Identifying the composition of large vesicles in the cytoplasm of oocytes
Karen L. Reader
A
B
Abstract
Oocyte vesicles, or vacuoles, have been described using transmission electron microscopy in most species. In sheep and cow oocytes, vesicles constitute up to 30% of the cytoplasm, their volume decreases during maturation and is lower in poorer quality oocytes, suggesting they are important for oocyte competence. However, the composition and function of these organelles is unknown.
This study aimed to ascertain the content of oocyte vesicles and examine the effect of different fixation methods on the size and preservation of these organelles.
Sheep oocytes were centrifuged to segregate organelles then stained with organelle-specific fluorescent dyes (Nile Red, LysoTracker, Fluo-4-AM and TMRM) and imaged by live cell confocal microscopy. The oocytes were fixed with either glutaraldehyde or paraformaldehyde and prepared for electron microscopy to confirm the distribution of organelles and compare ultrastructure and organelle size.
Nile Red staining has identified that vesicles contain lipid that is different to that in the osmium-stained lipid droplets observed by electron microscopy. Lipid droplets and vesicles were significantly smaller when prepared for electron microscopy compared to live cell imaging. Organelles were less likely to be fully segregated following centrifugation in oocytes prior to maturation (20%) compared to oocytes after maturation (77%; P < 0.0001).
Oocyte vesicles are lipid storing organelles that may be important for oocyte quality.
This study highlights the importance of lipid for oocyte quality and the need for further research to identify the optimal fatty acid content for in vitro maturation media and oocyte competence.
Keywords: confocal microscopy, electron microscopy, lipid, oocyte quality, vesicles.
Introduction
Good oocyte quality is essential for successful fertilisation, embryo development and pregnancy; however, the factors determining oocyte quality are poorly understood (Satouh and Sato 2023). Fully grown oocytes are relatively large cells containing many organelles and metabolites that are accumulated during follicular growth from the primordial to ovulatory stage. The luteinising hormone surge triggers ovulation and maturation of both the oocyte cytoplasm and nucleus. Cytoplasmic oocyte maturation involves the redistribution of organelles, an increase in the number of mitochondria and mRNAs, and changes to the quantity of proteins and other metabolites required for fertilisation and early embryogenesis (Krisher 2004; Ferreira et al. 2009; Trebichalska et al. 2021).
A major component of the cytoplasm of oocytes from most species are large vesicles, also referred to as vacuoles. These have been observed using transmission electron microscopy (TEM) in oocytes from many species including cow, pig, sheep, possum, mouse and human (Cran 1985; Hyttel et al. 1986; Ducibella et al. 1988; Van Blerkom 1990; Falconnier and Kress 1992; Reader et al. 2015; Coticchio et al. 2016; Tatíčková et al. 2023). These organelles are typically between 0.5 μm and 2 μm in diameter, are surrounded by a single-layered membrane and appear to be relatively empty, apart from some granular material and occasionally what appears to be degraded mitochondria (Fair et al. 1997; Reader et al. 2015). Oocytes also contain vesicles from the golgi but these are significantly smaller at ~60 nm in diameter and have distinctive protein-coated bilayer membranes (Klumperman 2011). Large vesicles have been shown to be present in sheep and cow oocytes from primordial stage follicles (Fair et al. 1997; Reader et al. 2012). These vesicles make up between 15 and 35% of the cytoplasmic volume in immature (germinal vesicle stage) sheep, cow and pig oocytes, and their volume decreases during maturation (Cran 1985; Auclair et al. 2013; Reader et al. 2015) suggesting the vesicle contents are utilised during the maturation process. Using a sheep model of oocyte quality, the volume of vesicles was shown to be lower in poorer quality lamb oocytes prior to maturation, when compared to higher quality adult ewe oocytes (Reader et al. 2015). Auclair et al. (2013) compared the area fraction of vesicles after in vitro maturation (IVM) between denuded oocytes (DO) and cumulus-enclosed oocytes (CEO). The DO had a greater vesicle area fraction but a lower blastocyst rate than the CEO. Together these results indicate that vesicles are important for oocyte competence and that cumulus cells are involved in the utilisation of the vesicle content during maturation.
Vesicles are often closely associated with mitochondria and smooth endoplasmic reticulum (SER) (Hyttel et al. 1986; Reader et al. 2015). Despite these large vesicles having been observed in oocytes using TEM since the early 1970s, their composition and function has never been confirmed. Some papers have described them as lipid droplets (Senger and Saacke 1970), distended SER (Tatíčková et al. 2023) or large vacuoles indicative of poor oocyte quality (Van Blerkom 1990), while others have overlooked them. Petr et al. (2001) used an oxalate-pyroantimonate fixation method to precipitate calcium in pig oocyte vesicles indicating these organelles may be a storage site for calcium. Positive staining for N-acetylgalactosamine in cow vacuoles was observed by de Paz et al. (2001) while Falconnier and Kress (1992) used glycol-protein precipitating agents on possum oocytes and observed small amounts of unidentified flocculent material in the vesicles. To our knowledge, these are the only publications that have attempted to identify the composition of oocyte vesicles, and the results were not conclusive.
Oocyte vesicles have a similar ultrastructure to acidocalcisomes, which are acidic lysosome-related organelles that also store polyphosphates and cations such as zinc, calcium and magnesium (Ramos et al. 2010; Docampo and Moreno 2011). These organelles are thought to have a role in calcium homeostasis, osmoregulation and pH maintenance (Docampo and Moreno 2011). Acidocalcisomes have been described in bacteria, protists and in the eggs of sea urchins, insects and chickens but have not been studied in mammalian oocytes. Oocyte vesicles potentially contain soluble cations such as calcium that are lost during sample preparation for electron microscopy. They may also be some form of acidic or lysosomal organelle for the degradation of damaged mitochondria, which are occasionally observed within vesicles.
Vesicles have also been described as lipid droplets. Conventional preparation of oocytes for electron microscopy involves fixation with glutaraldhyde (GA), post-fixation with osmium tetroxide, dehydration with solvents and embedding in resin. The glutaraldehyde cross-links carbohydrates and proteins while osmium tetroxide binds to unsaturated lipids (Cheng et al. 2009). These lipid droplets appear dark in the electron microscope due to the high molecular weight of the osmium which limits transmission of the electron beam through the droplet. In contrast, the vesicles appear white and transparent to the electron beam (Reader et al. 2015). There are many fluorescent stains available for labelling specific organelles and metabolites such as lipids, calcium, mitochondria, acidic organelles and lysosomes. However, the vesicles are dispersed throughout the oocyte cytoplasm amongst all the other organelles, and it is not possible to identify exactly which organelles are fluorescently labelled with these dyes due to the limited resolution of light microscopes.
Many studies quantifying lipids and other organelles use confocal microscopy (CM) imaging of paraformaldehyde (PFA) fixed and fluorescently stained oocytes (Silva et al. 2023). However, it is well known that PFA is not very good at preserving ultrastructure and may therefore affect the localisation of staining. In contrast, oocytes are usually fixed with glutaraldehyde for TEM studies which provides superior preservation of the ultrastructure, but the use of solvents in EM sample preparation is known to cause significant shrinkage and loss of lipid. Despite this common understanding, we were unable to find publications that directly compared ultrastructural preservation in the same samples fixed by either PFA or GA and examined by TEM.
We hypothesised that vesicles are storage organelles for saturated lipids and soluble calcium important for oocyte maturation, fertilisation and early embryo development. Cran (1987) demonstrated that centrifuging sheep, cow and pig oocytes resulted in segregation of the organelles into discrete layers. The aim of this study was to use centrifugation to separate the organelles into layers, followed by staining and live cell imaging with fluorescent dyes specific to mitochondria, calcium, lysosomes and lipid to identify the composition of oocyte vesicles which are important for oocyte quality. A better understanding of the exact composition and quantities of metabolites required for good oocyte quality, particularly lipids, is required to improve success rates in assisted reproductive technologies (Khan et al. 2021). This knowledge could be used to identify ways of increasing vesicle volume, either in vitro or in vivo, to improve oocyte quality. The second aim of our study was to examine the effect of PFA fixation on the ultrastructure of oocyte organelles and compare lipid droplet and vesicle size between unfixed oocytes imaged by CM and PFA or GA fixed oocytes imaged by TEM to identify the best method for quantifying lipids in oocyte quality studies.
Methods
All reagents were sourced from Sigma/Merck, Auckland, New Zealand, unless otherwise stated.
Oocyte collection and in vitro maturation
Following the methods described in Reader et al. (2015) and detailed here, abattoir-derived ovaries (~80–100 per collection date) were obtained from adult (>18 months) and juvenile (6–9 months) sheep reproductive tracts during March, April and May to coincide with the natural breeding season for sheep in New Zealand. Ovaries and oocytes were maintained at 37–38°C throughout the collection, staining and live cell imaging steps. Fully grown oocytes were aspirated from all visible follicles >1 mm using a 20-G needle on a 10-mL syringe with HEPES-buffered M199 medium containing 0.1 M kanamycin, 0.04% bovine serum albumin (BSA low endotoxin, MedRay, Auckland, New Zealand) and 5000 IU/mL heparin sodium (Pfizer, Auckland, New Zealand). Good quality oocytes that had a uniform density of cytoplasm and multiple, complete layers of cumulus cells were selected and pooled for each experiment. Cumulus oocyte complexes (COCs) were in vitro matured (IVM) in groups of 10 in 50-μL drops of bicarbonate-buffered M199 with 10% fetal calf serum (FCS), 10 μg mL−1 ovine FSH (Vetoquinol, Canada), 1 μg mL−1 17β-oestradiol, 0.2 mM sodium pyruvate and 0.1 mM cysteamine, under mineral oil for 18–22 h in 5% CO2 in air. Meiotic maturation status was not confirmed but for simplicity oocytes prior to IVM will be referred to as GV stage and post IVM as MII stage oocytes.
Oocyte denuding, centrifugation and fluorescent staining
Oocytes were transferred to 800 μL of phosphate-buffered saline (PBS) with 1 mg mL−1 polyvinylpyrrolidone (PVP) in a 4-well plate and cumulus cells were removed by vigorous pipetting with a 1-mL pipette. Denuded oocytes were transferred through three wells of PBS + PVP to wash away the cumulus cells. For centrifugation, oocytes were washed in H199 + 10% FCS, transferred to a 1.5-mL microfuge tube in 1 mL of media and centrifuged at 10,000g for 20 min to segregate the organelles into layers. The oocytes were resuspended by gentle pipetting and transferred to a 35-mm Petri dish containing H199 + 10% FCS before staining with organelle-specific fluorescent stains in a 4-well dish at 38°C in the dark. Groups of centrifuged oocytes were stained with either LysoTracker Red, Fluo-4-AM calcium indicator, tetramethylrhodamine (TMRM) or Nile Red lipid stain and some were also co-stained with DAPI. All stains were sourced from Thermo Fisher Scientific, Auckland, New Zealand. Stained oocytes were transferred in pools of ~10 oocytes in 5 μL of staining media onto a glass-bottomed Petri dish and overlaid with 2 mL of mineral oil to prevent evaporation. These were imaged on a warming stage set to 37°C with an Andor Dragonfly spinning disk confocal microscope (Andor, Australia). Fluorescent dye concentrations and staining times were based on a combination of previously published literature and recommendations from the suppliers (Genicot et al. 2005; Al-Zubaidi et al. 2019; Liu et al. 2019). The stain concentrations, staining times, excitation and emission filters, number of centrifuged oocytes imaged, and number of collection dates are presented in Table 1.
Stain | Conc | Time | Excitation (nm) | Emission (nm) | Number of oocytes | Number of collection dates | |
---|---|---|---|---|---|---|---|
LysoTracker Red | 50 nM | 1 h | 561 | 600 | 17 | 6 | |
Fluo-4-AM | 5 μM | 1 h | 488 | 521 | 11 | 4 | |
TMRM | 250 nM | 1 h | 561 | 600 | 8 | 3 | |
DAPI | 5 μM | 1 h | 405 | 450 | 5 | 2 | |
Nile Red | 10 μg mL−1 | 2 h | 488 and 561 | 600 | 10 | 4 |
Confocal microscopy image processing
Microscope detector noise was removed using a pre-trained AI image processing tool (Denoise.Ai, Nikon) to ensure accurate detection and measurement of vesicles in the acquired 3-dimensional volume. Digital images were captured using maximum intensity of Z projections to visualise fluorescent data through the image stacks of the oocyte. Oocyte images had a Z-Step of 0.22 μm. The mean diameters of 100 lipid droplets and 100 vesicles were measured in confocal micrographs of three unfixed, centrifuged, Nile Red-stained oocytes.
Transmission electron microscopy
The following groups of adult and juvenile oocytes (from one collection date each) were centrifuged and prepared for TEM: GV COC, GV DO, MII COC and MII DO to determine whether age, the presence of cumulus cells or maturation stage affected the ability of the organelles to be redistributed by centrifugation. Oocytes were prepared for TEM according to the protocol described by Reader et al. (2015) and chemicals were sourced from ProSciTech, Queensland, Australia. In brief, the oocytes were fixed in 2% glutaraldehyde in 0.1 M sodium cacodylate buffer (NaCac) (pH 7.4) for 1 h at room temperature, then overnight at 4°C, then washed in 0.1 M NaCac with 0.1% BSA added to minimise adhesion. Oocytes were individually encased in a drop of 3% molten low gelling temperature agarose in 0.1 M NaCac prior to post-fixation for 1 h in 1% osmium tetroxide in 0.1 M NaCac at room temperature. They were subsequently washed in 0.1 M NaCac then H2O, stained in 2% uranyl acetate, dehydrated through increasing concentrations of ethanol and 100% propylene oxide and embedded in Agar 100 resin (Agar, Essex, UK). Sections through the middle of the oocyte, 2-μm thick, were mounted on slides and stained with 1% methylene blue. Oocytes sectioned across all organelle layers were viewed on a light microscope and classified as being either well-layered or not layered. Ultrathin 80-nm sections were mounted on formvar-coated slot grids, then stained with uranyl acetate and lead citrate. Oocytes were imaged with a JEOL 1400 Flash TEM. One pool of MII stage centrifuged DO were fixed in 4% PFA in PBS and prepared for TEM as above. The diameters of all visible lipid droplets and vesicles in the TEM sections from the middle of centrifuged, GA or PFA fixed oocytes (5 of each) were measured. The number of lipid droplets measured ranged 12–78 per oocyte and vesicle number ranged 227–684 per oocyte.
Data analysis
Data was analysed using GraphPad Prism version 10.2.3. The proportions of GV and MII oocytes, or GV DO and GV COC, with well-segregated or poorly segregated organelle layers were analysed by a Fisher’s exact test. The mean lipid droplet and vesicle diameters for each oocyte comparing fixation methods were analysed by one-way ANOVA and corrected for multiple comparisons with the two-stage linear step-up procedure of Benjamini, Krieger and Yekutieli, with a false discovery rate (FDR) of P < 0.05 being considered significantly different. All data was normally distributed.
Results
Uncentrifuged sheep oocytes prepared for TEM showed a high proportion of the cytoplasm contained vesicles (Fig. 1a). In the MII stage oocytes, the mitochondria were evenly distributed while the lipid droplets were present in the periphery of the cytoplasm. After centrifugation, some oocytes exhibited organelles that were segregated into discrete layers (Fig. 1b) while the organelles in other oocytes did not segregate fully (Fig. 1c). In those oocytes with well-segregated organelle layers, mitochondria and lipid droplets were observed at opposite poles with the lipid droplets often present outside of the oolemma (Fig. 1d, e). A large layer of vesicles, some with mitochondria closely associated, formed next to the lipid droplet layer, overlaid by a thin layer of SER (Fig. 1f). A region of empty cytoplasm was visible between the SER and mitochondrial layers, and no rough ER were observed (Fig. 1b, d). Cortical granules remained located adjacent to the oolemma in the MII stage oocytes after centrifugation.
TEM images of sheep oocytes fixed with 2% glutaraldehyde. (a) Adult MII stage oocyte not centrifuged. (b) Adult MII stage oocyte centrifuged with well-segregated organelle layers. (c) Juvenile MII denuded oocyte centrifuged showing poorly segregated organelle layers. (d) Higher magnification image of mitochondria at one pole of a centrifuged oocyte. (e) Lipid droplet layer outside of oolemma. (f) Vesicles and smooth endoplasmic reticulum layers. Cumulus cells (cc), zona pellucida (zp), lipid droplets (LD and arrows), vesicles (v), mitochondria (m), cytoplasm (cy), smooth endoplasmic reticulum (ser).
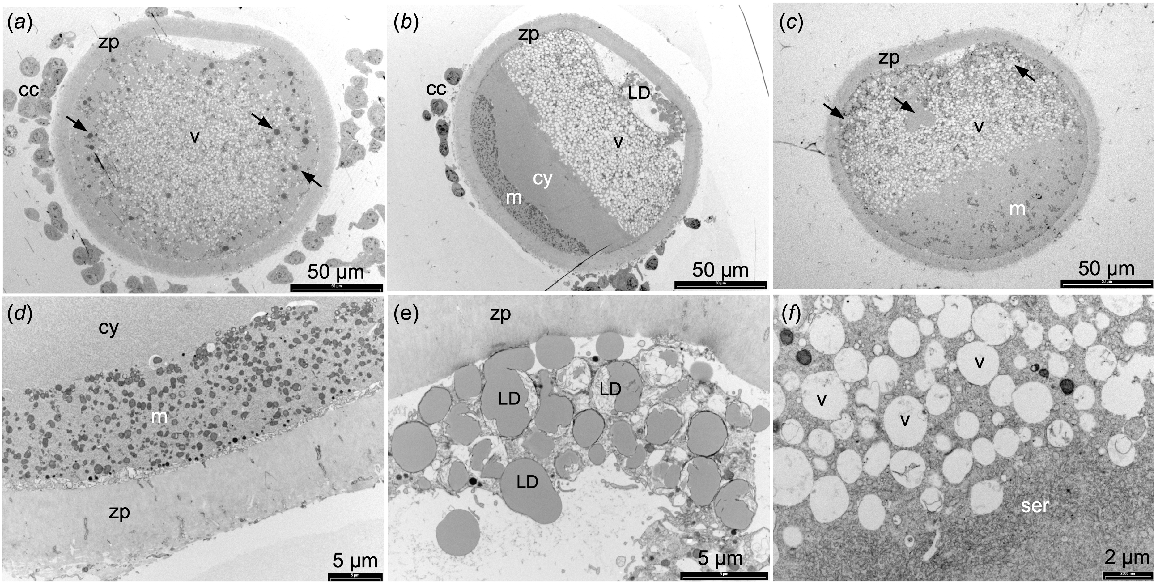
Table 2 presents the number of centrifuged DOs and COCs examined in the semi-thin sections by light microscopy from each maturation stage and animal age, and whether the organelles were well layered or not. There was no difference between adult and juvenile oocytes in the proportion of GV or MII stage oocytes that segregated following centrifugation so data from the two age groups was pooled and then analysed. Following centrifugation 77% of the MII oocytes (n = 65) exhibited well-segregated organelles while only 20% of GV stage oocytes (n = 56) were well segregated (P < 0.0001). There was no difference in the percentage of GV stage DOs with well-layered organelles (12%, n = 25) compared to the GV COC (26%, n = 31) indicating that the presence or absence of cumulus cells did not affect organelle segregation.
Stage | GV | MII | |||||||
---|---|---|---|---|---|---|---|---|---|
Age | Juvenile | Adult | Juvenile | Adult | |||||
Cumulus cells | COC | DO | COC | DO | COC | DO | COC | DO | |
Layered | 5 | 2 | 3 | 1 | 12 | 10 | 13 | 15 | |
Not layered | 9 | 14 | 14 | 8 | 4 | 4 | 3 | 4 |
When centrifuged oocytes were stained with Nile Red and excited with a 561-nm wavelength, some organelles in the vesicle layer were visible but none of the lipid droplets appeared to fluoresce (Fig. 2b, e). In contrast, using an excitation wavelength of 488 nm, organelles were visible in both the vesicle and lipid droplet layers (Fig. 2c, f). The merged images show vesicles fluorescing with both wavelengths in yellow (Fig. 2d, g). Some organelles in the vesicle layer were positive for LysoTracker Red staining that labels lysosomes (Fig. 2h). These likely correspond to the lysosomes observed in the TEM images which have a different ultrastructure to the vesicles and are of low abundance in most oocytes (Fig. 2h inset). The lysosomes were surrounded by a complete double-layered membrane and contained multiple small vesicles and granules, as opposed to the single and often disrupted membrane surrounding the relatively empty vesicles (Fig. 2h inset). As expected, TMRM staining was visible as small punctate fluorescence only in the mitochondrial layer at the pole of the oocyte (Fig. 2i). Fluo-4-AM calcium staining was observed in the mitochondrial layer of all oocytes examined (Fig. 2j). Calcium staining was also visible in the SER layer in one oocyte that was imaged in enough depth to observe this region, indicating that both mitochondria and SER, but not vesicles, are important storage sites for calcium (Fig. 2j). Interestingly, DAPI staining of DNA was visible in both the nucleus and the mitochondria (Fig. 2k) indicating that the mitochondrial DNA was able to be detected by the enhanced sensitivity of the spinning disk CM.
TEM images and CM 3D projections of centrifuged oocytes. (a) TEM image of centrifuged oocyte indicating the layers of vesicles (v), lipid droplets (LD), mitochondria (m) and smooth endoplasmic reticulum (ser). CM images of Nile Red-stained oocyte using 561-nm excitation (b, e), 488-nm excitation (c, f) and the merged image (d, g). (h) CM image of centrifuged oocyte stained with LysoTracker Red with positive staining in some of the vesicles as well as in the cumulus cells (arrowheads). (h, inset) TEM image showing vesicles (v) and a lysosome (arrow). (i) CM image of oocyte stained with TMRM for mitochondria. (j) CM image of oocyte stained with Fluo-4-AM for calcium showing positive staining in the mitochondria (m) and smooth endoplasmic reticulum (ser). (k) CM image of DAPI staining DNA in both the nucleus (n) and mitochondria (m).
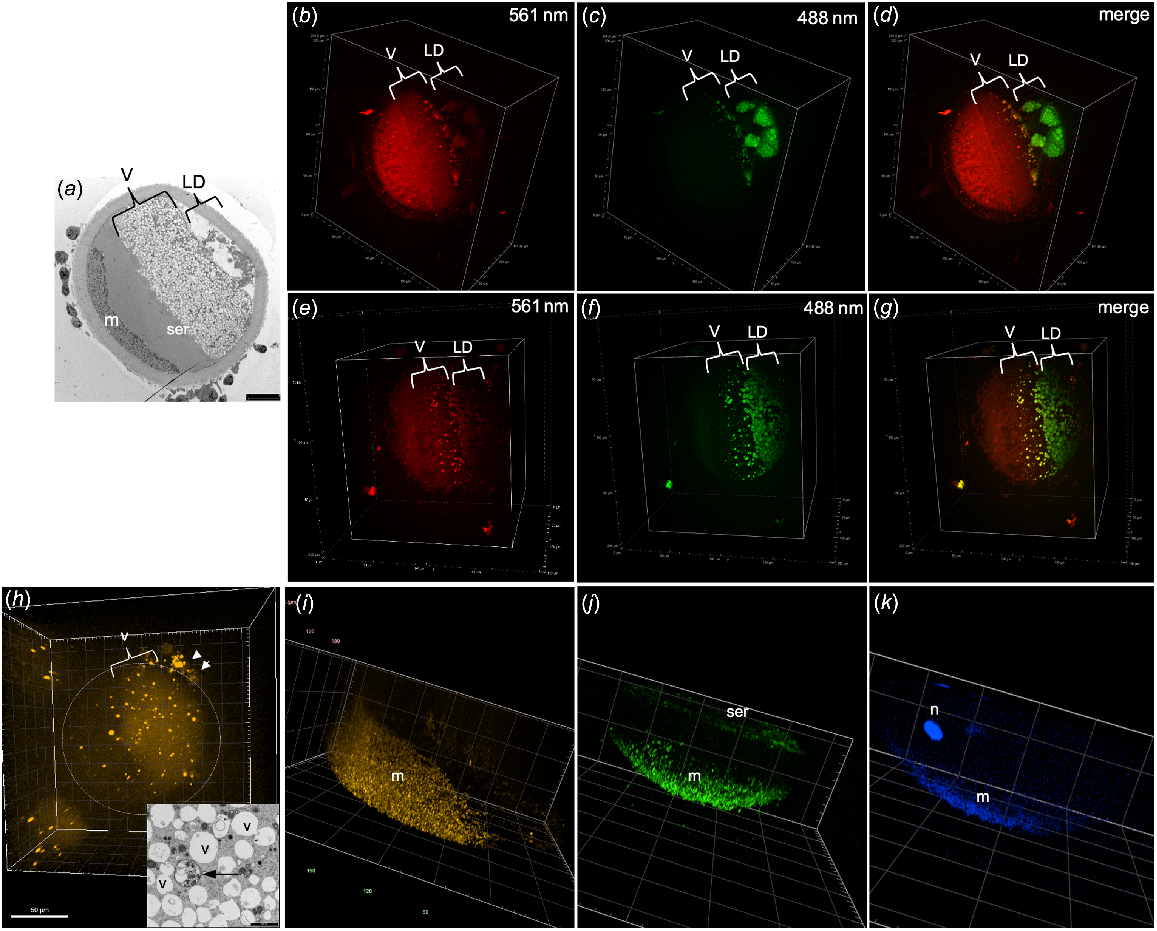
Lipid droplets were significantly larger than the vesicles in unfixed oocytes imaged by CM as well as in PFA and GA fixed oocytes imaged by TEM (P < 0.001; Fig. 3). There was no significant difference in the mean diameter of vesicles or lipid droplets between PFA and GA fixed oocytes imaged by TEM. Lipid droplets were 58% and 55% (P < 0.001) smaller following preparation for TEM with GA and PFA fixation, respectively, when compared to unfixed oocytes imaged by CM (Fig. 3). Vesicles exhibited slightly more shrinkage being 62% and 66% smaller in TEM with GA and PFA fixation, respectively, when compared to unfixed oocytes imaged by CM (Fig. 3).
Graph showing the mean (±s.e.m.) diameter of lipid droplets (LD) and vesicles (Ves) measured in either confocal microscope (CM) images of unfixed Nile Red stained oocytes (n = 3), or in glutaraldehyde (GA) fixed (n = 5) or paraformaldehyde (PFA) fixed (n = 5) oocytes imaged by TEM (EM). Different letters represent significant differences P < 0.001 by one-way ANOVA.
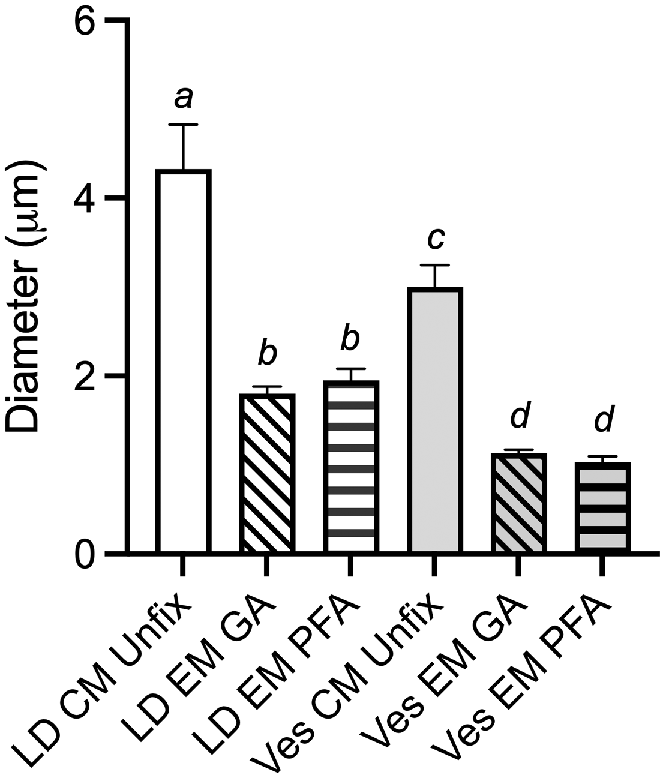
Oocytes fixed in PFA and prepared for TEM exhibited well-preserved zona pellucida, lipid droplets and mitochondria (Fig. 4a, c) that looked very similar to the GA fixed oocytes (Fig. 1). However, the vesicles and cytoplasm were poorly preserved with the vesicles appearing very irregular and the cytoplasm patchy and granular, suggesting loss of components (Fig. 4b, c). The SER surrounding the mitochondria also appeared to be swollen or distended in the PFA fixed oocytes (Fig. 4c).
TEM images of sheep oocytes fixed in 4% paraformaldehyde. (a) Image shows well-preserved zona pellucida (zp) and lipid droplets (arrows). (b) Vesicles (v) and cytoplasm (cy) are poorly fixed. (c) Mitochondria (m) appear to have well-preserved inner and outer membranes but the cytoplasm (cy) is patchy and granular and SER is distended (arrows).
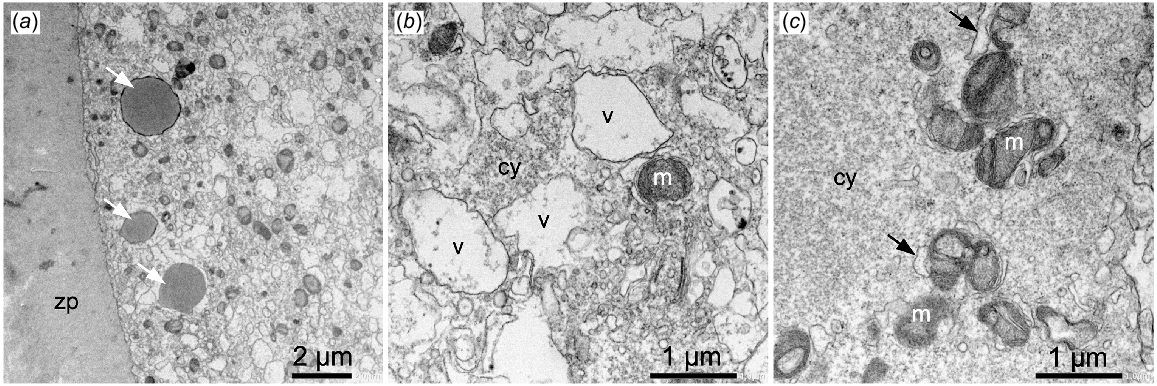
Discussion
This study has confirmed for the first time that at least a proportion of storage vesicles in oocytes contain lipids that are stained by the lipophilic dye Nile Red. Furthermore, these lipids differ in composition from the classical lipid droplets observed as large, osmium stained, spherical structures in cells prepared for TEM. Nile Red has been shown to be a sensitive method for detecting intracellular lipids such as phospholipids, triacylglycerol and cholesterol and has been used to measure lipid quantity in bovine oocytes and embryos (Greenspan et al. 1985; Leroy et al. 2005). It can potentially stain both saturated and unsaturated fatty acids but has a lower affinity for saturated fatty acids (Fowler et al. 1987). While Nile Red staining was observed in both the lipid droplet and vesicle layers of centrifuged oocytes, they were visible with different excitation wavelengths indicating these organelles contain different types of lipid. Schnitzler et al. (2017) has reported that in the presence of Nile Red, neutral lipids such as triacylglycerols are excited by a 488-nm laser while phospholipids are excited at 590 nm. Similarly, Diaz et al. (2008) compared Nile Red-stained lipids with different hydrophobic properties and demonstrated that oleic acid, mono olein and phosphatidyl choline had a higher ratio of red emission with 540/590-nm excitation/emission wavelengths than triolein and esterified cholesterol with 460/535-nm excitation/emission. However, this method was not able to determine the composition of mixtures of lipid present in droplets in cells. Romek et al. (2011) used Nile Red staining to compare the lipid content of different stages of oocyte maturation and embryo development in pigs. Their study used a single excitation wavelength and continuous emission spectra to determine the emission maxima to quantify triglycerides, phospholipids and cholesterol based on their different polarity. Our preliminary imaging did not observe differences between the vesicle and lipid droplet layers using different emission filters (600 nm, 620 nm or 700 nm) but did when using 488-nm and 561-nm excitation combined with a 600-nm emission filter. In the current study, the lipid droplet layer fluoresced with the 488-nm laser while the vesicle layer was visible with both 488 nm and 561 nm, but this did not allow us to conclude what type of fatty acids were present in the vesicles unlike the study done by Romek et al. (2011).
Centrifugation caused the oocyte organelles to be layered in the same order as described by Cran (1987) with the lipid droplets located at one pole of the oocyte, overlaid by a large layer of vesicles and then a thin layer of SER. This indicates the lipid droplets have a higher density than the vesicles, which corresponds to their larger diameter and additional density provided by the osmium stain. Osmium tetroxide is a heavy metal compound used for post-fixation and staining of lipids for electron microscopy; however, it only binds to unsaturated fatty acids (Adams et al. 1967; Cheng et al. 2009). Since the vesicles, which constituted a greater area of the oocyte cytoplasm, stained positively with the Nile Red lipid stain but appear empty or unstained in the TEM, it is likely that the vesicles contain saturated fatty acids that are not bound by the osmium tetroxide. In support of this hypothesis, saturated fatty acids such as palmitic and stearic acid are the most abundant fatty acids in cattle, pig and sheep oocytes (McEvoy et al. 2000). Aardema et al. (2011) have shown that supplementing bovine oocytes with either palmitic or stearic acid (saturated lipids) during IVM reduced the volume of lipid in oocytes, measured with Bodipy 493/503 staining, and decreased cleavage and blastocyst rates, while oleic acid (an unsaturated lipid) had the opposite effect. These results suggest that the Bodipy 493/503 may only be staining unsaturated lipid. The volume of osmophilic lipid droplets (unsaturated fatty acid) measured in TEM images did not change during IVM of pig and sheep oocytes while vesicle volume decreased (Cran 1985; Reader et al. 2015). Together, these studies suggest that vesicles contain saturated fatty acids that are utilised or removed during oocyte maturation, but an optimal concentration is required for oocyte competence. Fatty acid metabolism is important for oocyte developmental competence and is regulated by the maternal physiological environment (Dunning et al. 2014; Khan et al. 2021). Therefore, a better understanding of the composition and quantities of cytoplasmic lipids in good and poor quality oocytes may identify ways of improving oocyte quality in vitro and potentially in vivo through modification of diet.
As we had observed that some vesicles contained small structures and membranes that appeared to be degraded mitochondria, we hypothesised that these organelles may also function as some form of lysosomal organelle. Some organelles distributed throughout the vesicle layer stained positively with the lysosome-specific dye LysoTracker Red, but the number and size of these organelles varied between oocytes and staining was quickly bleached. Double-membrane bound lysosomes, similar to those observed in human oocytes (Trebichalska et al. 2021; Tatíčková et al. 2023), were present amongst the vesicle layer in centrifuged oocytes observed by TEM, and their number appeared to be quite variable between oocytes. Therefore, the positive LysoTracker Red staining observed by CM is likely to be in the lysosomes not vesicles. Dual staining with a more photo-stable lysosomal dye and Nile Red could be used to confirm this.
Staining with the Fluo-4-AM calcium-specific dye was observed in both the mitochondrial layer and SER. The SER is usually closely associated with the mitochondria and vesicles (Hyttel et al. 1986; Reader et al. 2015), but after centrifugation some of the SER became separated from these organelles to form a discrete narrow layer in the middle of the oocyte. This technique highlighted how little free SER is present in the oocyte cytoplasm.
Live cell CM imaging of uncentrifuged sheep oocytes was challenging with an apparent loss of fluorescent intensity from the middle of the oocyte cytoplasm and difficulty in imaging throughout the complete depth of the cell. This is likely due to the density of lipid within the cytoplasm either preventing the laser from penetrating into the centre of the oocyte or scattering the fluorescence, both of which would decrease the light reaching the objective. It is also possible that the relatively large volume of lipid and fluid in the spherical oocytes (and its corresponding refractive index) imposes a diffraction limit on the axial resolution of a confocal microscope for structures of this nature. Imaging centrifuged oocytes with the organelle layers oriented perpendicular to the laser improved the ability of the spinning disk CM to detect fluorescence from throughout the oocyte cytoplasm and could be a useful method for quantifying the volumes of different organelle compartments.
Preparation of the oocytes for TEM resulted in a significant reduction in the diameters of the lipid droplets and vesicles, presumably due to extraction of the lipids by the solvents used to dehydrate the specimens prior to resin embedding. Our results show that live cell imaging of these organelles in unfixed oocytes will allow more accurate measurement of their volumes, providing there is adequate resolution. However, after centrifugation the lipid droplets appeared to form larger conglomerates in the CM images compared to the TEM images. It was more difficult to accurately detect the edge of the fluorescently stained lipid droplets which could result in an overestimate of droplet diameter, or an underestimate of the number of droplets. It is also possible that centrifugation and fluorescent staining may cause the lipid droplets to merge.
As expected, the ultrastructural preservation was poorer with PFA fixation when compared to GA, particularly for the vesicles, SER and cytoplasm. This should be considered when interpreting fluorescent images of PFA fixed cells, in particular for experiments examining co-localisation of molecules which have the potential to move out of poorly fixed organelles and structures. Unfortunately, there are little to no published papers directly comparing the effect of different fixation protocols on the ultrastructural integrity of particular cells and organelles.
Organelles are known to redistribute during maturation with mitochondria and lipid droplets moving from the cortex to an even distribution presumably to ensure the cells of the developing embryo receive a sufficient complement of organelles and metabolites (Mao et al. 2014; Reader et al. 2015; Trebichalska et al. 2021). The observation that centrifuging GV stage oocytes was less likely to result in well-segregated layers of organelles compared to MII stage oocytes indicates that the organelles are immobilized somehow prior to maturation. Some studies have observed actin, intermediate filaments and microtubules in oocytes and their presence and distribution changes during oocyte maturation (Mao et al. 2014). Tatíčková et al. (2023) identified that disruption of actin filaments but not microtubules affected organelle distribution during human oocyte maturation, but whether these are involved in inhibiting organelle segregation with centrifugation is unknown. Centrifugation may be a useful method for non-destructively identifying which oocytes have completed successful cytoplasmic maturation and are of higher quality, as oocytes are able to develop after centrifugation (Wall et al. 1985; Wall and Hawk 1988).
To summarise, we have identified for the first time that at least a proportion of the large vesicles observed in sheep oocytes by TEM contain lipid that differs from that contained within the osmophilic lipid droplets. The vesicles did not stain for calcium so are not likely to be SER. Live cell imaging of unfixed oocytes may allow for more accurate quantification of fluorescently stained lipid volumes, than electron microscopy, but not all lipid is stained by these dyes. Further research is needed to confirm the exact composition of the vesicle lipids and whether they can be used as either a marker of oocyte quality or supplemented to improve oocyte quality.
Data availability
The data used to generate the results in this paper is available on request from the corresponding author.
Declaration of funding
This project was funded by a University of Otago Research Grant to KLR and Honours student project funding from the Dunedin School of Medicine.
Acknowledgements
We would like to thank Sylvia Zellhuber-McMillan for assistance with the oocyte collections and preparation of media and ultra-thin sections. Microscopy was performed in the Confocal Microscopy and Electron Microscopy units, Research Infrastructure Centre at the University of Otago, Dunedin, New Zealand. KLR would especially like to acknowledge the late Ken McNatty for starting her on this oocyte discovery journey and for his many years of supervision, encouragement and mentorship.
References
Aardema H, Vos PLAM, Lolicato F, Roelen BAJ, Knijn HM, Vaandrager AB, Helms JB, Gadella BM (2011) Oleic acid prevents detrimental effects of saturated fatty acids on bovine oocyte developmental competence. Biology of Reproduction 85(1), 62-69.
| Crossref | Google Scholar | PubMed |
Adams CWM, Abdulla YH, Bayliss OB (1967) Osmium tetroxide as a histochemical and histological reagent. Histochemie 9(1), 68-77.
| Crossref | Google Scholar | PubMed |
Al-Zubaidi U, Liu J, Cinar O, Robker RL, Adhikari D, Carroll J (2019) The spatio-temporal dynamics of mitochondrial membrane potential during oocyte maturation. Molecular Human Reproduction 25(11), 695-705.
| Crossref | Google Scholar | PubMed |
Auclair S, Uzbekov R, Elis S, Sanchez L, Kireev I, Lardic L, Dalbies-Tran R, Uzbekova S (2013) Absence of cumulus cells during in vitro maturation affects lipid metabolism in bovine oocytes. American Journal of Physiology - Endocrinology and Metabolism 304(6), E599-E613.
| Crossref | Google Scholar | PubMed |
Cheng J, Fujita A, Ohsaki Y, Suzuki M, Shinohara Y, Fujimoto T (2009) Quantitative electron microscopy shows uniform incorporation of triglycerides into existing lipid droplets. Histochemistry and Cell Biology 132(3), 281-291.
| Crossref | Google Scholar | PubMed |
Coticchio G, Dal Canto M, Fadini R, Mignini Renzini M, Guglielmo MC, Miglietta S, Palmerini MG, Macchiarelli G, Nottola SA (2016) Ultrastructure of human oocytes after in vitro maturation. Molecular Human Reproduction 22(2), 110-118.
| Crossref | Google Scholar | PubMed |
Cran DG (1985) Qualitative and quantitative structural changes during pig oocyte maturation. Journal of Reproduction and Fertility 74(1), 237-245.
| Crossref | Google Scholar | PubMed |
Cran DG (1987) The distribution of organelles in mammalian oocytes following centrifugation prior to injection of foreign DNA. Gamete Research 18(1), 67-76.
| Crossref | Google Scholar | PubMed |
de Paz P, Sánchez AJ, De la Fuente J, Chamorro CA, Alvarez M, Anel E, Anel L (2001) Ultrastructural and cytochemical comparison between calf and cow oocytes. Theriogenology 55(5), 1107-1116.
| Crossref | Google Scholar | PubMed |
Diaz G, Melis M, Batetta B, Angius F, Falchi AM (2008) Hydrophobic characterization of intracellular lipids in situ by Nile Red red/yellow emission ratio. Micron 39(7), 819-824.
| Crossref | Google Scholar | PubMed |
Docampo R, Moreno SNJ (2011) Acidocalcisomes. Cell Calcium 50(2), 113-119.
| Crossref | Google Scholar | PubMed |
Ducibella T, Rangarajan S, Anderson E (1988) The development of mouse oocyte cortical reaction competence is accompanied by major changes in cortical vesicles and not cortical granule depth. Developmental Biology 130(2), 789-792.
| Crossref | Google Scholar | PubMed |
Dunning KR, Russell DL, Robker RL (2014) Lipids and oocyte developmental competence: the role of fatty acids and β-oxidation. Reproduction 148(1), R15-R27.
| Crossref | Google Scholar | PubMed |
Fair T, Hulshof SC, Hyttel P, Greve T, Boland M (1997) Oocyte ultrastructure in bovine primordial to early tertiary follicles. Anatomy and Embryology 195(4), 327-336.
| Crossref | Google Scholar | PubMed |
Falconnier C, Kress A (1992) Ultrastructural aspects of oocyte growth in the marsupial Monodelphis domestica (grey short-tailed opossum). Journal of Anatomy 181(Pt 3), 481-98.
| Google Scholar | PubMed |
Ferreira EM, Vireque AA, Adona PR, Meirelles FV, Ferriani RA, Navarro PAAS (2009) Cytoplasmic maturation of bovine oocytes: structural and biochemical modifications and acquisition of developmental competence. Theriogenology 71(5), 836-848.
| Crossref | Google Scholar | PubMed |
Fowler SD, Brown WJ, Warfel J, Greenspan P (1987) Use of nile red for the rapid in situ quantitation of lipids on thin-layer chromatograms. Journal of Lipid Research 28(10), 1225-1232.
| Crossref | Google Scholar | PubMed |
Genicot G, Leroy JLMR, Soom AV, Donnay I (2005) The use of a fluorescent dye, Nile red, to evaluate the lipid content of single mammalian oocytes. Theriogenology 63(4), 1181-1194.
| Crossref | Google Scholar | PubMed |
Greenspan P, Mayer EP, Fowler SD (1985) Nile red: a selective fluorescent stain for intracellular lipid droplets. Journal of Cell Biology 100(3), 965-973.
| Crossref | Google Scholar | PubMed |
Hyttel P, Callesen H, Greve T (1986) Ultrastructural features of preovulatory oocyte maturation in superovulated cattle. Journal of Reproduction and Fertility 76(2), 645-656.
| Crossref | Google Scholar | PubMed |
Khan R, Jiang X, Hameed U, Shi Q (2021) Role of lipid metabolism and signaling in mammalian oocyte maturation, quality, and acquisition of competence. Frontiers in Cell and Developmental Biology 9, 639704.
| Crossref | Google Scholar | PubMed |
Klumperman J (2011) Architecture of the mammalian Golgi. Cold Spring Harbor Perspectives in Biology 3(7), a005181.
| Crossref | Google Scholar |
Krisher RL (2004) The effect of oocyte quality on development. Journal of Animal Science 82(E-Suppl), E14-23.
| Crossref | Google Scholar |
Leroy JLMR, Genicot G, Donnay I, Van Soom A (2005) Evaluation of the lipid content in bovine oocytes and embryos with nile red: a practical approach. Reproduction in Domestic Animals 40(1), 76-78.
| Crossref | Google Scholar | PubMed |
Liu YJ, Ji DM, Liu ZB, Wang TJ, Xie FF, Zhang ZG, Wei ZL, Zhou P, Cao YX (2019) Melatonin maintains mitochondrial membrane potential and decreases excessive intracellular Ca2+ levels in immature human oocytes. Life Sciences 235, 116810.
| Crossref | Google Scholar | PubMed |
Mao L, Lou H, Lou Y, Wang N, Jin F (2014) Behaviour of cytoplasmic organelles and cytoskeleton during oocyte maturation. Reproductive BioMedicine Online 28(3), 284-299.
| Crossref | Google Scholar | PubMed |
McEvoy TG, Coull GD, Broadbent PJ, Hutchinson JS, Speake BK (2000) Fatty acid composition of lipids in immature cattle, pig and sheep oocytes with intact zona pellucida. Journal of Reproduction and Fertility 118(1), 163-170.
| Crossref | Google Scholar | PubMed |
Petr J, Rozinek J, Hruban V, Jílek F, Sedmíková M, Vaňourková Z, Němeček Z (2001) Ultrastructural localization of calcium deposits during in vitro culture of pig oocytes. Molecular Reproduction and Development 58(2), 196-204.
| Crossref | Google Scholar | PubMed |
Ramos IB, Miranda K, Pace DA, Verbist KC, Lin FY, Zhang Y, Oldfield E, Machado EA, De Souza W, Docampo R (2010) Calcium- and polyphosphate-containing acidic granules of sea urchin eggs are similar to acidocalcisomes, but are not the targets for NAADP. Biochemical Journal 429(3), 485-495.
| Crossref | Google Scholar | PubMed |
Reader KL, Haydon LJ, Littlejohn RP, Juengel JL, McNatty KP (2012) Booroola BMPR1B mutation alters early follicular development and oocyte ultrastructure in sheep. Reproduction, Fertility and Development 24(2), 353-361.
| Crossref | Google Scholar | PubMed |
Reader KL, Cox NR, Stanton J-AL, Juengel JL (2015) Mitochondria and vesicles differ between adult and prepubertal sheep oocytes during IVM. Reproduction, Fertility and Development 27(3), 513-522.
| Crossref | Google Scholar | PubMed |
Romek M, Gajda B, Krzysztofowicz E, Kepczynski M, Smorag Z (2011) New technique to quantify the lipid composition of lipid droplets in porcine oocytes and pre-implantation embryos using Nile Red fluorescent probe. Theriogenology 75(1), 42-54.
| Crossref | Google Scholar | PubMed |
Satouh Y, Sato K (2023) Reorganization, specialization, and degradation of oocyte maternal components for early development. Reproductive Medicine and Biology 22(1), e12505.
| Crossref | Google Scholar | PubMed |
Schnitzler JG, Bernelot Moens SJ, Tiessens F, Bakker GJ, Dallinga-Thie GM, Groen AK, Nieuwdorp M, Stroes ESG, Kroon J (2017) Nile Red Quantifier: a novel and quantitative tool to study lipid accumulation in patient-derived circulating monocytes using confocal microscopy. Journal of Lipid Research 58(11), 2210-2219.
| Crossref | Google Scholar | PubMed |
Senger PL, Saacke RG (1970) Unusual mitochondria of the bovine oocyte. Journal of Cell Biology 46(2), 405-408.
| Crossref | Google Scholar | PubMed |
Silva TCF, Dode MAN, Braga TF, Marques MG, Vargas LN, de Faria OAC, de Souza AP, Albring D, Caetano AR, Franco MM (2023) Cumulus-oocyte complexes from sows show differences in lipid metabolism compared to cumulus-oocyte complexes from prepubertal gilts during in vitro maturation. Molecular Reproduction and Development 90(5), 323-335.
| Crossref | Google Scholar | PubMed |
Tatíčková M, Trebichalská Z, Kyjovská D, Otevřel P, Kloudová S, Holubcová Z (2023) The ultrastructural nature of human oocytes’ cytoplasmatic abnormalities and the role of cytoskeleton dysfunction. F&S Science 4(4), 267-278.
| Crossref | Google Scholar |
Trebichalska Z, Kyjovska D, Kloudova S, Otevrel P, Hampl A, Holubcova Z (2021) Cytoplasmic maturation in human oocytes: an ultrastructural study. Biology of Reproduction 104(1), 106-116.
| Crossref | Google Scholar | PubMed |
Van Blerkom J (1990) Occurrence and developmental consequences of aberrant cellular organization in meiotically mature human oocytes after exogenous ovarian hyperstimulation. Journal of Electron Microscopy Technique 16(4), 324-346.
| Crossref | Google Scholar | PubMed |
Wall RJ, Hawk HW (1988) Development of centrifuged cow zygotes cultured in rabbit oviducts. Reproduction 82(2), 673-680.
| Crossref | Google Scholar | PubMed |
Wall RJ, Pursel VG, Hammer RE, Brinster RL (1985) Development of porcine ova that were centrifuged to permit visualization of pronuclei and nuclei. Biology of Reproduction 32(3), 645-651.
| Crossref | Google Scholar | PubMed |