Current status of nonsurgical embryo transfer in swine
Maria A. Gil A B , Inmaculada Parrilla A B , Cristina Cuello A B and Emilio A. Martinez
A
B
Abstract
Embryo transfer in pigs is a reproductive technology with great potential because it enables the movement of high-value genetic material, in the form of embryos, with reduced transport costs and no health risks. However, its use has been limited for decades despite significant interest, primarily due to the need for laparotomy for embryo collection from donors and deposition into recipients. Over the past 30 years, various procedures have been developed to deposit embryos nonsurgically in the body of the uterus with promising results, but none sufficiently successful. Because the uterine body is not the ideal site for embryo deposition at the morula and blastocyst stages, a new method that is capable of depositing porcine embryos deep into the uterine horn has been developed. This nonsurgical deep uterine method is a simple, effective, and well-tolerated technique in gilts and sows, achieving high fertility and prolificacy using fresh, stored, and vitrified–warmed embryos under field conditions. The present review provides a brief overview of the current status of nonsurgical deep uterine embryo transfer and addresses the varied reproductive performance observed across farms when this technology was applied in commercial programs. In addition, several future directions for its on-farm commercial application are discussed.
Keywords: assisted reproductive technology, blastocyst, embryo, embryo transfer, fertility, intrauterine device, litter size, livestock, morula, pregnancy.
Introduction
Embryo transfer (ET) is a valuable tool in pig production. It facilitates the movement of desirable genetics with a marginal possibility of disease spread. Compared to transporting live animals, ET offers significant advantages, including reduced transportation costs and the avoidance of negative impacts on animal welfare. ET also plays a crucial role in the use of new genetic tools for genome editing (GE) in pigs. This technology allows targeted genetic modifications to alter the phenotype of pigs, which serves two key purposes. First, the creation of pigs with improved production traits relevant to agriculture, such as enhanced meat quality (Zou et al. 2018) and increased disease resistance (Aigner et al. 2010; Whitworth et al. 2014). Second, it facilitates the development of valuable biomedical pig models to advance disease research, regenerative medicine, and organ transplantation in humans (Hryhorowicz et al. 2020).
Despite these important applications and although the first litters from ET in pigs were obtained almost 75 years ago (Kvasnickii 2001), the commercial use of ET has been limited because of the need for surgical procedures for embryo collection and transfer. Additionally, the challenges associated with cryopreservation further restricted its commercial development. However, the situation has changed in the last two decades, primarily due to the development of a nonsurgical deep uterine (NsDU) ET method and vitrification procedures as an excellent alternative to the conventional slow freezing cryopreservation method. These advancements have opened the door for a more practical and efficient use of ET in the pig industry.
This review focuses on the practical applications of porcine NsDU-ET technology. We explore the fundamentals of this technology and its current achievements. We also discuss the key factors impacting the success rates of NsDU-ET and the experimental results achieved under field conditions. Additionally, we present the first findings from the implementation of this technology in commercial pig production programs and highlight the observed differences from the experimental results. Finally, we address several future indications for the commercial application of this technology. For the purposes of this review, we focus mainly on in vivo-derived embryos because they are currently the only type of embryo with potential for use in pig production.
A historical perspective on nonsurgical embryo transfer in pigs
Nonsurgical embryo transfer into the uterine body
While laparotomies were the only established method for embryo collection and transfer in the 1960s, a breakthrough occurred in 1968. Researchers demonstrated the possibility of achieving pregnancy in pigs using a nonsurgical ET technique (Polge and Day 1968). However, achieving consistent success with nonsurgical ET has remained elusive for many years. The anatomy of the cervical canal is a significant challenge for the insertion of a catheter during metestrus. Additionally, the length of the uterine horns makes it difficult to deposit embryos near the utero-tubal junction (UTJ), which is the natural location of morulae and blastocysts under normal physiological conditions. Renewed efforts in the 1990s explored new nonsurgical embryo deposition techniques. However, all of these experiments required recipient sedation before transfer. The resulting farrowing rates ranged from 5 to 30%, with litter sizes of 5 to 6 piglets (Reichenbach et al. 1993; Li et al. 1996). A significant advancement came in 1994 with a Dutch procedure for nonsurgical transfer of blastocysts directly into the uterine body (UB) of non-sedated sows (Hazeleger et al. 2000a). This method initially yielded promising results, with a pregnancy rate of 71% and a litter size of 11 piglets on day 37 of gestation. Unfortunately, these results declined when pregnancies progressed to term and resulted in farrowing rates of 33–40% and litter sizes of 6.7–7.4 piglets (Hazeleger and Kemp 1994; Ducro-Steverink et al. 2004). While these outcomes were encouraging, further advancements were clearly necessary to optimize fertility rates following nonsurgical ET. Recent developments include a novel nonsurgical ET catheter designed for simplified ET into the UB. This innovative catheter eliminates the need for extensive operator training. However, current research using a limited number of vitrified–warmed blastocysts per recipient has yielded farrowing rates that range from 25 to 67% and litter sizes of 3.0–3.5 piglets born alive (Hirayama et al. 2020; Tajima et al. 2020). These findings highlighted the need for further optimization.
Nonsurgical embryo transfer deep into the uterine horn
A key limitation of these nonsurgical ET procedures is that they only deposit embryos within the UB, which is not the natural location for transferred embryos. Surgical ET studies have shown significantly lower pregnancy rates (12%) when expanded blastocysts are placed in the UB compared to placement in the middle (88%) or the tip (81%) of the uterine horn (Wallenhorst and Holtz 1999). These findings suggest that the UB is not the optimal location for embryo deposition. To address this and other limitations associated with UB nonsurgical ET methods, we developed a research program in 1998. Our goal was to develop a novel nonsurgical method for transferring porcine morulae and blastocysts deeper into the uterine horns of non-sedated gilts and sows.
The greatest challenge in placing a catheter deep into a pig’s uterus without surgery is the cervix. Its firmness changes throughout the estrous cycle, and it is easier to pass the catheter through during metestrus. Additionally, the uterus itself is long and curved, which adds another obstacle. In 1999, we developed a special endoscope technique for NsDU insemination in non-sedated sows (Martinez et al. 2001) that overcomes these challenges. The endoscope was inserted via an artificial insemination (AI) spirette, crossed the cervical canal, and reached deep into the uterine horn. This procedure offered a safe and efficient approach and enabled rapid access to a uterine horn in a high proportion of sows. Despite its effectiveness, the cost of the endoscope and its delicate nature encouraged the development of a more practical solution: a flexible catheter inspired by the endoscope’s design (Fig. 1). This catheter was less expensive, easier to use, and worked in gilts and sows during a wider estrous cycle window, including days 4–6 of the estrous cycle (Martinez et al. 2004). The design was further optimized for ET. The tip was enlarged to prevent accidental perforation of the cervical and uterine walls during embryo placement. Additionally, high-quality material was used for the inner tubing to safeguard the embryos (Cuello et al. 2005). In 2008, Japanese researchers introduced a new design for an NsDU-ET catheter. Despite its innovative approach, the prototype has seen scarce use (Nakazawa et al. 2008; Yoshioka et al. 2012).
(a) Catheter designed for nonsurgical deep uterine embryo transfer (NsDU-ET) in gilts and sows during days 4–6 of the estrous cycle. The ET catheter is equipped with a soft, enlarged tip (ETCT) to minimize the risk of cervical or uterine wall injury. (b, c) Reproductive tract of a sow obtained from a slaughterhouse, longitudinally opened to reveal the uterine body (UB), cervical folds (CF), cervix entry (EC), and vagina. (d) Position of the artificial insemination catheter tip (AICT) at the cervix entry. (e) Detailed view of the ET catheter passing through the cervical folds and its correct placement within a uterine horn (arrowhead).
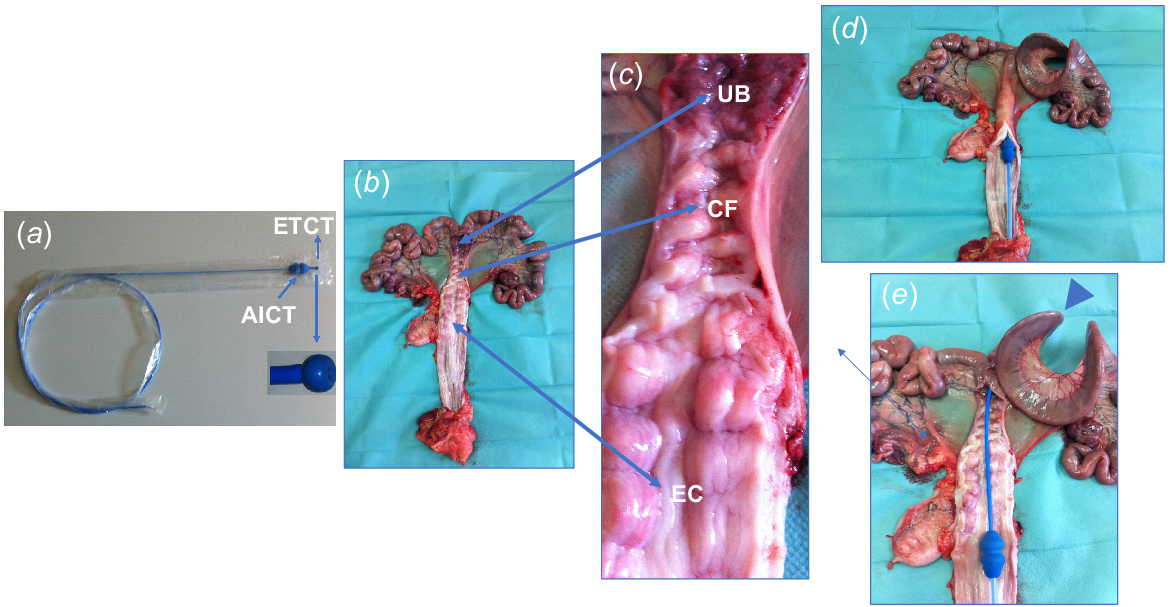
Accuracy of NsDU catheter placement
Successful NsDU-ET relies on the efficient insertion of the catheter and ensuring proper placement within the pig’s uterus. This catheter effectively passes through the cervical canal and reaches deep into a uterine horn in a high proportion of gilts and sows. Notably, it achieves this feat without causing any cervical or uterine perforations (Martinez et al. 2004).
One important factor in nonsurgical ET programs is the type of recipient. The number of previous estrous cycles in gilts is crucial for successful insertion, with higher success rates in gilts with more than two previous estrous cycles (Cuello et al. 2005). Weaning sows, especially sows with a greater number of previous parities, typically exhibit easier passage of the catheter through the cervix and into the uterus. Catheter insertion presented minimal difficulties in a high proportion of both gilts and sows. Notably, the procedure typically took less than 3 min, with no observable difference in time between gilts and sows (Martinez et al. 2013; Martinez et al. 2016a).
Correct catheter placement inside the uterine horn is essential for successful NsDU-ET in pigs. Previous studies demonstrated that it was possible to correctly insert a flexible catheter and reach the second and third quarters of the uterine horn in 90% of recipients (Martinez et al. 2004; Cuello et al. 2005). Furthermore, the ability to accurately identify potential catheter misplacement minimizes the number of wasted embryos and improves pregnancy rates.
Several other key factors influence the effectiveness and potential of the NsDU procedure, including operator training requirements and animal welfare considerations (Martinez et al. 2013). While the NsDU-ET procedure appears simple, operator training is needed. This training promotes the development of essential skills and ultimately leads to improved farrowing rates and faster insertion times. On the other hand, more than 90% of females exhibited good or moderate behavior throughout the procedure, with gilts showing slightly less tolerance than sows. This result suggests minimal discomfort and good overall acceptance of the NsDU-ET process. Furthermore, the absence of bleeding or trauma during and after insertion indicates a low risk of injury to the reproductive tract.
Factors influencing the efficiency of ET
Donors and recipients
Multiple factors associated with the donors and recipients impact the success rates of NsDU-ET. Traditionally, donor selection has focused on health, breeding history, and genetic merit. In addition, other factors such as parity, the weaning-to-estrus interval, and season do not significantly affect the number or quality of embryos collected (Nohalez et al. 2017). These findings indicate that a wider range of sows may be used as donors for longer periods. Recipient selection is another crucial but often overlooked factor. While health and body condition are typically considered, other aspects can impact success. Age and parity can influence reproductive outcomes post-AI, with multiparous sows generally performing better than younger animals (Clark et al. 1989). This aligns with another study that reported easier catheter insertion, better recipient behavior, and natural estrus synchronization in multiparous sows (Martinez et al. 2013). Additionally, other study revealed no significant effect of recipient parity on NsDU-ET efficiency or reproductive performance (Martinez et al. 2016b). This flexibility in recipient selection simplifies commercial ET programs by allowing the use of a wider range of sows that are readily available on farms. Overall, these findings suggest that NsDU-ET programs may benefit from a broader approach to donor and recipient selection to potentially improve program efficiency and accessibility.
Estrus synchronization and superovulation
Traditionally, NsDU-ET programs require twice as many embryo donors as recipients because of the limited number of oocytes released during ovulation (Martinez et al. 2013). To increase the number of embryos per donor and consequently reduce the donor:recipient ratio, the use of superovulation techniques has been proposed. In multiparous weaned sows, a specific hormone regimen (eCG and hCG) effectively increases the number of transferable embryos without impairing embryo quality or causing unwanted side effects, such as ovarian cysts. Additionally, the farrowing rates and litter sizes after NsDU-ET were similar for embryos from superovulated and non-superovulated donors (Angel et al. 2014a). These findings indicate that superovulation decreases the donor-to-recipient ratio from 2:1 to 1.5–1:1, which particularly benefits genetic companies by maximizing the number of embryos available from each donor.
However, the demand for donor sows in ET programs sometimes exceeds the number available from a single weaning. To address this shortage, producers may need to combine sows from multiple weaning to create a sufficient batch of donors for ET. It is known that short-term administration of the synthetic progestogen altrenogest delays the onset of estrus after weaning (Kraeling and Webel 2015). This estrus synchronization method holds promise for ET programs because it could extend the window for collecting donor sows from several weaning periods by 1 or 2 weeks. However, combining this short-term synchronization treatment with superovulation requires caution. Several studies indicated that combining these methods disrupts gene expression patterns in the ovaries and endometrium (Gonzalez-Ramiro et al. 2022). These disruptions manifest as abnormalities in follicle and oocyte development, fertilization, and embryo development. Therefore, a greater number of immature oocytes and degenerated embryos are observed, which lead to a significant decrease in the overall efficiency of embryo production (Gonzalez-Ramiro et al. 2021).
Estrus asynchrony between donor and recipient
Early research on surgical ET suggested higher pregnancy rates when recipients were in estrus after the donors (Polge 1982; Blum-Reckow and Holtz 1991). However, studies on nonsurgical methods into the UB have produced conflicting results (Hazeleger et al. 2000b). To clarify this apparent contradiction, a large study involving more than 130 NsDU-ETs was performed (Angel et al. 2014b). The findings revealed significantly lower farrowing rates when recipients were in estrus before the donors, compared to recipients in estrus 24 h after. These results are consistent with observations from surgical ET studies. The exact reason for the discrepancy between surgical methods and some nonsurgical methods is not clear. This difference may be related to the different locations of embryo deposition within the uterus in each procedure.
Reproductive performance of females in the cycle after embryo transfer
The obtaining of pig embryos traditionally involves surgery on the donor. We compared the impact of surgical embryo collection and the NsDU-ET method on the future reproduction of pig donors and recipients, with both groups receiving AI in the cycle after ET (Martinez et al. 2017). Surgical embryo collection did not significantly impact the likelihood of a successful farrowing rate for the donor sows. However, the number of piglets born decreased by two. This result suggests a need for improvements in surgical techniques to minimize negative effects on donors. However, the NsDU-ET procedure had no negative impact on the subsequent reproductive performance of the recipients. This finding highlights the safety and potential benefits of NsDU-ET technology for ET programs.
Experimental results obtained under field conditions using nonsurgical deep uterine embryo transfer
In addition to the previously discussed factors, several other factors can influence NsDU-ET outcomes. Among these factors, the state of the embryos for transfer is notable, whether fresh (recently collected), stored in a liquid state for up to 24 h, or vitrified.
While information on the surgical transfer of fresh embryos is scarce, existing studies report that farrowing rates and litter sizes are ~60–80% with 7–8 piglets born, respectively (Cameron et al. 1989; Galvin et al. 1994). Compared to surgical procedures, the nonsurgical transfer of embryos directly into the UB results in lower success rates. Farrowing rates using this method typically range from 33–40%, with an average litter size of 6.7–7.4 piglets (Hazeleger and Kemp 1994; Ducro-Steverink et al. 2004). Similarly, early attempts using the NsDU-ET technique achieved a 70% farrowing rate and an average litter size of 7 piglets (Martinez et al. 2004). Further improvements in the NsDU-ET procedure, including aseptic protocols, synchronized estrous cycles between donors and recipients, and proper operator training (as detailed in Martinez et al. 2013), significantly improved its effectiveness. Currently, NsDU-ET achieves farrowing rates of ~80% and a litter size of ~9.5 piglets per litter, which makes it the most successful nonsurgical ET method for pigs (Angel et al. 2014a, 2014b; Martinez et al. 2014).
The use of embryos stored for short durations (24–30 h) can impact the success of ET procedures. Surgical ET with embryos transported internationally (in vitro culture for 24–30 h) resulted in acceptable farrowing rates (50–60%) and litter sizes (5–8 piglets) (James et al. 1980; Niemann et al. 1989). However, more recently, NsDU-ET using fresh morulae cultured for 24 h achieved significantly better outcomes (90% farrowing rates with 9.0 piglets per litter) (Martinez et al. 2014). Several factors may contribute to the observed variations in success rates, including the number of embryos transferred, differences in culture media used, the developmental stage of the embryos at transfer, recipient characteristics, and the specific facilities and practices used on individual farms, particularly embryo handling and transfer procedures.
Recent advancements in vitrification protocols have significantly improved the post-warming survival rates of untreated morulae and blastocysts. Promising results have been reported in farrowing rates and litter sizes following the surgical transfer of vitrified embryos, but the number of studies is limited due to the need for large numbers of embryos at specific times (Martinez et al. 2013). The use of NsDU-ET and embryo vitrification is important for the broader application of porcine ET. Preliminary experiments in our laboratory combining these technologies yielded acceptable farrowing rates (40–50%) and litter sizes (5–10 piglets) (Cuello et al. 2005; Gomis et al. 2012), albeit with a low number of recipients. Later, we performed a separate study comparing surgical and NsDU-ET procedures for vitrified embryos under farm conditions in 109 sows. Our focus was to evaluate how the number of nonsurgically transferred vitrified embryos impacted the reproductive performance of recipients (Martinez et al. 2015). NsDU-ET with 30 vitrified–warmed embryos resulted in significantly lower farrowing rates and litter sizes compared to surgical and NsDU-ET with 30 and 40 vitrified embryos, respectively. We concluded that NsDU-ET achieved similar success rates to surgical ET when the appropriate number of embryos per transfer was used. The greater number of vitrified embryos needed for NsDU-ET than for surgical ET is likely due to differences in embryo deposition sites within the uterus. In surgical ET, embryos are deposited into the tip of a uterine horn (Polge 1982), whereas they are placed deeper in the uterine horn in NsDU-ET. Physiologically, pig embryos remain near the horn tip until days 6 or 7. Therefore, transferring morulae and blastocysts to the horn tip may be more beneficial. It is likely that the uterine environment in more caudal locations is less favorable for embryo development, which reduces survival rates.
From research to commercial farming applications
Several commercial programs for NsDU-ET have been implemented using embryos preserved for 24 h in a liquid state or using vitrified embryos. Despite the limited number of transfers, some observations can be made about the effectiveness of the procedure. In these programs, the embryos exhibited excellent or very good morphological quality just before transfer. However, compared to the results achieved under experimental conditions, reproductive performance varied significantly between farms. The farrowing rates ranged from 30.0 to 83.3%, and the litter sizes varied from 4.2 to 11.2 piglets. Notably, some farms experienced no pregnancy losses after transfers, whereas others reported losses beyond day 25 (Table 1). Additionally, piglet production efficiency exhibited significant variability. When the ratio of live-born piglets to the total number of embryos transferred was calculated, the efficiency ranged from 5.6 to 31.7%. When calculated using only farrowed recipients, the efficiency ranged from 13.5 to 38.5%. These results show much greater variation than the data obtained under experimental conditions (18.7 to 28.8% and 26.5 to 32.0%, respectively) (Fig. 2).
Commercial application | Embryo stage and handling | Recipients (N) | Transferred embryos (N) | Pregnancy N (%) | Farrowing N (%) | Live piglets (stillbirth) | Total piglet Mean ± s.d. | Survival | ||
---|---|---|---|---|---|---|---|---|---|---|
FarrowA | TotalB | |||||||||
Contingency plan for infectious disease | Postmortem morulae and blastocysts transported in liquid state for 3–4 h | 20 | 600 | 11 (55) | 6 (30.0) | 57 (0) | 9.5 ± 2.1 | 31.7 | 9.5 | |
Introduction of a new breeding program in a high-health status farm | In vivo-derived fresh morulae and blastocysts transported in liquid state for 3–4 h | 50 | 1535 | 34 (68) | 29 (58.0) | 212 (13) | 7.8 ± 2.7 | 24.4 | 13.8 | |
Commercial movement of genetic material | In vivo-derived fresh morulae and blastocysts transported in liquid state for 24 h | 16 | 525 | 9 (56.3) | 6 (37.5) | 57 (1) | 9.7 ± 3.6 | 31.7 | 9.2 | |
3 | 92 | 2 (66.7) | 2 (66.7) | 18 (0) | 9.0 ± 1.4 | 30.0 | 20.0 | |||
4 | 124 | 3 (75.0) | 3 (75.0) | 24 (1) | 8.3 ± 2.1 | 27.8 | 20.8 | |||
12 | 376 | 8 (66.7) | 5 (41.7) | 21 (4.2) | 4.2 ± 1.9 | 13.5 | 5.6 | |||
40 | 1149 | 26 (65.0) | 20 (50.0) | 137 (19) | 7.8 ± 2.9 | 23.6 | 11.9 | |||
6 | 164 | 5 (83.3) | 5 (83.3) | 52 (4) | 11.2 ± 1.9 | 38.5 | 31.7 | |||
7 | 195 | 6 (85.7) | 4 (57.1) | 34 (7) | 10.3 ± 4.3 | 30.6 | 17.4 | |||
Vitrified–warmed in vivo-derived blastocysts | 3 | 120 | 3 (100%) | 2 (66.7%) | 13 (0) | 6.5 ± 2.1 | 16.3 | 10.8 |
Box-and-whisker plots with individual data points overlaid illustrate the efficiency of piglet production following nonsurgical deep uterine embryo transfer under experimental conditions (published literature: Martinez et al. 2016a, 2019) and commercial situations (EA Martinez, J Sanchez-Osorio, I Parrilla, C Cuello, MA Gil, unpubl. data). Piglet production efficiency is defined as the ratio of live-born piglets to the total number of transferred embryos, considering only farrowed or transferred recipients. The boxes represent the interquartile range (25th to 75th percentiles), the solid line indicates the median, the dashed line marks the mean, and the whiskers show the maximum and minimum values.
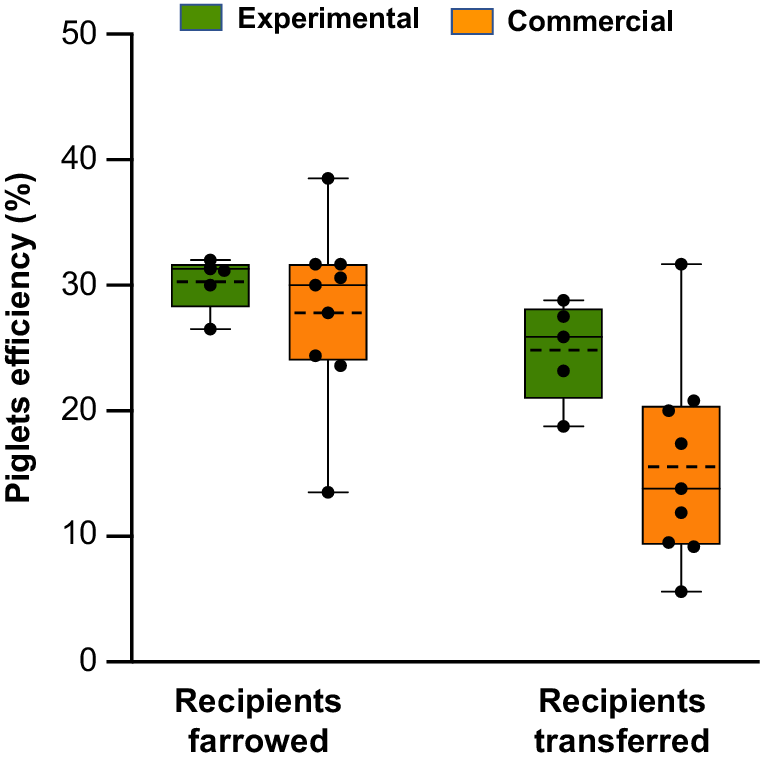
This variability can arise from a complex interplay of factors, such as the recipient’s genetics, sanitary standards, methods of detecting estrus, and management after transfer. However, the recipient’s uterine environment is likely one of the most influential factors. Studies have established a clear link between hygiene conditions, the estrous cycle stage, and the ability of the uterus to resist infections because gilts inoculated with bacteria during estrus exhibited much higher resistance to endometritis than gilts inoculated during diestrus (de Winter et al. 1994). These findings suggest that elevated estrogen levels during estrus offer a protective benefit. In contrast, the period following estrus, which is dominated by progesterone, increases the likelihood of infection (de Winter et al. 1992; Wulster-Radcliffe et al. 2003). Therefore, recipients are particularly vulnerable to uterine infections during and shortly after NsDU-ET (approximately days 5–6 of the cycle), which makes rigorous aseptic measures essential to prevent infections. The absence of these precautions may account for the pregnancy losses observed in some commercial farms following NsDU-ET. In fact, many sows that were initially confirmed to be pregnant via ultrasound but subsequently lost their pregnancies experienced vaginal discharge or symptoms of endometritis. In contrast to commercial NsDU-ET programs that housed recipients in their standard stalls without specific hygiene protocols, our experimental studies, although performed under field conditions, involved performing ETs in separate, meticulously maintained, and clean rooms where the recipients were kept until day 28 of pregnancy. This approach effectively prevented uterine infections and pregnancy losses (Fig. 3). Therefore, ensuring good health through biosecurity measures, proper facilities, effective management practices, accurate estrus detection, and stringent hygiene protocols is crucial for achieving optimal fertility and prolificacy after NsDU-ET. However, further research is necessary to identify and address the key factors that affect the commercial viability of this technology.
Box-and-whisker plots with individual data points overlaid illustrate the pregnancy and farrow rates, as well as litter sizes, following nonsurgical deep uterine embryo transfer under experimental (published literature: Martinez et al. 2016a, 2019) and commercial (EA Martinez, J Sanchez-Osorio, I Parrilla, C Cuello, MA Gil, unpubl. data) conditions. The boxes represent the interquartile range (25th to 75th percentiles), the solid line indicates the median, the dashed line marks the mean, and the whiskers show the maximum and minimum values.
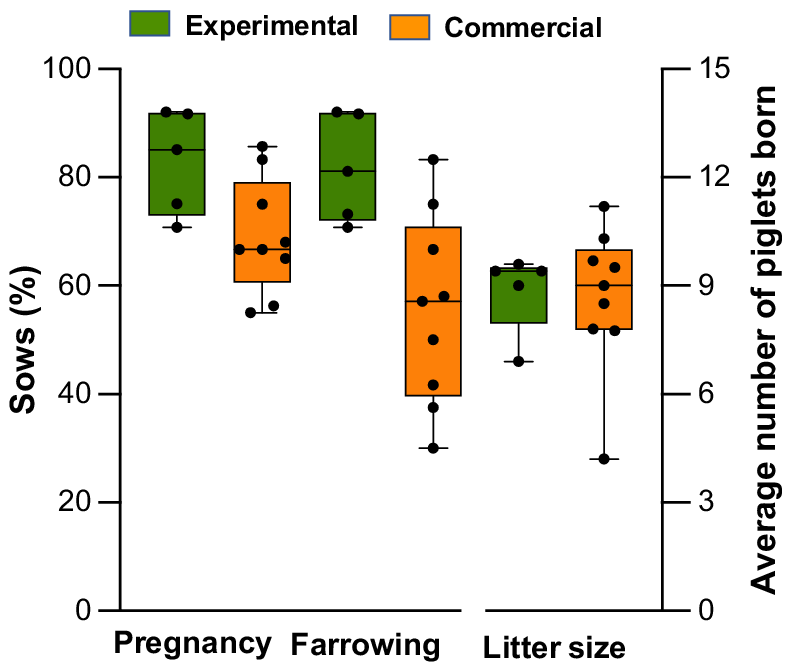
Upcoming directions
Despite significant advancements in porcine ET technology, several challenges remain. The primary obstacle to widespread adoption is the embryo collection method because in vivo-derived embryos require slaughtering the donors or performing surgery, which limit donor efficiency. The development of nonsurgical methods for embryo collection would allow repeated use of the same donor, which would greatly enhance efficiency. However, because of the complex anatomy of the sow’s reproductive tract, nonsurgical collection has not been successfully implemented. Researchers are currently revisiting methods used 30 years ago with limited success, including techniques involving surgically shunted uterine horns, but progress is still needed.
Another alternative to surgical embryo collection in NsDU-ET programs is the use of in vitro-produced (IVP) embryos. Many immature oocytes can be retrieved from slaughtered animals then matured and fertilized under controlled conditions. While cost-effective and time-saving, current IVP systems in pigs face challenges because of suboptimal culture conditions that lead to a variety of issues, such as polyspermy and poor embryo development (Gil et al. 2010). A successful and efficient IVP program, such as programs in cattle (Dyck et al. 2014), could enable the global exchange of valuable genetic material and accelerate breeding programs. However, a limitation of current IVP methods is the use of immature oocytes collected from slaughtered prepubertal gilts. These oocytes have low genetic value, and their use results in minimal impact on genetic progress. A promising approach to overcome that limitation is the use of Ovum Pick-Up (OPU), which is a well-established technology for retrieving oocytes from live animals with high genetic value that is commonly used in cows and mares. Although OPU in pigs is less documented due to anatomical challenges (Okuyama et al. 2017), advancements, such as sedation and epidural anesthesia during transvaginal OPU (Yoshioka et al. 2020; Oltedal et al. 2024a), address animal welfare concerns and make the procedure easier to perform. These refinements yield an average of over nine oocytes per session, with no reported difference in subsequent fertility or prolificacy compared to control sows (Oltedal et al. 2024b). These findings suggest the viability of OPU for oocyte retrieval in live sows and pave the way for the use of genetically superior oocytes in IVP protocols. This use could significantly accelerate genetic improvement programs in swine breeding through NsDU-ET technology.
Another major challenge facing current ET programs is post-transfer embryonic loss. The reproductive efficiency of sows is an economic factor that directly impacts the productivity of the swine industry. Numerous physiological and environmental factors affect fertility and prolificacy. Among these factors, embryonic and fetal survival play unprecedented roles (Spötter and Distl 2006) because prenatal mortality is the main determinant of litter size (Pope 1994; Spötter and Distl 2006), and therefore, it is one of the main concerns of pig companies. Under physiological conditions, ~20–30% of pig embryos produced after fertilization die before, during, or shortly after implantation, and another 10–15% die at mid-pregnancy (Vallet et al. 2011). Therefore, almost half of the embryos derived from natural breeding or AI do not progress to full term (Pope 1994). These data are even more pronounced after ET. The fertility and prolificacy results available in the literature after surgical or nonsurgical ET range between 50% and 80% with 6 to 10 piglets born per litter, respectively (Martinez et al. 2016a, Martinez et al. 2019). That is, fertility values are 10 to 30 points lower and litter sizes of 4 to 6 piglets lower than the values obtained by AI (Roca et al. 2016). Furthermore, the efficiency of piglet production after ET is ~30% (Angel et al. 2014b; Martinez et al. 2014), which means that 70% of the embryos used in each ET die in the uterus of the recipient. Several studies attempted to understand some of the causes of embryonic losses, including nutritional, genetic, environmental, and placental factors (Ford 1997; Wilson et al. 1998; Miles et al. 2008; Foxcroft et al. 2009). Other factors, such as immunity (Croy et al. 2009) and inadequate maternal–embryo communication (Wolf et al. 2003), also play fundamental roles in embryonic loss and pregnancy failure. Embryos produced under natural conditions (including AI) are hemiallogeneic to the mother because they contain genetic material from the father that generates unfamiliar proteins for the mother’s immune system. However, embryos are usually not rejected by the mother during pregnancy without affecting the preservation of effective immunity against pathogens because the immune system of the mother is controlled (or regulated locally) to favor embryo implantation (Parrilla et al. 2022). The regulation of the maternal immune response to the embryo may be more complicated and inefficient in pregnancies produced by ET because the embryos in these cases are allogeneic (they contain paternal and maternal genetic material unknown to the recipient female), which would reduce the developmental possibilities of many embryos. This supposition is supported by previous results obtained in our laboratory on cytokine levels and gene expression. We showed that after the transfer of allogeneic embryos, pig pregnancies show clear dysregulation of pro- and anti-inflammatory cytokine levels (Martinez et al. 2020), a lack of sufficient endometrial levels of leukemia inhibitory factor (Cambra et al. 2020), and severe downregulation of interferon-stimulated genes in the peri-implantation endometrium (Martinez et al. 2022), all of which could be associated with impaired maternal immune tolerance and could partially explain the high embryonic mortality associated with ET programs. Understanding the molecular omics interactions between mothers and embryos during the peri-implantation period could lead to strategies that improve embryo–maternal communication, increase gestation rates and reduce embryonic loss.
Another challenge is the cryopreservation procedure. Vitrification is currently the only efficient method for cryopreserving pig embryos (Martinez et al. 2019; Xingzhu et al. 2021). The most common technique is superfine open pulled straw (SOPS), which yields good embryo survival rates in vitro (Cuello et al. 2007, 2008; Sanchez-Osorio et al. 2008) and successful pregnancies after surgical and nonsurgical ET (Cuello et al. 2005, 2016; Martinez et al. 2015). However, SOPS has a significant practical limitation: only four to six embryos can be vitrified at a time per device without compromising the necessary volume for optimal preservation. This limitation is impractical for ET, which typically requires 20–40 embryos per recipient (Cuello et al. 2005, 2016; Martinez et al. 2015). To address this issue, the open Cryotop system has been introduced, which allows for the simultaneous vitrification of up to 20 embryos (Gonzalez-Plaza et al. 2022). This technique provides a significant procedural advantage by simplifying the vitrification, warming, and transfer process in species such as pigs. This method yields similar embryo survival rates and quality markers to fresh embryos and even outperforms the SOPS method (Gonzalez-Plaza et al. 2022). Additionally, it minimally affects embryo gene expression (Gonzalez-Plaza et al. 2023). However, further research is needed to understand the impact of Cryotop vitrification on embryo development after ET.
Data availability
Data sharing is not applicable as no new data were generated or analyzed during this study.
Declaration of funding
Some of the studies presented in this review were supported by the R&D&I project PID2022137645OB-I00 from of MCIU/AEI/10.13039/501100011033/FEDER/UE, Madrid, Spain.
Author contributions
M.A.G. and E.A.M. conceived the review content and wrote the first draft of the manuscript and edited the manuscript. All authors provided critical feedback and approved the manuscript.
Acknowledgements
The authors are grateful to Prof. Billy N. Day for his constant help and invaluable advice over the years. We thank J. Sanchez-Osorio and A. Muñoz for their assistance and to the staff of Agropor SA (Murcia, Spain), Porcisan (Murcia, Spain), Selección Batalle SA (Gerona, Spain), and Topigs Norsvin España (Madrid, Spain) for the excellent management of the donors and recipients in the studies reported here.
References
Aigner B, Renner S, Kessler B, Klymiuk N, Kurome M, Wünsch A, Wolf E (2010) Transgenic pigs as models for translational biomedical research. Journal of Molecular Medicine 88, 653-664.
| Crossref | Google Scholar | PubMed |
Angel MA, Gil MA, Cuello C, Sanchez-Osorio J, Gomis J, Parrilla I, Vila J, Colina I, Diaz M, Reixach J, Vazquez JL, Vazquez JM, Roca J, Martinez EA (2014a) The effects of superovulation of donor sows on ovarian response and embryo development after nonsurgical deep-uterine embryo transfer. Theriogenology 81, 832-839.
| Crossref | Google Scholar | PubMed |
Angel MA, Gil MA, Cuello C, Sanchez-Osorio J, Gomis J, Parrilla I, Vila J, Colina I, Diaz M, Reixach J, Vazquez JLJ, Vazquez JM, Roca J, Martinez EA (2014b) An earlier uterine environment favors the in vivo development of fresh pig morulae and blastocysts transferred by a nonsurgical deep-uterine method. Journal of Reproduction and Development 60, 371-376.
| Crossref | Google Scholar | PubMed |
Blum-Reckow B, Holtz W (1991) Transfer of porcine embryos after 3 days of in vitro culture. Journal of Animal Science 69, 3335-3342.
| Crossref | Google Scholar | PubMed |
Cambra JM, Jauregi-Miguel A, Alvarez-Rodriguez M, Parrilla I, Gil MA, Martinez EA, Cuello C, Rodriguez-Martinez H, Martinez CA (2020) Allogeneic embryos disregulate leukemia inhibitory factor (LIF) and its receptor in the porcine endometrium during implantation. Frontiers in Veterinary Science 7, 611598.
| Crossref | Google Scholar | PubMed |
Cameron RD, Durack M, Fogarty R, Putra DK, McVeigh J (1989) Practical experience with commercial embryo transfer in pigs. Australian Veterinary Journal 66, 314-318.
| Crossref | Google Scholar | PubMed |
Clark LK, Schinckel AP, Singleton WL, Einstein ME, Teclaw RF (1989) Use of farrowing rate as a measure of fertility of boars. Journal of the American Veterinary Medical Association 194, 239-243.
| Google Scholar | PubMed |
Croy BA, Wessels J, Linton N, Tayade C (2009) Comparison of immune cell recruitment and function in endometrium during development of epitheliochorial (pig) and hemochorial (mouse and human) placentas. Placenta 30, 26-31.
| Crossref | Google Scholar |
Cuello C, Berthelot F, Martinat-Botté F, Venturi E, Guillouet P, Vázquez JM, Roca J, Martínez EA (2005) Piglets born after non-surgical deep intrauterine transfer of vitrified blastocysts in gilts. Animal Reproduction Science 85, 275-286.
| Crossref | Google Scholar | PubMed |
Cuello C, Gil MA, Almiñana C, Sanchez-Osorio J, Parrilla I, Caballero I, Vazquez JM, Roca J, Rodriguez-Martinez H, Martinez EA (2007) Vitrification of in vitro cultured porcine two-to-four cell embryos. Theriogenology 68, 258-264.
| Crossref | Google Scholar | PubMed |
Cuello C, Sanchez-Osorio J, Almiñana C, Gil MA, Perals ML, Lucas X, Roca J, Vazquez JM, Martinez EA (2008) Effect of the cryoprotectant concentration on the in vitro embryo development and cell proliferation of OPS-vitrified porcine blastocysts. Cryobiology 56, 189-194.
| Crossref | Google Scholar | PubMed |
Cuello C, Martinez CA, Nohalez A, Parrilla I, Roca J, Gil MA, Martinez EA (2016) Effective vitrification and warming of porcine embryos using a pH-stable, chemically defined medium. Scientific Reports 6, 33915.
| Crossref | Google Scholar | PubMed |
de Winter PJJ, Verdonck M, de Kruif A, Devriese LA, Haesebrouck F (1992) Endometritis and vaginal discharge in the sow. Animal Reproduction Science 28, 51-58.
| Crossref | Google Scholar |
de Winter PJJ, Verdonck M, de Kruif A, Devriese LA, Haesebrouck F (1994) Influence of the oestrous cycle on experimental intrauterine E. coli infection in the sow. Journal of Veterinary Medicine Series A 41, 640-644.
| Crossref | Google Scholar | PubMed |
Ducro-Steverink DWB, Peters CGW, Maters CC, Hazeleger W, Merks JWM (2004) Reproduction results and offspring performance after non-surgical embryo transfer in pigs. Theriogenology 62, 522-531.
| Crossref | Google Scholar | PubMed |
Dyck MK, Zhou C, Tsoi S, Grant J, Dixon WT, Foxcroft GR (2014) Reproductive technologies and the porcine embryonic transcriptome. Animal Reproduction Science 149, 11-18.
| Crossref | Google Scholar | PubMed |
Ford SP (1997) Embryonic and fetal development in different genotypes in pigs. Journal of Reproduction and Fertility. Supplements 52, 165-176.
| Google Scholar | PubMed |
Foxcroft GR, Dixon WT, Dyck MK, Novak S, Harding JCS, Almeida FCRL (2009) Prenatal programming of postnatal development in the pig. Society for Reproduction and Fertility. Supplements 66, 213-231.
| Google Scholar | PubMed |
Galvin JM, Killian DB, Stewart AN (1994) A procedure for successful nonsurgical embryo transfer in swine. Theriogenology 41, 1279-1289.
| Crossref | Google Scholar | PubMed |
Gil MA, Cuello C, Parrilla I, Vazquez JM, Roca J, Martinez EA (2010) Advances in swine in vitro embryo production technologies. Reproduction in Domestic Animals 45, 40-48.
| Crossref | Google Scholar | PubMed |
Gomis J, Cuello C, Sanchez-Osorio J, Gil MA, Parrilla I, Angel MA, Maside C, del Olmo D, Vazquez JM, Roca J, Martinez EA (2012) Non-surgical deep intrauterine transfer of superfine open pulled straw (SOPS)-vitrified porcine embryos: Evaluation of critical steps of the procedure. Theriogenology 78, 1339-1349.
| Crossref | Google Scholar | PubMed |
Gonzalez-Plaza A, Cambra JM, Parrilla I, Gil MA, Martinez EA, Martinez CA, Cuello C (2022) The open cryotop system is effective for the simultaneous vitrification of a large number of porcine embryos at different developmental stages. Frontiers in Veterinary Science 9, 936753.
| Crossref | Google Scholar | PubMed |
Gonzalez-Plaza A, Cambra JM, Garcia-Canovas M, Parrilla I, Gil MA, Martinez EA, Rodriguez-Martinez H, Martinez CA, Cuello C (2023) Cryotop vitrification of large batches of pig embryos simultaneously provides excellent postwarming survival rates and minimal interference with gene expression. Theriogenology 206, 1-10.
| Crossref | Google Scholar | PubMed |
Gonzalez-Ramiro H, Cuello C, Cambra JM, Gonzalez-Plaza A, Vazquez JM, Vazquez JL, Rodriguez-Martinez H, Gil MA, Lucas-Sanchez A, Parrilla I, Martinez EA (2021) A short-term altrenogest treatment post-weaning followed by superovulation reduces pregnancy rates and embryo production efficiency in multiparous sows. Frontiers in Veterinary Science 8, 771573.
| Crossref | Google Scholar | PubMed |
Gonzalez-Ramiro H, Parrilla I, Cambra JM, Gonzalez-Plaza A, Gil MA, Cuello C, Martinez EA, Rodriguez-Martinez H, Martinez CA (2022) Combined synchronization and superovulation treatments negatively impact embryo viability possibly by the downregulation of WNT/β-catenin and Notch signaling genes in the porcine endometrium. Journal of Animal Science 100(11), skac315.
| Crossref | Google Scholar |
Hazeleger W, Kemp B (1994) Farrowing rate and litter size after transcervical embryo transfer in sows. Reproduction in Domestic Animals 29, 481-487.
| Crossref | Google Scholar |
Hazeleger W, Bouwman EG, Noordhuizen JP, Kemp B (2000a) Effect of superovulation induction on embryonic development on day 5 and subsequent development and survival after nonsurgical embryo transfer in pigs. Theriogenology 53, 1063-1070.
| Crossref | Google Scholar | PubMed |
Hazeleger W, Noordhuizen JPTM, Kemp B (2000b) Effect of asynchronous non-surgical transfer of porcine embryos on pregnancy rate and embryonic survival. Livestock Production Science 64, 281-284.
| Crossref | Google Scholar |
Hirayama Y, Takishita R, Misawa H, Kikuchi K, Misumi K, Egawa S, Motoyama S, Hasuta Y, Nakamura Y, Hashiyada Y (2020) Non-surgical transfer of vitrified porcine embryos using a catheter designed for a proximal site of the uterus. Animal Science Journal 91, e13457.
| Crossref | Google Scholar | PubMed |
Hryhorowicz M, Lipiński D, Hryhorowicz S, Nowak-Terpiłowska A, Ryczek N, Zeyland J (2020) Application of genetically engineered pigs in biomedical research. Genes 11, 670.
| Crossref | Google Scholar |
James JE, Reeser PD, Davis DL, Straiton EC, Talbot AC, Polge C (1980) Culture and long-distance shipment of swine embryos 1. Theriogenology 14, 463-469.
| Crossref | Google Scholar |
Kraeling RR, Webel SK (2015) Current strategies for reproductive management of gilts and sows in North America. Journal of Animal Science and Biotechnology 6, 3.
| Crossref | Google Scholar | PubMed |
Kvasnickii AV (2001) Research on interbreed ova transfer in pigs. Theriogenology 56, 1285-1289.
| Crossref | Google Scholar |
Li J, Rieke A, Day BN, Prather RS (1996) Technical note: porcine non-surgical embryo transfer. Journal of Animal Science 74, 2263-2268.
| Crossref | Google Scholar | PubMed |
Martinez EA, Vazquez JM, Roca J, Lucas X, Gil MA, Parrilla I, Vazquez JL, Day BN (2001) Successful non-surgical deep intrauterine insemination with small numbers of spermatozoa in sows. Reproduction 122, 289-296.
| Crossref | Google Scholar | PubMed |
Martinez EA, Caamaño JN, Gil MA, Rieke A, McCauley TC, Cantley TC, Vazquez JM, Roca J, Vazquez JL, Didion BA, Murphy CN, Prather RS, Day BN (2004) Successful nonsurgical deep uterine embryo transfer in pigs. Theriogenology 61, 137-146.
| Crossref | Google Scholar | PubMed |
Martinez EA, Gil MA, Cuello C, Sanchez-Osorio J, Gomis J, Parrilla I, Angel MA, Rodriguez-Martinez H, Lucas X, Vazquez JL, Vazquez JM, Roca J (2013) Current progress in non-surgical embryo transfer with fresh and vitrified/warmed pig embryos. Control of Pig Reproduction IX 101-112.
| Crossref | Google Scholar |
Martinez EA, Angel MA, Cuello C, Sanchez-Osorio J, Gomis J, Parrilla I, Vila J, Colina I, Diaz M, Reixach J, Vazquez JL, Vazquez JM, Roca J, Gil MA (2014) Successful non-surgical deep uterine transfer of porcine morulae after 24 hour culture in a chemically defined medium. PLoS ONE 9, e104696.
| Crossref | Google Scholar |
Martinez EA, Martinez CA, Nohalez A, Sanchez-Osorio J, Vazquez JM, Roca J, Parrilla I, Gil MA, Cuello C (2015) Nonsurgical deep uterine transfer of vitrified, in vivo-derived, porcine embryos is as effective as the default surgical approach. Scientific Reports 5, 1-9.
| Crossref | Google Scholar |
Martinez EA, Cuello C, Parrilla I, Martinez CA, Nohalez A, Vazquez JL, Vazquez JM, Roca J, Gil MA (2016a) Recent advances toward the practical application of embryo transfer in pigs. Theriogenology 85, 152-161.
| Crossref | Google Scholar | PubMed |
Martinez EA, Nohalez A, Martinez CA, Parrilla I, Vila J, Colina I, Diaz M, Reixach J, Vazquez JL, Roca J, Cuello C, Gil MA (2016b) The recipients’ parity does not influence their reproductive performance following non-surgical deep uterine porcine embryo transfer. Reproduction in Domestic Animals 51, 123-129.
| Crossref | Google Scholar | PubMed |
Martinez CA, Nohalez A, Parrilla I, Vazquez JL, Roca J, Cuello C, Rodriguez-Martinez H, Martinez EA, Gil MA (2017) Surgical embryo collection but not nonsurgical embryo transfer compromises postintervention prolificacy in sows. Theriogenology 87, 316-320.
| Crossref | Google Scholar | PubMed |
Martinez EA, Martinez CA, Cambra JM, Maside C, Lucas X, Vazquez JL, Vazquez JM, Roca J, Rodriguez-Martinez H, Gil MA, Parrilla I, Cuello C (2019) Achievements and future perspectives of embryo transfer technology in pigs. Reproduction in Domestic Animals 54, 4-13.
| Crossref | Google Scholar |
Martinez CA, Ruber M, Rodriguez-Martinez H, Alvarez-Rodriguez M (2020) Pig pregnancies after transfer of allogeneic embryos show a dysregulated endometrial/placental cytokine balance: a novel clue for embryo death? Biomolecules 10, 554.
| Crossref | Google Scholar |
Martinez CA, Alvarez-Rodriguez M, Rodriguez-Martinez H (2022) A decreased expression of interferon stimulated genes in peri-implantation endometrium of embryo transfer recipient sows could contribute to embryo death. Animal 16, 100590.
| Crossref | Google Scholar | PubMed |
Miles JR, Freking BA, Blomberg LA, Vallet JL, Zuelke KA (2008) Conceptus development during blastocyst elongation in lines of pigs selected for increased uterine capacity or ovulation rate. Journal of Animal Science 86, 2126-2134.
| Crossref | Google Scholar | PubMed |
Nakazawa Y, Misawa H, Fujino Y, Tajima S, Misumi K, Ueda J, Nakamura Y, Shibata T, Hirayama Y, Kikuchi K (2008) Effect of volume of non-surgical embryo transfer medium on ability of porcine embryos to survive to term. Journal of Reproduction and Development 54, 30-34.
| Crossref | Google Scholar | PubMed |
Niemann H, Wüst A, Gardon JC (1989) Successful intercontinental transport of porcine embryos from Europe to South America. Theriogenology 31, 525-530.
| Crossref | Google Scholar | PubMed |
Nohalez A, Martinez CA, Reixach J, Diaz M, Vila J, Colina I, Parrilla I, Vazquez JL, Roca J, Gil MA, Rodriguez-Martinez H, Martinez EA, Cuello C (2017) Factors of importance when selecting sows as embryo donors. Animal 11, 1330-1335.
| Crossref | Google Scholar | PubMed |
Okuyama MW, Sugiura T, Moriyoshi M, Yamashita K, Tamura J, Katagiri S (2017) A transvaginal endoscopy-based technique for performing ovarian examinations in sows. Journal of Reproduction and Development 63, 617-622.
| Crossref | Google Scholar | PubMed |
Oltedal A, Björkman S, Peltoniemi O, Gaustad AH, Oropeza-Moe M (2024a) Transvaginal ovum pick-up in sows: impacts on welfare and reproduction. Theriogenology 226, 68-75.
| Crossref | Google Scholar | PubMed |
Oltedal A, Gaustad AH, Peltoniemi O, Björkman S, Skaare A, Oropeza-Moe M (2024b) Experiences with transvaginal Ovum Pick-Up (OPU) in sows. Theriogenology 214, 157-165.
| Crossref | Google Scholar | PubMed |
Parrilla I, Gil MA, Cuello C, Cambra JM, Gonzalez-Plaza A, Lucas X, Vazquez JL, Vazquez JM, Rodriguez-Martinez H, Martinez EA (2022) Immunological uterine response to pig embryos before and during implantation. Reproduction in Domestic Animals 57(Suppl 5), 4-13.
| Crossref | Google Scholar | PubMed |
Polge C, Day B (1968) Pregnancy following non-surgical egg transfer in pigs. Veterinary Record 82, 712.
| Google Scholar |
Reichenbach HD, Mödl J, Brem G (1993) Piglets born after transcervical transfer of embryos into recipient gilts. Veterinary Record 133, 36-39.
| Crossref | Google Scholar | PubMed |
Roca J, Parrilla I, Bolarin A, Martinez EA, Rodriguez-Martinez H (2016) Will AI in pigs become more efficient? Theriogenology 86, 187-193.
| Crossref | Google Scholar | PubMed |
Sanchez-Osorio J, Cuello C, Gil MA, Almiñana C, Parrilla I, Caballero I, Garcia EM, Vazquez JM, Roca J, Martinez EA, Alminana C, Parrilla I, Caballero I, Garcia EM, Vazquez JM, Roca J, Martinez EA (2008) Factors affecting the success rate of porcine embryo vitrification by the open pulled straw method. Animal Reproduction Science 108, 334-344.
| Crossref | Google Scholar | PubMed |
Spötter A, Distl O (2006) Genetic approaches to the improvement of fertility traits in the pig. The Veterinary Journal 172, 234-247.
| Crossref | Google Scholar | PubMed |
Tajima S, Motoyama S, Wakiya Y, Uchikura K, Misawa H, Takishita R, Hirayama Y, Kikuchi K (2020) Piglet production by non-surgical transfer of vitrified embryos, transported to commercial swine farms and warmed on site. Animal Science Journal 91, e13476.
| Crossref | Google Scholar | PubMed |
Vallet JL, Freking BA, Miles JR (2011) Effect of empty uterine space on birth intervals and fetal and placental development in pigs. Animal Reproduction Science 125, 158-164.
| Crossref | Google Scholar | PubMed |
Wallenhorst S, Holtz W (1999) Transfer of pig embryos to different uterine sites. Journal of Animal Science 77, 2327-2329.
| Crossref | Google Scholar | PubMed |
Whitworth KM, Lee K, Benne JA, Beaton BP, Spate LD, Murphy SL, Samuel MS, Mao J, O’Gorman C, Walters EM, Murphy CN, Driver J, Mileham A, McLaren D, Wells KD, Prather RS (2014) Use of the CRISPR/Cas9 system to produce genetically engineered pigs from in vitro-derived oocytes and embryos. Biology of Reproduction 91, 78.
| Crossref | Google Scholar | PubMed |
Wilson ME, Biensen NJ, Youngs CR, Ford SP (1998) Development of Meishan and Yorkshire littermate conceptuses in either a Meishan or Yorkshire uterine environment to day 90 of gestation and to term. Biology of Reproduction 58, 905-910.
| Crossref | Google Scholar | PubMed |
Wolf E, Arnold GJ, Bauersachs S, Beier HM, Blum H, Einspanier R, Fröhlich T, Herrler A, Hiendleder S, Kölle S, Prelle K, Reichenbach H-D, Stojkovic M, Wenigerkind H, Sinowatz F (2003) Embryo-maternal communication in bovine – strategies for deciphering a complex cross-talk. Reproduction in Domestic Animals 38, 276-289.
| Crossref | Google Scholar | PubMed |
Wulster-Radcliffe MC, Seals RC, Lewis GS (2003) Progesterone increases susceptibility of gilts to uterine infections after intrauterine inoculation with infectious bacteria. Journal of Animal Science 81, 1242-1252.
| Crossref | Google Scholar | PubMed |
Xingzhu D, Qingrui Z, Keren C, Yuxi L, Yunpeng H, Shien Z, Xiangwei F (2021) Cryopreservation of porcine embryos: recent updates and progress. Biopreservation and Biobanking 19, 210-218.
| Crossref | Google Scholar | PubMed |
Yoshioka K, Noguchi M, Suzuki C (2012) Production of piglets from in vitro-produced embryos following non-surgical transfer. Animal Reproduction Science 131, 23-29.
| Crossref | Google Scholar | PubMed |
Yoshioka K, Uchikura K, Suda T, Matoba S (2020) Production of piglets from in vitro-produced blastocysts by ultrasound-guided ovum pick-up from live donors. Theriogenology 141, 113-119.
| Crossref | Google Scholar | PubMed |
Zou Y, Li Z, Zou Y, Hao H, Li N, Li Q (2018) An FBXO40 knockout generated by CRISPR/Cas9 causes muscle hypertrophy in pigs without detectable pathological effects. Biochemical and Biophysical Research Communications 498, 940-945.
| Crossref | Google Scholar | PubMed |