Taxonomy of the Nicotiana megalosiphon species complex (Solanaceae; Nicotiana section Suaveolentes): analyses of RADseq data identifies a new cryptic species
Mark W. Chase






A
B
C
D
E
F
G
Abstract
The Nicotiana megalosiphon Van Heurck & Müll.Arg. species complex has been shown to be composed of several morphologically cryptic species similar to N. simulans N.T.Burb. Using phylogenetic and population genetic approaches (maximum likelihood, co-ancestry, admixture proportions, Bayesian species delimitation and coalescent methods), we demonstrate that there is an additional undescribed species in this complex. The species limits of N. latifolia M.W.Chase & Christenh., N. latzii M.W.Chase, R.W.Jobson & Christenh., N. megalosiphon, N. sessilifolia (P.Horton) M.W.Chase & Christenh. and N. simulans, previously circumscribed based solely on a phylogenetic approach, are confirmed in the new analyses and a new species, N. palssonae M.W.Chase & Christenh., is described. A map of species distributions and a key to the species of the N. megalosiphon species complex are provided.
Keywords: admixture analysis, Bayesian species delimitation, coalescent methods, cryptic species, flora of eastern Australia, Nicotiana simulans, Nicotianoideae, wild tobacco.
Introduction
Nicotiana section Suaveolentes Goodsp. comprises all native Australian species of the genus that occur throughout the continent except for Tasmania. Cauz-Santos et al. (2024) demonstrated that after arrival in Australia c. 6 million years ago (Ma), the distribution was concentrated in mesic habitats in the northern monsoon region and along the east coast. However, the species subsequently moved into the Pilbara where the number of clades increased dramatically and adaptations to aridity developed, after which the species dispersed widely across the continent in several episodes over the past 5 Ma. Our research on this group was initiated due to the descending chromosome series and variable genome sizes, both occurring during the diploidisation phase while these allotetraploid species were adapting to a variety of habitats throughout the Eremaean Zone (Chase et al. 2023b). While studying these aspects of N. sect. Suaveolentes, we realised that in previous approaches to the taxonomy of this section (Horton 1981), the number of species had been underestimated at 16. Symon (1984, 1998), Clarkson and Symon (1991), Symon and Kenneally (1994) and Symon and Lepschi (2007) added 5 species to the section, bringing the total to 21 native species recorded for Australia by 2007. An additional 30 species have been described (reviewed in Chase et al. 2022), through elevation of subspecies and reinstatement of names in synonymy but mostly through the description of previously unrecognised species (reviewed in Chase et al. 2023a). New species occur throughout the range of the section, but Western Australia has yielded the highest number of new species.
Phylogenetic relationships for N. section Suaveolentes are described in Chase et al. (2022). The focus of this paper is a group of species related to N. megalosiphon Van Heurck & Müll.Arg. and N. simulans N.T.Burb. that we refer to as the N. megalosiphon species complex. These species form part of a larger clade in which the chromosome number is mostly n = 20 (Chase et al. 2023b) and the vestiture comprises a mixture of long, tiny, gland-bearing multicellular and short, gland-tipped hairs, with a few intermediate-length hairs, especially on the lower stems and petioles (Fig. 1). Different types of vestiture are characteristic of each of the major clades in the section. The long hairs on the upper stems may break off, leaving stout bases that cause the stems to feel rough to the touch. The N. megalosiphon species complex does not occur in Western Australia but the sister clade, the N. stenocarpa Wheeler species complex (also n = 20 with the same vestiture type (Chase and Christenhusz 2018), is largely concentrated there. As far as we can determine, the species we studied are the only species of the N. megalosiphon species complex.
Vestiture of species in the Nicotiana megalosiphon–simulans clade, comprising mixed long to short hairs with small apical glands.
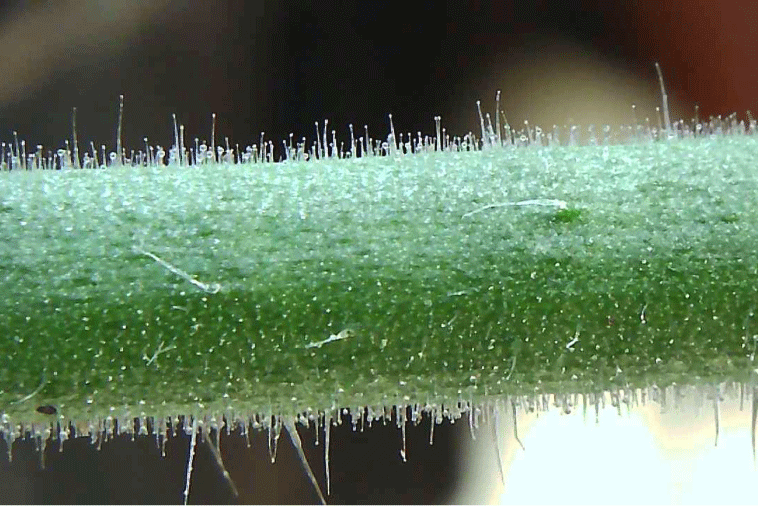
We evaluate species limits of the N. megalosiphon species complex to determine whether there is an additional undescribed species. During examination of specimens in several Australian herbaria (BRI, CANB and NSW), some specimens appeared to be N. simulans but these differed slightly morphologically and more importantly, the ecological characterisitcs deviated from those of N. simulans. Our phylogenetic analyses initially used a RAxML approach to determine interspecific relationships of the species, but as described in Cauz‐Santos et al. (2022), we constructed a coancestry heatmap and individual admixture proportions with NGSadmix to evaluate population structure and detect admixture. We also used a Bayesian species delimitation approach to estimate the most appropriate number of species for recognition. We built a multilocus, coalescent species tree using the optimal species model defined in the delimitation analyses to estimate the ages of species divergences in the N. megalosiphon species complex.
Materials and methods
Plant material
We sampled 36 individuals, 33 of which were from the N. megalosiphon species complex (4 from viable seeds recovered from herbarium specimens up to 27 years old) and 3 of N. walpa M.W.Chase, Dodsworth & Christenh. (Chase et al. 2021), the latter being an outgroup in the phylogenetic analysis (Supplementary Table S1). This sampling reflects the distribution of this species complex (Fig. 2) in New South Wales, Northern Territory, Queensland and South Australia (Fig. 2).
Map of the species in the N. megalosiphon species complex. Blue, N. sessilifolia; pink, N. simulans; red, N. latifolia; turquoise, N. latzii; green, N. megalosiphon; orange, N. palssonae. Created by M. Christenhusz from data downloaded from the Australian Virtual Herbarium website and our specimens that have not yet been databased in Australia.
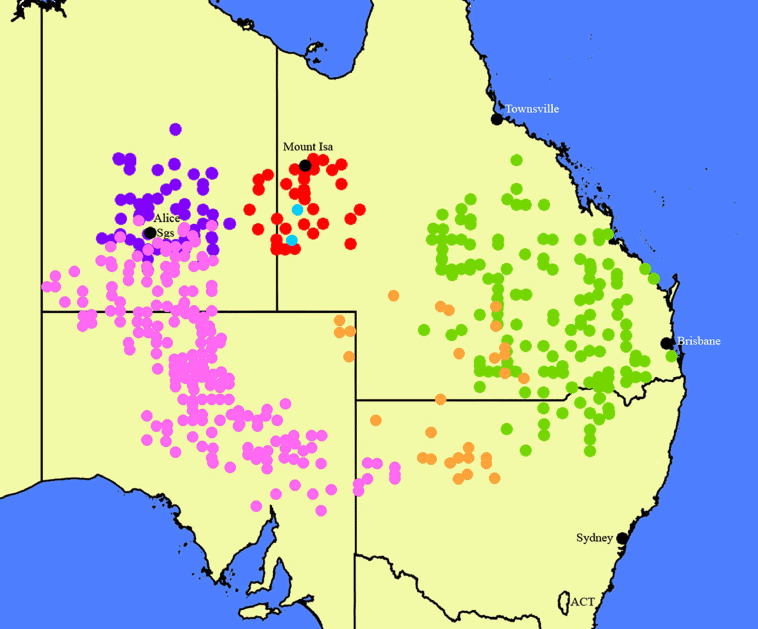
DNA extraction, library preparation and sequencing
Total genomic DNA was isolated from ~20 mg of silica-dried or fresh leaf tissue, pre-treated for 20 min in a buffer of cold sorbitol (100 mM tris-HCl, 5 mM EDTA, 0.35 M sorbitol, pH 8.0) under a cetyltrimethylammonium bromide (CTAB) procedure and purified according to the manufacturer’s instructions for the NucleoSpin gDNA clean-up Kit (Machery–Nagel, Düren, Germany).
Single-digestion RADseq libraries were prepared according to Paun et al. (2016) including digestion with PstI and use of index barcodes distinct by at least three nucleotide positions. Libraries were sequenced as pair-end reads of 125 bp at the VBCF NGS Unit (see www.vbcf.ac.at/ngs).
SNP calling from RADseq data
The raw reads were processed in the BamIndexDecoder (ver. 1.03, included in Picard Illumina2Bam package, see http://gq1.github.io/illumina2bam/) and demultiplexed in sublibraries according to index barcodes. We subsequently used process_radtags in Stacks (ver. 1.47, see https://catchenlab.life.illinois.edu/stacks/; Catchen et al. 2013) and inline barcode information to demultiplex the individual samples. This last process was performed in conjunction with quality filtering to remove low quality reads, allowing a maximum of one mismatch in the barcodes and cut-site sequences (Supplementary Table S2).
The mappings were performed in BWA MEM (ver. 0.7.17, see https://github.com/lh3/bwa; Li and Durbin 2009), using the genome of N. benthamiana (ver. 1.0.1, see https://solgenomics.net/organism/Nicotiana_benthamiana/genome; Bombarely et al. 2012) as a reference. For the mapping, we used the option −M to flag shorter split hits as secondary and the resulting aligned SAM file was sorted by reference coordinates. Read groups were added using the Picard Toolkit (see http://broadinstitute.github.io/picard/). Mapping quality was improved with a realignment around indels in the Genome Analysis Toolkit (GATK, ver. 3.8, see https://gatk.broadinstitute.org/hc/en-us; McKenna et al. 2010), thinning data per interval to a maximum of 100,000 reads.
Variant calling was performed in GATK, starting with generation of a gVCF file for each sample using the GNVCF mode of HaplotypeCaller, followed by the processing of all samples using the GenotypeGVCFs module. We used VCFtools (ver. 0.1.15, see https://vcftools.github.io/; Danecek et al. 2011) to retain only variants present in at least 50% of the individuals (Supplementary Table S1) and performed filtering in the VariantFiltration module of GATK using the following criteria: (1) depth of coverage (DP) < 500; (2) variant confidence (QUAL) < 30.00; (3) variant confidence divided by the unfiltered depth (QD) < 2; (4) Phred-scaled P-value for the Fisher’s exact test to detect strand bias (FS) > 60; (5) a root mean square of mapping quality across all samples (MQ) < 40; (6) u-based z-approximation from the rank sum test for mapping qualities (ReadPosRankSum) < −8.0; and (7) u-based z-approximation from the rank sum test for the distance from the end of the reads with the alternate allele (MQRankSum) < −12.5.
After first filtering in GATK, 1,656,211 variable sites were retained. Subsequently, an additional filtering was performed in VCFtools to retain only SNPs with a minor allele frequency of at least 0.055 (i.e. present in at least four haplotypes), an average depth greater than 20 and 20% maximum missing data. To avoid further use of any pooled paralogs, we filtered positions with maximum observed heterozygosity greater than 0.65 using the populations pipeline from Stacks. The final filtered file included 103,282 SNPs among the Nicotiana accessions included that was used for RAxML (maximum likelihood) analysis.
Phylogenomic analysis
To perform the phylogenetic analysis, we converted the final filtered VCF file to PHYLIP format using PGDspider (ver. 2.1.1.0, see https://software.bioinformatics.unibe.ch/pgdspider/; Lischer and Excoffier 2012) and used the ascbias.py script (see https://github.com/btmartin721/raxml:ascbias) to remove invariant sites. A maximum likelihood tree was produced with RAxML (ver. 8.2.12, see https://github.com/stamatak/standard-RAxML; Stamatakis 2014) using the Lewis (2001) correction for ascertainment bias. We identified the best ML tree for 36 accessions using the general time reversible model of nucleotide substitution and the CAT approximation of rate heterogeneity (GTRCAT), and performed 1000 rapid bootstrap replicates. The tree was finally visualised and annotated in R (ver. 4.4.2, R Foundation for Statistical Computing, Vienna, Austria, see https://www.r-project.org/) with ape (ver. 5.3, see http://ape-package.ird.fr/; Paradis and Schliep 2019), biostrings (ver. 2.72.1, H. Pagès, P. Aboyoun, R. Gentleman and S. DebRoy, see https://bioconductor.org/packages/Biostrings), ggplot2 (ver. 2.3.5.1, H. Wickham, W. Chang and M. H. Wickham, see https://CRAN.R-project.org/package=ggplot2; Wickham 2016), ggtree (ver. 3.20, see https://doi.org/doi:10.18129/B9.bioc.ggtree; Yu et al. 2017) and treeio (ver. 3.20, see https://doi.org/10.18129/B9.bioc.treeio; Wang et al. 2020).
Admixture and genetic clustering analyses
To identify admixture proportions and population structure for the 33 accessions comprising the N. megalosiphon species complex, we obtained genotype likelihoods using the indel-realigned.bam files and genotype-free method implemented in ANGSD (ver. 0.930, see https://github.com/ANGSD/angsd; Korneliussen et al. 2014). The analysis only kept sites with data for at least 50% of individuals, a minimum mapping quality of 20 and the GATK-based genotype likelihood model used to estimate major and minor allele frequencies. Filtering resulted in 1,215,636 high-confidence (P < 1 × 10−6) variable positions with a shared minor allele by three or more individuals.
Subsequently, we selected unlinked sites from the genotype likelihoods obtained in ANGSD (distance of 10,000 bp between variants, resulting in 39,293 positions) and estimated individual admixture proportions using NGSadmix (see https://www.popgen.dk/software/index.php/NgsAdmix; Skotte et al. 2013). We analysed K = 1–10 as the number of clusters, initialising the EM algorithm with 10 seeds and considering only variants with minor allele frequency higher than 0.055.
The clusters produced by use of the K values were evaluated with the Evanno method (Evanno et al. 2005) available in CLUMPAK (see http://clumpak.tau.ac.il/bestK.html; Kopelman et al. 2015) and the final plot was produced in R.
Species delimitation and species tree
Bayesian species delimitation was performed with a matrix of 19 individuals (16 of the N. megalosiphon complex and 3 of N. walpa) with at least 2 individuals per group identified in the models. This method cannot manage the 36 accessions used in the RAxML analysis, therefore we eliminated some individuals. We also explored the NGSadmix results and selected individuals without evidence of admixture. We used VCFtools to filter the VCF file, selecting unlinked biallelic SNPs (>50,000-bp distance on the same contig) and removing all missing data.
We evaluated six models for the N. megalosiphon complex using N. walpa as outgroup. The first model treated N. megalosiphon in the broadest possible sense and subsequently split the N. megalosiphon complex in up to seven groups. Bayesian species delimitation was performed in SNAPP (ver. 1.2.5, see https://www.beast2.org/snapp/; Bryant et al. 2012) and marginal likelihood values were obtained using 24 initialisation steps. The analysis was conducted with 1 million chain-lengths for each mode, storing a tree every 1000 generations and excluding the first 10% as burn in. The optimal model of species assignment was obtained by calculating the Bayes factor based on the marginal likelihood values.
We used the optimal model obtained in the species delimitation to construct a coalescent species tree in SNAPP (ver. 1.2.5). The analysis was based on the same dataset of unlinked SNPs (5475), with 10 million as the chain length using the same procedures for keeping trees. We used the ESS values from the log-file to check convergence of the run in Tracer (ver. 1.6, see https://github.com/beast-dev/tracer/releases/tag/v1.6; Rambaut et al. 2018). We calculated posterior probabilities using TreeAnnotator (ver. 1.8.3, see https://www.beast2.org/treeannotator/; Drummond et al. 2012) and visualised the SNAPP trees as a cloudogram with DensiTree 2 (ver. 2.3.1.0, see https://github.com/rbouckaert/DensiTree; Bouckaert and Heled 2018) that produced divergence times and re-scaled the trees. Divergence estimates were based on the total length of investigated sites in the included loci and the total number of polymorphic sites across the length using 5 × 10−9 as the rate of substitution per site per generation (Schiavinato et al. 2020) and 1 year as generation time (these species are all ephemerals or short-lived perennials that only rarely survive the summer).
Results
Phylogenomic analysis (RAxML)
Except for the position of N. simulans, the RAxML analysis produced a generally well supported tree (Fig. 3) with well differentiated relationships of all species concepts. One accession of N. palssonae M.W.Chase & Christenh. (18016) is only weakly supported as a member of the cluster assigned to this species.
Admixture and genetic clustering analyses
We initially used these analyses to select accessions that did not exhibit admixture, which would interfere with subsequent analyses (i.e. the coalescent species tree in SNAPP). The Structure plots ranged from K = 2–9 (Fig. 4) with K = 7 optimal and some accessions did not consistently exhibit the genetic identities that we assigned to species concepts based on RAxML results (Fig. 3). Several accessions of N. palssonae and N. megalosiphon exhibited admixture, some even outside the zone of geographical overlap and some geographical population structuring was present in several species, including N. palssonae and N. megalosiphon. The two accessions from northern New South Wales (18161, 18175) assigned to N. megalosiphon in the RAxML tree in some cases could be assigned to the N. latzii M.W.Chase, R.W.Jobson & Christenh. cluster. Two accessions of N. palssonae from the eastern part of the range in New South Wales appeared as unique genotypes in the K = 7 plot. An accession assigned to N. palssonae in the RAxML tree (but weakly supported) (18016) exhibited admixture in some of the structure plots with N. megalosiphon.
The 16 accessions of the N. megalosiphon species complex used in the Bayesian species delimitation were evaluated for the optimal number of species to be recognised (Fig. 5a) that was model 5 (with the best Bayes score). This analysis split the N. megalosiphon species complex into six clusters (species) and N. walpa, the outgroup. The next best result was model 6 that split the accessions of N. megalosiphon from New South Wales and Queensland, a result we will discuss below.
Model evaluation for recognition of genetic entities in the N. megalosiphon species complex using Bayesian species delimitation (a). Bayesian species tree for the N. megalosiphon species complex with age estimates (b).
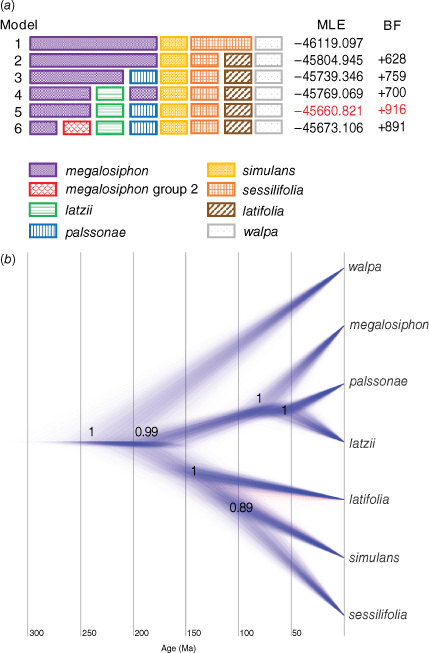
The coalescent species tree (Fig. 5b) generally agreed with the RAxML tree, except for the position of N. simulans that was weakly supported as sister to N. sessilifolia (P.Horton) M.W.Chase & Christenh. However this pair was well supported as a member of a clade with N. latifolia. Nicotiana palssonae was sister to N. latzii instead of N. megalosiphon as in the RAxML tree. When we calculated the divergence estimates, the N. megalosiphon species complex began to diverge c. 200,000 years ago with N. palssonae and N. latzii splitting only 60,000 years ago.
Discussion
The N. megalosiphon species complex began to radiate in north-eastern Australia only c. 200,000 years ago, with the first split in the Bayesian species tree (Fig. 5b) representing ancestors of the N. latifolia–N. sessilifolia–N. simulans and N. latzii–N. palssonae–N. megalosiphon clades. Biogeographically, this represents a split between the western and eastern parts of the overall range. However, if the RAxML tree is correct, the clade originated in the western part of the range and spread eastward from there. The relationship of N. simulans to the other species is different in the Bayesian species and RAxML trees, making a more detailed scenario for how divergences progressed ambiguous.
On morphological grounds, we are content with the assignments of accessions in the RAxML tree, but we note problematic accessions from a genetic standpoint in the Structure results (Fig. 3). One of these is the Prendergast 354 specimen (secondary voucher Chase & Christenhusz 18016) that falls with N. palssonae in the RAxML tree (Fig. 2) but with low support and is distinct in some of the Structure plots. Morphologically, this accession is most like N. palssonae and we include this in the latter but note that the accession is problematic. We acknowledge that our sampling for the genetic studies only includes one accession from the area in which N. palssonae and N. megalosiphon overlap, and this individual exhibits admixture in some cases. However, several other accessions of N. palssonae and N. megalosiphon exhibit admixture, including some outside the area of geographical overlap. Despite this evidence for some degree of gene flow, the two species remain morphologically distinct. Some species are known to have porous genomes (Lexer et al. 2009) but nonetheless maintain species boundaries and distinct morphological characteristics, as may be the case here. We would need multiple samples per population and more populations, especially from the area of geographical overlap, to determine the situation more conclusively. Despite these deficiencies, we rely upon the morphological distinctiveness of these two species to justify description of N. palssonae.
Our original attention to accessions of what we name N. palssonae was drawn by herbarium collections from outside the southern Northern Territory/South Australia that had been identified as N. simulans (not N. megalosiphon, with which these accessions were rarely identified). When we use the term ‘cryptic species’ we refer to the similarity of N. palssonae to N. simulans, not N. megalosiphon, the most morphologically divergent and easily diagnosed species in this species group. The specimens of both N. latzii and N. palssonae generally agreed with the concept of N. simulans (for which the type is from South Australia) but these differed sufficiently to elicit our interest. The first living material of the newly recognised species, N. palssonae, were plants that Ruth Palsson collected when searching for N. velutina Wheeler near Broken Hill during the COVID-19 years. Palsson did not find N. velutina but did yield material of a N. simulans-like accession that had ecological characteristics that differed from those of accessions from South Australia. Nicotiana simulans generally occurs in the open from the gibber plains in South Australia to the southernmost Northern Territory, whereas the new species occurs in sheltered sites under mulga (Acacia aneura F.Muell. ex Benth. species complex) and various eucalypt species from north-western New South Wales and north-easternmost South Australia to south-western Queensland. In years with plentiful rainfall, the species has occurred commonly and been collected frequently. The other species in this complex are typically associated with sheltered sites, although in years with the highest rainfall, these can also be found growing in more open habitats. The Western Australian and Northern Territory counterpart is the N. stenocarpa complex (including the species used here as outgroup, N. walpa), the species of which also mostly occur in shade (usually under mulga). Most authors have referred these specimens to N. simulans. Nicotiana palssonae and N. simulans also differ morphologically (leaf shape and generally long petioles, often with a slight wing in the latterand abruptly attenuate bases in N. palssonae). Nicotiana latzii, N. palssonae and N. simulans were readily distinguishable upon cultivation together but herbarium material of these species can be challenging to distinguish.
Morphologically, N. sessilifolia (Fig. 6) is easily distinguished from N. megalosiphon (Fig. 7) based on the shorter floral tubes and broadly winged petioles with auriculate bases. Nicotiana latifolia (Fig. 8) can be considered to have such broadly winged petioles as to appear sessile, distinguishing this species from both N. megalosiphon and N. sessilifolia. In addition, the latter two species have symmetrical perianth limbs with the four longer stamens of the same length, as opposed to the oblique floral tubes and didynamous stamens in N. palssonae (Fig. 9) and N. simulans (Fig. 10). Nicotiana latzii (Fig. 11) has symmetrical limbs and the four longer stamens of the same length, and differs from N. palssonae and N. simulans in these floral characteristics and the short, broadly winged petioles. Oblique floral tubes and didynamous stamens appear to revert or appear independently in many clades in N. sect. Suaveolentes but this condition makes a useful species character in many cases even though difficult to discern in herbarium material. The other often conspicuous floral trait of N. sect. Suaveolentes is the anther cups (in which the stamens are positioned) that are absent in all these species and their sister clade (including N. walpa and N. stenocarpa).
Illustration of Nicotiana sessilifolia based on living plants cultivated at the Royal Botanic Gardens, Kew, grown from seeds from Chase & Christenhusz 16046 (NT), collected at the Old Telegraph Station, Alice Springs, Northern Territory. Illustrated by Deborah Lambkin. (a) Floral limb, front view. (b) Corolla split open to reveal positions of stamens. (c) Flower, side view. (d) Carpel, style and stigma. (e) Pubescence on leaf surface near midvein. (f) Pubescence on leaf margin. (g) Pubescence on upper stem and pedicel. (h) Pubescence on lower stem. (i) Upper stem leaf. (j) Lower stem leaf. (k) Mature capsule. (l) Dehisced capsule with calyx removed. (m) Habit. Scale bars: a–d, k, l, 2.0 cm; e–h, 7.0 mm; i, j, 4.0 cm; plant in (m) is 110 cm tall. Reproduced from Chase et al. (2023a in Australian Systematic Botany) with permission.
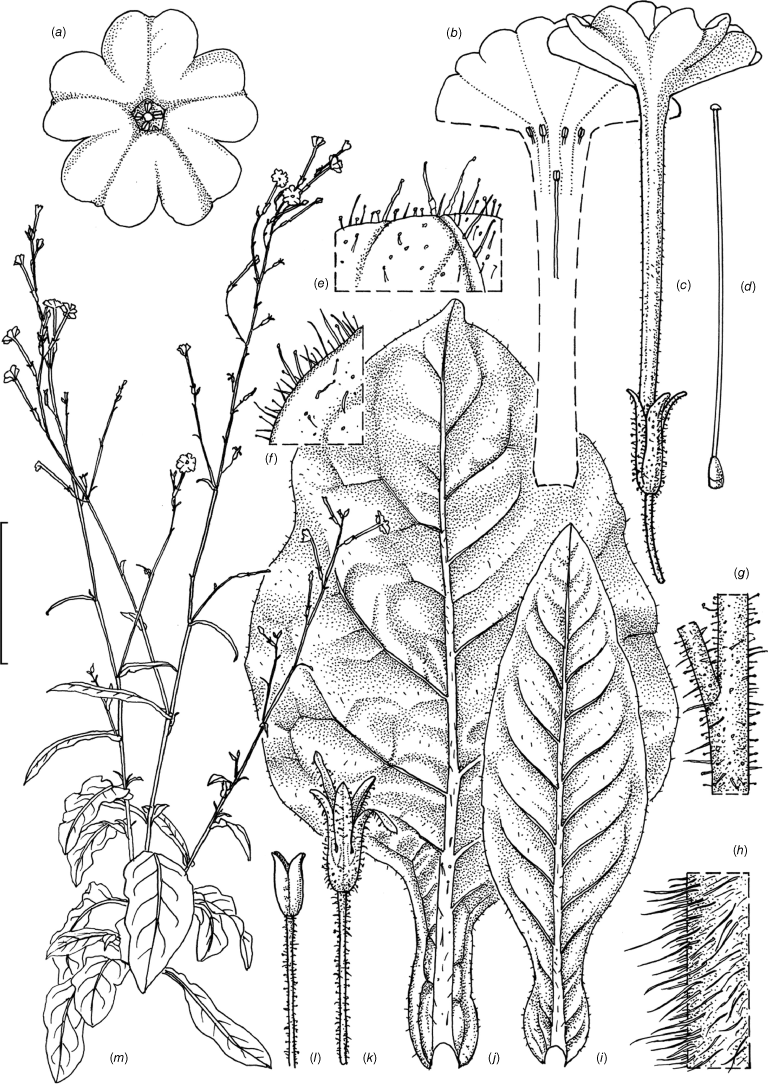
Illustration of Nicotiana megalosiphon based on living plants cultivated at the Royal Botanic Gardens, Kew, grown from seeds from with Chase & Christenhusz 17009, collected atTelleraga-Millie Road, bridge over Mehi River, New South Wales. Illustrated by Deborah Lambkin. (a) Carpel, style and stigma. (b) Corolla, opened out. (c) Flower, lateral view. (d) Corolla, dorsal view. (e) Capsule, with calyx. (f) Dehisced capsule, calyx removed. (g) Stem leaf. (h) Stem pubescence. (i) Calyx hairs. (j) Leaf margin pubescence. (k) Habit. Scale bars: a–f, 2.0 cm; g, 3.0 cm; h–j, 1.0 cm; plant in (k) is 90 cm tall. Reproduced from Chase and Christenhusz (2021 in Curtis’s Botanical Magazine) with permission.
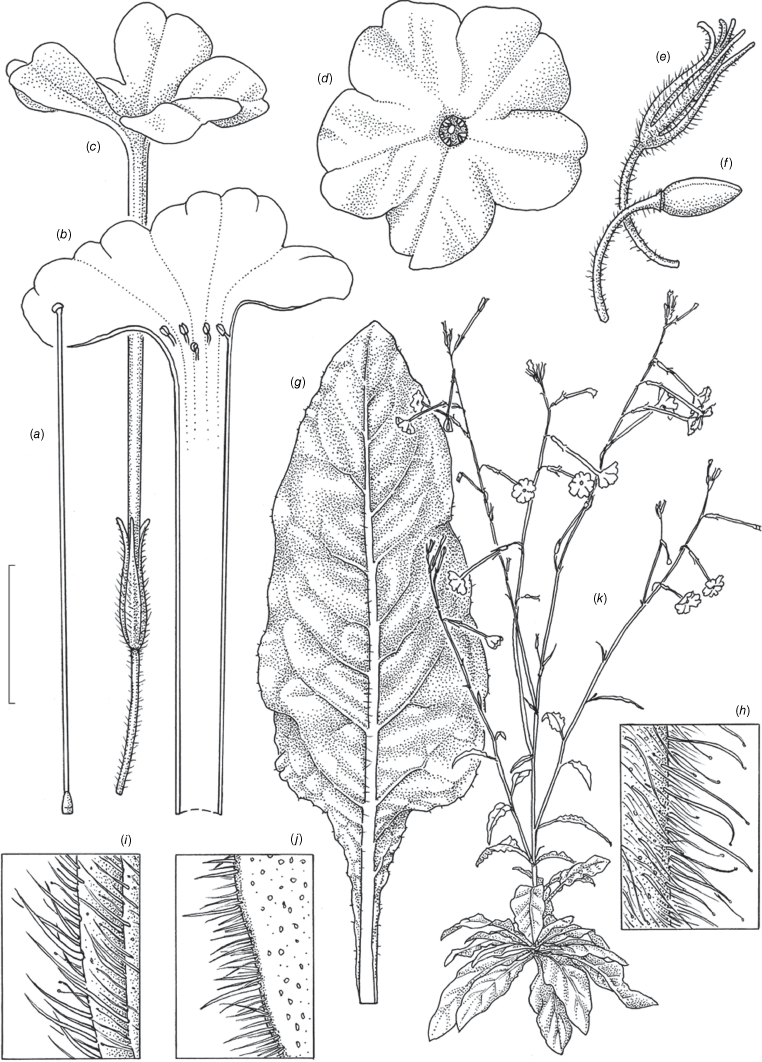
Illustration of Nicotiana latifolia based on living plants cultivated at the Royal Botanic Gardens, Kew, grown from seeds from Chase & Christenhusz 18177 (BRI), Cloncurry, on the banks of the Cloncurry River, Queenland. Illustrated by Deborah Lambkin. (a) Floral limb, dorsal view. (b) Flower, lateral view. (c) Corolla split open to reveal positions of stamens. (d) Carpel, style and stigma. (e) Mature capsule. (f) Dehisced capsule with calyx removed. (g) Pubescence on leaf margin. (h) Pubescence on leaf lamina. (i) Pubescence on lower stem. (j) Pubescence on upper stem. (k) Stem leaf. (l) Habit. Scale bars: a–f, 2.0 cm; g–j, 1.0 cm; k, 3.0 cm; plant in (l) is 90 cm tall. Reproduced from Chase et al. (2023a in Australian Systematic Botany) with permission.
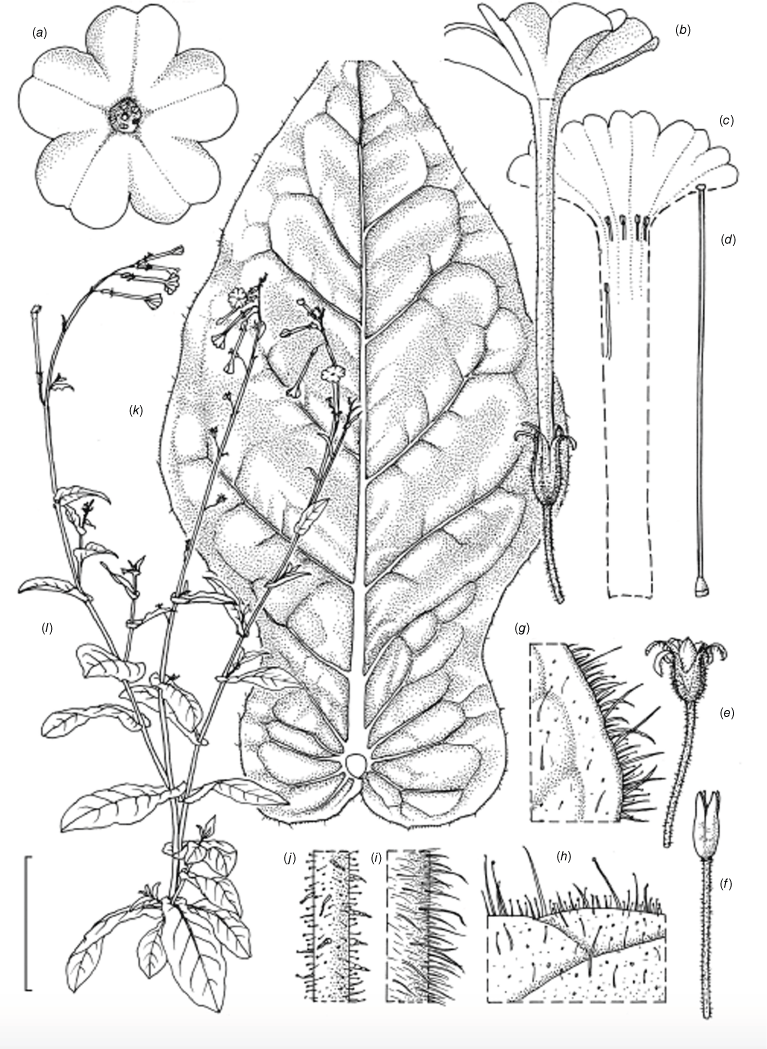
Illustration of Nicotiana palssonae based on living plants cultivated at the Royal Botanic Gardens, Kew, grown from seeds from Chase & Christenhusz 20014 (NSW), Living Desert State Park, Broken Hill, New South Wales. Illustrated by Deborah Lambkin. (a) Floral limb, dorsal view. (b) Corolla split open to reveal positions of stamens. (c) Flower, lateral view. (d) Carpel, style and stigma. (e) Lower leaf on stem. (f) Upper leaf on stem. (g) Mature fruit. (h) Dehisced capsule with calyx removed. (i) Pubescence on upper stem. (j) Pubescence on leaf margin. (k) Pubescence on lower stem. (l) Pubescence on calyx. (m) Habit. Scale bars: a–f, 2.0 cm; g–j, 1.0 cm; k, 3.0 cm; plant in (m) is 130 cm tall.
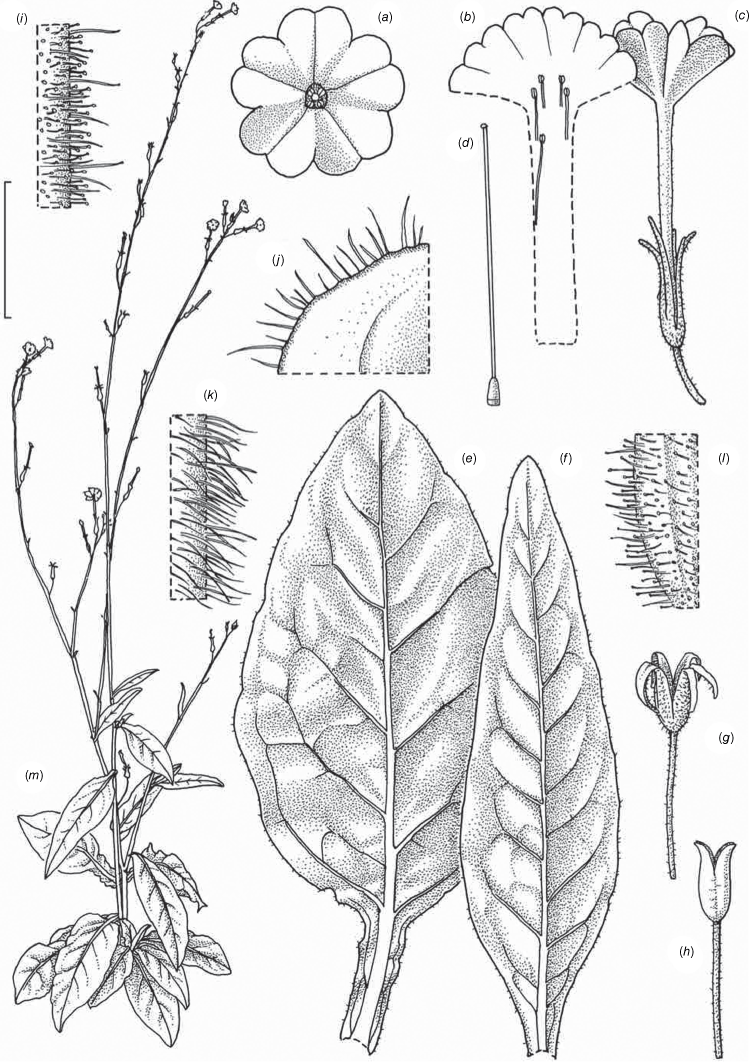
Illustration of Nicotiana simulans based on living plants cultivated at the Royal Botanic Gardens, Kew, grown from seeds from with a secondary voucher of Chase & Christenhusz 24005 (k) and original specimen, Conran 437 (AD), Paralana Springs, South Australia. (a) Plant 116 cm tall. (b, c) Two stem leaves to illustrate leaf variation of leaves on a single plant. (d). Floral limb, dorsal view. (e) Flower, lateral view. (f) Corolla split open to reveal positions of stamens. (g) Carpel, style and stigma. (h) Mature fruit. (i) Dehisced capsule with calyx removed. (j) Leaf margin pubescence. (k) Pubescence on upper stem. (l) Pubescence on lower stem. Scale bars: b, c, 3 cm; d–i, 2 cm; j–l, 1 cm; plant in (a) is 85 cm tall.
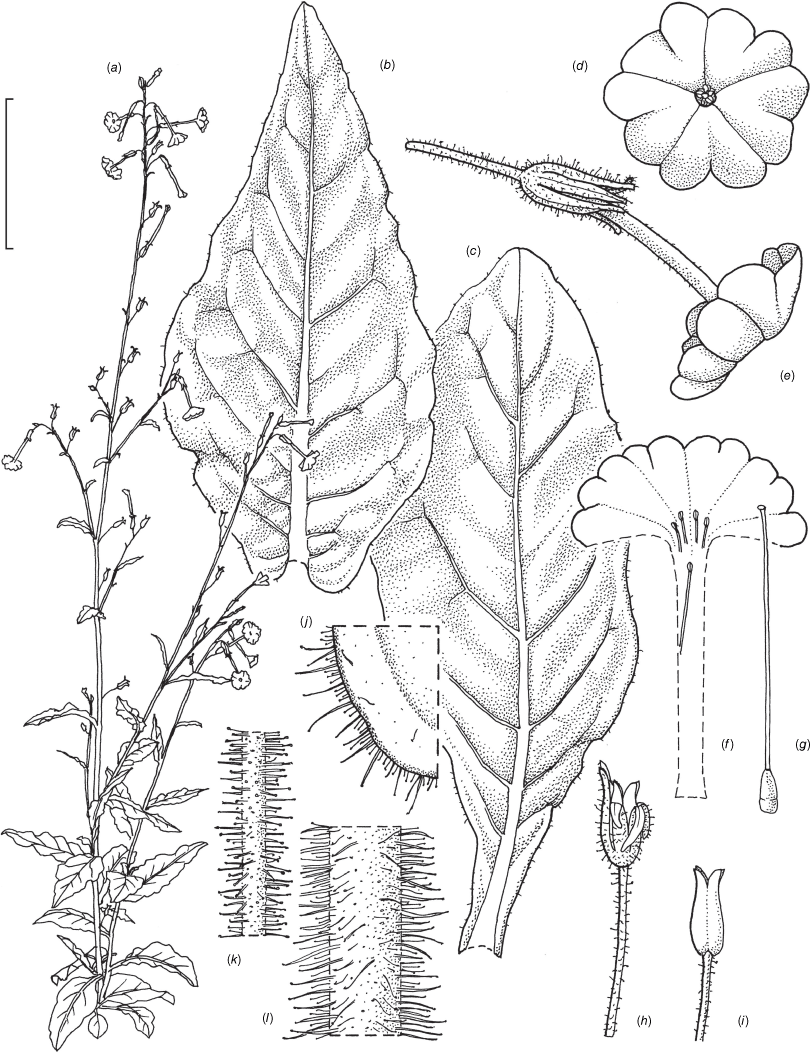
Illustration of Nicotiana latzii based on living plants cultivated at the Royal Botanic Gardens, Kew, grown from seeds from Latz 21442 (NT A0110082), Ethabuka Station, Queensland. Illustrated by Deborah Lambkin, vouchered as Chase & Christenhusz 18074 (K). (a) Floral limb, dorsal view. (b) Flower, lateral view. (c) Corolla split open to reveal positions of stamens. (d) Carpel, style and stigma. (e) Stem leaf. (f) Pubescence on leaf margin. (g) Pubescence on leaf surface. (h) Pubescence on upper stem with leaf base and axillary shoot. (i) Pubescence on lower stem. (j) Pubescence on calyx. (k) Dehisced mature fruit. (l) Capsule with calyx removed. (m) Habit. Scale bars: a–e, 2.0 cm; f–j, 5.0 mm; k, l, 1.0 cm; plant in (m) is 77 cm tall. Reproduced from Chase et al. (2023a in Australian Systematic Botany) with permission.
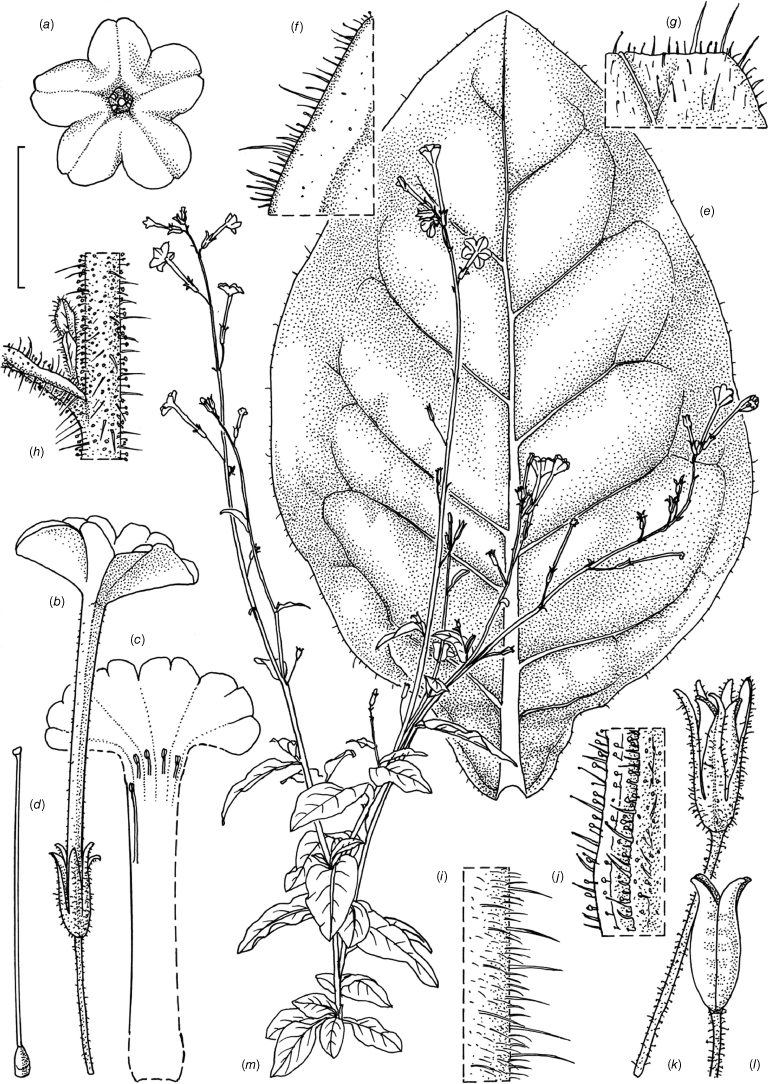
Nicotiana megalosiphon has a major split between the New South Wales and Queensland accessions (the latter 18175 and 18161; Fig. 3), and there is a case for recognising two entities on morphological grounds (longer floral tubes that remain pale green externally even during anthesis). The McPherson Range in Queensland on the border with New South Wales appears to be responsible for this north–south disjunction that has been noted in several animal and plant species complexes (Cracraft 1991; Crisp et al. 1995; Chapple et al. 2011; Simpson et al. 2018). The McPherson Range is an east–west spur of the predominantly north–south Great Dividing Range. The range has montane forests that represent a barrier to lowland and dry-forest plant species such as Nicotiana. Currently we refrain from recognising the New South Wales plants as a new species but further study with more extensive sampling may prove this to be warranted.
The techniques we used to genetically distinguish the species that we initially recognised as distinct on morphological, ecological and geographical grounds provide new dimensions to the issue of species delimitation. These also provide an objective basis for species delimitation that is independent of the other considerations (such as morphological interpretations) typically used in species circumscription. In situations such as that here, these techniques can provide clear results that support conclusions made on morphological grounds. These analyses confirm our previous delimitations (Chase and Christenhusz 2021; Chase et al. 2023a) for N. latifolia, N. latzii, N. megalosiphon and N. sessilifolia based solely on a RAxML analysis.
At this stage of our studies, these are the only known members of this species complex. As for all species in N. sect. Suaveolentes, these species are problematic to distinguish if the material is in poor condition or fragmentary. Examining the leaves on the lower halves of the stems to determine whether there is a petiole, and if so, whether this is winged with an auriculate base, is important. The length of the floral tube is also a useful character and this is not significantly affected by the conditions under which the plants grew. In a season with plentiful rainfall, these species can all produce plants of 1.5–2.0 m tall, in which case identification is much easier, but if the plant is less than 50 cm tall, accurate identification will be difficult based on the morphological features and geographic locality should be emphasised. Establishing certainty regarding whether the specimen is a member of this species complex, for which the most distinctive feature is the mixture of hair types: long often multicellular hairs with a swollen base and short, small gland-tipped hairs especially on the upper stems and leaves, is also important (Fig. 1). The swollen bases of the long hairs (Fig. 1) remain on the stems and make these rough to the touch, especially when the stems are dry. As mentioned above, discerning the stamen condition (didynamous or not) and floral symmetry (oblique or symmetrical tubes) is difficult in herbarium specimens.
1 | Leaves with a winged petiole and auriculate base...2 Leaves with or without a winged petiole, without an auriculate base...4 |
2 | Floral tube (above the calyx) 1.0–2.0 cm long, oblique with didynamous stamens, South Australia and Northern Territory...N. simulans Floral tube (above the calyx) longer than 2.0 cm, not oblique, longer four stamens of same length, Northern Territory, Queensland, only north-easternmost South Australia...3 |
3 | Petiole so broadly winged as to appear sessile, easternmost Northern Territory and Queensland...N. latifolia Petiole not broadly winged, Northern Territory...N. sessilifolia |
4 | Petiole absent, western Queensland...N. latzii Petiole present, eastern Queensland and New South Wales...5 |
5 | Floral tube (above the calyx) longer than 4.0 cm, not oblique, longer four stamens of same length, northern New South Wales and eastern Queensland...N. megalosiphon Floral tube (above the calyx) 1.6–2.5 cm long, oblique, stamens didynamous, south-western Queensland, northern New South Wales and north-easternmost South Australia...N. palssonae |
Taxonomy
Nicotiana palssonae M.W.Chase & Christenh., sp. nov.
Type: AUSTRALIA. New South Wales: Broken Hill, Living Desert State Park, open mulga woodland, 302 m, 31°53′30″S, 141°25′58″E, 14 Oct. 2005, Palsson 419 (holo: NE 110953; iso: CANB, NSW).
Nicotiana palssonae is closely related (Fig. 3, 5) and similar in morphology to N. megalosiphon in lacking a winged petiole and auriculate leaf base but N. palssonae has a much shorter floral tube than N. megalosiphon. This species has the longer four stamens of two lengths (didynamous), whereas in N. megalosiphon all four stamens are of the same length. The new species is most morphologically similar to N. simulans but the latter often bears both petiolate and sessile leaves, whereas N. palssonae rarely has sessile leaves. The ecological characteristics are different and the two species are disjunct (see below).
Erect, herbaceous annual herbs forming a minimal rosette but with numerous large leaves in the basal portion of the stems, the main stem with major branches in the lower half of the inflorescence but with only a few small branches in the upper half. Leaves without petiole wings or with only narrowly winged petioles, the wing up to 1.0–1.5 cm wide, sometimes bullate, blades 8.2–12.8 × 1.5–4.8 cm (including petiole), broadly ovate to lanceolate, the apex blunt to acute in the basal leaves, becoming acuminate in those higher up, upper leaf base gently attenuate, in some cases slightly auriculate, margins entire, undulate, often basally bullate, uppermost leaves often sessile. Vestiture composed of short, dense, gland-tipped hairs and longer multicellular, non-glandular hairs on all surfaces. Inflorescence bracts sessile, linear lanceolate, ~0.5–2.3 cm long, the apex acuminate. Calyx 1.4–1.6 × 0.2 cm, one lobe slightly longer and one shorter than the others, the tips acuminate, slightly flaring to clasping, slightly wider and longer in fruit, extending 0.5 cm beyond and surrounding the capsule, the calyx slightly enlarging at maturity. Flowers white, outwardly to upward facing, upper part of the floral tube 0.2 cm longer than the lower part. Corolla tube 2.0–2.5 cm long (from tip of the calyx), oblique, 0.2 cm in diameter, with no throat cup, the limb 1.6–1.8 cm across, the lobes slightly cleft, cleft 0.1 cm deep, sinus 0.4 cm deep, lobes 0.6 cm long; four stamens near throat of the floral tube in two pairs, didynamous, the lower pair 0.1–0.2 cm longer than the upper pair and the fifth ~0.7 cm deeper in the tube. Fruit a capsule splitting in four lobes, 0.8–1.1 cm long at maturity (Fig. 8).
Occurring in south-western Queensland and north-easternmost South Australia through north-western New South Wales. The species is found in the north-western portion of the Murray–Darling and adjacent Lake Eyre Basins (Fig. 2).
Generally occurring in shaded sites under the shade of mulga, eucalypt, Casuarina or Callitris species but also in the open during seasons of high rainfall.
Named for Ruth Palsson, botanist associated with the Beadle Herbarium, University of New England (NE), who collected this species several times, including the type material.
Collections of this species have been confused with N. simulans but rarely with N. megalosiphon, with which N. palssonae has a partial range overlap. As far as we can determine, these never occur microsympatrically despite having generally similar habitat preferences. The species differs from N. simulans in leaf shape (lacking the winged petiole and often auriculate leaf base of the latter) and from N. megalosiphon in the smaller flowers (floral tubes 2.0–2.5 cm long v. 3.3–5.0 cm). The holotype is represented in the phylogenetic analysis (Fig. 4) by a secondary specimen grown from seeds collected at the same time as the type by Palsson and vouchered as Chase & Christenhusz 20014 (K, NE, CANB). The other accessions (Fig. 3, 4) with secondary vouchers at K are Chase & Christenhusz 18016 (Prendergast 354), Chase & Christenhusz 20005 (Palsson 321), Chase & Christenhusz 20010 (Palsson 410) and Chase & Christenhusz 20012 (Palsson 412).
AUSTRALIA. New South Wales: Yandama station, ~40 m W of Milparinka, −29°41′S, 141°25′E, 1 Apr. 1910, Collier s.n. (NSW48817); 44 miles [~70.8 km] W of Wilcannia, −31°42′S, 142°46′E, 11 Jan. 1959, Filson 1357 (CANB335359); 21 km north on Tilpa Road, N from Cobar-Wilcannia Road, −31°25′S, 145°53′E, 12 Aug. 1973, Cunningham 916 (CANB 834574); 500 m inside the gate on Pooncarie Road, Kinchega National Park, −32°31′12″S, 142°10′12″E, Sep. 1983, Fox 8309018 (NSW 575645); Nocoleche Nature Reserve, W side of Paroo River, ~15.4 km due S of Shearer’s Quarters, −29°59′20″S, 144°7′9″E, 18 Jan. 2017, Albrecht 14779 (CANB 897287); Narran Lake NR, Kurrajong Road, 142 m, −29°44′43″S, 147°33′13.2″E, 23 May 2020, Palsson 321 (NE110374); South of Bourke, Kidman Way, ~8 km north of intersection with Wilga Downs Rd, 205 m, −30°57′14″S, 145°53′51″E, 29 Sep. 2020, Palsson 410 (NE 110888); Gundabooka National Park, Ben Lomond Gorge, 192 m, −30°34′18″S, 145°42′47″E, 8 Sep 2020, Palsson 412 (NE 110890). Queensland: Warrego, 15.5 km SE of Wynburn turn-off, on old Quilpie-Charleville road, 230 m, −26°52′24.9″S, 144°35′33.3″E, 22 Sep. 1990, Prendergast 354 (MSB 87373; BRI AQ0501528, K).
Declaration of funding
Financial support to prepare the RAD library and analyse data was provided by research grants P26548-B22 and P33028-B of the Austrian Science Fund (FWF) granted to Rose Samuel. Fieldwork was funded by honorary and visiting professorships from The University of Western Australia and Curtin University to M. W. Chase.
Acknowledgements
We thank the agencies and rangers responsible for the national parks and reserves of Western Australia, the Northern Territory, Queensland and New South Wales for issuing collecting permits in the years 2015–2019 to M. W. Chase and for providing assistance on the ground. We thank the curators of the following herbaria: AD, BRI, CANB, CNS, NSW, NT, PERTH and K for removal of viable seeds from herbarium specimens. Seeds were imported to the UK and living material was cultivated in the Quarantine House at the Royal Botanic Gardens, Kew under the supervision of Sara Redstone and Joanna Bates, whose assistance is gratefully acknowledged. Herbarium specimens made from these cultivated specimens have been returned to Australian herbaria.
References
Bombarely A, Rosli HG, Vrebalov J, Moffett P, Mueller LA, Martin B (2012) A draft genome sequence of Nicotiana benthamiana to enhance molecular plant–microbe biology research. Molecular Plant-Microbe Interactions 25, 1523-1530.
| Crossref | Google Scholar | PubMed |
Bouckaert RR, Heled J (2018) DensiTree 2: seeing trees through the forest. bioRxiv 012401 [Preprint, posted 8 December 2014].
| Crossref | Google Scholar |
Bryant D, Bouckaert R, Felsenstein J, Rosenberg NA, RoyChoudhury A (2012) Inferring species trees directly from biallelic genetic markers: bypassing gene trees in a full coalescent analysis. Molecular Biology and Evolution 29, 1917-1919.
| Crossref | Google Scholar | PubMed |
Catchen J, Hohenlohe PA, Bassham S, Amores A, Cresko WA (2013) Stacks: an analysis tool set for population genomics. Molecular Ecology 22, 3124-3140.
| Crossref | Google Scholar | PubMed |
Cauz‐Santos LA, Dodsworth S, Samuel R, Christenhusz MJ, Patel D, Shittu T, Jakob A, Paun O, Chase MW (2022) Genomic insights into recent species divergence in Nicotiana benthamiana and natural variation in Rdr1 gene controlling viral susceptibility. The Plant Journal 111, 7-18.
| Crossref | Google Scholar | PubMed |
Cauz-Santos LA, Samuel R, Metschina D, Christenhusz MJM, Dodsworth S, Dixon KW, Conran JG, Paun O, Chase MW (2024) Recent speciation and adaptation to aridity in the ecologically diverse Pilbara region of Australia enabled the native tobaccos (Nicotiana; Solanaceae) to colonize all Australian deserts. Molecular Ecology 33, e17498.
| Crossref | Google Scholar | PubMed |
Chapple DG, Hoskin CJ, Chapple SN, Thompson MB (2011) Phylogeographic divergence in the widespread delicate skink (Lampropholis delicata) corresponds to dry habitat barriers in eastern Australia. BMC Evolutionary Biology 11, 191.
| Crossref | Google Scholar | PubMed |
Chase MW, Christenhusz MJM (2018) Nicotiana stenocarpa. Curtis’s Botanical Magazine 35, 318-327.
| Crossref | Google Scholar |
Chase MW, Christenhusz MJM (2021) Nicotiana megalosiphon. Curtis’s Botanical Magazine 38, 425-434.
| Crossref | Google Scholar |
Chase MW, Dodsworth S, Christenhusz MJM (2021) Nicotiana walpa. Curtis’s Botanical Magazine 38, 298-308.
| Crossref | Google Scholar |
Chase MW, Cauz-Santos LA, Dodsworth S, Christenhusz MJM (2022) Taxonomy of the Australian Nicotiana benthamiana complex (Nicotiana section Suaveolentes; Solanaceae): five species, four newly described, with distinct ranges and morphologies. Australian Systematic Botany 35, 345-363.
| Crossref | Google Scholar |
Chase MW, Christenhusz MJ, Cauz-Santos LA, Nollet F, Bruhl JJ, Andrew DD, Palsson R, Jobson RW, Taseski GM, Samuel R (2023a) Nine new species of Australian Nicotiana (Solanaceae). Australian Systematic Botany 36, 167-205.
| Crossref | Google Scholar |
Chase MW, Samuel R, Leitch AR, Guignard MS, Conran JG, Nollet F, Fletcher P, Jakob A, Cauz-Santos LA, Vignolle G, Dodsworth S (2023b) Down, then up: non-parallel genome size changes and a descending chromosome series in a recent radiation of Australian allotetraploid plant species, Nicotiana section Suaveolentes (Solanaceae). Annals of Botany 131, 123-142.
| Crossref | Google Scholar | PubMed |
Clarkson JR, Symon DE (1991) Nicotiana wuttkei (Solanaceae), a new species from north-eastern Queensland with an unusual chromosome number. Austrobaileya 3, 389-392.
| Crossref | Google Scholar |
Cracraft J (1991) Patterns of diversification within continental biotas: hierarchical congruence among the areas of endemism of Australian vertebrates. Australian Systematic Botany 4, 211-227.
| Crossref | Google Scholar |
Crisp MD, Linder HP, Weston PH (1995) Cladistic biogeography of plants in Australia and New Guinea: congruent pattern reveals two endemic tropical tracks. Systematic Biology 44, 457-473.
| Crossref | Google Scholar |
Danecek P, Auton A, Abecasis G, Albers CA, Banks E, DePristo MA, Handsaker RE, Lunter G, Marth GT, Sherry ST, McVean G (2011) The variant call format and VCFtools. Bioinformatics 27, 2156-2158.
| Crossref | Google Scholar | PubMed |
Drummond AJ, Suchard MA, Xie D, Rambaut A (2012) Bayesian phylogenetics with BEAUti and the BEAST 1.7. Molecular Biology and Evolution 29, 1969-1973.
| Crossref | Google Scholar | PubMed |
Evanno G, Regnaut S, Goudet J (2005) Detecting the number of clusters of individuals using the software structure: a simulation study. Molecular Ecology 14, 2611-2620.
| Crossref | Google Scholar | PubMed |
Horton P (1981) A taxonomic revision of Nicotiana (Solanaceae) in Australia. Journal of the Adelaide Botanical Garden 3, 1-56 Available at https://www.jstor.org/stable/23872354.
| Google Scholar |
Korneliussen TS, Albrechtsen A, Nielsen R (2014) ANGSD: analysis of next generation sequencing data. BMC Bioinformatics 15, 356.
| Crossref | Google Scholar | PubMed |
Kopelman NM, Mayzel J, Jakobsson M, Rosenberg NA, Mayrose I (2015) CLUMPAK: a program for identifying clustering modes and packaging population structure inferences across K. Molecular Ecology Resources 15(5), 1179-1191.
| Crossref | Google Scholar |
Lewis PO (2001) A likelihood approach to estimating phylogeny from discrete morphological character data. Systematic Biology 50(6), 913-925.
| Crossref | Google Scholar |
Lexer C, Joseph J, van Loo M, Prenner G, Heinze B, Chase MW, Kirkup D (2009) The use of digital image‐based morphometrics to study the phenotypic mosaic in taxa with porous genomes. Taxon 58, 349-364.
| Crossref | Google Scholar |
Li H, Durbin R (2009) Fast and accurate short read alignment with Burrows-Wheeler transform. Bioinformatics 25, 1754-1760.
| Crossref | Google Scholar | PubMed |
Lischer HEL, Excoffier L (2012) PGDSpider: an automated data conversion tool for connecting population genetics and genomics programs. Bioinformatics 28, 298-299.
| Crossref | Google Scholar | PubMed |
McKenna A, Hanna M, Banks E, Sivachenko A, Cibulskis K, Kernytsky A, Garimella K, Altshuler D, Gabriel S, Daly M, DePristo MA (2010) The genome analysis toolkit: a MapReduce framework for analyzing next-generation DNA sequencing data. Genome Research 20, 1297-1303.
| Crossref | Google Scholar | PubMed |
Paradis E, Schliep K (2019) ape 5.0: an environment for modern phylogenetics and evolutionary analyses in R. Bioinformatics 35, 526-528.
| Crossref | Google Scholar | PubMed |
Paun O, Turner B, Trucchi E, Munzinger J, Chase MW, Samuel R (2016) Processes driving the adaptive radiation of a tropical tree (Diospyros, Ebenaceae) in New Caledonia, a biodiversity hotspot. Systematic Biology 65, 212-227.
| Crossref | Google Scholar | PubMed |
Rambaut A, Drummond AJ, Xie D, Baele G, Suchard MA (2018) Posterior summarization in Bayesian phylogenetics using Tracer 1.7. Systematic Biology 67, 901-904.
| Crossref | Google Scholar | PubMed |
Schiavinato M, Marcet-Houben M, Dohm JC, Gabaldón T, Himmelbauer H (2020) Parental origin of the allotetraploid tobacco Nicotiana benthamiana. The Plant Journal 102, 541-554.
| Crossref | Google Scholar | PubMed |
Simpson L, Clements MA, Crayn DM, Nargar K (2018) Evolution in Australia’s mesic biome under past and future climates: insights from a phylogenetic study of the Australian Rock Orchids (Dendrobium speciosum complex, Orchidaceae). Molecular Phylogenetics and Evolution 118, 32-46.
| Crossref | Google Scholar | PubMed |
Skotte L, Korneliussen TS, Albrechtsen A (2013) Estimating individual admixture proportions from next generation sequencing data. Genetics 195, 693-702.
| Crossref | Google Scholar | PubMed |
Stamatakis A (2014) RAxML version 8: a tool for phylogenetic analysis and post-analysis of large phylogenies. Bioinformatics 30, 1312-1313.
| Crossref | Google Scholar | PubMed |
Symon DE (1984) A new species of Nicotiana (Solanaceae) from Dalhousie Springs, South Australia. Journal of the Adelaide Botanical Garden 7, 117-121 Available at https://www.jstor.org/stable/23874136.
| Google Scholar |
Symon DE (1998) A new Nicotiana (Solanaceae) from near Coober Pedy, South Australia. Journal of the Adelaide Botanical Garden 18, 1-4 Available at https://www.jstor.org/stable/23874102.
| Google Scholar |
Symon DE, Kenneally KF (1994) A new species of Nicotiana (Solanaceae) from near Broome. Western Australia. Nuytsia 9, 421-425.
| Crossref | Google Scholar |
Wang L-G, Lam TT-Y, Xu S, Dai Z, Zhou L, Feng T, Guo P, Dunn CW, Jones BR, Bradley T, Zhu H, Guan Y, Jiang Y, Yu G (2020) treeio: an R package for phylogenetic tree input and output with richly annotated and associated data. Molecular Biology and Evolution 37(2), 599-603.
| Crossref | Google Scholar |
Symon DE, Lepschi BJ (2007) A new status in Nicotiana (Solanaceae): N. monoschizocarpa (P.Horton) Symon & Lepschi. Journal of the Adelaide Botanical Garden 21, 92.
| Google Scholar |
Yu G, Smith DK, Zhu H, Guan Y, Lam TT (2017) ggtree: an R package for visualization and annotation of phylogenetic trees with their covariates and other associated data. Methods in Ecology and Evolution 8, 28-36.
| Crossref | Google Scholar |