What we can learn from the bovine embryo and mouse models to enable in vitro gametogenesis in cattle
Anna C. Denicol
A
Abstract
The development of in vitro gametogenesis (IVG) in the mouse opened up unforeseen possibilities for assisted reproduction. The development of this technology to be used in cattle production could accelerate the rate of genetic selection by dramatically reducing the generation interval, while decreasing the environmental impact of livestock production as the need to grow animals in the process of genetic selection would be reduced or even eliminated. Although several steps of the process of IVG such as in vitro oocyte maturation and fertilization, and embryo production are already routinely performed in cattle, other steps of the system such as in vitro follicle and oocyte development are still rudimentary. The stable derivation of bovine pluripotent stem cells is the starting point without which IVG cannot be realized. However, producing a primordial germ cell and taking this cell through oogenesis and folliculogenesis in a dish will require a more detailed understanding of the milestones that need to be accomplished in vivo before they can be recapitulated in vitro. In particular, understanding the regulatory circuitry of germ cell specification in the embryo, the timing and events related to development of the germ cell program, and the factors necessary to make a competent egg, will need to be uncovered. Here, we review the process of IVG and provide a brief description of the current advances and bottlenecks related to in vitro oogenesis and folliculogenesis in cattle. Finally, we provide a brief comparison between mice and cows in this regard.
Keywords: bovine, cattle, embryo, embryonic stem cell, fetus, folliculogenesis, gametogenesis, oocyte, stem cell.
Introduction
The publication of a detailed report of in vitro gametogenesis (IVG) in the mouse in 2016 (Hikabe et al. 2016) changed the way we see reproductive biology, and more specifically, our potential to replicate the creation of life in the laboratory. At that time, there were no reported stable pluripotent stem cells in the bovine, making this groundbreaking milestone a distant reality for cattle reproductive biologists. The first publication of long-term culture of stable bovine embryonic stem cells (bESCs) in 2018 (Bogliotti et al. 2018) and establishment of bovine-induced pluripotent stem cells (biPSCs) in 2021 (Pillai et al. 2021; Su et al. 2021) changed this scenario, although there are still significant challenges to overcome as we learn more about bovine pluripotent stem cells and how to turn them into gametes (Botigelli et al. 2023).
When thinking about female gametogenesis – the production of oocytes – one must consider all the different phases that germ cells go through before reaching their full potential. The germ cell founders, or primordial germ cells (PGCs), are specified in the epiblast of the early gastrula-stage embryo. These very special cells undergo extensive reprogramming while they migrate from their place of origin to their destination: the primitive gonad. At the time of gonad colonization, PGCs are not yet fully committed to the female gametogenic program; this commitment is dependent on timely expression of the RNA-binding protein DAZL (Gill et al. 2011). As female germ cells – now oogonia – enter and arrest at the prophase I of meiosis I, they assemble in germ cell cysts which will gradually break up giving rise to an oocyte surrounded by a thin layer of pre-granulosa cells as primordial follicles first appear. Gametogenesis continues as the process of folliculogenesis, or the development of follicles within the ovary, begins; during this process, the oocyte must continually communicate with its surrounding somatic cells for activation and development through the different preantral and then antral follicle phases before reaching the pre-ovulatory stage (Matzuk et al. 2002; Martinez et al. 2023).
In order to replicate such a complex and long process in vitro, it is imperative that we have a deep understanding of the cellular and molecular milestones achieved by the germ and somatic cells at different stages, including critical markers that would inform whether a process is leading to survival or atresia. Such details and precise markers are still largely elusive in the bovine species. Extrapolation of knowledge from mouse models into the bovine has provided numerous successes and also important setbacks. At the same time, the incredible advancement of bovine embryo technologies, together with state-of-the-art studies of the embryo of closer domestic species such as the pig (Kobayashi et al. 2017) and rabbit (Kobayashi et al. 2021), greatly facilitated the transfer of knowledge from other species to the bovine embryo.
Bovine embryos are readily produced in vitro in large scale (Viana 2022). But although the number of embryos produced in vitro grew exponentially within the last 10 years, oocyte yield/donor cow has remained virtually the same (Fig. 1; Viana 2022). To take advantage of the large pool of gametes present in the ovary, technologies exploiting the growth of preantral follicles (ideally primordial follicles, the most abundant stage) need to be developed. Isolating and growing bovine preantral follicles in the laboratory is no trivial task, and to this day there are no reports of successful production of a fully-grown, GV-stage oocyte in vitro from a primordial follicle. Most, if not all, techniques such as 3D culture, co-culture with somatic cells, or in situ follicle culture inside ovarian fragments have been attempted in bovine after successful reports in the mouse, but did not yield similarly promising results. The long time necessary for bovine follicles to develop from the primordial to the antral stage – estimated to be at least 100 days (Lussier et al. 1987; Fair 2010) – and the dramatic changes that follicles undergo during development likely contribute to this lack of success. But perhaps most importantly, there is a general lack of knowledge about bovine preantral folliculogenesis, which remains largely a black box within the field of ovarian biology. Becoming more familiar with the molecular processes regulating the development of bovine PGC, oogonia, early oocytes and follicles will likely require large scale descriptive studies, which is facilitated nowadays by the evermore accessible -omics techniques. Although progress in the development of advanced assisted reproductive technologies involving IVG will continue to be made during this period, the full potential of these technologies will be unlocked as our knowledge of basic reproductive and developmental biology is expanded.
Although the number of cows reported as oocyte donors in North America has increased dramatically from 15,537 in 2012 to 178,682 in 2022, the number of oocytes retrieved/procedure has not accompanied this increase, and instead remained relatively unchanged during the same 10-year period. Numbers above the yellow line depict the average oocytes/donor cow aspirated in each year. Source: Viana (2022).
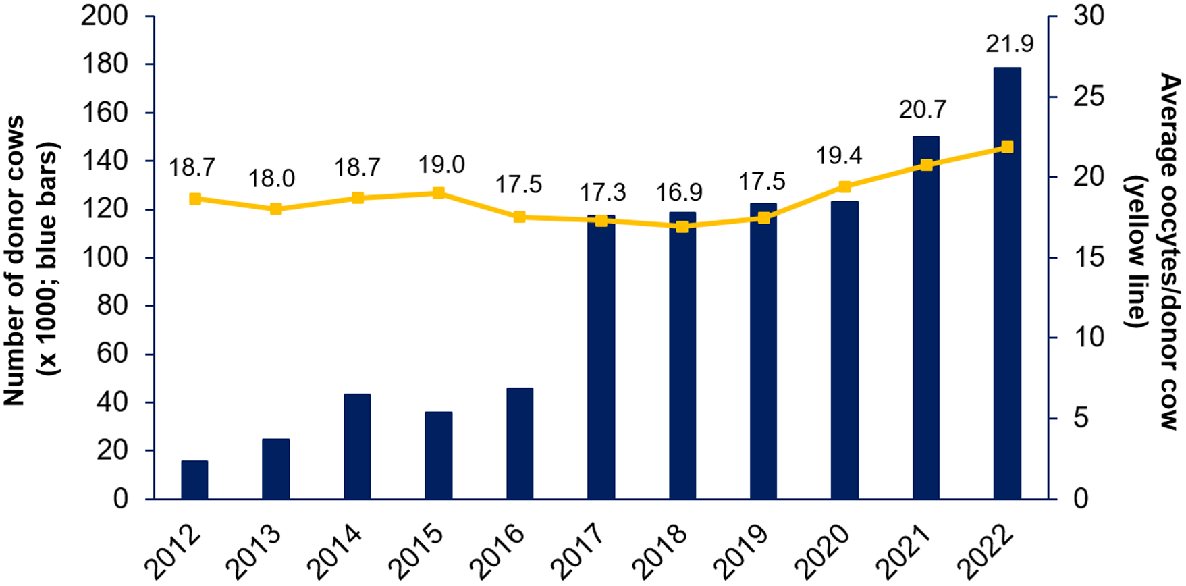
In this review, we will cover the advances made in mouse IVG and the current status of this technology in cattle. We will also discuss oogenesis and folliculogenesis in the context of relevance to assisted reproduction. Finally, we will draw parallels between mice and cattle in regards to development and reproduction, emphasizing aspects that still need to be learned in the cow to fulfill the promise of IVG in this species.
The concept of in vitro gametogenesis (IVG)
As the term implies, IVG entails the replication of the entire gametogenic cycle – from a primordial germ cell to a fully-grown oocyte – in a Petri dish. The production of gametes in vitro from other cell sources – notably, stem cells – has widely different applications in different species. For cattle, it would mean a dramatic acceleration in genetic progress considering that an oocyte could potentially be derived from a stem cell in approximately 4–5 months (Goszczynski et al. 2019) compared with the breeding schemes used today which rely on the physiological 2-year generational interval. Indeed, it has been estimated that breeding programs employing IVG associated with genomic selection could achieve 10× more genetic improvement in the same amount of time compared to currently employed breeding strategies and genomic selection (Goszczynski et al. 2019).
In the mouse, IVG encompasses several steps (Hayashi and Saitou 2013; Hikabe et al. 2016). The protocol employs pluripotent stem cells which after a short period of pre-differentiation into epiblast-like cells, are subjected to induction into primordial germ cell-like cells (PGC-LCs). These cells can be sorted from non-induced cells via the utilization of fluorescently-tagged reporter germ cell markers, and successfully induced PGC-LCs are combined with somatic cells (from fetal ovaries or from differentiation of PSCs; Yoshino et al. 2021) to form ovary aggregates. Over the course of approximately 3 weeks, these aggregates begin to show the development of follicles, which are then isolated and cultured for an additional 11 days until a fully-grown GV stage oocyte is produced. This oocyte can finally be subjected to in vitro maturation to yield a metaphase II oocyte capable of fertilization and embryonic development. The embryos resulting from this process could be transferred and generate viable and fertile offspring. Moreover, the inner cell mass of blastocysts produced via in vitro oogenesis originated new embryonic stem cell lines, from which a second round of MII oocytes could be produced (Hikabe et al. 2016). Although this report is still unparalleled in the novelty of its advances, the authors acknowledged that the low developmental (<30% maturation from GV to MII oocyte) and birth rates (3.5%) were likely results of unintended reprogramming effects (or lack thereof) of the prolonged in vitro environment.
Germ cell specification and development in the bovine embryo
One of the main bottlenecks in developing an efficient pipeline to enable IVG is the fact that we still have a poor understanding of many processes guiding the specification and development of germ cells in cattle, as well as a lack of reliable methods for the culture of germ cells and early-stage follicles in vitro. Early attempts to report the timing of PGC emergence in cattle placed this event around day 18 of development (Wrobel and Süß 1998). At this time, the bovine embryo is undergoing gastrulation and the myriad of signaling inputs necessary for differentiation of the three germ layers – ectoderm, mesoderm, and endoderm – is fully in place (Maddox-Hyttel et al. 2003). Compared to the pig – a species in which a detailed description of germ cell specification placed it between days 11.5–12.5 of development, during the pre- to early-primitive streak stages (Kobayashi et al. 2017) – PGC specification in cattle would be already underway at day 18. In fact, our recent observations determined that a small number of PGC is present in the bovine embryo at day 16 of development (C Guiltinan, RC Botigelli, RB Arcanjo, J Smith, AC Denicol, unpubl. obs.). It remains to be determined if this is the earliest timepoint or whether these cells can already be identified earlier. In the pig and other species that develop as a bilaminar disk embryo (such as rabbits), the emerging PGC co-express the early germ cell marker triad PRDM1, SOX17, and TFAP2C (Kobayashi et al. 2017, 2021). The unique and timely co-expression of these or other markers remains to be demonstrated in cattle embryos. Between specification and colonization of the forming ovary, PGCs undergo extensive epigenetic reprogramming including DNA demethylation, X chromosome reactivation, and repression of the somatic program (Alberio et al. 2021). For this critical period of formation of the germline, our knowledge of the events happening in the cow embryo is still incomplete, although it is generally accepted that they are shared with other bilaminar disk embryos.
Folliculogenesis in the cow from the establishment of the ovarian reserve to development of antral follicles
Similar to humans and other large mammals with prolonged gestation length, the establishment of the ovarian follicular reserve in cattle occurs during fetal life (Fortune et al. 2013), making it more challenging to study compared to mouse follicles which are established neonatally. The appearance of the first primordial follicles following breakdown of the germinal cysts has been reported to occur at approximately 90 days of bovine fetal development. Interestingly, and as reported in other species, the first follicles appear in the inner cortex, while the outer cortex is still occupied by an abundant population of less developed, pre-meiotic oogonia. Between day 90 and 150 there is a dramatic increase in the number of follicles, and activation of some primordial follicles begins to occur (Yang and Fortune 2008). The asynchronous nature and the timeline of germ cell development during fetal life in the bovine is remarkably similar to that in humans, in which a dynamic pattern of expression of OCT4, DDX4, and other markers of germ cell progression was observed between weeks 14.5 and 40 of development (Stoop et al. 2005). This highlights the cow as a close and readily available model species to humans to better understand germ cell development in the ovary.
The primordial follicles formed during fetal development constitute the ovarian reserve, i.e. the population of germ cells that will be available during a female’s reproductive life. These follicles contain one oocyte arrested at the first meiotic prophase, enclosed by one layer of pre-granulosa cells. Although most primordial follicles remain dormant until puberty, when the female begins to have reproductive cycles, the oocyte and granulosa cells are already in communication. Indeed, evidence from the mouse shows that it is signals from the oocyte that trigger activation of the follicle to initiate growth. Local signaling pathways, notably PI3K/PTEN signaling, are known to play a definitive role in primordial follicle activation by triggering exclusion of the transcription factor FOXO3 from the oocyte nucleus into the cytoplasm (Adhikari and Liu 2009; Bromfield and Sheldon 2013). Local upstream regulators such as AMH and Kit ligand have been identified as important regulators of PI3K signaling and primordial follicle activation; however, it is still not clear how some follicles are selected for activation while others remain dormant (Adhikari and Liu 2009).
As a primordial follicle begins to grow and transition into a primary follicle, its granulosa cells assume a cuboidal shape (from the previous flattened shape) and this morphological change is accompanied by a rapid increase in metabolic activity in these cells. At this point, there are no evident gap junctions between somatic and germ cell compartments, although bilateral communication is already present. Proliferation of granulosa cells is an important hallmark of folliculogenesis, and as the follicle begins to form a second and subsequent layers, it transitions from the primary into the secondary stage. Up until this stage, ovarian follicles are still categorized as preantral as they have not yet developed the antral cavity. It is generally accepted that during preantral stages, there is no requirement for systemic input into follicle development, although this notion has been changing as more evidence demonstrates that hormones such as FSH play roles in preantral follicle development (reviewed by Morton et al. 2023).
Adequate antral development relies heavily on proper preparation of the follicle during the preantral stage. Accumulation of RNAs within the oocyte for future use by the embryo before embryonic genome activation largely happens during the preantral-to-antral transition, and ceases in the mid-antral stage (Lodde et al. 2007, 2008). Approximately 95% of the total and poly-A RNA that will be passed on to the zygote have been accumulated by the oocyte at 65–70% of its final volume (Sternlicht and Schultz 1981); this places the oocyte at the secondary/early antral stage when this critical step is achieved and again highlights the importance of a better understanding of the regulation of early, namely preantral folliculogenesis.
Advances and bottlenecks to recapitulate oogenesis and folliculogenesis in vitro in cattle
The first report of differentiation and preliminary characterization of PGC-LCs from bovine ESCs was published in 2024 (Shirasawa et al. 2024), utilizing a combination of WNT signaling activation and inhibition in primed pluripotent cells. Compared to results previously reported with mouse (Hikabe et al. 2016) and human (Vijayakumar et al. 2023; Irie et al. 2024) cells, the induction of bovine ESCs had overall low efficiency and marked heterogeneity between the cell lines utilized. Likewise, candidate surface markers well established in other species such as ITGA6 and PDPN did not show a distinct pattern of expression in bovine PGC-LCs compared to non-induced bovine ESCs, although the authors acknowledged the limitation regarding validated antibodies for use in bovine cells (Shirasawa et al. 2024).
There are no reports of complete folliculogenesis in vitro from the primordial to the pre-ovulatory stage except in mice. Bovine follicles >200 μm diameter (secondary stage containing multiple layers of granulosa cells and the beginnings of a theca cell layer) can grow and form an antrum in 2D systems (Ferreira et al. 2020). Although it is generally accepted that death is a common endpoint throughout follicle development, the approximate proportion of primary follicles that would be destined to grow versus undergo atresia at the time of isolation from the ovary, even though they appear normal morphologically (McDonnell et al. 2022), is unknown. Therefore, it is possible that a large proportion of the bovine follicles placed in culture are already destined to become atretic, or that the culture systems are simply not adequate to promote their growth.
Attempts to devise 3D systems to improve survival and growth of preantral follicles at the primordial or primary stages have included the use of alginate at different concentrations, Matrigel, collagen, and other matrices. Alginate has been reported for encapsulation of goat ovarian tissue explants in an attempt to improve primordial follicle survival in vitro, but the improvement was limited (Correia et al. 2020). We have recently reported the culture of primary follicles in a bio-degradable poly-ethylene glycol (PEG) hydrogel designed with crosslinked peptides that are targets of enzymes produced by growing preantral follicles. The idea behind this approach is to mimic the extracellular matrix remodeling that occurs as follicles grow in their native environment. Follicles at the primary stage (41–60 μm diameter) had a modest growth in 10 days when encapsulated in the PEG system, but not in the 2D system (Candelaria et al. 2024). Further studies keeping follicles in culture for longer will be critical to evaluate if growth can be sustained in the PEG system.
Primordial and primary follicles do not yet possess a layer of theca cells; this is a characteristic of the secondary stage of development. Therefore, culture systems designed to grow these earlier stages will likely need to include somatic support cells that can be recruited into the follicular unit as it occurs in the native ovary. Several cell types have been used either as feeder cells, co-encapsulated within the hydrogel or used as a source of factors in conditioned culture media (Green et al. 2019; Silva et al. 2024), with success. We have used a mixture of bovine cortical cells freshly isolated from the ovary and co-cultured with preantral follicles in PEG hydrogels with controlled degradation. We found that the ovarian cells remained viable over a 10-day culture system; however, they lost the pre-theca cell gene expression profile. In this context, the directed differentiation of the now available bovine pluripotent stem cells into pre-theca and other ovarian somatic cells could be advantageous to build a more defined artificial ovary environment (Candelaria et al. 2024).
Cows are not big mice: what can and cannot be extrapolated, and what we still do not know
Many of the advancements in in vitro oogenesis/gametogenesis and folliculogenesis in mice have been tested in the cow, but with limited and variable success. The preparation for gastrulation in the murine embryo results in formation of the egg cylinder, whereas bovine embryos (as well as human, pig, and rabbit embryos) develop as a bilaminar disk structure (Alberio et al. 2021). This morphological difference is accompanied by a distinct pattern of expression of the transcription factors fundamental for specification of primordial germ cells. In the mouse, PGCs express SOX2, whereas in bilaminar disk embryos SOX2 must be downregulated for PGCs to specify. Instead, these embryos upregulate SOX17 as one of the earliest transcription factors in the nascent PGCs. PRDM14, another transcription factor that plays a critical role in murine PGC specification, is weakly expressed in bilaminar disk embryos (Kobayashi et al. 2017, 2021).
Folliculogenesis begins in the cow fetus with the first primordial follicles appearing in the ovary at approximately day 90 of gestation (end of the first trimester; Yang and Fortune 2008; RB Arcanjo, RC Botigelli, C Guiltinan, J Smith, AC Denicol, unpubl. obs.). At approximately day 120, primary follicles and later secondary follicles can be identified, indicating that activation of the recently-formed ovarian reserve starts taking place before birth. These observations hold true for humans who have a very similar gestation length to the cow. In the mouse ovary, primordial follicles are not formed until 1–2 postnatal days and are followed by timely growth of the first wave of primary and secondary follicles, which can be seen by the second week of postnatal life. This precise synchrony in follicular development makes the mouse a practical species to study the molecular mechanisms regulating folliculogenesis, but does not reflect the subtler changes experienced by the slower growing bovine follicles. In a postpubertal female mouse, the entire folliculogenesis cycle from activation of primordial follicles to the preovulatory follicle stage takes approximately 22 days. In cows, similar to humans, the same cycle is estimated to take between 100 and 180 days (Fair 2010; Latorraca et al. 2024). Primordial follicles in mice and cattle have similar sizes: around 30 μm in diameter. The mouse preovulatory follicle reaches 400–500 μm diameter (Richard et al. 2024), representing an 8000× volumetric expansion. The cow follicle, on the other hand, ovulates at an average size of 15 mm (Ginther 2016), which accounts for a volumetric expansion of 100 million times or more (Fig. 2). From this, it becomes clear that the extent of remodeling that the follicle must exert in the surrounding ovarian stroma (or synthetic matrix) is very different between the two species, and perhaps one of the big reasons why in vitro culture systems designed for mouse follicles fail to promote follicular growth in cattle.
Follicular growth in large (cow, human) and small mammals (mice). Primordial follicles have similar size; the preovulatory follicle, however, grows significantly more in cows and humans compared to mice, and in a longer period of time. Follicular volume change calculations are based on the formula V = 4/3 × π × r3 where R is the follicle radius.
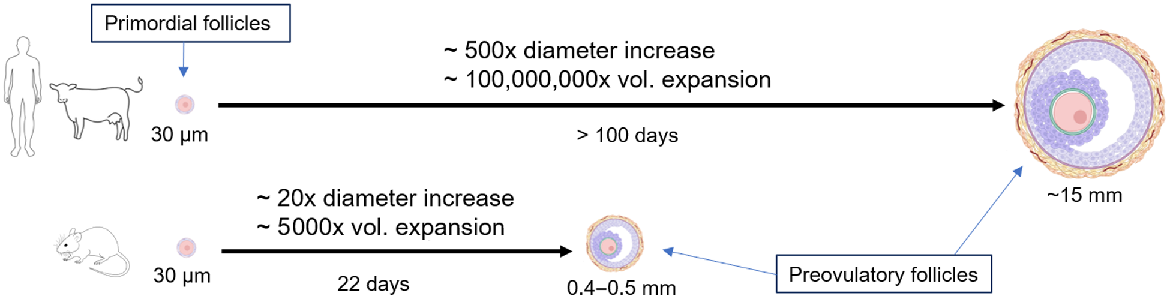
Another very important difference to consider when attempting to translate mouse systems into cows is the fact that mice are poly-ovulatory species while the cow is mono-ovulatory. Beyond the well-known differences in selection and dominance processes during the antral phase, this may have important implications to the rate of follicle atresia during preantral development. We have observed a high rate of variability in follicle growth between ovaries and within the same ovary when culturing preantral follicles at the primary stage in both 2D and 3D conditions (Candelaria et al. 2024). This has been observed by others as well (Ferreira et al. 2020) and raises the possibility that there is an inherently higher rate of atresia in growing bovine preantral follicles compared to mice. This would be important to investigate as it has important implications for assisted reproduction.
Future directions and concluding remarks
In vitro gametogenesis will undoubtfully become an available technology for assisted reproduction of cattle in the future. A more detailed understanding of the biology of PGC specification and oogenesis will be critical to maximize the potential of this technology, although the two fields will likely evolve in parallel. As with other assisted reproductive technologies, it will be imperative that the epigenetic landscape of in vitro-produced oocytes be studied and compared to that of in vivo-produced oocytes.
Cattle has traditionally been a pioneer species in the use of assisted reproductive technologies with advances in embryo production in vivo and in vitro that are still unparalleled in other domestic species. As more species are studied in detail, it becomes clearer that relying only on the mouse as the main model may not be the best strategy as for many developmental and reproductive processes, mice could be regarded as the exception and not the rule. A deeper understanding of the molecular determinants of bovine embryo development and reproductive biology will be necessary as assisted reproductive technologies become more dependent on in vitro systems, such as is the case with IVG. The advances made to date in bovine in vitro PGC differentiation and follicle growth, although may seem modest, are very promising considering the young age of this research in cattle compared to the mouse.
References
Adhikari D, Liu K (2009) Molecular mechanisms underlying the activation of mammalian primordial follicles. Endocrine Reviews 30(5), 438-464.
| Crossref | Google Scholar | PubMed |
Alberio R, Kobayashi T, Surani MA (2021) Conserved features of non-primate bilaminar disc embryos and the germline. Stem Cell Reports 16(5), 1078-1092.
| Crossref | Google Scholar | PubMed |
Bogliotti YS, Wu J, Vilarino M, Okamura D, Soto DA, Zhong C, Sakurai M, Sampaio RV, Suzuki K, Izpisua Belmonte JC, Ross PJ (2018) Efficient derivation of stable primed pluripotent embryonic stem cells from bovine blastocysts. Proceedings of the National Academy of Sciences of the United States of America 115(9), 2090-2095.
| Crossref | Google Scholar |
Botigelli RC, Guiltinan C, Arcanjo RB, Denicol AC (2023) In vitro gametogenesis from embryonic stem cells in livestock species: recent advances, opportunities, and challenges to overcome. Journal of Animal Science 101, skad137.
| Crossref | Google Scholar |
Bromfield JJ, Sheldon IM (2013) Lipopolysaccharide reduces the primordial follicle pool in the bovine ovarian cortex ex vivo and in the murine ovary in vivo. Biology of Reproduction 88(4), 98.
| Crossref | Google Scholar | PubMed |
Candelaria JI, Botigelli RC, Guiltinan C, Shikanov A, Denicol AC (2024) Three-dimensional culture in a bioengineered matrix and somatic cell complementation to improve growth and survival of bovine preantral follicles. BioRxiv [Preprint].
| Crossref | Google Scholar |
Correia HHV, Lima LF, Sousa FGC, Ferreira ACA, Cadenas J, Paes VM, Alves BG, Shikanov A, Figueiredo JR (2020) Activation of goat primordial follicles in vitro: influence of alginate and ovarian tissue. Reproduction in Domestic Animals 55(1), 105-109.
| Crossref | Google Scholar | PubMed |
Fair T (2010) Mammalian oocyte development: checkpoints for competence. Reproduction, Fertility and Development 22(1), 13-20.
| Crossref | Google Scholar | PubMed |
Ferreira ACA, Sá NAR, Cadenas J, Correia HHV, Guerreiro DD, Alves BG, Lima LF, Celestino JJH, Rodrigues APPR, Gastal EL, Figueiredo JR (2020) Pituitary porcine FSH, and recombinant bovine and human FSH differentially affect growth and relative abundances of mRNA transcripts of preantral and early developing antral follicles in goats. Animal Reproduction Science 219, 106461.
| Crossref | Google Scholar | PubMed |
Fortune JE, Yang MY, Allen JJ, Herrick SL (2013) Triennial reproduction symposium: the ovarian follicular reserve in cattle: what regulates its formation and size? Journal of Animal Science 91(7), 3041-3050.
| Crossref | Google Scholar | PubMed |
Gill ME, Hu YC, Lin Y, Page DC (2011) Licensing of gametogenesis, dependent on RNA binding protein DAZL, as a gateway to sexual differentiation of fetal germ cells. Proceedings of the National Academy of Sciences of the United States of America 108(18), 7443-7448.
| Crossref | Google Scholar | PubMed |
Ginther OJ (2016) The theory of follicle selection in cattle. Domestic Animal Endocrinology 57, 85-99.
| Crossref | Google Scholar | PubMed |
Goszczynski DE, Cheng H, Demyda-Peyrás S, Medrano JF, Wu J, Ross PJ (2019) In vitro breeding: application of embryonic stem cells to animal production. Biology of Reproduction 100(4), 885-895.
| Crossref | Google Scholar | PubMed |
Green LJ, Zhou H, Padmanabhan V, Shikanov A (2019) Adipose-derived stem cells promote survival, growth, and maturation of early-stage murine follicles. Stem Cell Research & Therapy 10(1), 102.
| Crossref | Google Scholar | PubMed |
Hayashi K, Saitou M (2013) Generation of eggs from mouse embryonic stem cells and induced pluripotent stem cells. Nature Protocols 8(8), 1513-1524.
| Crossref | Google Scholar | PubMed |
Hikabe O, Hamazaki N, Nagamatsu G, Obata Y, Hirao Y, Hamada N, Shimamoto S, Imamura T, Nakashima K, Saitou M, Hayashi K (2016) Reconstitution in vitro of the entire cycle of the mouse female germ line. Nature 539(7628), 299-303.
| Crossref | Google Scholar |
Irie N, Kobayashi T, Azim Surani M (2024) Human primordial germ cell-like cell induction from pluripotent stem cells by SOX17 and PRDM1 expression. Methods in Molecular Biology 2770, 87-97.
| Crossref | Google Scholar | PubMed |
Kobayashi T, Zhang H, Tang WWC, Irie N, Withey S, Klisch D, Sybirna A, Dietmann S, Contreras DA, Webb R, Allegrucci C, Alberio R, Surani MA (2017) Principles of early human development and germ cell program from conserved model systems. Nature 546(7658), 416-420.
| Crossref | Google Scholar | PubMed |
Kobayashi T, Castillo-Venzor A, Penfold CA, Morgan M, Mizuno N, Tang WWC, Osada Y, Hirao M, Yoshida F, Sato H, Nakauchi H, Hirabayashi M, Surani MA (2021) Tracing the emergence of primordial germ cells from bilaminar disc rabbit embryos and pluripotent stem cells. Cell Reports 37(2), 109812.
| Crossref | Google Scholar | PubMed |
Latorraca LB, Galvão A, Rabaglino MB, D’Augero JM, Kelsey G, Fair T (2024) Single-Cell profiling reveals transcriptome dynamics during bovine oocyte growth. BMC Genomics 25(1), 335.
| Crossref | Google Scholar |
Lodde V, Modina S, Galbusera C, Franciosi F, Luciano AM (2007) Large-scale chromatin remodeling in germinal vesicle bovine oocytes: interplay with gap junction functionality and developmental competence. Molecular Reproduction and Development 74(6), 740-749.
| Crossref | Google Scholar | PubMed |
Lodde V, Modina S, Maddox-Hyttel P, Franciosi F, Lauria A, Luciano AM (2008) Oocyte morphology and transcriptional silencing in relation to chromatin remodeling during the final phases of bovine oocyte growth. Molecular Reproduction and Development 75(5), 915-924.
| Crossref | Google Scholar | PubMed |
Lussier JG, Matton P, Dufour JJ (1987) Growth rates of follicles in the ovary of the cow. Journal of Reproduction and Fertility 81(2), 301-307.
| Crossref | Google Scholar | PubMed |
Maddox-Hyttel P, Alexopoulos NI, Vajta G, Lewis I, Rogers P, Cann L, Callesen H, Tveden-Nyborg P, Trounson A (2003) Immunohistochemical and ultrastructural characterization of the initial post-hatching development of bovine embryos. Reproduction 125(4), 607-623.
| Crossref | Google Scholar | PubMed |
Martinez CA, Rizos D, Rodriguez-Martinez H, Funahashi H (2023) Oocyte-cumulus cells crosstalk: new comparative insights. Theriogenology 205, 87-93.
| Crossref | Google Scholar | PubMed |
Matzuk MM, Burns KH, Viveiros MM, Eppig JJ (2002) Intercellular communication in the mammalian ovary: oocytes carry the conversation. Science 296(5576), 2178-2180.
| Crossref | Google Scholar | PubMed |
McDonnell SP, Candelaria JI, Morton AJ, Denicol AC (2022) Isolation of small preantral follicles from the bovine ovary using a combination of fragmentation, homogenization, and serial filtration. Journal of Visualized Experiments 187, e64423.
| Crossref | Google Scholar | PubMed |
Morton AJ, Candelaria JI, McDonnell SP, Zgodzay DP, Denicol AC (2023) Review: roles of follicle-stimulating hormone in preantral folliculogenesis of domestic animals: what can we learn from model species and where do we go from here? Animal 17(Suppl 1), 100743.
| Crossref | Google Scholar |
Pillai VV, Koganti PP, Kei TG, Gurung S, Butler WR, Selvaraj V (2021) Efficient induction and sustenance of pluripotent stem cells from bovine somatic cells. Biology Open 10(10), bio058756.
| Crossref | Google Scholar |
Richard S, Zhou Y, Jasoni CL, Pankhurst MW (2024) Ovarian follicle size or growth rate can both be determinants of ovulatory follicle selection in mice. Biology of Reproduction 110(1), 130-139.
| Crossref | Google Scholar |
Shirasawa A, Hayashi M, Shono M, Ideta A, Yoshino T, Hayashi K (2024) Efficient derivation of embryonic stem cells and primordial germ cell-like cells in cattle. Journal of Reproduction and Development 70(2), 82-95.
| Crossref | Google Scholar | PubMed |
Silva AFB, Lima LF, Sousa RP, Silva RF, Neves GCS, Carvalho MAM, Ferreira ACA, Oliveira AC, Alves BG, Rodrigues APR, Gastal EL, Bordignon V, Figueiredo JR (2024) Stem cell-conditioned medium improves methylation patterns and quality of caprine preantral follicles. Reproduction 168(3), e230483.
| Crossref | Google Scholar |
Sternlicht AL, Schultz RM (1981) Biochemical studies of mammalian oogenesis: kinetics of accumulation of total and poly(A)-containing RNA during growth of the mouse oocyte. Journal of Experimental Zoology 215(2), 191-200.
| Crossref | Google Scholar | PubMed |
Stoop H, Honecker F, Cools M, de Krijger R, Bokemeyer C, Looijenga LHJ (2005) Differentiation and development of human female germ cells during prenatal gonadogenesis: an immunohistochemical study. Human Reproduction 20(6), 1466-1476.
| Crossref | Google Scholar | PubMed |
Su Y, Wang L, Fan Z, Liu Y, Zhu J, Kaback D, Oudiz J, Patrick T, Yee SP, Tian XC, Polejaeva I, Tang Y (2021) Establishment of bovine-induced pluripotent stem cells. International Journal of Molecular Sciences 22(19), 10489.
| Crossref | Google Scholar | PubMed |
Viana J (2022) 2022 statistics of embryo production and transfer in domestic farm animals: the main trends or the world embryo industry still stand. Embryo Transfer Newsletter 41(4), 20-38.
| Google Scholar |
Vijayakumar S, Sala R, Kang G, Chen A, Pablo MA, Adebayo AI, Cipriano A, Fowler JL, Gomes DL, Ang LT, Loh KM, Sebastiano V (2023) Monolayer platform to generate and purify primordial germ-like cells in vitro provides insights into human germline specification. Nature Communications 14(1), 5690.
| Crossref | Google Scholar | PubMed |
Wrobel KH, Süß F (1998) Identification and temporospatial distribution of bovine primordial germ cells prior to gonadal sexual differentiation. Anatomy and Embryology 197, 451-467.
| Crossref | Google Scholar | PubMed |
Yang MY, Fortune JE (2008) The capacity of primordial follicles in fetal bovine ovaries to initiate growth in vitro develops during mid-gestation and is associated with meiotic arrest of oocytes. Biology of Reproduction 78(6), 1153-1161.
| Crossref | Google Scholar | PubMed |
Yoshino T, Suzuki T, Nagamatsu G, Yabukami H, Ikegaya M, Kishima M, Kita H, Imamura T, Nakashima K, Nishinakamura R, Tachibana M, Inoue M, Shima Y, Morohashi KI, Hayashi K (2021) Generation of ovarian follicles from mouse pluripotent stem cells. Science 373(6552), eabe0237.
| Crossref | Google Scholar |