amendment band (i.e. 0, 14 and 28 cm from the centre of the amendment band). Treatment resulted in a significant main effect on fungal dissimilarity from samples within the amendment band (i.e. 0 cm) (F (3,44) = 2.07, R2 = 0.12, P = 0.0013). However, no treatment effect was observed at 14 cm (F (3,43) = 1.26, R2 = 0.08, P = 0.1203) or 28 cm (F (3,44) = 0.90, R2 = 0.06, P = 0.6547) from the amendment band.
Following the significant main effect result within the amendment band, pairwise PERMANOVA analysis was conducted to determine differing treatment pairs. Significant fungal dissimilarity was observed between CMP and the control (F = 3.44, R2 = 0.14, Adj. P = 0.0078) and between WS + FN and the control (F = 2.48, R2 = 0.10, Adj. P = 0.0126). Significant fungal dissimilarity was also reported between CMP and FN (F = 2.57, R2 = 0.10, Adj. P = 0.0242) while no difference was reported between WS + FN and FN. Furthermore, no significant fungal dissimilarity was reported between FN and the control, or between CMP and WS + FN samples.
PERMDISP analysis was completed to further examine the beta-diversity between treatments. Fig. 2 represents the dispersion of data points (samples) within each treatment relative to the treatment’s centroid, reflecting the average position of data points in a multivariate space based on the fungal composition. Increased distance from the centroid signifies more heterogeneity in the fungal composition of samples within the respective treatment.
Boxplot of the Multivariate Analysis of Dispersion (PERMDISP) of the fungal community composition within each experimental treatment at each sampling distance. The distance to the centroid (y-axis) is calculated based on the fungal composition of each sample within each treatment (x-axis) via Bray–Curtis distance and refers to the measure of how dispersed the data points (samples) are within each treatment, relative to the centroid (the average position of data points in a multivariate space) of that treatment. The greater this distance from the centroid, the more heterogenous the samples in fungal composition. Treatments differing in assigned letters were determined to be statistically different in dispersion at P < 0.05. (a) Within the amendment band (0 cm), (b) distance of 14 cm from the amendment band; and (c) distance of 28 cm from the amendment band. Red, chicken manure pellets (CMP); blue, reep-ripped with no amendment (control); green, fluid nutrients (FN); purple, nutrient-enhanced wheat straw (WS + FN).
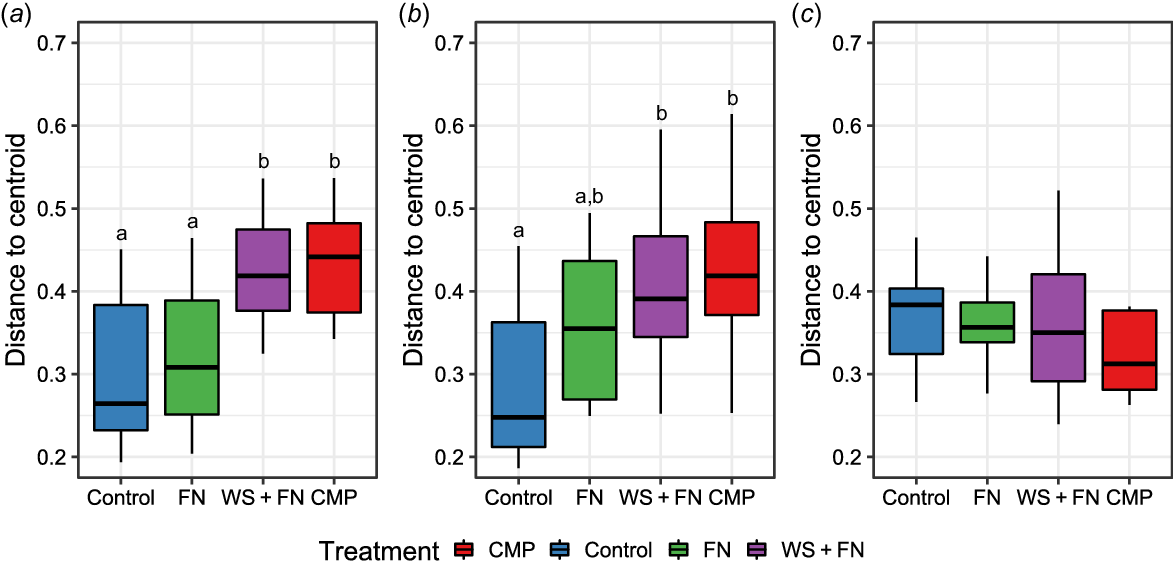
At 0 cm, an overall treatment effect was observed (F (3,44) = 9.25, P < 0.0001). Pairwise permutation analysis indicated the fungal community of CMP was significantly more heterogeneous than the control (P = 0.0003) and FN (P = 0.0015) (Fig. 2a). Likewise, the fungal composition of WS + FN was also more heterogenous than the control (P = 0.0019) and FN (P = 0.0059) (Fig. 2a). No dispersion difference was observed between FN and control or between CMP and WS + FN.
While no significant PERMANOVA result was observed between treatments at 14 cm from the amendment, a small but significant PERMDISP result was observed (F (3,43) = 3.04, P = 0.0398) (Fig. 2b). Pairwise permutation analysis indicated that dispersion differed between CMP and the control (P = 0.0237), and between WS + FN and the control (P = 0.0460) at 14 cm from the amendment band (Fig. 2b). However, at 28 cm, PERMDISP indicated no significant treatment effect on dispersion (F (3,44) = 0.16, P = 0.9232) (Fig. 2c).
Beta-diversity between experimental treatments at each sampling distance was explored visually via PCoAs. Fig. 3a demonstrated a considerable proportion of CMP and WS + FN samples were separated from the control based on ordination distance. This separation suggests that a substantial number of CMP and WS + FN samples were dissimilar in fungal taxonomic composition compared to control within the amendment band, which is supports the significant PERMANOVA result. Additionally, Fig. 3a shows that both CMP and WS + FN samples are considerably more dispersed across the ordination space than those associated with the control, which is supported by the significant PERMDISP result.
Principal coordinates analysis (PCoA) of the fungal genus level composition associated with each sample within each experimental treatment based on Bray–Curtis distance. (a) Samples taken from (a) within the amendment band (0 cm), (b) 14 cm from the amendment band, and (c) 28 cm from the amendment band. Red, chicken manure pellets (CMP); blue, deep-ripped with no amendment (control); green, fluid nutrients (FN); purple, nutrient-enhanced wheat straw (WS + FN). Within-group variability is visualised as treatment point dispersion across the ordination space (i.e. more dispersed = greater within-group variability. Distance between sample points indicates the degree of fungal community dissimilarity (i.e. closer sample points = more similar fungal community composition).
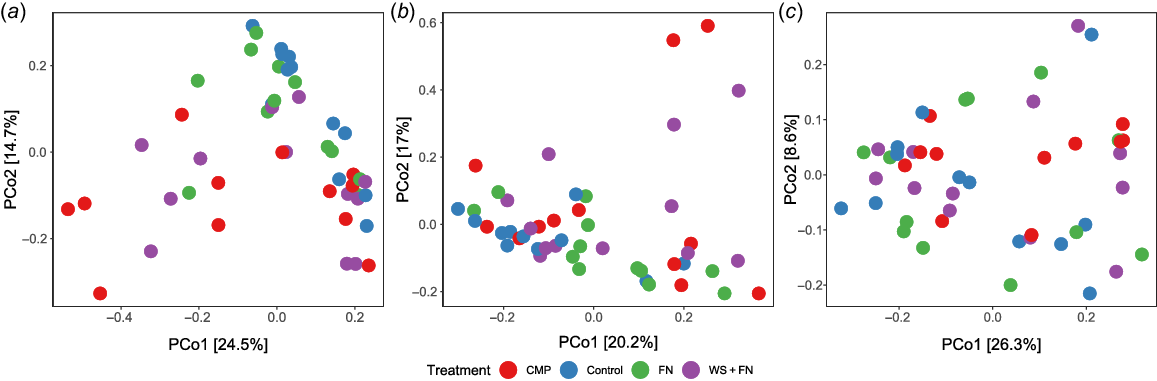
At a distance of 14 cm from the amendment band, a notable decrease in the spatial separation between most CMP and WS + FN samples was evident when compared to both the control and FN samples (Fig. 3b). This suggests a majority of CMP and WS + FN samples were more similar in fungal composition to the control and FN samples at 14 cm from the amendment band than those taken from within the amendment band (Fig. 3a), which is supported by an insignificant PERMANOVA result. Nevertheless, it is apparent that CMP and WS + FN exhibited diminished yet noticeably greater dispersion among samples compared to the control across the ordination space, a finding corroborated by the modest yet statistically significant PERMDISP outcome (Fig. 3b).
At 28 cm from the amendment band (Fig. 3c), all treatments displayed similar fungal beta-diversity in terms of both inter-treatment distances and dispersion, which further supports absence of significant results in both PERMANOVA and PERMDISP.
Based on the findings from PERMANOVA, PERMDISP, and PCoA analyses, the fungal community associated with CMP and WS + FN within the amendment band exhibited notable dissimilarity from the control, alongside considerable heterogeneity across sampling locations. However, at a distance of 14 cm from the amendment band, the instances of samples with distinct community compositions compared to the control decreased, with community composition displaying greater homogeneity albeit with moderate variability. Conversely, at 28 cm from the amendment band, all treatments demonstrated similarity in fungal composition and within-group variability.
Beta-diversity analysis of the bacterial taxonomic composition between treatments
PERMANOVA analysis indicated no significant main effect of treatment on the overall bacterial community composition (F (3139) = 1.58, R2 = 0.03, P = 0.0543). Further examination by subsetting the dataset based on sampling distance (0, 14, and 28 cm) revealed no dissimilarity in bacterial community between treatments at 0 cm (F (3,44) = 1.20, R2 = 0.08, P = 0.2365), 14 cm (F (3,43) = 0.72, R2 = 0.05, P = 0.8122), or 28 cm (F (3,44) = 0.84, R2 = 0.05, P = 0.6439) from the amendment band.
PERMDISP analysis underscored that treatment type did not exert a significant impact on dispersion concerning the bacterial community composition at 0 cm (F (3,44) = 0.43, P = 0.7326) (Fig. 4a), 14 cm (F (3,43) = 0.08, P = 0.9720) (Fig. 4b) and 28 cm (F (3,44) = 0.23, P = 0.8755) (Fig. 4c) from the amendment band. Furthermore, PCoAs conducted on the bacterial community composition at each sampling distance visually showed the highly analogous distribution and dispersion of treatment samples, leading to non-significant outcomes in both PERMANOVA and PERMDISP analyses (Fig. 5a–c).
Boxplot of the Permutational Multivariate Analysis of Dispersion (PERMDISP) of the bacterial community composition within each experimental treatment at each sampling distance. The distance to the centroid (y-axis) is calculated based on the bacterial composition of each sample within each treatment (x-axis) via Bray–Curtis distance and refers to the measure of how dispersed the data points (samples) are within each treatment, relative to the centroid (the average position of data points in a multivariate space) of that group. The greater this distance from the centroid, the more heterogenous the samples in bacterial composition. Treatments differing in assigned letters were determined to be statistically different in dispersion at P < 0.05, while no lettering indicates no significant differences in dispersion between treatments. (a) Within the amendment band (0 cm), (b) distance of 14 cm from the amendment band, and (c) distance of 28 cm from the amendment band. Red, chicken manure pellets (CMP); blue, deep-ripped with no amendment (control); green, fluid nutrients (FN); purple, nutrient-enhanced wheat straw (WS + FN).
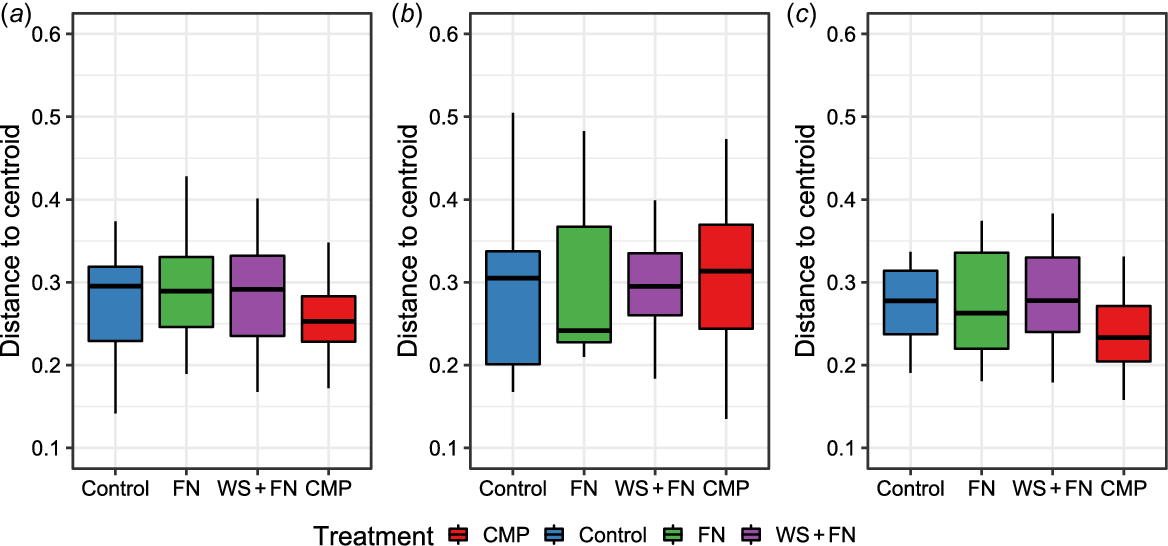
Principal coordinates analysis (PCoA) of the bacteria genus level composition associated with each sample within each experimental treatment based on Bray–Curtis distance. Samples taken from (a) within the amendment band (0 cm), (b) 14 cm from the amendment band; and (c) 28 cm from the amendment band. Red, chicken manure pellets (CMP); blue, deep-ripped with no amendment (control); green, fluid nutrients (FN); purple, nutrient-enhanced wheat straw (WS + FN). Within-group variability is visualised as treatment point dispersion across the ordination space (i.e. more dispersed = greater within-group variability. Distance between sample points indicates the degree of bacterial community dissimilarity (i.e. closer sample points = more similar bacterial community composition).
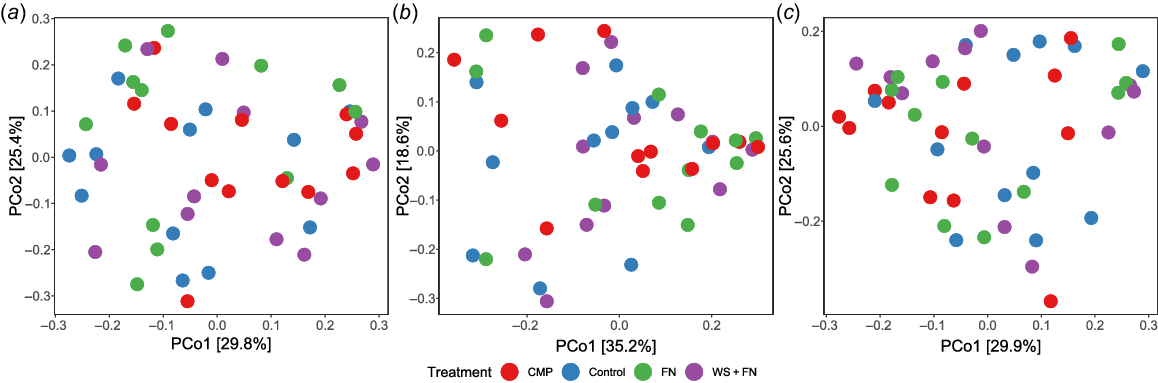
Differential abundance analysis of the fungal genera between treatments
After obtaining significant beta-diversity results, DESeq2 analysis was conducted to compare the differentially abundant fungal genera in CMP and WS + FN relative to the control within the amendment band (0 cm) and at a distance of 14 cm from the amendment band. However, no differential abundance analysis was performed at 28 cm, nor on the bacterial community composition, due to the non-significant results obtained from PERMANOVA and PERMDISP analyses.
A total of 28 fungal genera exhibited differential abundance in CMP samples obtained from within the amendment band compared to the control (Table 1a). Among these, 21 (75.0%) were significantly enriched in CMP, and seven (25.0%) were found to be in lower abundance compared to the control. Notably, 14 (66.6%) of the fungal genera showing increased abundance in CMP were categorised as saprotrophic, with 12 of them classified as filamentous mycelium. Moreover, a higher abundance of potentially plant pathogenic genera (i.e. Fusarium, Drechslera, and Gibberella) was observed in CMP samples compared to the control. Eight fungal genera displayed an adjusted P-value of <0.0001, all of which were found to be in higher abundance in CMP.
Genus | Ecological lifestyleA | %B | LFCC | Adj. P D | |
---|---|---|---|---|---|
(A) Within the amendment band | |||||
Botryotrichum | Saprotroph (F) | 4.92 | 9.76 | <0.0001**** | |
Unknown Microascaceae | Saprotroph (F) | 3.45 | 9.64 | <0.0001**** | |
Unknown Gymnoascaceae | Saprotroph (F) | 0.28 | 7.19 | <0.0001**** | |
Sordaria | Saprotroph (F) | 0.64 | 6.73 | <0.0001**** | |
Scopulariopsis | Saprotroph (F) | 0.51 | 6.59 | 0.0008*** | |
Clitopilus | Saprotroph (F) | 0.31 | 6.50 | <0.0001**** | |
Cephalotrichum | Saprotroph (F) | 0.37 | 5.25 | 0.0001*** | |
Gibberella | Plant pathogen | 0.52 | 4.26 | <0.0001**** | |
Apophysomyces | Saprotroph (F) | 0.38 | 4.16 | 0.0001*** | |
Clonostachys | Saprotroph (F) | 1.00 | 3.37 | <0.0001**** | |
Unknown Basidiomycota | Unknown | 0.59 | 3.21 | 0.0001*** | |
Drechslera | Plant pathogen (F) | 0.41 | 3.03 | 0.0004*** | |
Chrysosporium | Saprotroph (F) | 0.66 | 2.97 | <0.0001**** | |
Unknown Ascomycota | Unknown | 1.15 | 2.60 | 0.0002*** | |
Humicola | Saprotroph (F) | 1.07 | 1.91 | 0.0084** | |
Fusarium | Plant pathogen (F) | 5.34 | 1.89 | 0.0011** | |
Unknown Onygenales | Unknown | 1.35 | 1.77 | 0.0175* | |
Solicoccozyma | Saprotroph | 8.69 | 1.65 | 0.0008*** | |
Lachancea | Saprotroph | 0.25 | 1.51 | 0.0081** | |
Penicillium | Saprotroph (F) | 0.84 | 1.35 | 0.0023** | |
Unknown Fungi | Unknown | 3.40 | 1.15 | 0.0163* | |
Unknown Powellomycetaceae | Saprotroph | 0.22 | −0.93 | 0.0248* | |
Unknown Extremaceae | Unknown | 0.21 | −1.01 | 0.0175* | |
Pleuroascus | Saprotroph (F) | 0.94 | −1.10 | 0.0406* | |
Unknown Tremellales | Unknown | 0.48 | −1.20 | 0.0012** | |
Unknown Lasiosphaeriaceae | Saprotroph (F) | 0.30 | −1.88 | 0.0127* | |
Stachybotrys | Saprotroph (F) | 1.41 | −2.13 | 0.0004*** | |
Podospora | Saprotroph (F) | 0.86 | −2.86 | 0.0023** | |
(B) 14 cm from amendment band | |||||
Sordaria | Saprotroph (F) | 0.28 | 4.51 | 0.0109* | |
Unknown Gymnoascaceae | Saprotroph (F) | 0.15 | 4.44 | <0.0001**** | |
Botryotrichum | Saprotroph (F) | 2.55 | 3.57 | 0.0074** | |
Unknown Microascaceae | Saprotroph (F) | 2.71 | 3.41 | 0.0074** | |
Unknown Ascomycota | Unknown | 1.92 | 3.08 | 0.0057** | |
Oidiodendron | Saprotroph (F) | 0.96 | 2.51 | 0.0388* | |
Latorua | Saprotroph (F) | 0.68 | 2.45 | 0.0114* | |
Chrysosporium | Saprotroph (F) | 0.62 | 1.89 | 0.0074** | |
Unknown Onygenales | Unknown | 0.78 | 1.86 | 0.0388* | |
Unknown Fungi | Unknown | 3.87 | 1.31 | 0.0208* | |
Podospora | Saprotroph (F) | 0.38 | −2.57 | 0.0179* | |
Ilyonectria | Plant pathogen | 0.23 | −3.13 | 0.0208* |
At a distance of 14 cm, findings revealed differential abundance in 12 fungal genera in CMP samples compared to the control (Table 1b). Among these, 10 (83.3%) were found to be in higher abundance in CMP samples, while two (16.7%) were in lower abundance. Notably, eight (80.0%) of the fungal genera exhibiting enrichment in CMP samples were classified as saprotrophic, all falling under the category of filamentous mycelium. These results underscore that the fungal response to CMP is most pronounced within the amendment band, with a noticeable reduction in the number of differentially abundant genera observed at 14 cm away from the amendment band.
A total of 22 fungal genera were reported as differentially abundant in WS + FN samples taken from within the amendment band compared to the control (Table 2a). Eighteen (81.8%) were determined to be enriched in WS + FN samples and four (18.2%) were in a lower abundance. Eleven (61.1%) of the fungal genera shown to be enriched in WS + FN samples were saprotrophic, and all were classified as filamentous mycelium. Five fungal genera reported an adjusted P-value of <0.0001 and four were observed in increased relative abundance in WS + FN.
Genus | Ecological lifestyleA | %B | LFCC | Adj. P D | |
---|---|---|---|---|---|
(A) Within the amendment band | |||||
Scopulariopsis | Saprotroph (F) | 0.51 | 12.43 | <0.0001**** | |
Botryotrichum | Saprotroph (F) | 4.92 | 9.20 | <0.0001**** | |
Clitopilus | Saprotroph (F) | 0.31 | 4.51 | 0.0108* | |
Gibberella | Plant pathogen | 0.52 | 4.29 | 0.0001*** | |
Unknown Microascaceae | Saprotroph (F) | 3.45 | 4.25 | 0.0053** | |
Unknown Basidiomycota | Unknown | 0.59 | 3.51 | 0.0001*** | |
Apophysomyces | Saprotroph (F) | 0.38 | 2.81 | 0.0147* | |
Conocybe | Saprotroph (F) | 1.38 | 2.80 | 0.0437* | |
Trichoderma | Mycoparasite (F) | 0.32 | 2.52 | 0.0089** | |
Chrysosporium | Saprotroph (F) | 0,66 | 2.41 | 0.0016** | |
Trechispora | Saprotroph (F) | 0.49 | 2.26 | 0.0276* | |
Unknown Gymnoascaceae | Saprotroph(F) | 0.28 | 2.25 | 0.0385 * | |
Unknown Ascomycota | Unknown | 1.15 | 2.05 | 0.0076** | |
Metarhizium | Animal parasite (F) | 1.65 | 2.04 | 0.0147* | |
Pseudeurotium | Saprotroph (F) | 1.85 | 1.98 | 0.0146* | |
Unknown Hypocreales | Unknown | 2.07 | 1.83 | 0.0131* | |
Unknown Fungi | Unknown | 3.40 | 1.21 | 0.0076** | |
Penicillium | Saprotroph (F) | 0.84 | 1.17 | 0.0147** | |
Pleuroascus | Saprotroph (F) | 0.94 | −1.52 | 0.0053** | |
Unknown Tremellales | Unknown | 0.48 | −1.56 | <0.0001**** | |
Unknown Lasiosphaeriaceae | Saprotroph (F) | 0.30 | −1.89 | 0.0147* | |
Rhizophlyctis | Saprotroph | 0.39 | −2.99 | 0.0005*** | |
(B) 14 cm from amendment band | |||||
Botryotrichum | Saprotroph (F) | 2.55 | 5.68 | <0.0001**** | |
Unknown Microascaceae | Saprotroph (F) | 2.71 | 3.66 | 0.0043** | |
Conocybe | Saprotroph (F) | 2.43 | 3.49 | 0.0136* | |
Stachybotrys | Saprotroph (F) | 0.93 | −1.74 | 0.0220* |
At a depth of 14 cm, four distinct fungal genera exhibited varying abundance levels in WS + FN samples compared to the control group (Table 2b). Among these, three genera (75.0%) were notably more abundant in WS + FN samples, while one genus (25.0%) showed decreased abundance. Notably, all three fungal genera (100%) that exhibited increased abundance in WS + FN samples were categorised as saprotrophs and filamentous mycelium. These findings underscore a pronounced fungal response to WS + FN within the amendment band, while also highlighting a reduction in the number of differentially abundant fungi at 14 cm, echoing similar trends observed in CMP analysis.
Differential abundance analysis of the fungal ecological composition between treatments
After observing a notable variation in saprotrophic fungal genera between CMP and WS + FN when compared to the control (Tables 1a and 2a), DESeq2 analysis was conducted to assess the overall fungal functional composition across treatments. The abundance of fungi classified as ‘unspecified saprotrophs’ was greater in CMP (% total community = 11.57, LFC = 1.89, adj. P = 0.0048) and WS + FN (% total community = 11.57, LFC = 1.55, adj. P = 0.0264) compared to the control using samples taken from within the amendment band. At 14 cm, ‘unspecified saprotroph’ abundance continued to be greater in CMP (% total community = 10.37, LFC = 1.55, adj. P = 0.0439) and WS + FN (% total community = 10.37, LFC = 2.02, adj. P = 0.0013) samples compared to the control.
Analysis of soil organic matter and total organic carbon content between treatments
Percentages of SOM, TOC, and soil moisture was analysed for each treatment using samples taken from within the amendment band. ANOVA indicated no significant difference in the mean percentages of SOM (F (3,44) = 0.98, P = 0.412), TOC (F (3,44) = 0.39, P = 0.759), and soil moisture (F (3,44) = 2.83, P = 0.050) between treatments and the control. Fig. 6 demonstrates visually the range of SOM and TOC measurements associated with samples within each treatment. Fig. 6c demonstrated that the percentage soil moisture of CMP samples appeared lower than the control, which was the primary driver behind the low P-value (P = 0.050) indicated via ANOVA.
Boxplot displaying the distribution of (a) soil organic matter, (b) total organic carbon, and (c) soil moisture measurements (%) associated with samples taken from within (0 cm) the amendment band of each experimental treatment. Treatments differing in assigned letters were determined to be statistically different in dispersion at P < 0.05. Red, chicken manure pellets (CMP); blue, eeep-ripped with no amendment (control); green, fluid nutrients (FN); purple, nutrient-enhanced wheat straw (WS + FN).
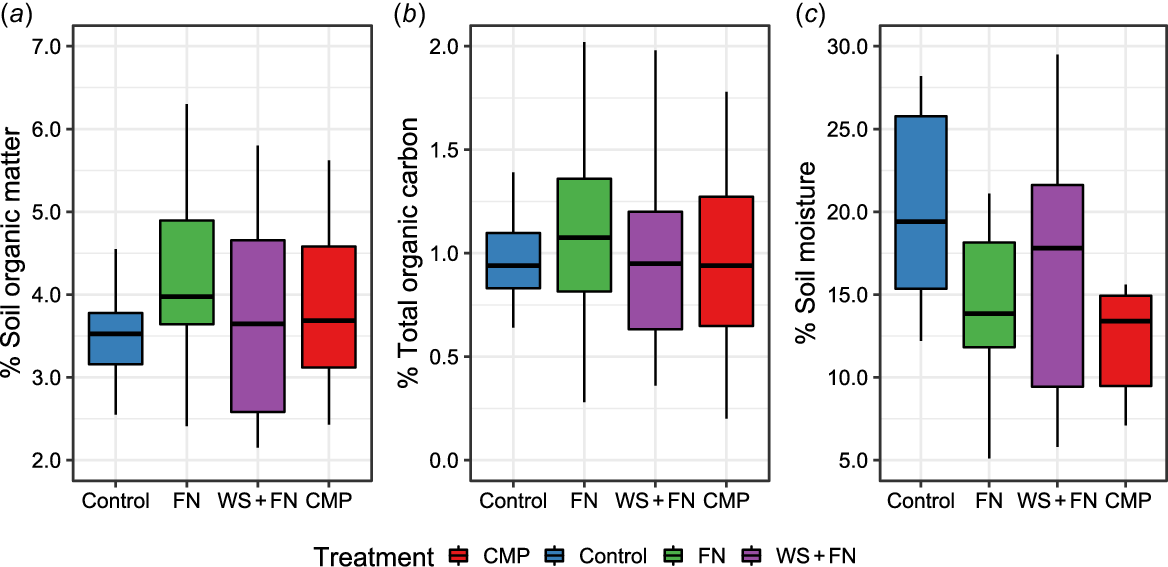
PERMANOVA indicated TOC had a significant impact on fungal community composition (F (1,46) = 4.90, R2 = 0.10, P = 0.0001), and SOM (F (1,46) = 2.52, R2 = 0.05, P = 0.0093) and moisture (F (1,46) = 2.20, R2 = 0.04, P = 0.0162) also significantly influenced fungal composition (F (1,46) = 2.52, R2 = 0.05, P = 0.0093). However, no significant PERMANOVA interaction was observed between treatment and TOC (F = 1.40, R2 = 0.07, P = 0.0701), SOM (F = 0.76, R2 = 0.04, P = 0.8458) and moisture (F = 0.86, R2 = 0.05, P = 0.6938).
Additionally, SOM (F (1,46) = 4.07, R2 = 0.08, P = 0.0011), TOC (F (1,46) = 4.91, R2 = 0.10, P = 0.0002) and moisture (F (1,46) = 6.44, R2 = 0.12, P = 0.0001) had a significant impact on bacterial community composition. Similar to the fungal results, no interaction effect was observed between treatment and SOM (F = 0.53, R2 = 0.03, P = 0.9809), TOC (F = 0.93, R2 = 0.05, P = 0.5435) and soil moisture (F = 0.68, R2 = 0.04, P = 0.8734) regarding the bacterial community composition.
Discussion
Fungi dominate the extended microbial response to deep-banded organic amendments
Our analysis revealed a distinct fungal community composition in soil treated with CMP and WS + FN compared to deep-ripped unamended soil (control). However, no significant dissimilarity was observed between the unamended control and soil treated with FN alone. This suggests that only amendments incorporating organic substrates, (i.e. carbon substrate), induced a more pronounced and extended fungal response, surpassing the impact of the mechanical deep-ripping process.
Our results demonstrated that the fungal community responses to CMP and WS + FN were mostly localised within the amendment band, with only a small dispersion difference noted 14 cm away in the bulk subsoil. Organic amendments, such as CMP and WS + FN, are posited to induce heightened localised legacy effects on soil physicochemistry (Uddin et al. 2022). This phenomenon is predominantly attributed to the gradual nutrient release and the introduction of diverse carbon and nitrogen sources facilitated by the incorporation of complex organic substrates into the subsoil (Liu et al. 2017; Uddin et al. 2022). Consequently, the incorporation of organic substrates into the subsoil results in a slower decomposition rate and promotes fungal breakdown and utilisation of the incorporated complex C-substrates (López-Mondéjar et al. 2018; Yu et al. 2022; Raza et al. 2023). In contrast, the inorganic fluid nutrients used in this study provide readily available nutrients to the microbial communities and promote a rapid microbial response that may be short-lived (Clark et al. 2007). As a result, the organic amendments promoted a fungal community shift 2 years following deep-banding, while the inorganic fluid nutrients did not.
Restrictions on microbial activity are likely to be imposed by the unfavourable conditions in the dense clay subsoils such as high sodicity, low oxygen, variable water content, and high bulk density, which are likely to contribute to reduced decomposition rates of organic substrates. However, our findings indicated that, after a 2-year period, the levels of SOM/TOC in the treated soils closely resembled those in the control group, suggesting the absence of the initially introduced organic materials. Furthermore, no interaction effect was observed between treatment and SOM/TOC on both bacterial and fungal composition. However, notable distinctions in fungal community composition were still observed within the amendment bands of both CMP and WS + FN treated soils. This suggests that the influence of organic material from CMP and WS + FN may endure beyond the restoration of SOM/TOC levels to baseline. We posit that the observed variations in the fungal community within the amendment band of CMP and WS + FN cannot be attributed to persistent alterations in soil physicochemical properties, specifically pH, in conjunction with SOM, TOC, and moisture. This assertion is grounded in the absence of discernible bacterial community dissimilarity between treatments, as bacterial communities are known to be markedly more sensitive to shifts in soil pH than fungi (Rousk et al. 2010; Tripathi et al. 2016; Cruz-Paredes et al. 2021; Wang et al. 2022).
This persistence in fungal community alterations may be attributed to several fungal-specific traits, namely their multicellular nature, slower growth, and turnover as well as their resilient cell wall construction (specifically the presence of chitin) (Nitsche et al. 2012; Yu et al. 2022). The intrinsic resilience of fungi to environmental changes and nutrient storage abilities substantiates their ability to maintain community-level impacts even when the elevated C substrate concentrations in the soil, which drive the variation, have become undetectable. Consequently, despite the reversion of SOM and TOC concentrations, the lasting impact of CMP and WS + FN on fungal communities persists.
Both CMP and WS + FN amendments resulted in significant variability in the fungal composition between replicate samples, which was found to be greater than the variability observed between replicate control samples. This was observed mostly within the amendment band (Figs 2a and 3a) but was still evident 14 cm from the band (Figs 2b and 3b). Soil is heterogenous due to high spatial variability in physicochemical properties such as pH, soil organic matter, nutrient availability, and moisture content (MacEwan et al. 2010; Li et al. 2021). Consequently, a significant amount of fungal community variability is to be expected between replicate samples across a large field site environment (Gravuer et al. 2020). As the impact of fertilisers/manures on fungal communities is dependent on the soil environment within the localised point of placement, the variation in the fungal response between amendment replicates is likely to exceed the ‘naturally occurring’ variation observed across the field site (Heinze et al. 2010). This explanation aligns strongly with our findings.
Furthermore, the steering of the tractor was not guided by GPS and was subject to operator error, meaning that the amendment bands were not positioned in perfectly straight lines along the plots. This may have resulted in imprecise identification and sampling of the actual amendment band – which was based on a taught string line between the rip-line identifiers (at the end of the 20-m plots). In combination with an heterogenous fungal response to the amendments, this may have also contributed to further variability in fungal community composition between replicate samples for the amendments. Moreover, considering the absence of discernible variations in composition and minimal dispersion differences among treatments 14 cm from the amendment band, we assert that the fungal community’s response was predominantly confined to the amendment band. Hence, the observed dispersion differences at 14 cm are likely attributable to inherent soil heterogeneity rather than being indicative of a treatment-induced effect.
Despite this variability in community composition, differential abundance analysis demonstrated that there were 21 and 19 fungal genera that increased significantly within the CMP and WS + FN amendment bands, respectively, as compared with the control (Table 1). This enrichment also occurred 14 cm from the band, but significantly fewer genera were involved. Thus, the organic amendments provided a significant enrichment in specific fungal taxa. However, there were differences between the CMP and the WS + FN amendments in the fungal genera that were significantly enriched, compared to the control (Tables 1 and 2). Around half of the genera enriched by CMP in the amendment band were not affected by WS + FN, and around a third of the genera enriched by WS + FN were unaffected by CMP. Furthermore, of the fungal genera enriched by both these amendments, CMP resulted in greater enrichment in most genera compared to WS + FN. As CMP contains more diverse organic substrates (i.e. bedding such as sawdust or wood shavings, faeces, feathers, and spilled feed) compared to wheat straw, we hypothesise that this difference in amendment chemistry contributed to a more variable fungal response.
Bacterial community changes were undetectable 2 years after the addition of deep-banded amendments
Bacterial beta-diversity analysis indicated no differences between the amendment treatments and the control 2 years after amendment placement. Celestina et al. (2019c) also reported undetectable bacterial changes 3 years after deep-banded amendment addition in a similar field site in south-east Australia. However, deep-banded amendments are known to drive changes in bacterial communities in short-term controlled environment experiments (Celestina et al. 2019a, 2019b; Wang et al. 2020).
The lack of readily available nutrients and the absence of active root growth may have contributed to an undetectable bacterial response. CO2 respiration in amended soil was determined to initially spike before becoming similar to that of unamended soil after 174 days of incubation (Clark et al. 2007). Whilst this study was conducted under controlled conditions in the absence of plant growth, it suggests that microbial activity initially spikes indicating the metabolism of readily available nutrients before activity decreases on account of subsequent nutrients depletion. Therefore, we hypothesise the bacterial community response is mostly restricted to this initial window of increased nutrient availability, which supports the findings of previous studies (Celestina et al. 2019a, 2019b; Wang et al. 2020). However, fungi are acknowledged to be the primary decomposers of SOM and have a greater capacity to degrade a wider and more recalcitrant range of substrates (Yu et al. 2022). Therefore, we hypothesise that once the readily available nutrients within the amendments are depleted, bacterial communities may mostly revert back to pre-amendment composition, while the fungal community remains altered through their enhanced ability to consume the complex and recalcitrant organic material.
Previous research has reported that the bacterial response to deep-banded amendments is primarily enhanced by plant root proliferation and rhizodeposition, with a relatively minimal direct response to the amendments (Vido et al. 2024). As sampling for this study was conducted in March 2020, some 4 months after plants from the 2019 barley crop had senesced and died, active root growth in the subsoil was no longer present. Consequently, any rhizosphere bacterial response driven by amendment-enhanced root growth and rhizodeposition would be undetected by this study.
Filamentous fungi dominated the positive response to CMP and WS + FN. This particular growth trait is likely to have provided an advantage over bacteria in accessing organic substrate banded in dense-clay subsoils. This is due to the fact that filamentous growth allows fungi to autonomously move through the soil matrix, while the distribution of single celled bacteria is heavily reliant on water movement and restricted by pore spaces (Boswell et al. 2003; Yang and van Elsas 2018). Therefore, in poorly structured subsoils with high bulk density and limited water infiltration, filamentous fungal movement may not be as restricted as compared to bacteria, allowing them greater access to the banded organic substrate and to dominate the overall microbial response.
Moreover, the microbial composition, encompassing both bacteria and fungi, exhibited sensitivity to SOM/TOC levels throughout the entire field site, irrespective of treatment conditions. However, despite the absence of discernible differences in SOM/TOC between treatments, fungal composition differed based on treatment, while bacterial composition did not. This finding suggests fungi exhibit greater resilience which allows them to persist within the soil, despite the elevated SOM/TOC levels which initially stimulated their community shift returning to baseline.
Unravelling the ecological significance of the fungal response to deep-banded organic amendments
More than half of the fungal genera reported to have increased abundance in both CMP and WS + FN were classified as saprotrophic. Furthermore, these amendments resulted in a significantly higher abundance of ‘unspecified saprotrophs’, which comprised a substantial proportion of the total functional community. This was observed both within the amendment band as well as 14 cm away. Saprotrophic fungi receive nutrients from non-living organic matter such as crop residues, which means they are critical in agricultural C transformations and plant productivity (Cooke and Rayner 1984; Clocchiatti et al. 2020). Furthermore, the saprotrophic fungal community was dominated by filamentous taxa that are known to be associated with the formation of soil macroaggregates via hyphal binding of soil particles and organic matter (Beare et al. 1997; Nichols and Halvorson 2013; Wang et al. 2020). Given that the major objective of using deep-banded organic amendments is to improve subsoil aggregation and structure, this finding that filamentous fungi were persisting in the subsoil, 2 years after organic amendment placement, is of significance. Additionally, as these amendments were placed within the subsoil where oxygen is expected to be limited, an enrichment in aerobic fungi may also suggest increased oxygen levels which could be attributed to soil structural modifications (Cooke and Rayner 1984). Previous research has shown mechanical disruption of the soil from tillage and potentially deep-ripping negatively impacts these fungal communities through damaging mycelial networks (Beare et al. 1997; Young and Ritz 2000; Verbruggen and Toby Kiers 2010). However, the addition of organic amendments may remediate some of the negative impact of the mechanical disruption necessary for amendment deep-placement, demonstrated by the enriched saprotrophic community associated with CMP and WS + FN.
It is likely that the organic material introduced by the deep placement of CMP and WS + FN was the main driver of fungal community composition change within the amendment band (Gill et al. 2009; Uddin et al. 2022). This is supported by FN, which lacked C substrates, having no detectable impact on fungal communities and the enrichment of saprotrophic fungal genera. The addition of manures and plant residues to soil has previously been shown to enhance saprotrophic fungal communities as they rely on complex non-living organic material as a nutrient source (Cooke and Rayner 1984; Clocchiatti et al. 2020; Ye et al. 2021). However, while the enrichment of fungal saprotrophs may suggest the persistence of organic substrates in the subsoil for an extended timeframe, no differences in SOM and TOC were observed between treatments within the amendment band.
We propose that the enduring enrichment of fungal saprotrophs and alterations in fungal community composition constitute a legacy effect stemming from the introduction of organic matter. Despite SOM/TOC concentrations in CMP and WS + FN being equivalent to baseline levels at the time of sampling, the deep-banding application of CMP and WS + FN would have significantly elevated SOM/TOC concentrations. This elevation facilitated the enrichment of fungal saprotrophs, utilising the introduced organic material, leading to a distinct alteration in community composition compared to the control. However, over a 2-year period, SOM/TOC levels gradually decreased as a result of microbial consumption, eventually reaching equivalence with the control. Our hypothesis posits that the fungal community changes induced by the organic material introduced by CMP and WS + FN persisted despite the decline in SOM/TOC concentrations. This suggests a sustained impact on fungal community dynamics, highlighting the intricate and lasting consequences of organic matter amendments on soil microbial ecology.
Saprotrophic fungal enrichment may also be in response to increased crop root biomass in the subsoil both within the amendment band and 14 cm away. Enhanced crop root growth within the soil surrounding deep-banded amendments has been well documented across both controlled column and field-based studies (Celestina et al. 2018, 2019b, 2019d; Sale et al. 2019; Wang et al. 2020; Armstrong et al. 2021; Vido et al. 2024). More specifically, Jin et al. (2023) reported a significant increase in leaf N concentrations and shoot biomass in wheat and barley crops grown in soil treated with CMP at this study site in 2018 and 2019, respectively. This outcome implies enhanced nutrient acquisition and consequently increased root biomass in these crops. Thus, the observed changes in the fungal community may additionally be linked to increases in root biomass from previous crop rotations. Similar to the way that fungi respond to organic material introduced by amendments, increased root biomass in response to the amendments could elicit a comparable fungal community change. Fungal saprotrophs are known contributors to the decomposition of plant residues in soil, and the heightened decomposition of root biomass in the amended soil likely contributed to their enrichment, as indicated by previous studies (Cooke and Rayner 1984; Crowther et al. 2012).
The increased abundance of saprotrophs observed at a distance of 14 cm from the amendment band suggests that the influence of organic amendments on saprotroph communities may extend into the bulk soil. The predominant positive response of filamentous genera to CMP and WS + FN implies that this growth form confers a selective advantage, enabling these fungi to autonomously proliferate beyond the initial enrichment zone within the amendment band. However, we are hesitant to draw strong conclusions on the results obtained at 14 cm due to the potential human errors involved in deep-banding, no composition differences and weak statistical dispersion differences at this sampling distance, as well as evident soil heterogeneity. Therefore, we resolve that the fungal community response, and the enrichment of saprotrophic taxa was primarily restricted to within the amendment band of soil treated with CMP and WS + FN.
Conclusion
Chicken manure pellets and nutrient-enhanced wheat straw were found to sustain fungal community differences in the subsoil 2 years after placement, while fluid inorganic nutrients did not achieve this outcome. This demonstrates the advantage of organic amendments in facilitating prolonged fungal community responses. Additionally, no sustained bacterial community changes were detected to any of the three amendments. The majority of the fungal response to these organic amendments occurred within the amendment band, demonstrating a highly localised impact. As fungal saprotrophs dominated the positive response to both of these amendments, we hypothesise that the complex organic materials in chicken manure pellets and wheat straw pieces in the nutrient enhanced wheat straw were able to facilitate a fungal community response which could persist in the subsoil for 2 years following their deep placement. In combination with the reported increased crop root growth from previous years, this contributed to a sustained enrichment in saprotrophic fungal abundance in the subsoil. However, as no treatment differences in SOM/TOC were detected, we hypothesise that the fungal community variations induced by increased organic material through the amendments and enhanced root biomass are a legacy effect which persisted after the SOM/TOC levels returned to baseline. As filamentous fungal saprotrophs are of significant importance in agricultural systems in terms of their role in C and N cycling and soil aggregation, deep-banding these organic amendments is therefore a useful practice given that it provides a way of enriching these fungi in the subsoil for extended time periods. Taken together, our findings highlight the novel impact a single application of deep-banded amendments has on enriching agriculturally significant fungi within problematic subsoils.
Data availability
The unprocessed sequencing data generated and utilised for the current study is available in the NCBI Sequence Read Archive (SRA) repository. Bacterial 16S rRNA sequences available at http://www.ncbi.nlm.nih.gov/bioproject/967047 (BioProject ID: PRJNA967047). Fungal ITS sequences available at http://www.ncbi.nlm.nih.gov/bioproject/967110 (BioProject ID: PRJNA967110). Processed data tables utilised for analysis are available upon request.
Declaration of funding
This research was supported by the Grains Research and Development Corporation projects funding scheme (project DAV00149).
References
Abarenkov K, Nilsson RH, Larsson K-H, Taylor AFS, May TW, Frøslev TG, Pawlowska J, Lindahl B, Põldmaa K, Truong C, Vu D, et al. (2024) The UNITE database for molecular identification and taxonomic communication of fungi and other eukaryotes: sequences, taxa and classifications reconsidered. Nucleic Acids Research 52, D791-D797.
| Crossref | Google Scholar | PubMed |
Anderson MJ, Walsh DCI (2013) PERMANOVA, ANOSIM, and the Mantel test in the face of heterogeneous dispersions: what null hypothesis are you testing? Ecological Monographs 83, 557-574.
| Crossref | Google Scholar |
Anderson MJ, Ellingsen KE, McArdle BH (2006) Multivariate dispersion as a measure of beta diversity. Ecology Letters 9, 683-693.
| Crossref | Google Scholar | PubMed |
Andersen KS, Kirkegaard RH, Karst SM, Albertsen M (2018) ampvis2: an R package to analyse and visualise 16S rRNA amplicon data. BioRxiv 299537.
| Google Scholar |
Apprill A, McNally S, Parsons R, Weber L (2015) Minor revision to V4 region SSU rRNA 806R gene primer greatly increases detection of SAR11 bacterioplankton. Aquatic Microbial Ecology 75, 129-137.
| Crossref | Google Scholar |
Beare MH, Hu S, Coleman DC, Hendrix PF (1997) Influences of mycelial fungi on soil aggregation and organic matter storage in conventional and no-tillage soils. Applied Soil Ecology 5, 211-219.
| Crossref | Google Scholar |
Benjamini Y, Hochberg Y (1995) Controlling the false discovery rate: a practical and powerful approach to multiple testing. Journal of the Royal Statistical Society: Series B (Methodological) 57, 289-300.
| Crossref | Google Scholar |
Bokulich NA, Kaehler BD, Rideout JR, Dillon M, Bolyen E, Knight R, Huttley GA, Gregory Caporaso J (2018) Optimizing taxonomic classification of marker-gene amplicon sequences with QIIME 2’s q2-feature-classifier plugin. Microbiome 6, 90.
| Crossref | Google Scholar |
Bolyen E, Rideout JR, Dillon MR, Bokulich NA, Abnet CC, Al-Ghalith GA, Alexander H, Alm EJ, Arumugam M, Asnicar F, Bai Y, Bisanz JE, Bittinger K, Brejnrod A, Brislawn CJ, Brown CT, Callahan BJ, Caraballo-Rodríguez AM, Chase J, Cope EK, Da Silva R, Diener C, Dorrestein PC, Douglas GM, Durall DM, Duvallet C, Edwardson CF, Ernst M, Estaki M, Fouquier J, Gauglitz JM, Gibbons SM, Gibson DL, Gonzalez A, Gorlick K, Guo J, Hillmann B, Holmes S, Holste H, Huttenhower C, Huttley GA, Janssen S, Jarmusch AK, Jiang L, Kaehler BD, Kang KB, Keefe CR, Keim P, Kelley ST, Knights D, Koester I, Kosciolek T, Kreps J, Langille MGI, Lee J, Ley R, Liu Y-X, Loftfield E, Lozupone C, Maher M, Marotz C, Martin BD, McDonald D, McIver LJ, Melnik AV, Metcalf JL, Morgan SC, Morton JT, Naimey AT, Navas-Molina JA, Nothias LF, Orchanian SB, Pearson T, Peoples SL, Petras D, Preuss ML, Pruesse E, Rasmussen LB, Rivers A, Robeson MS, II, Rosenthal P, Segata N, Shaffer M, Shiffer A, Sinha R, Song SJ, Spear JR, Swafford AD, Thompson LR, Torres PJ, Trinh P, Tripathi A, Turnbaugh PJ, Ul-Hasan S, van der Hooft JJJ, Vargas F, Vázquez-Baeza Y, Vogtmann E, von Hippel M, Walters W, Wan Y, Wang M, Warren J, Weber KC, Williamson CHD, Willis AD, Xu ZZ, Zaneveld JR, Zhang Y, Zhu Q, Knight R, Caporaso JG (2019) Reproducible, interactive, scalable and extensible microbiome data science using QIIME 2. Nature Biotechnology 37, 852-857.
| Crossref | Google Scholar | PubMed |
Boswell GP, Jacobs H, Davidson FA, Gadd GM, Ritz K (2003) Growth and function of fungal mycelia in heterogeneous environments. Bulletin of Mathematical Biology 65, 447-477.
| Crossref | Google Scholar | PubMed |
Callahan BJ, McMurdie PJ, Rosen MJ, Han AW, Johnson AJA, Holmes SP (2016) DADA2: high-resolution sample inference from Illumina amplicon data. Nature Methods 13, 581-583.
| Crossref | Google Scholar | PubMed |
Celestina C, Midwood J, Sherriff S, Trengove S, Hunt J, Tang C, Sale P, Franks A (2018) Crop yield responses to surface and subsoil applications of poultry litter and inorganic fertiliser in south-eastern Australia. Crop & Pasture Science 69, 303-316.
| Crossref | Google Scholar |
Celestina C, Sale PWG, Tang C, Franks AE (2019a) Organic and inorganic amendments did not affect microbial community composition in the bulk soil differently but did change the relative abundance of selected taxa. European Journal of Soil Science 70, 796-806.
| Crossref | Google Scholar |
Celestina C, Wood JL, Manson JB, Wang X, Sale PWG, Tang C, Franks AE (2019b) Microbial communities in top- and subsoil of repacked soil columns respond differently to amendments but their diversity is negatively correlated with plant productivity. Scientific Reports 9, 8890.
| Crossref | Google Scholar | PubMed |
Celestina C, Sale PWG, Hunt JR, Tang C, Franks AE (2019c) A single application of fertiliser or manure to a cropping field has limited long-term effects on soil microbial communities. Soil Research 57, 228-238.
| Crossref | Google Scholar |
Celestina C, Hunt JR, Sale PWG, Franks AE (2019d) Attribution of crop yield responses to application of organic amendments: a critical review. Soil and Tillage Research 186, 135-145.
| Crossref | Google Scholar |
Clark GJ, Dodgshun N, Sale PWG, Tang C (2007) Changes in chemical and biological properties of a sodic clay subsoil with addition of organic amendments. Soil Biology and Biochemistry 39, 2806-2817.
| Crossref | Google Scholar |
Clocchiatti A, Hannula SE, van den Berg M, Korthals G, de Boer W (2020) The hidden potential of saprotrophic fungi in arable soil: patterns of short-term stimulation by organic amendments. Applied Soil Ecology 147, 103434.
| Crossref | Google Scholar |
Crowther TW, Boddy L, Hefin Jones T (2012) Functional and ecological consequences of saprotrophic fungus–grazer interactions. The ISME Journal 6, 1992-2001.
| Crossref | Google Scholar | PubMed |
Cruz-Paredes C, Bang-Andreasen T, Christensen S, Ekelund F, Frøslev TG, Jacobsen CS, Johansen JL, Mortensen LH, Rønn R, Vestergård M, Kjøller R (2021) Bacteria respond stronger than fungi across a steep wood ash-driven pH gradient. Frontiers in Forests and Global Change 4, 781844.
| Crossref | Google Scholar |
Ding J, Jiang X, Guan D, Zhao B, Ma M, Zhou B, Cao F, Yang X, Li L, Li J (2017) Influence of inorganic fertilizer and organic manure application on fungal communities in a long-term field experiment of Chinese Mollisols. Applied Soil Ecology 111, 114-122.
| Crossref | Google Scholar |
Fierer N, Bradford MA, Jackson RB (2007) Toward an ecological classification of soil bacteria. Ecology 88, 1354-1364.
| Crossref | Google Scholar | PubMed |
Gill JS, Sale PWG, Peries RR, Tang C (2009) Changes in soil physical properties and crop root growth in dense sodic subsoil following incorporation of organic amendments. Field Crops Research 114, 137-146.
| Crossref | Google Scholar |
Gravuer K, Eskelinen A, Winbourne JB, Harrison SP (2020) Vulnerability and resistance in the spatial heterogeneity of soil microbial communities under resource additions. Proceedings of the National Academy of Sciences 117, 7263-7270.
| Crossref | Google Scholar |
Heanes DL (1984) Determination of total organic-C in soils by an improved chromic acid digestion and spectrophotometric procedure. Communications in Soil Science and Plant Analysis 15, 1191-1213.
| Crossref | Google Scholar |
Heinze S, Raupp J, Joergensen RG (2010) Effects of fertilizer and spatial heterogeneity in soil pH on microbial biomass indices in a long-term field trial of organic agriculture. Plant and Soil 328, 203-215.
| Crossref | Google Scholar |
Hermans SM, Buckley HL, Case BS, Curran-Cournane F, Taylor M, Lear G (2020) Using soil bacterial communities to predict physico-chemical variables and soil quality. Microbiome 8, 79.
| Crossref | Google Scholar | PubMed |
Ihrmark K, Bödeker ITM, Cruz-Martinez K, Friberg H, Kubartova A, Schenck J, Strid Y, Stenlid J, Brandström-Durling M, Clemmensen KE, Lindahl BD (2012) New primers to amplify the fungal ITS2 region – evaluation by 454-sequencing of artificial and natural communities. FEMS Microbiology Ecology 82, 666-677.
| Crossref | Google Scholar | PubMed |
Jin J, Hart M, Armstrong R, Sale P, Tang C (2023) Physiological responses to subsoil manuring in crop species across high and medium rainfall regions. Field Crops Research 302, 109068.
| Crossref | Google Scholar |
Kabir Z (2005) Tillage or no-tillage: impact on mycorrhizae. Canadian Journal of Plant Science 85, 23-29.
| Crossref | Google Scholar |
Kõljalg U, Nilsson HR, Schigel D, Tedersoo L, Larsson K-H, May TW, Taylor AFS, Jeppesen TS, Frøslev TG, Lindahl BD, Põldmaa K, et al. (2020) The taxon hypothesis paradigm – on the unambiguous detection and communication of taxa. Microorganisms 8, 1910.
| Crossref | Google Scholar | PubMed |
Lear G, Dickie I, Banks J, Boyer S, Buckley HL, Buckley TR, Cruickshank R, Dopheide A, Handley KM, Hermans S, Kamke J, Lee CK, MacDiarmid R, Morales SE, Orlovich DA, Smissen R, Wood J, Holdaway R (2018) Methods for the extraction, storage, amplification and sequencing of DNA from environmental samples. New Zealand Journal of Ecology 42, 10.
| Crossref | Google Scholar |
Li G, Wang M, Ma C, Tao R, Hou F, Liu Y (2021) Effects of soil heterogeneity and species on plant interactions. Frontiers in Ecology and Evolution 9, 756344.
| Crossref | Google Scholar |
Liu Z, Rong Q, Zhou W, Liang G (2017) Effects of inorganic and organic amendment on soil chemical properties, enzyme activities, microbial community and soil quality in yellow clayey soil. PLoS ONE 12, e0172767.
| Crossref | Google Scholar | PubMed |
López-Mondéjar R, Brabcová V, Štursová M, Davidová A, Jansa J, Cajthaml T, Baldrian P (2018) Decomposer food web in a deciduous forest shows high share of generalist microorganisms and importance of microbial biomass recycling. The ISME Journal 12, 1768-1778.
| Crossref | Google Scholar | PubMed |
Love MI, Huber W, Anders S (2014) Moderated estimation of fold change and dispersion for RNA-seq data with DESeq2. Genome Biology 15, 550.
| Crossref | Google Scholar |
MacEwan RJ, Crawford DM, Newton PJ, Clune TS (2010) High clay contents, dense soils, and spatial variability are the principal subsoil constraints to cropping the higher rainfall land in south-eastern Australia. Australian Journal of Soil Research 48, 150-166.
| Crossref | Google Scholar |
Martin M (2011) Cutadapt removes adapter sequences from high-throughput sequencing reads. EMBnet.journal 17, 10-12.
| Crossref | Google Scholar |
McKnight DT, Huerlimann R, Bower DS, Schwarzkopf L, Alford RA, Zenger KR (2019) Methods for normalizing microbiome data: an ecological perspective. Methods in Ecology and Evolution 10, 389-400.
| Crossref | Google Scholar |
McMurdie PJ, Holmes S (2013) phyloseq: an R package for reproducible interactive analysis and graphics of microbiome census data. PLoS ONE 8, e61217.
| Crossref | Google Scholar |
Nichols KA, Halvorson JJ (2013) Roles of biology, chemistry, and physics in soil macroaggregate formation and stabilization. The Open Agriculture Journal 7, 107-117.
| Crossref | Google Scholar |
Nilsson RH, Larsson K-H, Taylor AFS, Bengtsson-Palme J, Jeppesen TS, Schigel D, Kennedy P, Picard K, Glöckner FO, Tedersoo L, Saar I, Kõljalg U, Abarenkov K (2019) The UNITE database for molecular identification of fungi: handling dark taxa and parallel taxonomic classifications. Nucleic Acids Research 47, D259-D264.
| Crossref | Google Scholar | PubMed |
Nitsche BM, Jørgensen TR, Akeroyd M, Meyer V, Ram AFJ (2012) The carbon starvation response of Aspergillus niger during submerged cultivation: insights from the transcriptome and secretome. BMC Genomics 13, 380.
| Crossref | Google Scholar | PubMed |
Nkebiwe PM, Neumann G, Müller T (2017) Densely rooted rhizosphere hotspots induced around subsurface NH4+-fertilizer depots: a home for soil PGPMs? Chemical and Biological Technologies in Agriculture 4, 29.
| Crossref | Google Scholar |
Põlme S, Abarenkov K, Henrik Nilsson R, Lindahl BD, Clemmensen KE, Kauserud H, Nguyen N, Kjøller R, Bates ST, Baldrian P, Frøslev TG, Adojaan K, Vizzini A, Suija A, Pfister D, Baral H-O, Järv H, Madrid H, Nordén J, Liu J-K, Pawlowska J, Põldmaa K, Pärtel K, Runnel K, Hansen K, Larsson K-H, Hyde KD, Sandoval-Denis M, Smith ME, Toome-Heller M, Wijayawardene NN, Menolli N, Jr., Reynolds NK, Drenkhan R, Maharachchikumbura SSN, Gibertoni TB, Læssøe T, Davis W, Tokarev Y, Corrales A, Soares AM, Agan A, Machado AR, Argüelles-Moyao A, Detheridge A, de Meiras-Ottoni A, Verbeken A, Dutta AK, Cui B-K, Pradeep CK, Marín C, Stanton D, Gohar D, Wanasinghe DN, Otsing E, Aslani F, Griffith GW, Lumbsch TH, Grossart H-P, Masigol H, Timling I, Hiiesalu I, Oja J, Kupagme JY, Geml J, Alvarez-Manjarrez J, Ilves K, Loit K, Adamson K, Nara K, Küngas K, Rojas-Jimenez K, Bitenieks K, Irinyi L, Nagy LG, Soonvald L, Zhou L-W, Wagner L, Aime MC, Öpik M, Mujica MI, Metsoja M, Ryberg M, Vasar M, Murata M, Nelsen MP, Cleary M, Samarakoon MC, Doilom M, Bahram M, Hagh-Doust N, Dulya O, Johnston P, Kohout P, Chen Q, Tian Q, Nandi R, Amiri R, Perera RH, dos Santos Chikowski R, Mendes-Alvarenga RL, Garibay-Orijel R, Gielen R, Phookamsak R, Jayawardena RS, Rahimlou S, Karunarathna SC, Tibpromma S, Brown SP, Sepp S-K, Mundra S, Luo Z-H, Bose T, Vahter T, Netherway T, Yang T, May T, Varga T, Li W, Coimbra VRM, de Oliveira VRT, de Lima VX, Mikryukov VS, Lu Y, Matsuda Y, Miyamoto Y, Kõljalg U, Tedersoo L (2020) FungalTraits: a user-friendly traits database of fungi and fungus-like stramenopiles. Fungal Diversity 105, 1-16.
| Crossref | Google Scholar |
Quast C, Pruesse E, Yilmaz P, Gerken J, Schweer T, Yarza P, Peplies J, Glöckner FO (2012) The SILVA ribosomal RNA gene database project: improved data processing and web-based tools. Nucleic Acids Research 41, D590-D596.
| Crossref | Google Scholar | PubMed |
Raza T, Qadir MF, Khan KS, Eash NS, Yousuf M, Chatterjee S, Manzoor R, Rehman Su, Oetting JN (2023) Unraveling the potential of microbes in decomposition of organic matter and release of carbon in the ecosystem. Journal of Environmental Management 344, 118529.
| Crossref | Google Scholar | PubMed |
Rousk J, Bååth E, Brookes PC, Lauber CL, Lozupone C, Caporaso JG, Knight R, Fierer N (2010) Soil bacterial and fungal communities across a pH gradient in an arable soil. The ISME Journal 4, 1340-1351.
| Crossref | Google Scholar | PubMed |
Sale PW, Gill JS, Peries RR, Tang C (2019) Crop responses to subsoil manuring. I. Results in south-western Victoria from 2009 to 2012. Crop & Pasture Science 70, 44-54.
| Crossref | Google Scholar |
Sale P, Tavakkoli E, Armstrong R, Wilhelm N, Tang C, Desbiolles J, Malcolm B, O’Leary G, Dean G, Davenport D, Henty S, Hart M (2021) Ameliorating dense clay subsoils to increase the yield of rain-fed crops. Advances in Agronomy 165, 249-300.
| Crossref | Google Scholar |
Tanunchai B, Ji L, Schroeter SA, Wahdan SFM, Hossen S, Delelegn Y, Buscot F, Lehnert A-S, Alves EG, Hilke I, Gleixner G, Schulze E-D, Noll M, Purahong W (2023) FungalTraits vs. FUNGuild: comparison of ecological functional assignments of leaf- and needle-associated fungi across 12 temperate tree species. Microbial Ecology 85, 411-428.
| Crossref | Google Scholar | PubMed |
Thiele-Bruhn S, Bloem J, de Vries FT, Kalbitz K, Wagg C (2012) Linking soil biodiversity and agricultural soil management. Current Opinion in Environmental Sustainability 4, 523-528.
| Crossref | Google Scholar |
Tripathi BM, Song W, Slik JWF, Sukri RS, Jaafar S, Dong K, Adams JM (2016) Distinctive tropical forest variants have unique soil microbial communities, but not always low microbial diversity. Frontiers in Microbiology 7, 376.
| Crossref | Google Scholar |
Uddin S, Oates A, Aslam N, Armstrong D, Pitt W, Hildebrand S, Dehaan R, Sandral G, Fang Y, McDonald GK, Schilling R, Armstrong R, Tavakkoli E (2020) Soil amelioration–magnitude of crop productivity improvements on hostile subsoils? In ‘Grains Research and Development Corporation Update 38’. (Grains Research & Develoment Corporation)
Uddin S, Williams SW, Aslam N, Fang Y, Parvin S, Rust J, Van Zwieten L, Armstrong R, Tavakkoli E (2022) Ameliorating alkaline dispersive subsoils with organic amendments: are productivity responses due to nutrition or improved soil structure? Plant and Soil 480, 227-244.
| Crossref | Google Scholar |
Verbruggen E, Toby Kiers E (2010) Evolutionary ecology of mycorrhizal functional diversity in agricultural systems. Evolutionary Applications 3, 547-560.
| Crossref | Google Scholar | PubMed |
Vido JJ, Wang X, Sale PWG, Celestina C, Shindler AE, Hayden HL, Tang C, Wood JL, Franks AE (2024) Bacterial community shifts occur primarily through rhizosphere expansion in response to subsoil amendments. Environmental Microbiology 26, e16587.
| Crossref | Google Scholar | PubMed |
Wang X, Sale P, Hayden H, Tang C, Clark G, Armstrong R (2020) Plant roots and deep-banded nutrient-rich amendments influence aggregation and dispersion in a dispersive clay subsoil. Soil Biology and Biochemistry 141, 107664.
| Crossref | Google Scholar |
Wang T, Cao X, Chen M, Lou Y, Wang H, Yang Q, Pan H, Zhuge Y (2022) Effects of soil acidification on bacterial and fungal communities in the Jiaodong Peninsula, Northern China. Agronomy 12, 927.
| Crossref | Google Scholar |
Yang P, van Elsas JD (2018) Mechanisms and ecological implications of the movement of bacteria in soil. Applied Soil Ecology 129, 112-120.
| Crossref | Google Scholar |
Ye G, Banerjee S, He J-Z, Fan J, Wang Z, Wei X, Hu H-W, Zheng Y, Duan C, Wan S, Chen J, Lin Y (2021) Manure application increases microbiome complexity in soil aggregate fractions: results of an 18-year field experiment. Agriculture, Ecosystems & Environment 307, 107249.
| Crossref | Google Scholar |
Yilmaz P, Parfrey LW, Yarza P, Gerken J, Pruesse E, Quast C, Schweer T, Peplies J, Ludwig W, Glöckner FO (2014) The SILVA and “all-species living tree project (LTP)” taxonomic frameworks. Nucleic Acids Research 42, D643-D648.
| Crossref | Google Scholar | PubMed |
Young IM, Ritz K (2000) Tillage, habitat space and function of soil microbes. Soil and Tillage Research 53, 201-213.
| Crossref | Google Scholar |
Yu K, van den Hoogen J, Wang Z, Averill C, Routh D, Smith GR, Drenovsky RE, Scow KM, Mo F, Waldrop MP, Yang Y, et al. (2022) The biogeography of relative abundance of soil fungi versus bacteria in surface topsoil. Earth System Science Data 14, 4339-4350.
| Crossref | Google Scholar |