The role of placental kisspeptin in trophoblast invasion and migration: an assessment in Kiss1r knockout mice, BeWo cell lines and human term placenta
E. N. Panting A # , J. H. Weight A # , J. A. Sartori


A
B
Handling Editor: Ellen Menkhorst
Abstract
There is mounting evidence implicating kisspeptin signalling in placental development and function.
This study aimed to elucidate kisspeptin’s role in trophoblast invasion and migration using three experimental models.
First, we examined the mouse fetus and placenta in a kisspeptin receptor (Kiss1r) knockout (KO) model. Fetal/placental weights and gene expression (quantitative polymerase chain reaction) were assessed. Second, we determined kisspeptin effects on a human trophoblast (BeWo) cell line in vitro. Third, we examined KISS1 and KISS1R gene expression in human placenta from term and pre-term pregnancies.
No difference was found in fetal or placental weight between Kiss1r KO and wildtype mice. However, expression of the trophoblast invasion marker, Mmp2 mRNA, was greater in the placental labyrinth zone of Kiss1r KO mice. BeWo cell models of villus cytotrophoblast and syncytiotrophoblast cells exhibited kisspeptin protein expression, with greater expression in syncytiotrophoblast, consistent with KISS1 mRNA. Kisspeptin treatment inhibited the migratory potential of cytotrophoblast-like cells. Finally, while no difference was seen in KISS1 and KISS1R mRNA between term and pre-term placentas, we saw a difference in the relative expression of each gene pre-term. We also observed a positive correlation between KISS1 expression and maternal body mass index.
Our results indicate that kisspeptin may inhibit trophoblast invasion.
Further investigation is required to clarify specific regulatory mechanisms.
Keywords: GPR54, invasion, Kiss1, kisspeptin, migration, placenta, preeclampsia, pregnancy.
Introduction
The placenta is essential for maintaining fetal development in utero. Comprised of both fetal and maternal tissue, it facilitates the exchange of metabolic substrates and waste between the fetal and maternal circulations, while providing a necessary barrier between the two (Reynolds and Redmer 1995; Georgiades et al. 2002). Establishment of the placenta involves the tightly regulated invasion of trophoblast cells into the uterine endometrium and inner third of the myometrium (Bass et al. 1994). Trophoblast migration and invasion differs greatly among species. The murine placenta is far less invasive than the human placenta, with the extravillous trophoblast equivalents not invading and widening the spiral arteries of the murine placenta as they do in the human placenta (Georgiades et al. 2002). Various hormones and cellular mechanisms are involved in regulating placental invasion, and disruption to these regulatory mechanisms can lead to pregnancy pathologies such as preeclampsia (Bischoff et al. 2000; Steegers et al. 2010).
A potential regulator of placental invasion and proliferation is the neuropeptide kisspeptin. Originally discovered as a metastasis inhibitor (Lee et al. 1996), kisspeptin refers to a number of small peptides, each with a common C-terminal sequence and a final 10-amino acid (kisspeptin-10) sequence vital for receptor signalling. Kisspeptin is best known as a hypothalamic regulator of the neuroendocrine reproductive axis (de Roux et al. 2003; Seminara et al. 2003; Gottsch et al. 2004). Attention has turned towards kisspeptin’s presence and role beyond the hypothalamus, particularly in the placenta. Interest was generated following the discovery of high levels of endogenous kisspeptin and its receptor KISS1R in placental trophoblast cells (Horikoshi et al. 2003; Bilban et al. 2004), which are directly involved in inhibiting cellular invasion, migration and angiogenesis (Gomes and Sones 2021). Development of the placental vascular network is tightly regulated, and disruption to regulatory factors often results in placental pathologies. There is mounting evidence implicating kisspeptin signalling in placental development (Gomes and Sones 2021). Kisspeptin has been implicated in a number of antenatal complications (Hu et al. 2019; Tsoutsouki et al. 2022), specifically hypertensive disorders of pregnancy and pre-term birth (Abbara et al. 2022).
Prior studies have investigated the effects of kisspeptin in the placenta using transgenic mouse models. Expressions of Kiss1 mRNA and kisspeptin peptide are upregulated in the maternal-fetal interface of a genetic mouse model of preeclampsia (Gomes et al. 2022). However, no direct kisspeptin mechanism of action or functional role have been established (Herreboudt et al. 2015). This may be due to Kiss1 knockout (KO) placentas still having active kisspeptin receptors and kisspeptin from neighbouring wildtype (WT) placentas reaching these placentas. Our study initially aimed to examine murine placental development in the absence of Kiss1r signalling by assessing fetal and placental weight parameters, as well as the relative expression of key genes involved in trophoblast invasion and vascularisation. Given kisspeptin’s role in metastasis inhibition, it was hypothesised that in the Kiss1r KO mouse placenta there would be an increase in trophoblast invasion and vascularisation, suggesting a physiological role of kisspeptin in placental development. Our study also aimed to investigate the effect of kisspeptin on the placenta via an in vitro model. An immortal trophoblast cell line of human origin, BeWo cells, are a validated model of undifferentiated placental villus trophoblast cells that undergo differentiation in a manner similar to in vivo trophoblasts (Pattillo and Gey 1968; Wice et al. 1990). We aimed to determine endogenous kisspeptin expression and investigate whether incubation with kisspeptin or a kisspeptin antagonist would alter BeWo cellular migration and genetic markers for invasion and/or angiogenesis. We hypothesised that kisspeptin treatment would restrict BeWo cellular migration and this would be attenuated by paired antagonist treatment. Our final aim was to examine KISS1 and KISS1R gene expression in placentas from women following term or pre-term birth. Placental KISS1 expression has been shown to be higher in pre-term delivery than in term delivery, and within cases of preeclampsia (Bilban et al. 2004; Torricelli et al. 2008; Cartwright and Williams 2012; Abbara et al. 2022). However, other studies have shown no difference (Janneau et al. 2002). In alignment with the majority of studies, we hypothesised that KISS1 and KISS1R expression would be higher in pre-term pregnancies.
The purpose of these experiments was to elucidate the role of kisspeptin in human placental development and determine its potential mechanism of action. In furthering our knowledge of placental development, the clinical application of findings from these studies will assist in the characterisation of placental pathologies such as preeclampsia.
Materials and methods
Experiment 1: placental development in the absence of Kiss1r in mice
We used Kiss1r KO mice, as described previously (Tolson et al. 2014). This mouse line has a C57B1x129S1/SVImJ background generated by Omeros Corporation (Seattle, WA, USA) via retroviral mutagenesis. All animal procedures were approved by the Animal Ethics Committee of The University of Western Australia, within the National Health and Medical Research Council (NHMRC) guidelines for animal experimentation. Mice had ad libitum access to chow and water, and were maintained under a 12 h light/dark cycle (lights on at 0700 h). Using standard colony mating procedures, female Kiss1r heterozygotes (Het) in proestrus were paired overnight with Kiss1r Het males, to generate pregnancies carrying feto-placental units of all three possible genotypes (WT, Het, KO). Only WT and KO were used for our experiments. In the morning, females were assessed for a copulatory plug, indicative of pregnancy, and designated as embryonic day 1 (E1) of pregnancy.
Pregnant mice were anaesthetised by isofluorane inhalation on embryonic day 14 (E14) for tissue collection. All fetal and placental pairs were removed from the uterus and weighed (with means representing a sex or genotype if more than one was present). Placentas were dissected into the junctional and labyrinth zones, weighed, snap frozen in liquid nitrogen, and stored at −80°C for quantitative reverse-transcription polymerase chain reaction (qRT-PCR) analyses. Genomic DNA (gDNA) was isolated from fetal tail tips for genotyping and sex confirmation (PCR analysis for the presence or absence of the Sry gene).
Experiment 2: the effect of kisspeptin on BeWo human trophoblast gene expression and cell migration
BeWo cells were sourced from the American Type Culture Collection (Manassas, VA, USA) and cultured in a 75 cm2 flask with Dulbecco’s Modified Eagle’s medium (DMEM)/Nutrient Mixture F-12 (F12K) media (50:50 mixture; Thermo-Fisher Scientific, Scoresby, Vic, Australia), 10% fetal calf serum (FCS; Sigma-Aldrich, Sydney, NSW, Australia) and 1% penicillin/streptomycin (Sigma-Aldrich) in a 37°C/5% CO2 incubator. BeWo cultures were incubated for 48 h with either 0.1% dimethyl sulfoxide (DMSO, vehicle control) or 60 μM forskolin (Sigma-Aldrich) in vehicle to produce either a cytotrophoblast-like or a syncytiotrophoblast-like model, respectively. Syncytialisation of the forskolin-incubated culture was confirmed visually (Masuzaki et al. 1997).
BeWo cells were seeded onto glass coverslips in four 60 mm plates (cell density of 0.2 × 106 cells/well) and, after reaching approximately 60% confluence, were washed in 0.1 M phosphate-buffered solution (PBS). Fixation was performed with a 10 min incubation of 4% paraformaldehyde. After a series of PBS washes, antigen retrieval was performed using heated citrate buffer (pH 6.0). Wells were washed in a blocking serum containing rabbit serum, then incubated in primary antibody GQ2 diluted 1:5000 for three nights at 4°C (GQ2, sheep polyclonal human kisspeptin-54 specific, kindly supplied by Prof. Stephen Bloom, Imperial College, London, UK (Ramaswamy et al. 2010; Smith et al. 2010)). Secondary Alexa488 antibody incubation followed (Thermo Fisher) diluted 1:500 and then Hoechst (1 mg/mL, Thermo Fisher) nucleus staining. Each coverslip was mounted onto slides with anti-fade fluorescence (DAKO, Santa Clara, USA).
Imaging was performed on a Nikon C2+ confocal microscope (Nikon, Sydney, NSW, Australia), and representative images were exported to ImageJ (ver. 1.52, National Institutes of Health (NIH), Bethesda, MD, USA) for measurement of absolute Alexa488 (green) fluorescence. The integrated density was calculated for each image. Relative quantification of kisspeptin expression in each image was determined by dividing the Alexa488 integrated density by the number of nuclei (determined by Hoechst staining, blue). Measuring the number of nuclei accounted for the variable number of cells between images.
BeWo cells were cultured as above. Scratch migration assays were performed on confluent cells in 20 mm plates, with the cells initially seeded at 0.1 × 106 cells/well and treated for 48 h to produce either a syncytiotrophoblast-like or cytotrophoblast-like model. Media was removed from the wells and a ‘scratch’ made by lightly scraping a p10 pipette tip across the well. Wells were subsequently washed in PBS, then replenished with media containing one of the four treatments: DMSO, kisspeptin (1 nM, kisspeptin-10 decapeptide YNWNSFGLRF-NH2, obtained from Phoenix Pharmaceuticals Ltd, Belmont, CA, USA), p271 (1 nM, kisspeptin antagonist synthesised by EZBiolab Inc., Carmel, IN, USA [ac-(D-A)NWNGFG(D-W)RF-penetratin-NH2] (Roseweir et al. 2009; Smith et al. 2011)) or kisspeptin + p271 (as above). Images immediately post-scratch (0 h) were taken with a Leica DM IL LED inverted light microscope (Leica Microsystems, Macquarie Park, NSW, Australia). A second set of images was taken 18 h later of the same section of the scratch. Two images were taken of each scratch/well and averaged across five replicate wells for each experimental condition. Scratch width at 0 and 18 h was determined using the ImageJ ROI function (NIH) at 12 parallel points of the image, between any migratory cells and the opposite side of the scratch. The widths within the image were compared with the corresponding width at the same point at 0 h to create a proportional unit.
Experiment 3: KISS1 and KISS1R gene expression in term and pre-term placentas
All participants in this study gave informed written consent before participating. The tissue collection protocol was approved by the Human Research Ethics Committees at Edith Cowan University (ECU). Pregnant women were recruited from participating hospitals in Perth, WA, Australia, via the Placenta Project (ECU). Clinical characteristics for each patient were recorded, including maternal age (years), maternal body mass index (BMI), gestational age (weeks), and fetal birth weight (g) (Nelson and Burton 2011). After gross morphological examination, each placenta was sectioned into four quadrants and a tissue sample was extracted via an 8-mm punch biopsy. Biopsies were removed from the maternal surface and consisted of maternal and fetal tissue. For this study, samples were sourced from the left lower quadrant. The samples were cryopreserved using liquid nitrogen and stored at −80°C.
Analysis of placental gene expression
For all experiments, total RNA was isolated for qRT-PCR using QIAzol (Qiagen, Venlo, Limburg, Netherlands) in accordance with the manufacturer’s protocol and converted to cDNA via reverse transcription (Promega, Sydney, NSW, Australia). PCR was performed with Qiagen SYBR Green PCR Kit (Qiagen) in duplicate using a Corbett Rotor-Gene 6000 (Corbett Industries, Sydney, NSW, AU). QuantiTect primer assays were used according to the manufacturer’s instructions (Qiagen; Table 1). Samples were compared to a standard curve (10-fold dilution), and relative gene expression was normalised using the GENorm algorithm to the reference genes peptidylpropyl isomerase A (Ppia), succinate hydrogenase (Sdha), and TATA box binding protein (Tbp) for Experiment 1, and glyceraldehyde-3-phosphate dehydrogenase (GAPDH) for Experiments 2 and 3. No significant differences in reference genes were found.
Gene | QuantiTect primer assay detail | NCBI reference sequence | Cat. no. | Product size | |
---|---|---|---|---|---|
Mmp2 | Mm_Mmp2_1_SG | NM_008610 | QT00116116 | 145 bp | |
Timp3 | Mm_Timp3_1_SG | NM_011595 | QT00105469 | 131 bp | |
Vegfa | Mm_Vegfa_1_SG | NM_001025250 | QT00160769 | 117 bp | |
Pgf | Mm_Pgf_1_SG | NM_008827 | QT00103222 | 79 bp | |
Slc38a4 | Mm_Slc38a4_1_SG | NM_027052 | QT00146769 | 72 bp | |
Slc2a3 | Mm_Slc2a3_1_SG | NM_011401 | QT00159691 | 110 bp | |
KISS1 | Hs_KISS1_1_SG | NM_002256 | QT00016044 | 130 bp | |
KISS1R | Hs_KISS1R_1_SG | NM_032551 | QT00043134 | 113 bp | |
MMP2 | Hs_MMP2_1_SG | NM_004530 | QT00088396 | 95 bp | |
TIMP3 | Hs_TIMP3_1_SG | NM_000362 | QT00046382 | 105 bp | |
VEGFA | Hs_VEGFA_1_SG | NM_001025366 | QT01010184 | 273 bp | |
PGF | Hs_PGF_1_SG | NM_001207012 | QT00030688 | 130 bp | |
GAPDH | Hs_GAPDH_1_SG | NM_002046 | QT00079247 | 95 bp |
Gene | Geneworks forward primer | Geneworks reverse primer | Product size | |
---|---|---|---|---|
mPpia | AGCATACAGGTCCTGGCATC | TTCACCTTCCCAAAGACCAC | 127 bp | |
mSdha | TGGGGAGTGCCGTGGTGTCA | CTGTGCCGTCCCCTGTGCTG | 149 bp | |
mTbp | GGGAGAATCATGGACCAGAA | CCGTAAGGCATCATTGGACT | 113 bp |
Statistical analysis
All statistical analysis was conducted using GraphPad Prism (GraphPad Software Inc., La Jolla, CA, USA). Overall genotype distribution of the Kiss1r KO mouse colony was examined for Mendelian ratio (WT, 25%; Het, 50%; KO, 25%) using Chi-square analysis in Microsoft Excel. Data in Experiment 1 were analysed via two-way ANOVAs. Significant genotype or sex effects were further analysed using Fisher’s least-significant-difference (l.s.d.) post hoc test. For Experiment 2, BeWo cell kisspeptin immunofluorescence was examined by unpaired t-test. Scratch migration assay data and gene expression data were examined by two-way ANOVAs. Arcsine transformation was used on cell migration ratio data to account for non-normality. In Experiment 3, gene expression was compared by unpaired t-test and data for KISS1 mRNA was log-transformed to account for non-normality. The relationship between variables was determined by Pearson correlation and simple linear regression. All statistical significance was determined at the level of P < 0.05.
Results
Experiment 1: placental development in the absence of Kiss1r in mice
Chi-square analysis revealed a non-Mendelian distribution of genotypes for the entire Kiss1r KO colony with numbers lower than expected in KO mice (total numbers and frequency: WT 361, 27%; Het 696, 52%; KO 282, 21%; P < 0.01). No significant differences were observed in fetal weight or placental weight between WT and Kiss1r KO mice (Fig. 1a, b, respectively), and no sex differences were observed. The relative frequency of fetal weight distribution was further tested with no clear change between WT and and Kiss1r KO mice (sexes combined, see Supplementary Fig. S1). Junctional and labyrinth zone weights, along with fetal/placental weight ratio and labyrinth/junctional zone weight ratio, were also similar between WT and KO placentas (Fig. S1).
Fetal weight (a), placental weight (b), placental junctional zone weight (c), placental labyrinth zone weight (d), in wild-type (WT) and Kiss1r knockout (KO) mice at E14. Data are presented as the mean ± s.e.m. for male and female mice (n = 6–8 for all groups). Fetal weights were assessed to evaluate placental functional capacity. Data were analysed using two-way ANOVAs; no significant differences were observed.
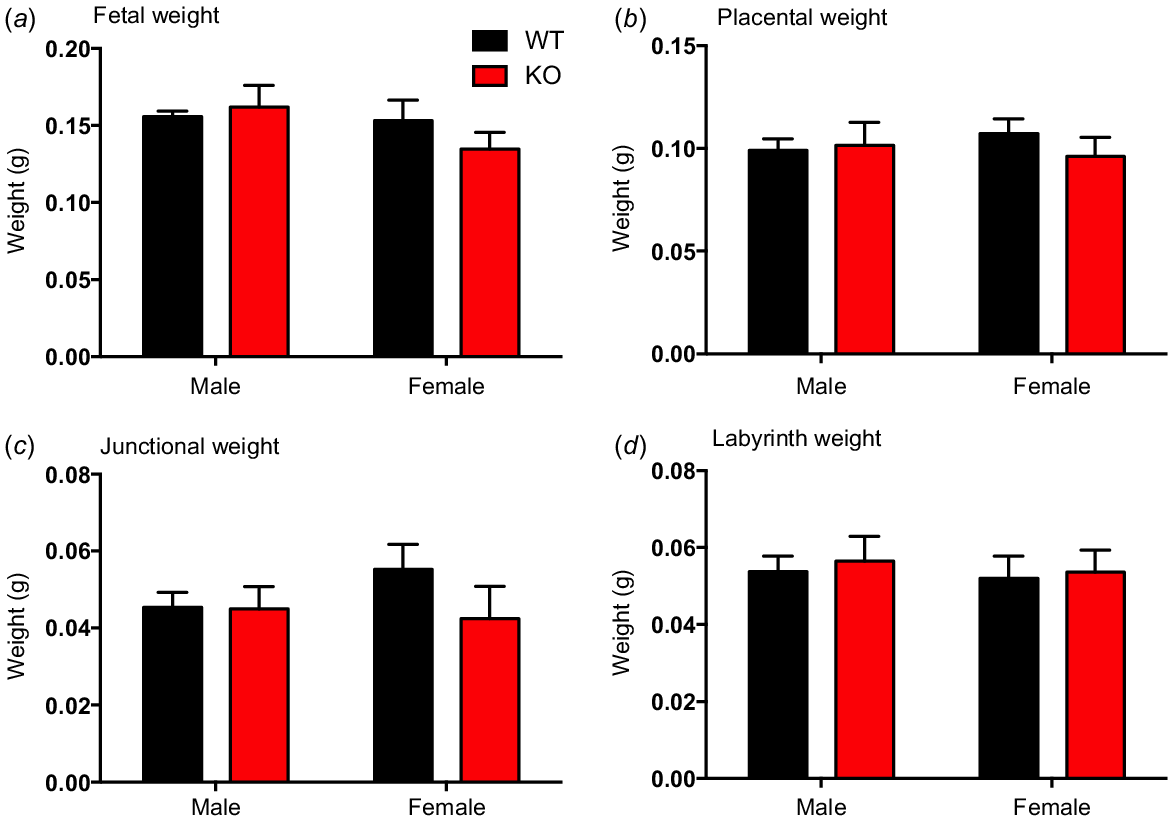
Genetic markers of trophoblast invasion, Mmp2 and Timp3, were assessed. Mmp2 mRNA expression did not differ with genotype or sex in the placental junctional zone (Fig. 2a). However, in the labyrinth zone, Mmp2 mRNA expression was greater in Kiss1r KO placentas compared to WT placentas in females (P < 0.05, Fig. 2b). Timp3 mRNA expression in the junctional zone was lower in Kiss1r KO placentas compared to WT placentas in females (P < 0.05, Fig. 2c). There were no differences in Timp3 mRNA expression in the labyrinth zone of male or female placentas between genotype (Fig. 2d).
Relative gene expression of Mmp2 (a, b), Timp3 (c, d), Vegfa (e, f) and Pgf (g, h) in the labyrinth and junctional zones of wild-type (WT) and Kiss1r knockout (KO) placentas at E14. Data are presented as the mean ± s.e.m. for male and female mice (n = 6–8 for all groups). Data were analysed using two-way ANOVAs, followed by Fisher’s l.s.d. post hoc test upon significance; *P < 0.05.
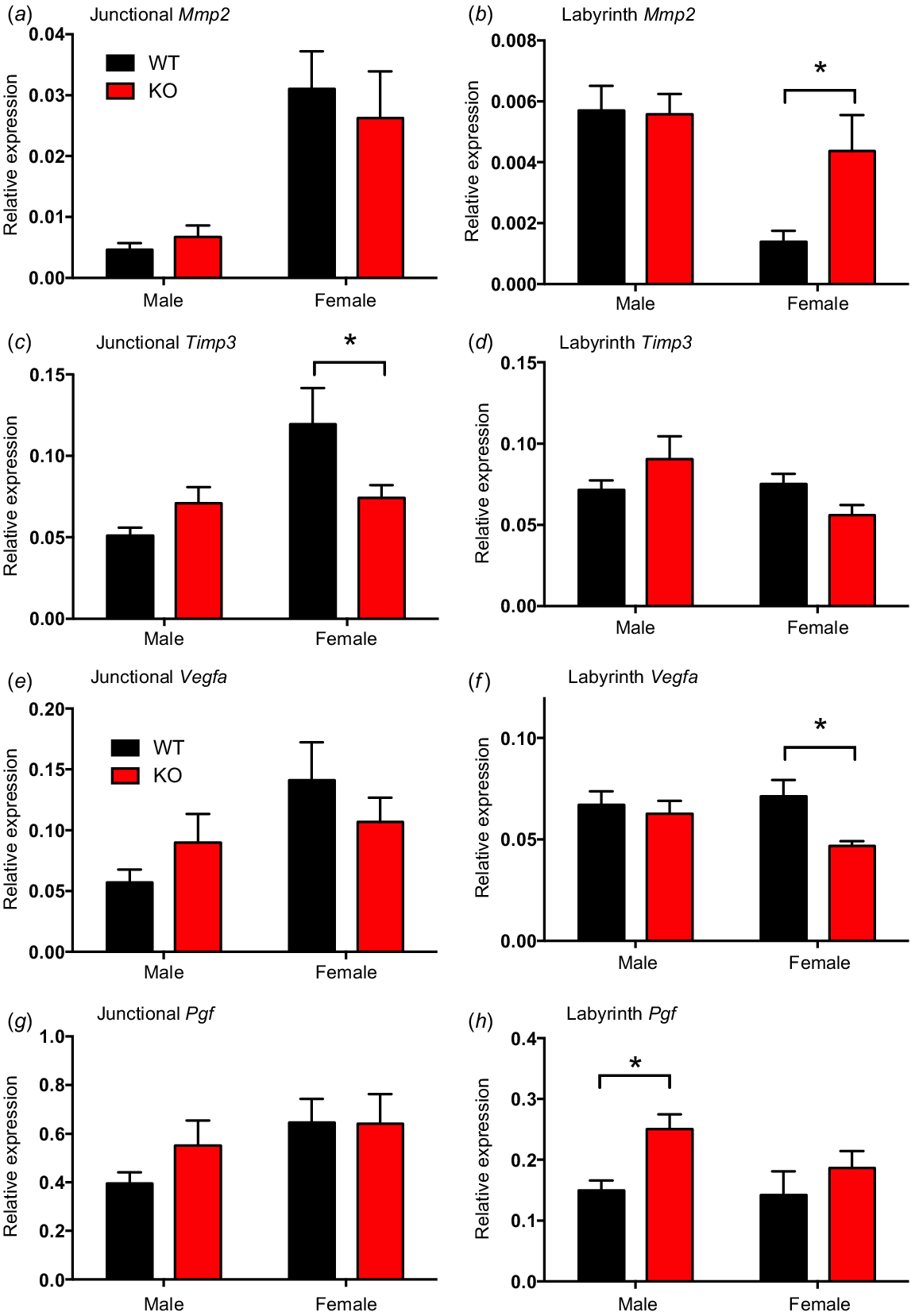
Expression of the placental vascularisation factor Vegfa mRNA did not differ between genotype or sex in the placental junctional zone (Fig. 2e). In the labyrinth zone, Vegfa mRNA expression was lower in Kiss1r KO placentas compared to WT placentas in females (P < 0.05, Fig. 2f). Placental growth factor Pgf mRNA expression did not differ with genotype or sex in the placental junctional zone (Fig. 2g). In the labyrinth zone, Pgf mRNA expression was greater in Kiss1r KO placentas compared to WT placentas in males (P < 0.05, Fig. 2h), with no change observed between genotypes in females.
Relative mRNA expression of the amino acid transporter Slc38a4 (SNAT4) and the glucose transporter Slc2a3 (GLUT3) were similar between genotype and sex in both the placental junctional and labyrinth zones (Fig. S2).
Experiment 2: the effect of kisspeptin on BeWo human trophoblast gene expression and cell migration
The syncytiotrophoblast-like BeWo model exhibited a significantly higher density of kisspeptin immunoreactivity than the cytotrophoblast-like model (Fig. 3). Density measurement of relative kisspeptin expression, accounting for variable cell numbers per image, indicated that kisspeptin expression is approximately 20 times greater in the syncytiotrophoblast-like model than the cytotrophoblast-like model (P < 0.001, Fig. 3g).
Representative fluorescent images of cytotrophoblast-like (a–c) and syncytiotrophoblast-like (d–f) BeWo cultures. Blue fluorescence represents nuclear staining (Hoescht) and green fluorescence represents kisspeptin staining. Images C and D are from no-antibody controls. Scale bars = 200 μm. (g) Comparison of kisspeptin staining (Alexa488/Hoescht ratio) between the cytotrophoblast-like and syncytiotrophoblast-like models. The Alexa488/Hoescht ratio is a measurement of relative kisspeptin expression, accounting for a variable cell number per image. Data are presented as the mean ± s.e.m. (n = 4 per group). Data were analysed using Student’s unpaired t-test; ***P < 0.001.
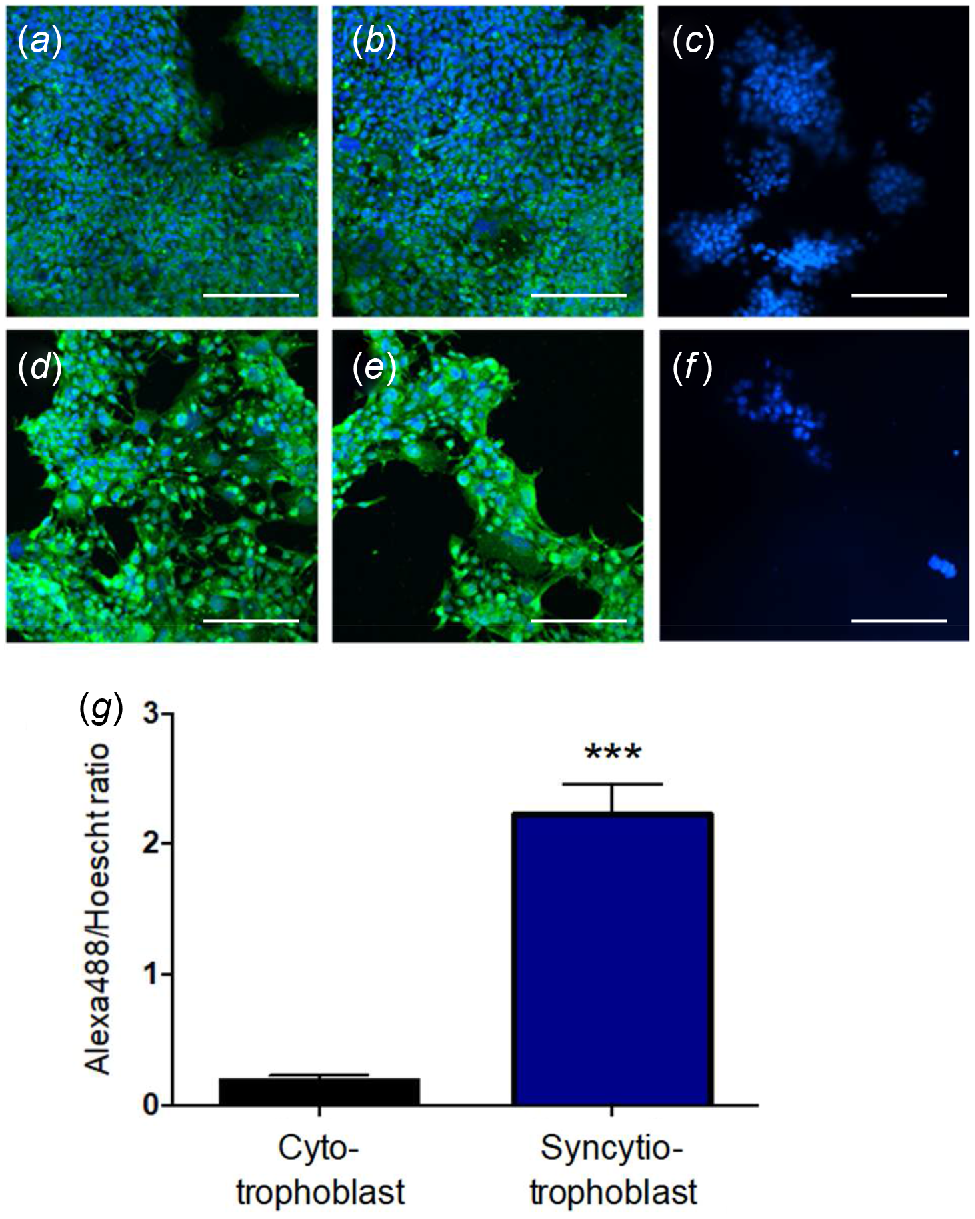
Scratch migration assays (SMAs) were performed as a measurement of kisspeptin’s effect on trophoblast migration (Fig. 4). There was a significant overall treatment effect on cell migration ratio in both the cytotrophoblast-like and the syncytiotrophoblast-like models (P < 0.01). There was no overall significant difference between trophoblast models. Specifically, within the cytotrophoblast, kisspeptin treatment inhibited scratch narrowing relative to other treatments (P < 0.05, Fig. 4c, d, i). Co-treatment with kisspeptin antagonist restricted the kisspeptin effect with values similar to control (Fig. 4g–i). No specific treatment effects were seen in the syncytiotrophoblast-like models (Fig. 4i).
Representative images of scratch migration assays in cytotrophoblast-like BeWo cultures at 0 h (a, c, e, g) and 18 h post scratch (b, d, f, h). BeWo cultures were treated with vehicle, kisspeptin, kisspeptin antagonist (p271), or both kisspeptin and p271. Scale bars = 200 μm. Cell migration ratios of scratch width at 18 h relative to 0 h in treatment groups and BeWo models (i). Kisspeptin treated cytotrophoblast-like BeWo cells had impaired narrowing of the scratch relative to other treatments. Data are presented as the mean ± s.e.m. (n = 5 per group). Data were analysed using two-way ANOVAs, followed by Fisher’s l.s.d. post hoc test; *P < 0.05.
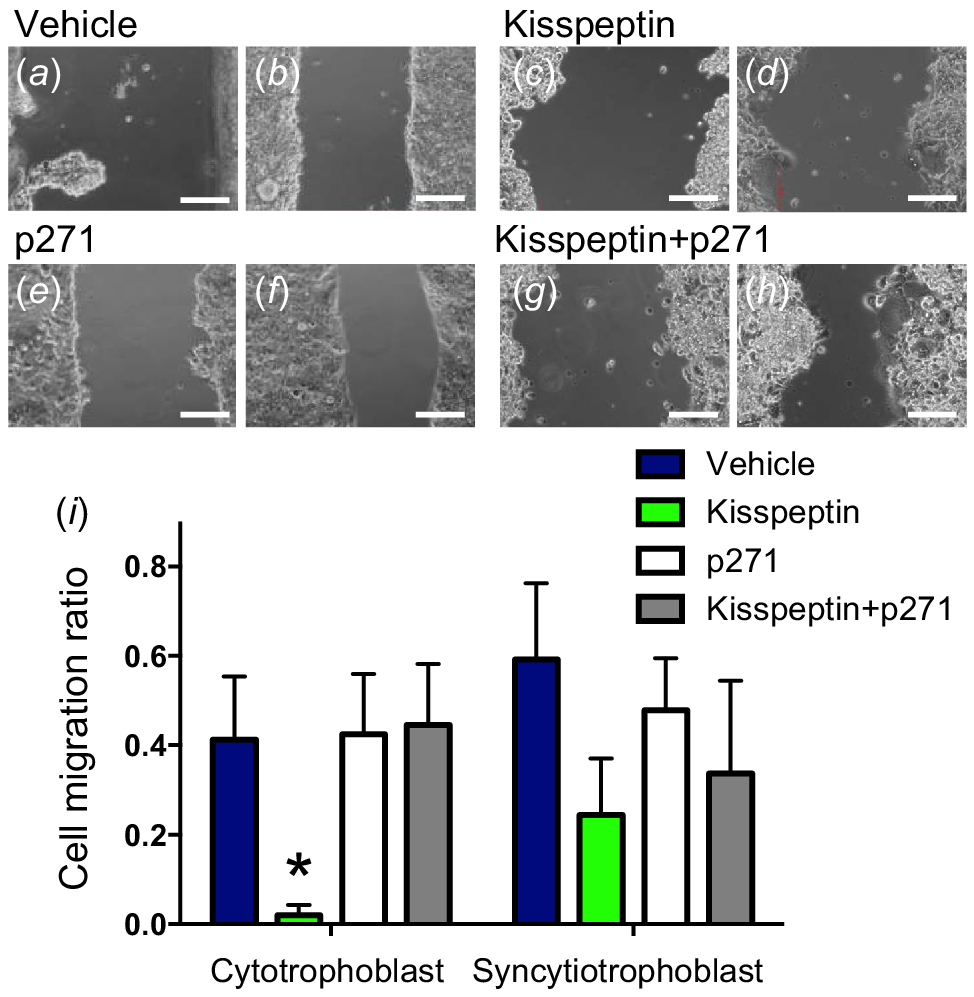
Analysis of gene expression (relative to the GAPDH reference gene) in treatment groups for both trophoblast models was performed. There were no significant differences between treatment groups in all genes examined. However, overall differences between trophoblast models were observed, with KISS1, TIMP3 and VEGFA all significantly greater in the syncytiotrophoblast model compared to the cytotrophoblast model (P < 0.05, Fig. 5).
Relative gene expression of KISS1 (a), KISS1R (b), MMP2 (c), TIMP3 (d), VEGFA (e) and PGF (f) in BeWo culture scratch migration assay treatment groups. KISS1 and VEGFA mRNA expression were significantly higher in the syncytiotrophoblast-like model compared to the cytotrophoblast-like model, but no treatment effects were observed. Data are presented as the mean ± s.e.m. (n = 4 per group). Data were analysed using two-way ANOVAs; *P < 0.05.
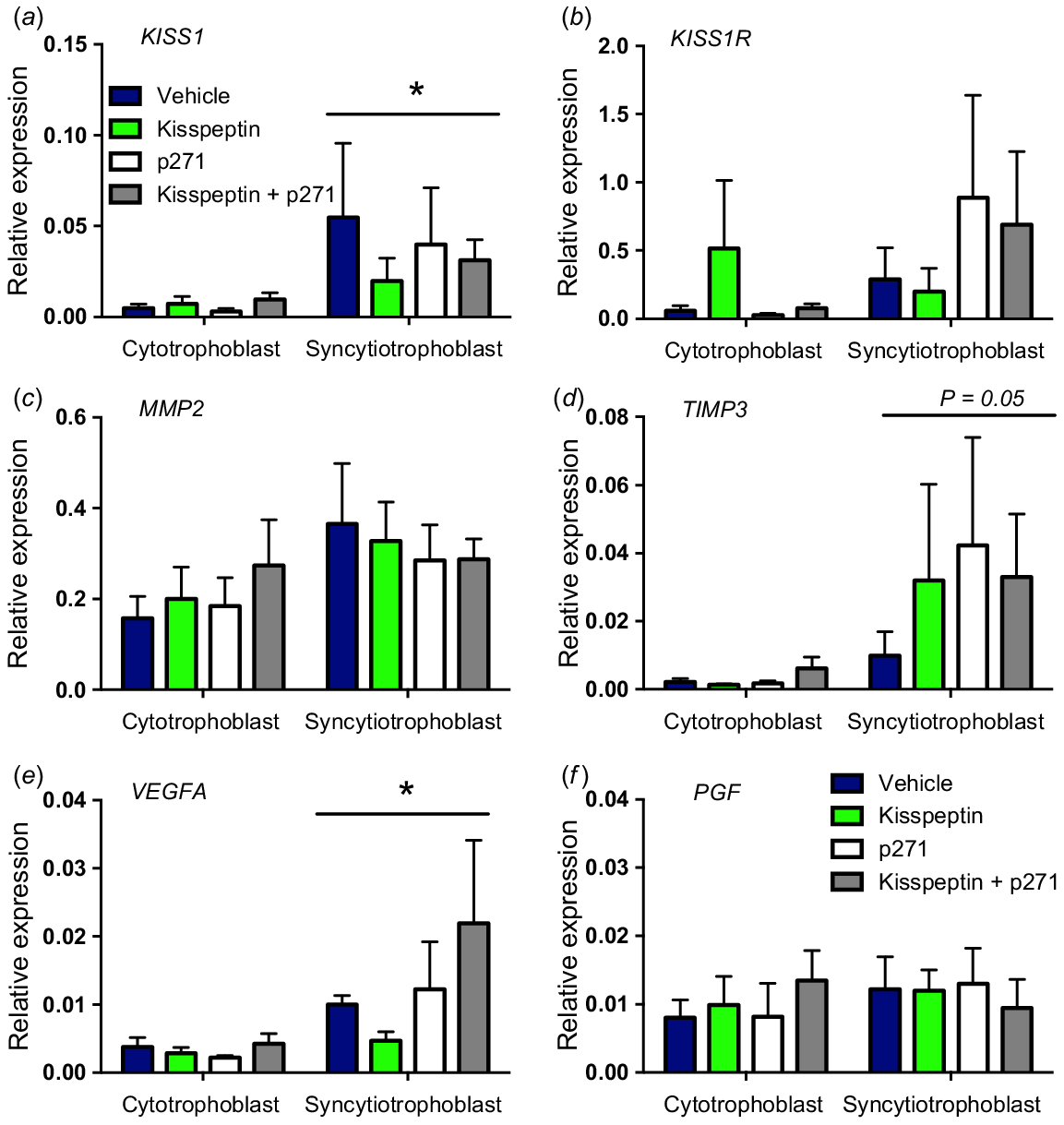
Experiment 3: expression of KISS1 and KISS1R in human term and pre-term placenta
Clinical pregnancy and perinatal characteristics are summarised in Table 2. No significant change was seen in relative gene expression for KISS1 or KISS1R between term and pre-term placenta (Fig. 6a, b, respectively). A non-significant increase in KISS1 expression was seen in the pre-term placenta (P = 0.06), and so the relative expression between KISS1 and KISS1R was examined (Fig. 6c). Positive correlations were observed between KISS1 and KISS1R in both term (R2 = 0.82; P < 0.001) and pre-term (R2 = 0.66; P < 0.01) placenta and the relationship between the two also differed significantly (P < 0.05). Here, a higher paired expression of KISS1 relative to KISS1R was seen in the pre-term placenta.
Term | Pre-term | |||
---|---|---|---|---|
N | 10 | 10 | ||
Gestational age (weeks) | 39.7 ± 0.2 | 36.2 ± 0.1 | *** | |
Maternal BMI | 25.8 ± 4.4 | 27.8 ± 6.3 | n.s. | |
Birth weight (g) | 3544 ± 113 | 2640 ± 294 | *** | |
Maternal age (years) | 27.9 ± 1.6 | 30.8 ± 1.8 | n.s. |
n.s., not significant.
***P < 0.001. Values are displayed as mean ± s.e.m.
Relative gene expression of KISS1 (a) and KISS1R (b) in term and pre-term human placentas. Data are presented as the mean ± s.e.m. (n = 10 per group). Data were analysed using Student’s unpaired t-test. The relationship between placenta KISS1 and KISS1R mRNA expression (c) and between each gene and gestation age (d, e) and maternal BMI (f) was determined by Pearson correlation and simple linear regression with R2 and P values noted where relevant. The dashed line (f) indicates a positive correlation between placental KISS1 mRNA expression and maternal BMI in both term and pre-term pregnancies.
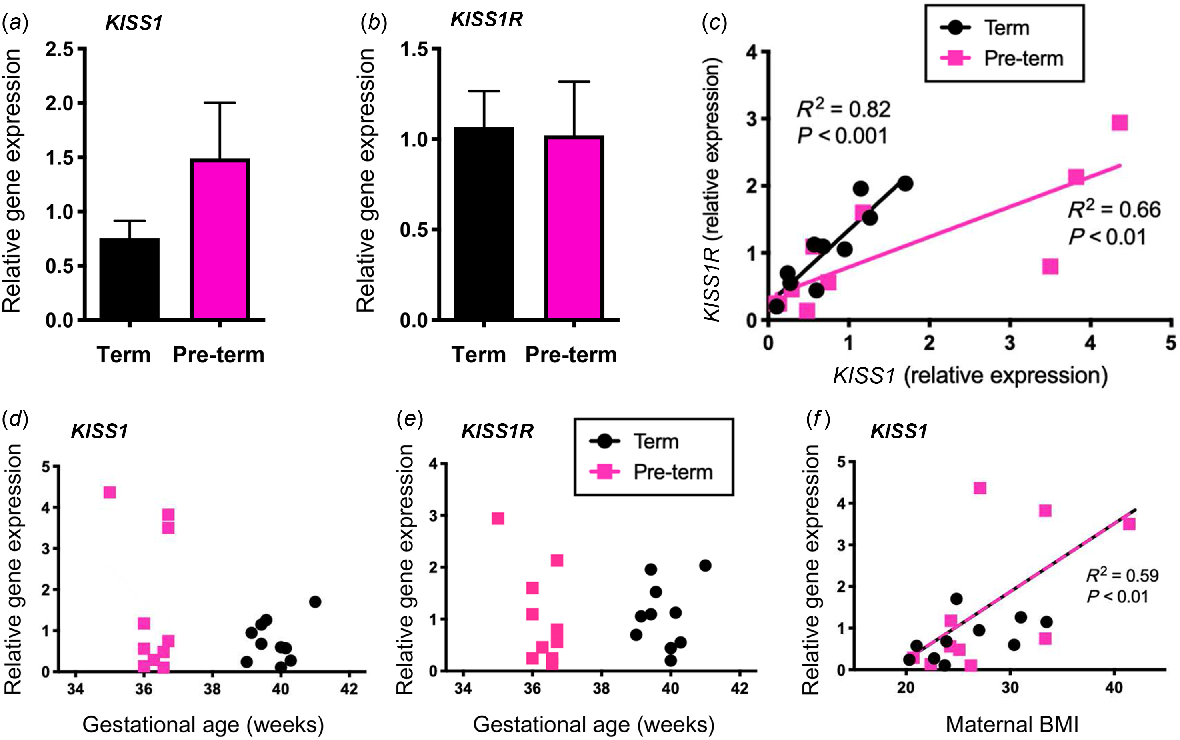
Placental KISS1 and KISS1R gene expression was also examined relative to clinical characteristics for each patient. A non-significant increase for increased KISS1R expression with decreasing gestational age in pre-term placentas was observed (P = 0.06, Fig. 6e). Furthermore, a non-significant positive correlation was seen between KISS1 and maternal body mass index (BMI) in pre-term placentas (P = 0.06, Fig. 6f). When grouping both term and pre-term placentas, the correlation between BMI and relative KISS1 gene expression was significant (R2 = 0.59; P < 0.01). No significant correlation of placental KISS1 or KISS1R gene expression was noted with birth weight or maternal age (Fig. S3).
Discussion
An increasing body of evidence suggests a fundamental role for kisspeptin in placental development and function. Despite this, there remains a limited understanding regarding the mechanisms underlying this regulation. Moreover, there is uncertainty whether animal models, particularly the mouse, adequately replicate the potential role for kisspeptin in human placental function. In this study, we observed no difference in fetal or placental weight between Kiss1r KO and WT mice. We did however see a small but significant shift in the expected Mendelian distribution of KO mice (lower than the expected 25%) and changes in genetic markers of invasion and vascularisation, suggesting a more subtle phenotype in Kiss1r KO placental function. In human trophoblast derived BeWo cells, we confirmed the expression of kisspeptin and observed a reduction in the migratory potential of cytotrophoblast-like cells when treated with kisspeptin. In human placenta, we saw no change in KISS1 or KISS1R mRNA expression between term and pre-term placentas. However, the relationship between the two differed and an overall positive correlation was noted in KISS1 and maternal BMI.
Expression of the kisspeptin protein, Kiss1 mRNA and Kiss1r mRNA have been confirmed in the mouse placenta (Zhang et al. 2014; Herreboudt et al. 2015). Despite the potential regulatory role for kisspeptin in placental development, particularly in the context of invasion and vascularisation, recent findings by Herreboudt and colleagues (Herreboudt et al. 2015) have cast doubt over these claims. Here, it was observed that in the absence of kisspeptin synthesis (Kiss1 KO), there was no detrimental effect on placental development, rendering the kisspeptin regulatory mechanism non-significant in mouse placentation. However, we propose that their experimental method (Kiss1 Het x Het mating) is potentially flawed due to hormone diffusion through the amniotic fluid and the maternal bloodstream (vom Saal 1989; Ryan and Vandenbergh 2002). Thus, Kiss1 KO placentas still had active kisspeptin receptors that may be activated by kisspeptin synthesised from neighbouring Kiss1 WT and Kiss1 heterozygous littermates. Alternatively, successfully mated female Kiss1 KO mice fail to achieve pregnancy due to defects in embryo implantation (Calder et al. 2014). This is indicative of a maternal requirement for kisspeptin signalling in implantation, with functional kisspeptin expressed in the luminal and glandular epithelia of the uterus (Fayazi et al. 2015). Moreover, rescue of Kiss1r in the hypothalamus of Kiss1r KO mice fails to restore endometrial gland formation (Leon et al. 2016), further emphasising the critical role for uterine kisspeptin signalling in endometrial gland development and function. While informative, data from these mouse models is unable to provide information on placental kisspeptin signalling.
The Kiss1r KO mouse model was selected in our study as most appropriate, as it eliminates the possibility of kisspeptin synthesis from neighbouring littermates being recognised by the KO fetus and placenta. Our evidence suggests kisspeptin receptor signalling does not play a crucial role in placental growth or development. There was no difference in E14 fetal weight or placental weight between the Kiss1r KO mice and their WT littermates. Despite this, we did see a reduction in the expected Mendelian ratio for KOs in the Kiss1r KO colony, suggestive of an early trophoblast invasion phenotype potentially causing selective loss of Kiss1r KO fetuses. Differences were also observed in the placental marker of trophoblast invasion Mmp2 with mRNA expression greater in the female KO labyrinth zone indicating increased promotion of invasion. Matrix metalloproteinases (MMPs) are tissue remodelling molecules that are concomitant with the invasive phenotype of trophoblast cells (Librach et al. 1991; Cohen et al. 2006). Interestingly, we also saw a decrease in female KO junctional zone Timp3 mRNA. To control the invasive capacity of trophoblast cells, the activity of MMPs is tightly regulated by inhibitory effects of TIMPs (endogenous tissue inhibitors of MMPs). These results are consistent with the proposed role for kisspeptin as a placental invasion inhibitor (Bilban et al. 2004) and a repressor of MMPs (Yan et al. 2001).
The expression of Vegfa mRNA was significantly reduced in the labyrinth zone of female Kiss1r KO mice at E14. This result was unexpected as previously kisspeptin has been shown to down-regulate vascular endothelial growth factor (VEGF) gene expression in primary cultures of first trimester human trophoblast cells, limiting vascularisation and angiogenesis of the placenta (Francis et al. 2014). Alternatively, in the absence of Kiss1r signalling, Pgf mRNA expression was significantly increased in the male labyrinth zone, suggestive of increased vascularisation. Placental growth factor (PGF) is a member of the VEGF family and a critical mediator of angiogenesis during placental development as it promotes remodelling of the uterine vasculature (Osol et al. 2008; Hoffmann et al. 2013). Upregulation of Pgf mRNA expression in the Kiss1r KO male labyrinth zone is consistent with our expectations and is indicative of increased vascularisation in the absence of kisspeptin signalling. However, regulation in this manner likely exhibits elements of sexual dimorphism, as these effects were only apparent in placentas of one sex. Moreover, these subtle changes observed in gene expression led us to believe that subtle changes in placental development may also exist. Further investigation by stereology and placental vascular casting may determine whether the observed changes in gene expression are reflective of fine structural differences. Striking differences in the structure of placental vasculature have previously been observed without recording significant changes in placental weight or volume (Rennie et al. 2015).
The second aim of this study was to determine whether kisspeptin and kisspeptin receptor are expressed in the BeWo cell culture, and if there was a change in expression between the cytotrophoblast-like and the syncytiotrophoblast-like model. Expression of kisspeptin peptide and KISS1 mRNA in the syncytiotrophoblast-like model was significantly higher compared to the cytotrophoblast-like model. No significant differences were found in KISS1R mRNA expression. This is consistent with studies in both placental samples and primary trophoblast cultures (Bilban et al. 2004; Park et al. 2012). Furthermore, using a scratch migration assay, kisspeptin treatment inhibited cytotrophoblast-like cellular migration, with no effect of kisspeptin treatment in the syncytiotrophoblast-like culture. Importantly, the effect of kisspeptin was prevented by co-treatment with a kisspeptin antagonist. Such an effect may indicate a paracrine role for kisspeptin in the syncytiotrophoblast (where there is greater expression) on the cytotrophoblast (where there is lower endogenous kisspeptin produced but a greater effect on migration). One limitation of using BeWo cell culture is that these cells model villus trophoblast, thereby preventing direct inferences on trophoblast invasion. Also it is unclear whether closure of the scratch migration assay is due to cell migration or proliferation closing the gap. A significant inhibition in cell migration following kisspeptin treatment has been shown using human villous explant and primary trophoblast cell cultures (Bilban et al. 2004; Francis et al. 2014) and is consistent with the established anti-metastatic properties of kisspeptin (Ohtaki et al. 2001).
Kisspeptin antagonist treatment did not augment scratch narrowing in the cytotrophoblast-like or the syncytiotrophoblast-like BeWo models. The kisspeptin antagonist is a competitive antagonist binding to KISS1R without activating it (Roseweir et al. 2009). These results indicate that the antagonist cannot compete with the exogenous kisspeptin applied or endogenous kisspeptin produced in this model. Similarly, neither kisspeptin nor kisspeptin antagonist treatment impacted mRNA expression of KISS1, KISS1R, invasion-related (MMP2, TIMP3) genes or vasculogenic (VEGFA, PGF) genes. This may be due to the high endogenous levels of kisspeptin observed in the BeWo cells. Consistent with our protein expression, KISS1 mRNA expression was greater in the syncytiotrophoblast-like BeWo models as was TIMP3 and VEGFA mRNA. This is potentially consistent with the observed reduction in the expression of these genes in the absence of kisspeptin receptor signalling in the Kiss1r KO mouse placenta.
Given the potential links between kisspeptin and trophoblast invasion, the clinical significance of kisspeptin signalling in preeclampsia and hypertensive disorders of pregnancy are of interest. As indicated above, kisspeptin inhibits trophoblast migration in human villous explant and cell cultures (Bilban et al. 2004; Francis et al. 2014), and also increases extra-villous trophoblast cell adhesion to type-1 collagen (Taylor et al. 2014) preventing migration. Moreover, circulating kisspeptin levels during pregnancy are higher during hypertensive disorders of pregnancy – such as preeclampsia (Abbara et al. 2022), which is linked to suboptimal trophoblast invasion (Khong et al. 1986; Meekins et al. 1994) and pre-term birth (Abbara et al. 2022). Alternatively, our data shows a near-significant result for increased KISS1 mRNA expression in placentas from pre-term pregnancies compared to term pregnancies, while KISS1R expression was similar between the two. Kisspeptin expression was elevated in pre-term placentas (Torricelli et al. 2008), as well as preeclamptic placental tissue (collected post-delivery), with no significant changes in KISS1R levels (Zhang et al. 2011; Qiao et al. 2012; Matjila et al. 2016). It is worth noting that in the Torricelli et al. (2008) study, pre-term placentas were collected on average at 32.3 weeks, defined by the World Health Organization as ‘very pre-term’, compared to 36.1 weeks in our study, defined as ‘moderate pre-term’. We further examined the comparative expression of KISS1 and KISS1R and observed that the ligand and receptor share linearly related expression characteristics – but the nature of this correlation differed significantly between term and pre-term pregnancies. Thus, in pre-term placentas, there appears to be greater KISS1 output relative to KISS1R. Interestingly, our analysis of clinical characteristics for each patient also revealed a significant relationship between maternal BMI and placental KISS1 mRNA expression. This result appeared independent from term and pre-term placentas and may point to a potential link between high maternal BMI and gestational weight gain and the associated risk of pregnancy complications (Santos et al. 2019).
In conclusion, we sought to further our understanding of kisspeptin’s role in the placenta. In mice, only a subtle phenotype was observed in Kiss1r KO placentas in gene expression, and these changes were sex dependant, with no change in placental weight. In BeWo cells, the migratory potential of cytotrophoblast-like cells was restricted when treated with kisspeptin, indicating a functional role. In human placental samples, we found an association between KISS1 and KISS1R mRNA expression that differed between term and pre-term placentas, also noting a relationship between KISS1 and maternal BMI. Thus, if the role of kisspeptin in the placenta is accurately determined, it may be possible to offer novel therapeutic treatments for human placental insufficiency, thus improving pregnancy outcomes.
Data availability
The data that support this study will be shared upon reasonable request to the corresponding author.
Declaration of funding
This research did not receive any specific grant from funding agencies in the public, commercial, or not-for-profit sectors.
Acknowledgements
The authors thank Dr Peter Mark, Dr Caitlin Wyrwoll and Sarah Lovett for cell culture assistance, as well as Dijana Tesic, Dr Cassandra Yap and Dr Rachael Crew for PCR assistance.
References
Abbara A, Al-Memar M, Phylactou M, Daniels E, Patel B, Eng PC, Nadir R, Izzi-Engbeaya C, Clarke SA, Mills EG, Hunjan T, Pacuszka E, Yang L, Bech P, Tan T, Comninos AN, Kelsey TW, Kyriacou C, Fourie H, Bourne T, Dhillo WS (2022) Changes in circulating kisspeptin levels during each trimester in women with antenatal complications. The Journal of Clinical Endocrinology & Metabolism 107, e71-e83.
| Crossref | Google Scholar | PubMed |
Bass KE, Morrish D, Roth I, Bhardwaj D, Taylor R, Zhou Y, Fisher SJ (1994) Human cytotrophoblast invasion is up-regulated by epidermal growth factor: evidence that paracrine factors modify this process. Developmental Biology 164, 550-561.
| Crossref | Google Scholar | PubMed |
Bilban M, Ghaffari-Tabrizi N, Hintermann E, Bauer S, Molzer S, Zoratti C, Malli R, Sharabi A, Hiden U, Graier W, Knofler M, Andreae F, Wagner O, Quaranta V, Desoye G (2004) Kisspeptin-10, a KiSS-1/metastin-derived decapeptide, is a physiological invasion inhibitor of primary human trophoblasts. Journal of Cell Science 117, 1319-1328.
| Crossref | Google Scholar | PubMed |
Bischoff P, Meisser A, Campana A (2000) Paracrine and autocrine regulators of trophoblast invasion – a review. Placenta 21(Suppl A), S55-S60.
| Crossref | Google Scholar |
Calder M, Chan Y-M, Raj R, Pampillo M, Elbert A, Noonan M, Gillio-Meina C, Caligioni C, Berube NG, Bhattacharya M, Watson AJ, Seminara SB, Babwah AV (2014) Implantation failure in female Kiss1-/- mice is independent of their hypogonadic state and can be partially rescued by leukemia inhibitory factor. Endocrinology 155, 3065-3078.
| Crossref | Google Scholar | PubMed |
Cartwright JE, Williams PJ (2012) Altered placental expression of kisspeptin and its receptor in pre-eclampsia. Journal of Endocrinology 214, 79-85.
| Crossref | Google Scholar | PubMed |
Cohen M, Meisser A, Bischof P (2006) Metalloproteinases and human placental invasiveness. Placenta 27, 783-793.
| Crossref | Google Scholar | PubMed |
de Roux N, Genin E, Carel J-C, Matsuda F, Chaussain J-L, Milgrom E (2003) Hypogonadotropic hypogonadism due to loss of function of the KiSS1-derived peptide receptor GPR54. Proceedings of the National Academy of Sciences 100, 10972-10976.
| Crossref | Google Scholar |
Fayazi M, Calder M, Bhattacharya M, Vilos GA, Power S, Babwah AV (2015) The pregnant mouse uterus exhibits a functional kisspeptin/KISS1R signaling system on the day of embryo implantation. Reproductive Biology and Endocrinology 13, 105.
| Crossref | Google Scholar | PubMed |
Francis VA, Abera AB, Matjila M, Millar RP, Katz AA (2014) Kisspeptin regulation of genes involved in cell invasion and angiogenesis in first trimester human trophoblast cells. PLoS ONE 9, e99680.
| Crossref | Google Scholar | PubMed |
Georgiades P, Ferguson-Smith AC, Burton GJ (2002) Comparative developmental anatomy of the murine and human definitive placentae. Placenta 23, 3-19.
| Crossref | Google Scholar | PubMed |
Gomes VCL, Sones JL (2021) From inhibition of trophoblast cell invasion to proapoptosis: what are the potential roles of kisspeptins in preeclampsia? The American Journal of Physiology-Regulatory, Integrative and Comparative Physiology 321, R41-R48.
| Crossref | Google Scholar |
Gomes VCL, Woods AK, Crissman KR, Landry CA, Beckers KF, Gilbert BM, Ferro LR, Liu C-C, Oberhaus EL, Sones JL (2022) Kisspeptin is upregulated at the maternal-fetal interface of the preeclamptic-like BPH/5 mouse and normalized after synchronization of sex steroid hormones. Reproductive Medicine 3, 263-279.
| Crossref | Google Scholar | PubMed |
Gottsch ML, Cunningham MJ, Smith JT, Popa SM, Acohido BV, Crowley WF, Seminara S, Clifton DK, Steiner RA (2004) A role for kisspeptins in the regulation of gonadotropin secretion in the mouse. Endocrinology 145, 4073-4077.
| Crossref | Google Scholar | PubMed |
Herreboudt AM, Kyle VRL, Lawrence J, Doran J, Colledge WH (2015) Kiss1 mutant placentas show normal structure and function in the mouse. Placenta 36, 52-58.
| Crossref | Google Scholar | PubMed |
Hoffmann DC, Willenborg S, Koch M, Zwolanek D, Muller S, Becker A-KA, Metzger S, Ehrbar M, Kurschat P, Hellmich M, Hubbell JA, Eming SA (2013) Proteolytic processing regulates placental growth factor activities. The Journal of Biological Chemistry 288, 17976-17989.
| Crossref | Google Scholar | PubMed |
Horikoshi Y, Matsumoto H, Takatsu Y, Ohtaki T, Kitada C, Usuki S, Fujino M (2003) Dramatic elevation of plasma metastin concentrations in human pregnancy: metastin as a novel placenta-derived hormone in humans. The Journal of Clinical Endocrinology & Metabolism 88, 914-919.
| Crossref | Google Scholar | PubMed |
Hu K-L, Chang H-M, Zhao H-C, Yu Y, Li R, Qiao J (2019) Potential roles for the kisspeptin/kisspeptin receptor system in implantation and placentation. Human Reproduction Update 25, 326-343.
| Crossref | Google Scholar | PubMed |
Janneau J-L, Maldonado-Estrada J, Tachdjian G, Miran I, Motte N, Saulnier P, Sabourin J-C, Cote J-F, Simon B, Frydman R, Chaouat G, Bellet D (2002) Transcriptional expression of genes involved in cell invasion and migration by normal and tumoral trophoblast cells. The Journal of Clinical Endocrinology & Metabolism 87, 5336-5339.
| Crossref | Google Scholar | PubMed |
Khong TY, De Wolf F, Robertson WB, Brosens I (1986) Inadequate maternal vascular response to placentation in pregnancies complicated by pre-eclampsia and by small-for-gestational age infants. BJOG: An International Journal of Obstetrics & Gynaecology 93, 1049-1059.
| Crossref | Google Scholar | PubMed |
Lee J-H, Miele ME, Hicks DJ, Phillips KK, Trent JM, Weissman BE, Welch DR (1996) KiSS-1, a novel human malignant melanoma metastasis-suppressor gene. JNCI Journal of the National Cancer Institute 88, 1731-1737.
| Crossref | Google Scholar |
Leon S, Fernandois D, Sull A, Sull J, Calder M, Hayashi K, Bhattacharya M, Power S, Vilos GA, Vilos AG, Tena-Sempere M, Babwah AV (2016) Beyond the brain-peripheral kisspeptin signaling is essential for promoting endometrial gland development and function. Scientific Reports 6, 29073.
| Crossref | Google Scholar | PubMed |
Librach CL, Werb Z, Fitzgerald ML, Chiu K, Corwin NM, Esteves RA, Grobelny D, Galardy R, Damsky CH, Fisher SJ (1991) 92-kD type IV collagenase mediates invasion of human cytotrophoblasts. The Journal of Cell Biology 113, 437-449.
| Crossref | Google Scholar | PubMed |
Masuzaki H, Ogawa Y, Sagawa N, Hosoda K, Matsumoto T, Mise H, Nishimura H, Yoshimasa Y, Tanaka I, Mori T, Nakao K (1997) Nonadipose tissue production of leptin: leptin as a novel placenta-derived hormone in humans. Nature Medicine 3, 1029-1033.
| Crossref | Google Scholar | PubMed |
Matjila M, Millar R, van der Spuy Z, Katz A (2016) Elevated placental expression at the maternal-fetal interface but diminished maternal circulatory kisspeptin in preeclamptic pregnancies. Pregnancy Hypertension: An International Journal of Women’s Cardiovascular Health 6, 79-87.
| Crossref | Google Scholar | PubMed |
Meekins JW, Pijnenborg R, Hanssens M, McFadyen IR, van Asshe A (1994) A study of placental bed spiral arteries and trophoblast invasion in normal and severe pre-eclamptic pregnancies. BJOG: An International Journal of Obstetrics & Gynaecology 101, 669-674.
| Crossref | Google Scholar | PubMed |
Nelson DM, Burton GJ (2011) A technical note to improve the reporting of studies of the human placenta. Placenta 32, 195-196.
| Crossref | Google Scholar | PubMed |
Ohtaki T, Shintani Y, Honda S, Matsumoto H, Hori A, Kanehashi K, Terao Y, Kumano S, Takatsu Y, Masuda Y, Ishibashi Y, Watanabe T, Asada M, Yamada T, Suenaga M, Kitada C, Usuki S, Kurokawa T, Onda H, Nishimura O, Fujino M (2001) Metastasis suppressor gene KiSS-1 encodes peptide ligand of a G-protein-coupled receptor. Nature 411, 613-617.
| Crossref | Google Scholar | PubMed |
Osol G, Celia G, Gokina N, Barron C, Chien E, Mandala M, Luksha L, Kublickiene K (2008) Placental growth factor is a potent vasodilator of rat and human resistance arteries. American Journal of Physiology – Heart and Circulatory Physiology 294, H1381-H1387.
| Crossref | Google Scholar | PubMed |
Park D-W, Lee S-K, Hong SR, Han A-R, Kwak-Kim J, Yang KM (2012) Expression of Kisspeptin and its receptor GPR54 in the first trimester trophoblast of women with recurrent pregnancy loss. American Journal of Reproductive Immunology 67, 132-139.
| Crossref | Google Scholar | PubMed |
Pattillo RA, Gey GO (1968) The establishment of a cell line of human hormone-synthesizing trophoblastic cells in vitro. Cancer Res 28, 1231-1236.
| Google Scholar | PubMed |
Qiao C, Wang C, Zhao J, Liu C, Shang T (2012) Elevated expression of KiSS-1 in placenta of Chinese women with early-onset preeclampsia. PLoS ONE 7, e48937.
| Crossref | Google Scholar | PubMed |
Ramaswamy S, Guerriero KA, Gibbs RB, Plant TM (2010) Structural interactions between kisspeptin and GnRH neurons in the mediobasal hypothalamus of the male rhesus monkey (Macaca mulatta) as revealed by double immunofluorescence and confocal microscopy. Endocrinology 149, 4387-4395.
| Crossref | Google Scholar | PubMed |
Rennie MY, Rahman A, Whiteley KJ, Sled JG, Adamson SL (2015) Site-specific increases in utero- and fetoplacental arterial vascular resistance in eNOS-deficient mice due to impaired arterial enlargement. Biology of Reproduction 92, 48.
| Crossref | Google Scholar | PubMed |
Reynolds LP, Redmer DA (1995) Utero-placental vascular development and placental function. Journal of Animal Science 73, 1839-1851.
| Crossref | Google Scholar | PubMed |
Roseweir AK, Kauffman AS, Smith JT, Guerriero KA, Morgan K, Pielecka-Fortuna J, Pineda R, Gottsch ML, Tena-Sempere M, Moenter SM, Terasawa E, Clarke IJ, Steiner RA, Millar RP (2009) Discovery of potent kisspeptin antagonists delineate physiological mechanisms of gonadotropin regulation. The Journal of Neuroscience 29, 3920-3929.
| Crossref | Google Scholar | PubMed |
Ryan BC, Vandenbergh JG (2002) Intrauterine position effects. Neuroscience & Biobehavioral Reviews 26, 665-678.
| Crossref | Google Scholar | PubMed |
Santos S, Voerman E, Amiano P, Barros H, Beilin LJ, Bergstrom A, Charles M-A, Chatzi L, Chevrier C, Chrousos GP, Corpeleijn E, Costa O, Costet N, Crozier S, Devereux G, Doyon M, Eggesbo M, Fantini MP, Farchi S, Forastiere F, Georgiu V, Godfrey KM, Gori D, Grote V, Hanke W, Hertz-Picciotto I, Heude B, Hivert M-F, Hryhorczuk D, Huang R-C, Inskip H, Karvonen AM, Kenny LC, Koletzko B, Kupers LK, Lagstrom H, Lehmann I, Magnus P, Majewska R, Makela J, Manios Y, McAuliffe FM, McDonald SW, Mehegan J, Melen E, Mommers M, Morgen CS, Moschonis G, Murray D, Ni Chaoimh C, Nohr EA, Nybo Andersen A-M, Oken E, Oostvogels AJJM, Pac A, Papadopoulou E, Pekkanen J, Pizzi C, Polanska K, Porta D, Richiardi L, Rifas-Shiman SL, Roeleveld N, Ronfani L, Santos AC, Standl M, Stigum H, Stoltenberg C, Thiering E, Thijs C, Torrent M, Tough SC, Trnovec T, Turner S, van Gelder MMHJ, van Rossem L, von Berg A, Vrijheid M, Vrijkotte TGM, West J, Wijga AH, Wright J, Zvinchuk O, Sorensen TIA, Lawlor DA, Gaillard R, Jaddoe VWV (2019) Impact of maternal body mass index and gestational weight gain on pregnancy complications: an individual participant data meta-analysis of European, North American and Australian cohorts. BJOG: An International Journal of Obstetrics & Gynaecology 126, 984-995.
| Crossref | Google Scholar | PubMed |
Seminara SB, Messager S, Chatzidaki EE, Thresher RR, Acierno JS, Jr, Shagoury JK, Bo-Abbas Y, Kuohung W, Schwinof KM, Hendrick AG, Zahn D, Dixon J, Kaiser UB, Slaugenhaupt SA, Gusella JF, O’Rahilly S, Carlton MBL, Crowley WF, Jr, Aparicio SAJR, Colledge WH (2003) The GPR54 gene as a regulator of puberty. New England Journal of Medicine 349, 1614-1627.
| Crossref | Google Scholar | PubMed |
Smith JT, Shahab M, Pereira A, Pau KY, Clarke IJ (2010) Hypothalamic expression of KISS1 and gonadotropin inhibitory hormone genes during the menstrual cycle of a non-human primate. Biology of Reproduction 83, 568-577.
| Crossref | Google Scholar | PubMed |
Smith JT, Li Q, Yap KS, Shahab M, Roseweir AK, Millar RP, Clarke IJ (2011) Kisspeptin is essential for the full preovulatory LH surge and stimulates GnRH release from the isolated ovine median eminence. Endocrinology 152, 1001-1012.
| Crossref | Google Scholar | PubMed |
Steegers EAP, von Dadelszen P, Duvekot JJ, Pijnenborg R (2010) Pre-eclampsia. Lancet 376, 631-644.
| Crossref | Google Scholar | PubMed |
Taylor J, Pampillo M, Bhattacharya M, Babwah AV (2014) Kisspeptin/KISS1R signaling potentiates extravillous trophoblast adhesion to type-I collagen in a PKC- and ERK1/2-dependent manner. Molecular Reproduction and Development 81, 42-54.
| Crossref | Google Scholar | PubMed |
Tolson KP, Garcia C, Yen S, Simonds S, Stefanidis A, Lawrence A, Smith JT, Kauffman AS (2014) Impaired kisspeptin signaling decreases metabolism and promotes glucose intolerance and obesity. Journal of Clinical Investigation 124, 3075-3079.
| Crossref | Google Scholar |
Torricelli M, Galleri L, Voltolini C, Biliotti G, Florio P, De Bonis M, Petraglia F (2008) Changes of placental Kiss-1 mRNA expression and maternal/cord kisspeptin levels at preterm delivery. Reproductive Sciences 15, 779-784.
| Crossref | Google Scholar | PubMed |
Tsoutsouki J, Patel B, Comninos AN, Dhillo WS, Abbara A (2022) Kisspeptin in the prediction of pregnancy complications. Frontiers in Endocrinology 13, 942664.
| Crossref | Google Scholar | PubMed |
vom Saal FS (1989) Sexual differentiation in litter-bearing mammals: influence of sex of adjacent fetuses in utero. Journal of Animal Science 67, 1824-1840.
| Crossref | Google Scholar |
Wice B, Menton D, Geuze H, Schwartz AL (1990) Modulators of cyclic AMP metabolism induce syncytiotrophoblast formation in vitro. Experimental Cell Research 186, 306-316.
| Crossref | Google Scholar | PubMed |
Yan C, Wang H, Boyd DD (2001) KiSS-1 represses 92-kDa type IV collagenase expression by down-regulating NF-κB binding to the promoter as a consequence of IκBα-induced block of p65/p50 nuclear translocation. The Journal of Biological Chemistry 276, 1164-1172.
| Crossref | Google Scholar | PubMed |
Zhang H, Long Q, Ling L, Gao A, Li H, Lin Q (2011) Elevated expression of KiSS-1 in placenta of preeclampsia and its effect on trophoblast. Reproductive Biology 11, 99-115.
| Crossref | Google Scholar | PubMed |
Zhang P, Tang M, Zhong T, Lin Y, Zong T, Zhong C, Zhang BP, Ren M, Kuang H (2014) Expression and function of kisspeptin during mouse decidualization. PLoS ONE 9, e97647.
| Crossref | Google Scholar | PubMed |