Can seed banking assist in conserving the highly endemic New Zealand indigenous flora?
Sarah V. Wyse
A
B
C
D
E
Abstract
Globally, plant species are facing numerous threats; an issue particularly acute for island floras, which often exhibit high levels of endemism. Ex situ conservation in seed banks is an important tool for plant conservation. However not all species’ seeds can be stored in conventional seed banks. Data on seed storage behaviour are therefore vital for conservation decision making.
To review available seed storage information for the New Zealand (NZ) indigenous seed plant flora, 86% of which are endemic.
We compiled seed storage information for the NZ flora from databases and existing literature, and used boosted regression trees models to investigate predictors of seed storage behaviour for NZ woody plants. We used existing global models to predict the likely storage behaviour for the NZ woody flora where this was unknown, to examine the overall contribution that conventional seed banking could make towards NZ plant conservation.
Data were available for 412 of 1823 seed plants, of which 83% produced orthodox seeds that can be stored in a conventional seed bank. Of the woody flora, the incidence of non-orthodox seeds was positively correlated with seed mass, plant height, biotic dispersal, and habitat diurnal temperature range. Eighty-one percent of the woody flora are predicted to produce orthodox seeds.
Conventional seed banking is likely to be suitable for a high proportion of the NZ flora. However, work is required to gain further seed storage behaviour data for NZ species, and to develop protocols for alternative ex situ conservation strategies for non-orthodox species, especially those facing in situ conservation threats.
Keywords: Aotearoa, conservation, ex situ, island flora, New Zealand, orthodox, recalcitrant, seed banking, seed storage behavior.
Introduction
Globally, plant species are facing numerous and pervasive threats including habitat loss, impacts of invasive species, novel pathogens, and the increasing effects of climate change (Nic Lughadha et al. 2020). Both in situ and ex situ conservation efforts are therefore vital to protect plant diversity from further declines. Although in situ conservation is essential to safeguard communities and ecosystems, and allow natural evolutionary processes to be maintained, ex situ conservation acts as an important ‘back-up’ for conserving the diversity of, and within, individual plant species.
Ex situ plant conservation is the preservation of plants or germplasm outside of the areas and situations in which the species naturally occur. Techniques include the maintenance of cultivated collections in botanic gardens or arboreta; cryopreservation of seeds, embryos, or other tissues in liquid nitrogen; or storage of dried and deep-frozen seeds in seed banks (Li and Pritchard 2009; Mounce et al. 2017). Of these techniques, the storage of seeds in seed banks (also known as ‘conventional seed banking’) is the most used, due to the ease of preserving high levels of genetic diversity for relatively low cost, in minimal space, and for long time periods (Wyse et al. 2018). However, not all seed plants produce seeds that are capable of being stored in this manner. Seed responses to temperature and moisture conditions define their ‘storage behaviour’, determining the ability of the seeds to survive storage in seed bank conditions and thus dictating the ex situ conservation options available for a plant species (Walters 2015). It is therefore critical to understand seed storage behaviour to determine the most appropriate conservation management approaches for plant species.
The process of seed conservation in a conventional seed bank involves the desiccation of seeds to the point that they are in equilibrium with 15% relative humidity air, before storage at −20°C (Li and Pritchard 2009). Most seed plant species (estimated at approximately 92%; Wyse and Dickie 2017) produce seeds that survive this drying and freezing process and are referred to as ‘orthodox’ (also known as ‘desiccation-tolerant’) species (Berjak and Pammenter 2008). However, ‘recalcitrant’ (‘desiccation-sensitive’) species produce seeds that remain metabolically active at the time of shedding, and do not survive the drying process required for storage in a conventional seed bank. Further, it is evident that some species have seeds that are shed with a relatively high water content, but can withstand drying to reasonably low levels, although not as low as orthodox seeds. These seeds also often fail to survive sub-zero temperatures and can be categorised as having an ‘intermediate’ seed storage behaviour (Berjak and Pammenter 2008). However, it should be noted that while these three seed storage behaviour categories (orthodox, intermediate, and recalcitrant) are useful from a management perspective, physiologically individual species may sit along a continuum of survival response to both moisture and temperature (Berjak and Pammenter 2008; Walters 2015). Globally, there are some evident trends in seed storage behaviour, with respect to habitat type, taxonomy, and plant growth form (Tweddle et al. 2003; Wyse and Dickie 2017). Such trends can allow prediction of species’ likely seed storage behaviours to anticipate available conservation options and aid in conservation decision making (Wyse and Dickie 2018).
Aotearoa New Zealand is a temperate Pacific archipelago with an indigenous vascular flora of approximately 2210 seed plant taxa, 86% of which are endemic (de Lange and Rolfe 2010). The main islands of New Zealand (Te Ika a Māui North Island, Te Waipounamu South Island, and Rakiura Stewart Island) span approximately 13° in latitude, from 34° to 47°S, with a strong east-west rainfall gradient either side of the axial ranges of the South Island. Offshore islands extend the latitudinal range north to 29°S (Rangitāhua Kermadec Islands) and south to 52°S (Motu Ihupuku Campbell Island group). Prior to human settlement the landscape was primarily forested below the treeline (McGlone 1983); however, the two waves of human settlement from Polynesia and Europe in the late 13th century CE (Wilmshurst et al. 2008) and c. 1800 CE, respectively, saw considerable and systematic forest loss. Today, only ~23% of the land area remains forested, primarily in wetter and higher elevation sites (Ewers et al. 2006). In addition to habitat loss, the New Zealand flora faces multiple and varied threats from plant pathogens such as myrtle rust (Austropuccinia psidii) and kauri dieback disease (Phytophthora agathidicida), and the impacts of introduced mammals. Herbivores introduced by European colonists, including ungulates and the Australian brushtail possum (Trichosurus vulpecula), have resulted in stalled forest successions, the loss of palatable species, and canopy tree death (Nugent et al. 2001; Husheer et al. 2003; Richardson et al. 2014). The impacts of mammalian predators on the New Zealand avifauna can lead to plant reproductive failure through the loss of pollinators and seed dispersers (Craig et al. 2000), while introduced rodents have additional effects as seed predators, particularly for larger-seeded species (Carpenter et al. 2018a). Further, there is evidence that climate change is leading to reduced seed production in dominant canopy tree species (Yukich Clendon et al. 2023). Thus, there is an urgent need to continue and expand plant conservation action in New Zealand.
Ex situ conservation must form an integral component of New Zealand’s plant conservation activities, particularly given the threats from plant pathogens that are difficult to manage in situ. Concerningly, both myrtle rust and kauri dieback predominantly affect northern forests, where plant diversity and endemism are also highest (Ogden 1995). To this end, there is increasing interest in ex situ plant conservation in New Zealand including establishment of seed banks, notably among Aotearoa New Zealand’s indigenous Māori population, who were guaranteed sovereignty over the indigenous flora by Te Tiriti o Waitangi (the Treaty of Waitangi) in 1840. However, progress on conserving plant species ex situ, and knowledge of seed storage behaviour of the New Zealand flora, remains limited compared to countries such as Australia and the United Kingdom (Sommerville et al. 2018). It is evident that dominant forest trees from podocarp-broadleaf forests (e.g. members of the Lauraceae, Meliaceae, and Podocarpaceae; Wyse and Dickie 2017; Sommerville et al. 2018), as well as fleshy-fruited Myrtaceae species (Nadarajan et al. 2021), are likely to pose challenges for ex situ conservation, yet there has not been a synthesis of seed storage information for the New Zealand flora, nor an assessment of the knowledge gaps. Here, we attempt to address this deficiency by compiling seed storage behaviour information for the highly endemic New Zealand seed plant flora to review the current state of knowledge. We then examine potential predictors of seed storage behaviour for New Zealand woody plants, the group for which the greatest proportion of information is known. Further, we use existing global models to predict the likely seed storage behaviour of woody species for which this information is unavailable, to assess the contribution conventional seed banking could make to ex situ plant conservation at national and regional levels.
Materials and methods
Data sources
The New Zealand seed plant flora was defined according to the New Zealand indigenous vascular plant checklist (de Lange and Rolfe 2010). For these species, we then obtained seed storage behaviour and seed mass from the Royal Botanic Gardens, Kew’s Seed Information Database (SID; Royal Botanic Gardens Kew 2017), accessible via the TRY trait database (Kattge et al. 2020). From SID we were able to obtain records of seed storage behaviour for 217 species. We then supplemented these data with additional records obtained from published material via extensive literature searching (completed in August 2021), adding records of seed storage behaviour for a further 203 species (these latter records are available as a Supplementary file). We searched individual species names alongside the terms ‘seed’, ‘storage’, ‘recalcitran*’, ‘intermediate’, and ‘orthodox’ using Google Scholar, Scopus, and Web of Science, and checked all search results for potential storage behaviour information. In addition, national library databases, including the National Forestry Library and the New Zealand Alpine Garden Society, were checked for grey literature relating to seed storage.
Growth form, plant height, and dispersal mode data were obtained from the dataset for the New Zealand angiosperm flora compiled by McGlone and Richardson (2022). In their dataset, McGlone and Richardson (2022) defined woody species as those with significant lignification of above ground parts, including trees (self-supporting and at least 5 m in height), shrubs (self-supporting and less than 5 m in height), and lianas (species reliant on other plants for support; includes true lianas, climbers, and scramblers). In contrast, herbaceous species had no or insignificant lignification of above ground parts and included herbs (self-supporting; definition includes sub-shrubs, which have herbaceous canopies but woody root stocks and lower stems) and vines (non-lignified species reliant on other plants for support). Plant height was the maximum height at maturity and taken as the upper bound (but not extreme maximum) of the range given for a species in flora compilations (http://www.nzflora.info/). McGlone and Richardson (2022) inferred dispersal mode from fruit characteristics described in flora compilations and supplemented by Thorsen et al. (2009), as species with fleshy fruits are ingested and dispersed by animals (biotic means; endozoochory), while those without fleshy fruits are dispersed by abiotic means such as wind, water, or gravity. We then supplemented the McGlone and Richardson (2022) angiosperm dataset with growth form, plant height, and dispersal mode data for the New Zealand gymnosperm flora (20 species) using their definitions and from the same data source, the Flora of New Zealand series (specifically Allan (1961) for the gymnosperm species). The most recent conservation status of the New Zealand seed plant flora was obtained from de Lange et al. (2017).
These different datasets were all based upon slightly different taxonomies, varying in spelling of species names, definitions of species, and placements of species in genera and families. We combined these datasets initially via fuzzy matching using the agrep function in R ver. 4.0.5 (R Core Team 2021). Species that were unable to be matched between datasets were then manually checked, with taxonomy edited following the New Zealand Plant Conservation Network (https://www.nzpcn.org.nz/) taxonomic treatments. Any remaining species that were unable to be satisfactorily matched were removed from further analysis.
Habitat environmental data
Given that the relative abundances of species with different seed storage behaviours varies considerably by habitat (Wyse and Dickie 2017), we obtained environmental variables describing the habitats in which each woody species typically occurs at the centre of its ecological niche. Species’ distribution data were acquired from the Global Biodiversity Information Facility (GBIF; GBIF.org 2022) limiting the data obtained to occurrences within New Zealand recorded as having reliable geographic locations, and for which a species could be matched taxonomically with at least 95% confidence. We extracted the annual precipitation, mean annual temperature, diurnal temperature range, and elevation at ~1 km resolution (Fick and Hijmans 2017). To reduce the potential effects of bias within the GBIF data, we used environmental filtering (Varela et al. 2014); only including occurrences in environmental space that were at least 0.1°C apart for temperature variables, were 25 mm apart for the precipitation variable, and 50 m apart for the elevation variable. For those species with at least 10 post-filtered occurrences, we calculated the centre of the ecological niche via Mahalanobis distance using a minimum covariance determinant approach (with k = 0.95); an approach that has been demonstrated to be robust to small samples sizes, errors, and bias (Etherington 2021). Analysis was done using R software (R Core Team 2021) with the packages rgbif (Chamberlain and Boettiger 2017), MASS (Venables and Ripley 2002), terra (Hijmans 2002), and sf (Pebesma 2018).
Data analysis
In the seed storage behaviour datasets, the following character states were used: ‘orthodox’; ‘orthodox?’; ‘intermediate’; ‘intermediate?’; ’sub-orthodox’; ‘recalcitrant’; and ‘recalcitrant?’. For our analyses, we treated all questionable classifications as being of that character state (i.e. ‘orthodox?’ became ‘orthodox’), and considered ‘sub-orthodox’ to be synonymous with ‘intermediate’. Thus, our seed storage behaviour dataset consisted of four character states: ‘orthodox’, ‘intermediate’, ‘recalcitrant’, and ‘unknown’. Where multiple conflicting records existed for a single species, we favoured orthodox over intermediate or recalcitrant (and intermediate over recalcitrant) following the rationale that an orthodox seed treated incorrectly could appear recalcitrant; for example, if harvested too soon, whilst a truly recalcitrant seed could not possibly exhibit orthodox behaviour.
Using the seed storage behaviour data, we first assessed the proportions of species where seed storage behaviour was known (i.e. ‘orthodox’, ‘intermediate’, or ‘recalcitrant’) by growth form and, for woody species, by plant family. Due to the ecological importance of canopy forming forest trees, which form and define forest habitats, we also compiled a list of dominant forest canopy trees to explicitly examine the seed storage behaviours of these species (Appendix 1). Dominant forest canopy trees were identified using data derived from New Zealand’s Land Use and Carbon Analysis System (LUCAS), a national network of permanent plots covering over 7.8 million ha that systematically samples natural forests established pre-1990 for the purpose of monitoring carbon sequestration rates (Coomes et al. 2002). Plots within the LUCAS network were each established on an 8-km grid across the main islands of New Zealand, and were 0.04 ha (20 m × 20 m) in area (Bellingham et al. 2020). Of the 1040 plots established initially, 874 randomly selected plots were remeasured from 2009 to 2014 and analysed by McGlone et al. (2024). Data from these re-measured plots were used here to identify dominant canopy tree species by calculating the mean basal areas of all tree species (as defined previously) across all plots in which they were present. Dominant canopy species were then defined as those tree species with mean basal areas of at least 3 m2 ha−1. This resulted in a list of 43 tree species, approximately 30% of the 140 indigenous tree species in the LUCAS plot network.
As a result of the low proportion of seed behaviour information available for herbaceous species, and the high level of taxonomic discrepancies within this group of plants, after initial data summaries further analyses were only conducted on woody plants. Globally, species that produce non-orthodox seeds that are unable to be conserved in a seed bank following conventional methodologies are typically woody plants (Tweddle et al. 2003; Wyse and Dickie 2017).
For the woody seed plant flora, we then examined the seed storage behaviour character states with respect to conservation status. Globally, the incidence of non-orthodox seeds (‘intermediate’ and ‘recalcitrant’) increases with increasing threat, as non-orthodox species are most prevalent in habitat types (i.e. tropical moist forests) facing highest levels of destruction as a result of human activities (Wyse et al. 2018). Thus, in a global context, the species facing the greatest in situ conservation threats will pose the greatest challenges for ex situ conservation.
Finally, we examined the incidence of species with different seed storage behaviours, including that of ‘unknown’ seed storage behaviour, with respect to the plant traits and environmental variables compiled here. For the woody species of known seed storage behaviour, we undertook a boosted regression trees (BRT) analysis (Elith et al. 2008) to examine the ability of the plant trait and environmental predictor variables to predict the likelihood that a species produces non-orthodox seeds, and assess which variables are the strongest predictors of this trait as well as potential interactions between variables. Models were constructed and evaluated within the gbm.step function from the dismo package (Hijmans et al. 2017) using 10-fold cross-validation. Following testing, modelling was undertaken using a tree complexity (number of interactions) of 2, and learning rate (the contribution of each individual tree to the developing model) of 0.001, and a bag fraction (the proportion of observations used in each step) of 0.6. The number of trees used was 2950. Functionality within the gbm.step function was used to calculate the relative contributions (relative importance) of each predictor variable, the area under the receiver operating curve (AUC), and the correlation of the data with predicted values. These latter two metrics provided an assessment of model performance.
Predicting seed storage behaviour for entire New Zealand woody flora
For the New Zealand woody seed plant flora (620 species), we were able to obtain seed storage information for approximately 30% of species (Table 1). To understand the potential extent of the woody seed plant flora that is likely to be able to be conserved in an ex situ seed bank using conventional methodologies, we used existing global models to predict the likely seed storage behaviour for the unknown species (Wyse and Dickie 2018). These models were developed to provide decision-making support for ex situ plant conservation and use data on species seed traits, habitat environmental variables, and the seed storage behaviour of known related species to predict the likelihood of a species producing non-orthodox seeds.
Herb | Liana | Shrub | Tree | Vine | Total | ||
---|---|---|---|---|---|---|---|
Orthodox | 191 | 9 | 60 | 61 | 7 | 328 | |
Intermediate | 8 | 0 | 3 | 12 | 0 | 23 | |
Recalcitrant | 9 | 3 | 4 | 28 | 0 | 44 | |
Unknown | 981 | 19 | 284 | 138 | 6 | 1428 | |
Total | 1189 | 31 | 351 | 239 | 13 | 1823 |
Using the combined datasets of known storage behaviour and predicted storage behaviour for the remaining species, we examined how the relative proportions of orthodox versus non-orthodox species varied with geographical region for all 620 woody species. Using our GBIF data and a similar workflow to Brandt et al. (2021), we determined the number of orthodox species, recalcitrant and intermediate species, and species with insufficient information to predict storage behaviour within each of the 16 mainland political regions across New Zealand (Stats NZ 2019). To guard against outliers or errors we only included those records on the main islands of New Zealand (Land Information New Zealand 2011) and required at least five occurrences for a species to be counted within a region.
Results
We compiled data for 1823 New Zealand indigenous seed plants (Table 1); a number lower than the 2080 angiosperm taxa examined by McGlone and Richardson (2022) due to discrepancies in taxonomy between datasets. Here, we report on species where we were able to satisfactorily resolve any taxonomy differences between datasets. Most of the incongruities between the datasets (i.e. taxa that were included by McGlone and Richardson (2022) but excluded from our analyses) were herbaceous species.
Of our 1823 seed plants, the seed storage behaviour is currently known for just 412 species (22%; Table 1, Fig. 1a). Of these, 344 species (83%) produce orthodox seeds. Proportionally, we know least about the seed storage behaviours of New Zealand’s indigenous herbaceous plants (Fig. 1b). However given their numeric dominance in the flora (1189 of 1823 species examined here; 65%; Table 1), this is unsurprising. Of New Zealand’s woody plants, most data for seed storage behaviour pertains to tree species, even though there are more shrub than tree species (Table 1). Indeed, our knowledge of the seed storage behaviour for tree species is proportionally twice that of shrub species (Fig. 1b). Among the most species-rich woody plant families in the New Zealand flora, we have the most knowledge, proportionately, about Nothofagaceae, Fabaceae, and Myrtaceae, but none about the seed storage behaviour for woody Loranthaceae, Rosaceae, or Santalaceae (Fig. 1c). The two species that we know the seed storage behaviour of in the Alseuosmiaceae (Alseuosmia macrophylla and Alseuosmia pusilla) are both intermediate species, while our remaining families contain either both orthodox and non-orthodox species or, to date, entirely comprise orthodox woody species (Fig. 2a). Those families that may pose the greatest difficulty for conserving in a seed bank (i.e. contain a high proportion of non-orthodox species) are the Araliaceae, Pittosporaceae, Podocarpaceae, and Rubiaceae, while families including the Nothofagaceae, Fabaceae, and Plantaginaceae may be most well suited to conventional seed banking (Fig. 2a). Knowledge on seed storage behaviour was proportionally much higher amongst the 43 dominant tree species in the New Zealand flora defined here than across the woody flora as a whole (Fig. 2b), as storage behaviour was known for 74% of tree species (32 of 43 species) compared to 29% of all woody plants (180 of 620 species). Of the known species, the incidence of non-orthodox species was also higher amongst the dominant tree species than amongst all woody plants, at 41% (13 of 32 species) compared to 28% (50 of 180 species).
The state of knowledge of seed storage behaviour for New Zealand seed plants. (a) The proportions of the New Zealand seed plant flora for which the seed storage behaviour is recalcitrant, intermediate, orthodox, or currently unknown. (b) The proportions of the seed plant flora with known seed storage behaviour, by growth form. (c) For all woody species (lianas, shrubs, and trees), the proportions of species with known seed storage behaviour by family, for the most diverse families (those with at least five woody species in the New Zealand flora). Numbers above bars in (b) and (c) indicate the number of species in each growth form category, or woody species in each family. Taxonomy follows the New Zealand Plant Conservation Network (www.nzpcn.org.nz); growth form classification follows McGlone and Richardson (2022).
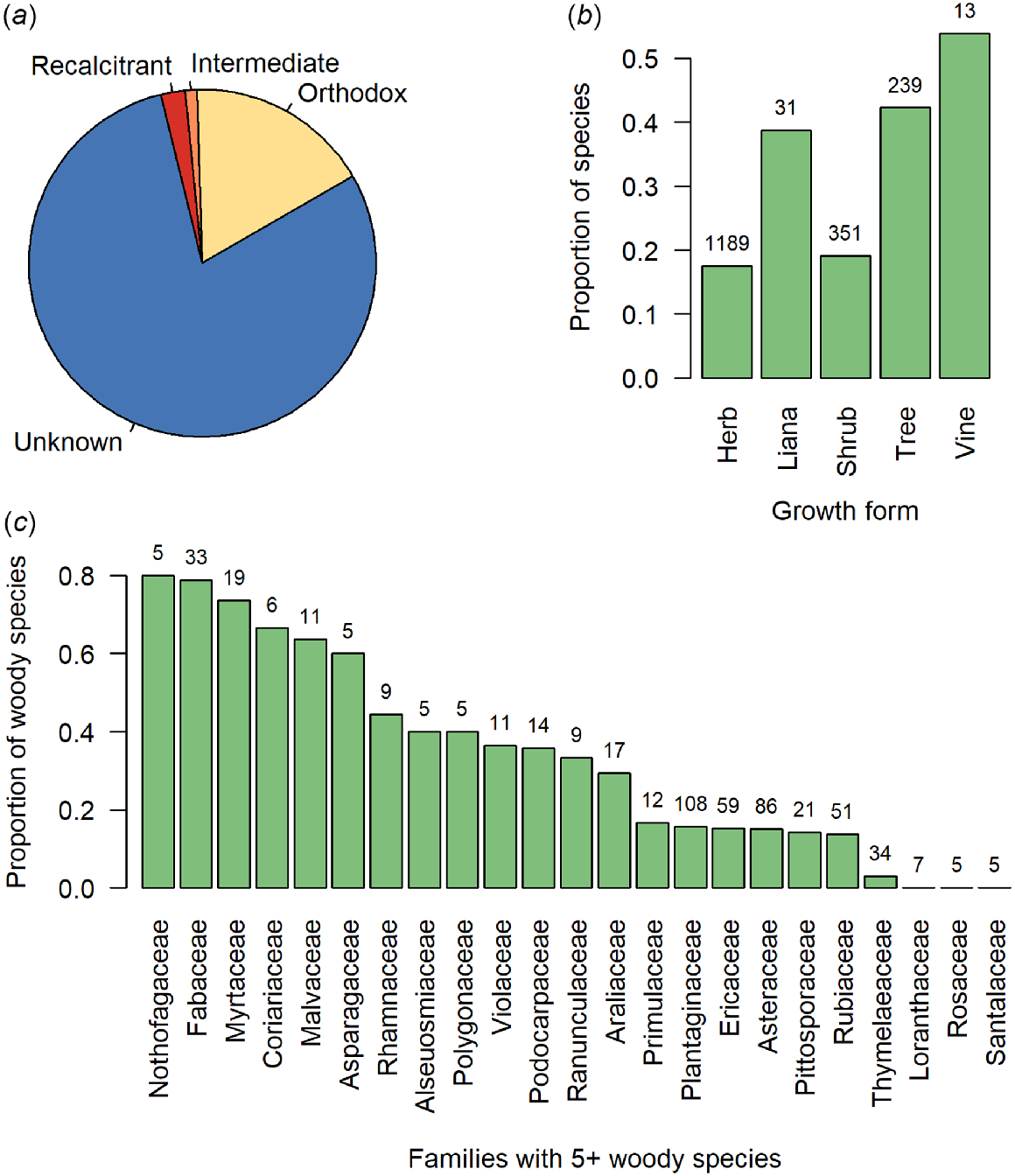
The proportions of species with seeds that have orthodox, intermediate, recalcitrant or unknown storage behaviour: (a) per family for families with at least five woody species in the New Zealand flora, and in total, for woody species in the New Zealand flora; and (b) per family, and in total, for all dominant canopy trees in New Zealand forests (tree species with a mean basal area ≥3 m2 ha−1 in plots in which they are present). Numbers above the bars indicate the numbers of species represented in each bar. Taxonomy follows the New Zealand Plant Conservation Network (www.nzpcn.org.nz). See Appendix 1 for the list of dominant canopy trees.
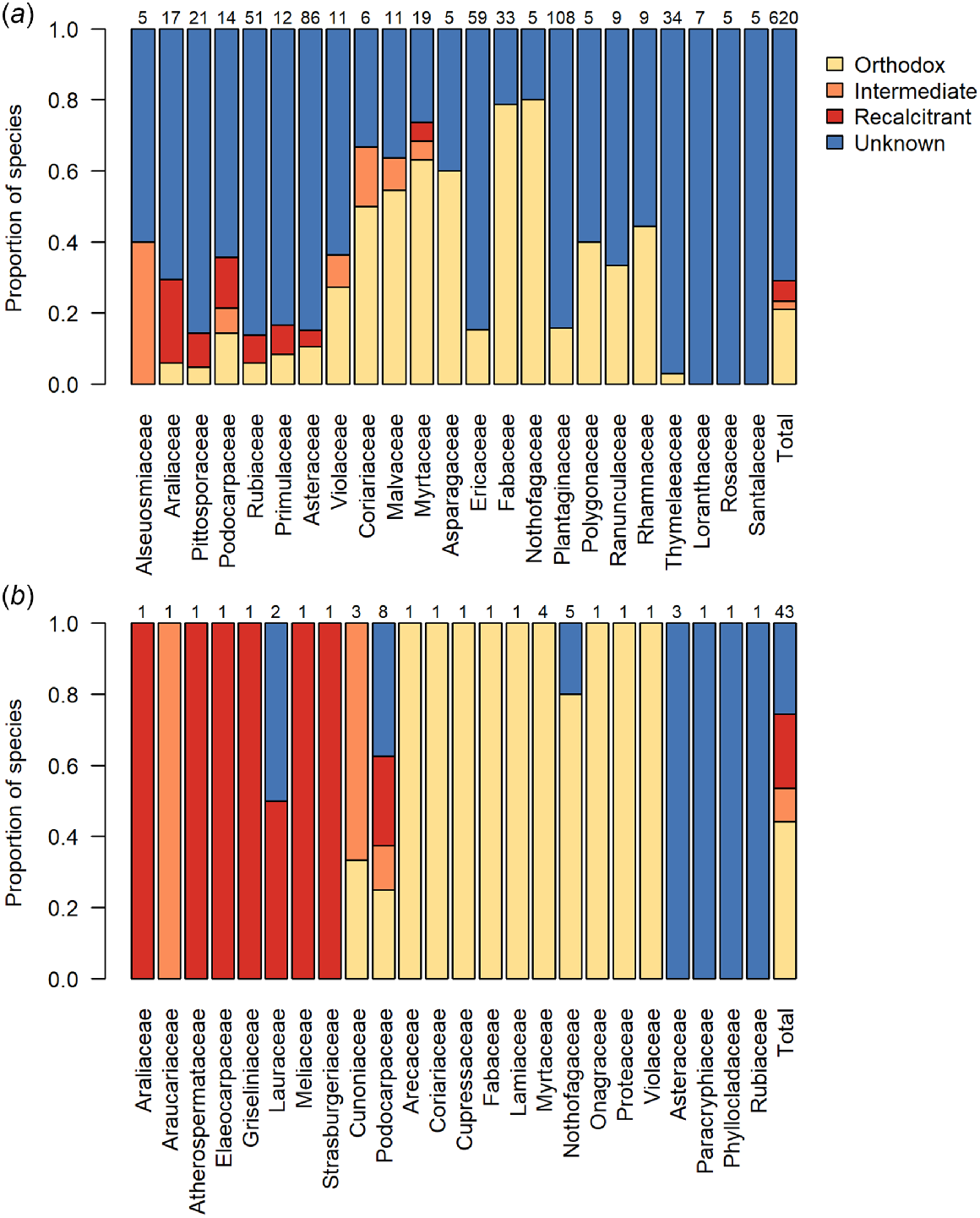
Many of the dominant trees in the New Zealand flora are species from globally important plant families, which are represented by only one or a few species in New Zealand. Such families include the Araucariaceae (Agathis australis), Elaeocarpaceae (dominant tree species Elaeocarpus dentatus; also three more species), Lauraceae (dominant tree species Beilschmiedia tarairi and B. tawa; also two more species), and Meliaceae (Didymocheton spectabilis). With the exception of Beilschmiedia tarairi (unknown), these dominant and ecologically important trees all have recalcitrant seeds (Fig. 2b). Other such families include Cunoniaceae (dominant tree species Ackama rosifolia, Pterophylla racemosa, Pterophylla silvicola; also one other species), of which two have intermediate seeds, and Cupressaceae (dominant tree species Libocedrus bidwillii; also one other species) and Lamiaceae (Vitex lucens), which both have orthodox seeds (Fig. 2b). The single endemic palm of the main islands of New Zealand (Rhopalostylis sapida; Arecaceae) also has orthodox seeds. Amongst the New Zealand Myrtaceae, a family where the majority of New Zealand species are classed as threatened, the four dominant tree species (Kunzea ericoides s.l., Leptospermum scoparium, Metrosideros robusta, and M. umbellata) all produce orthodox seeds in dry capsules, while the rare, fleshy-fruited Syzygium maire is recalcitrant. The fleshy-fruited shrubs Lophomyrtus bullata and Lophomyrtus obcordata were defined as orthodox based on a finding of seed desiccation tolerance (Nadarajan et al. 2021), but further evidence suggests these species may more correctly be defined as intermediate due to viability loss following storage at −18°C (van der Walt 2023). Metrosideros excelsa is also an intermediate species. There does not seem to be any higher representation of non-orthodox species among threatened woody species than those that are at risk or not threatened (Fig. 3). Proportionally, there is more knowledge about the seed storage behaviour of threatened species than those that are at risk.
The proportions of extant woody species with seeds that have orthodox, intermediate, recalcitrant or unknown storage behaviour by conservation status. Numbers above the bars indicate the numbers of woody species per category. Taxonomy follows the New Zealand Plant Conservation Network (www.nzpcn.org.nz). Conservation status follows de Lange et al. (2017).
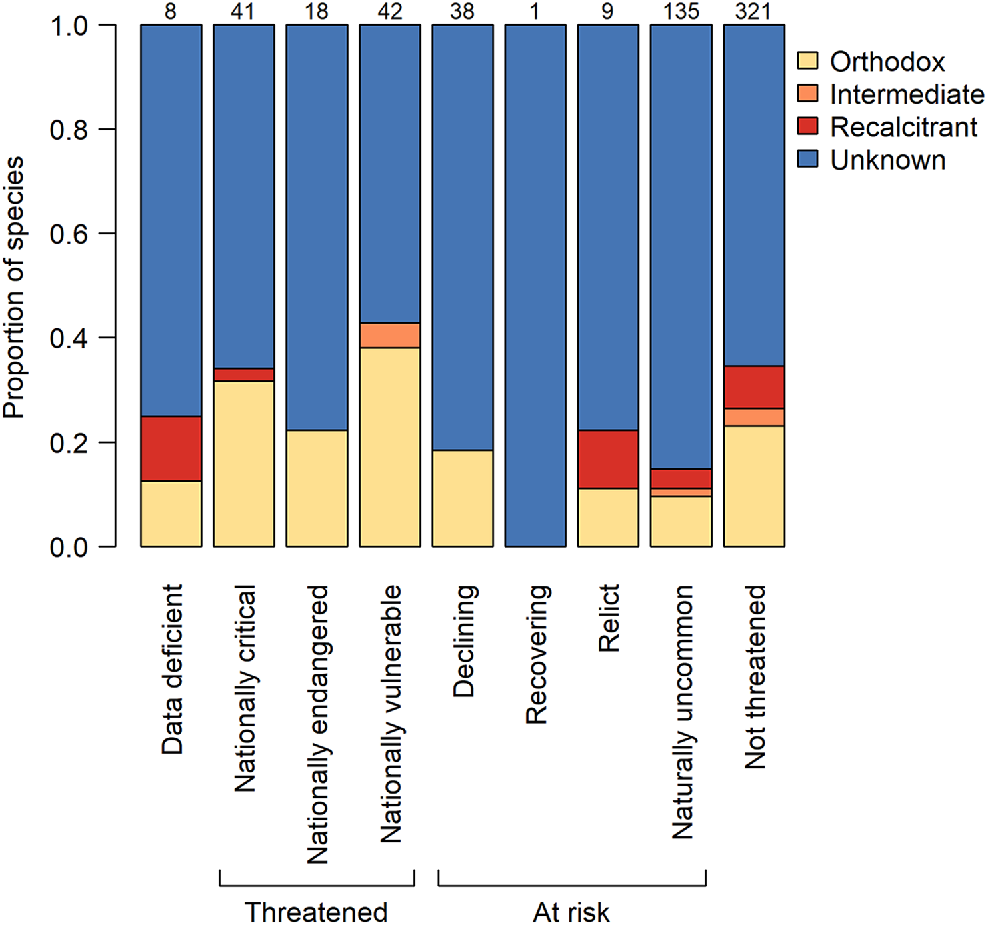
Trees have a higher incidence of non-orthodox seeds than shrubs in New Zealand’s woody flora (Fig. 4a), and intermediate and recalcitrant species are taller than orthodox species (Fig. 4c). Non-orthodox species also have heavier seeds, are more frequently fleshy-fruited, and are dispersed more frequently by biotic means than orthodox species (Fig. 4b, d). There is little difference in annual precipitation in the habitats of non-orthodox than orthodox species (Fig. 4e), although non-orthodox species more frequently occur in lower elevation habitats with higher mean annual temperatures and lower diurnal temperature ranges (Fig. 4f–h). Our knowledge of species seed storage behaviour is biased towards taller species, from lower elevations (Fig. 4c, h).
The seed storage behaviour of New Zealand woody plants with respect to plant traits and habitat characteristics. Growth form classification and dispersal mode follow McGlone and Richardson (2022). For parts (c–h), small grey lines within beans indicate individual data points, solid black lines represent means for each group, dashed line indicates overall mean.
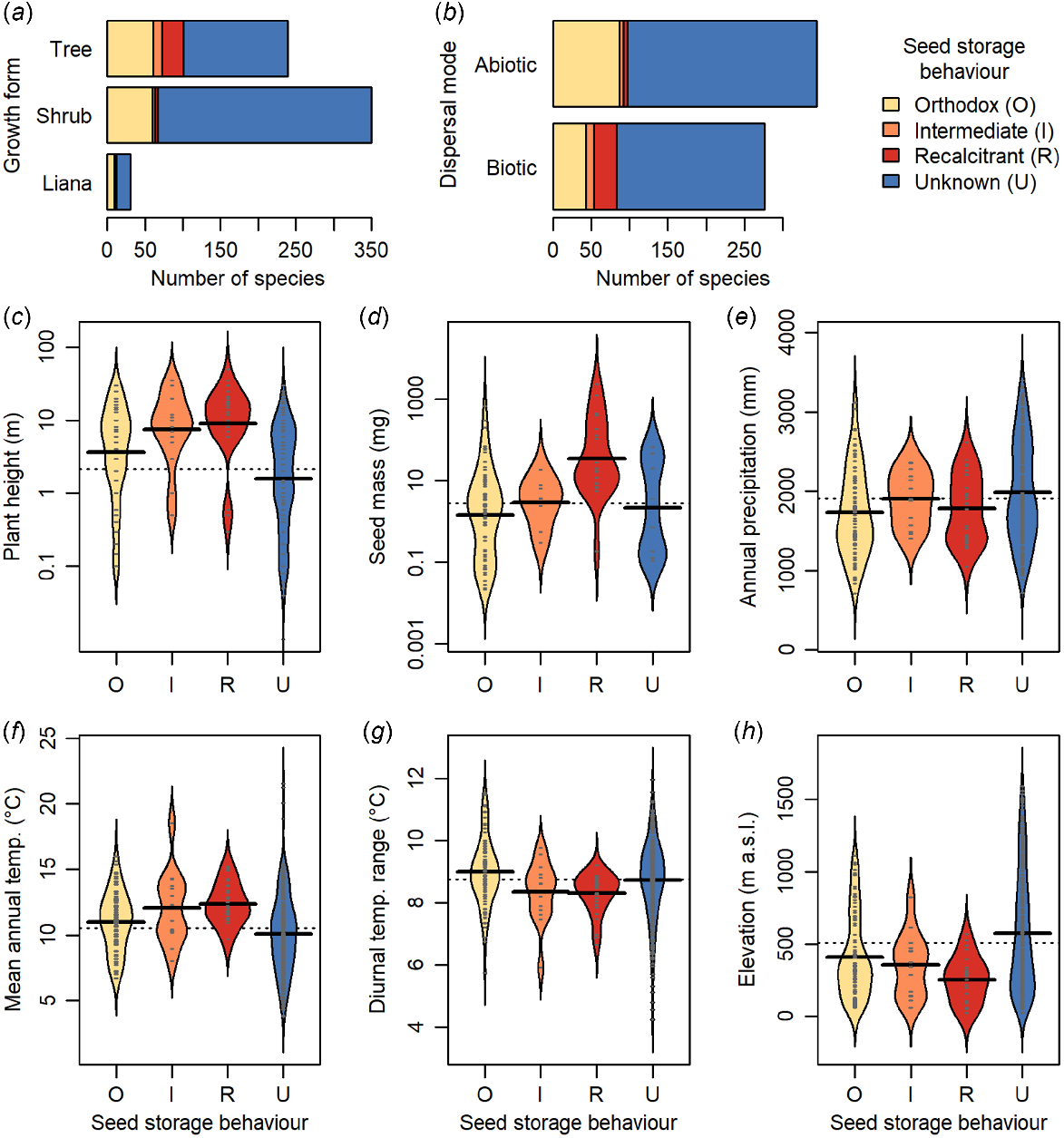
Dispersal mode was the strongest predictor of whether a woody species is likely to produce non-orthodox seed, followed by seed mass and plant height (Table 2; boosted regression trees analysis, AUC score of 0.791 and a correlation of 0.487). Diurnal temperature range was the habitat environmental variable with the strongest predictive ability. The strongest interactions between variables were between plant height and both dispersal mode and diurnal temperature range (Table 3). A weaker interaction also occurred between dispersal mode and diurnal temperature range. The influence of increasing plant height or decreasing diurnal temperature range on the likelihood of a species producing non-orthodox seeds was stronger for seeds dispersed by biotic than abiotic means (Fig. 5a, b). For the interaction between plant height and diurnal temperature range, there was a stronger effect of increasing plant height on the likelihood of a species producing non-orthodox seeds for species in habitats with lower diurnal temperature ranges (Fig. 5c).
Predictor variable | Relative importance (%) | |
---|---|---|
Dispersal mechanism | 24.95 | |
Seed mass | 18.08 | |
Plant height | 16.33 | |
Diurnal temperature range | 15.72 | |
Annual precipitation | 9.73 | |
Elevation | 7.48 | |
Mean annual temperature | 6.19 | |
Growth form | 1.52 |
Dispersal | Plant height | Diurnal temp. range | Annual precipitation | Mean annual temp. | Growth form | Elevation | ||
---|---|---|---|---|---|---|---|---|
Seed mass | 0.21 | 0.19 | 0.42 | 0.89 | 0.01 | 0.00 | 0.23 | |
Dispersal | – | 9.88 | 1.51 | 0.09 | 0.03 | 0.35 | 0.94 | |
Plant height | – | – | 3.89 | 0.00 | 0.25 | 0.00 | 0.43 | |
Diurnal temp. range | – | – | – | 0.15 | 0.03 | 0.02 | 0.00 | |
Annual precipitation | – | – | – | – | 0.34 | 0.00 | 0.05 | |
Mean annual temp. | – | – | – | – | – | 0.00 | 0.03 | |
Growth Form | – | – | – | – | – | – | 0.00 |
Interactions between variables in the boosted regression trees analysis examining the ability of plant traits and habitat environmental variables to predict the likelihoods of woody New Zealand species having non-orthodox (i.e. recalcitrant or intermediate) seeds. Figure shows the strongest interactions between variables as determined by the analysis (relative interaction strengths >1; see Table 3 for further details).
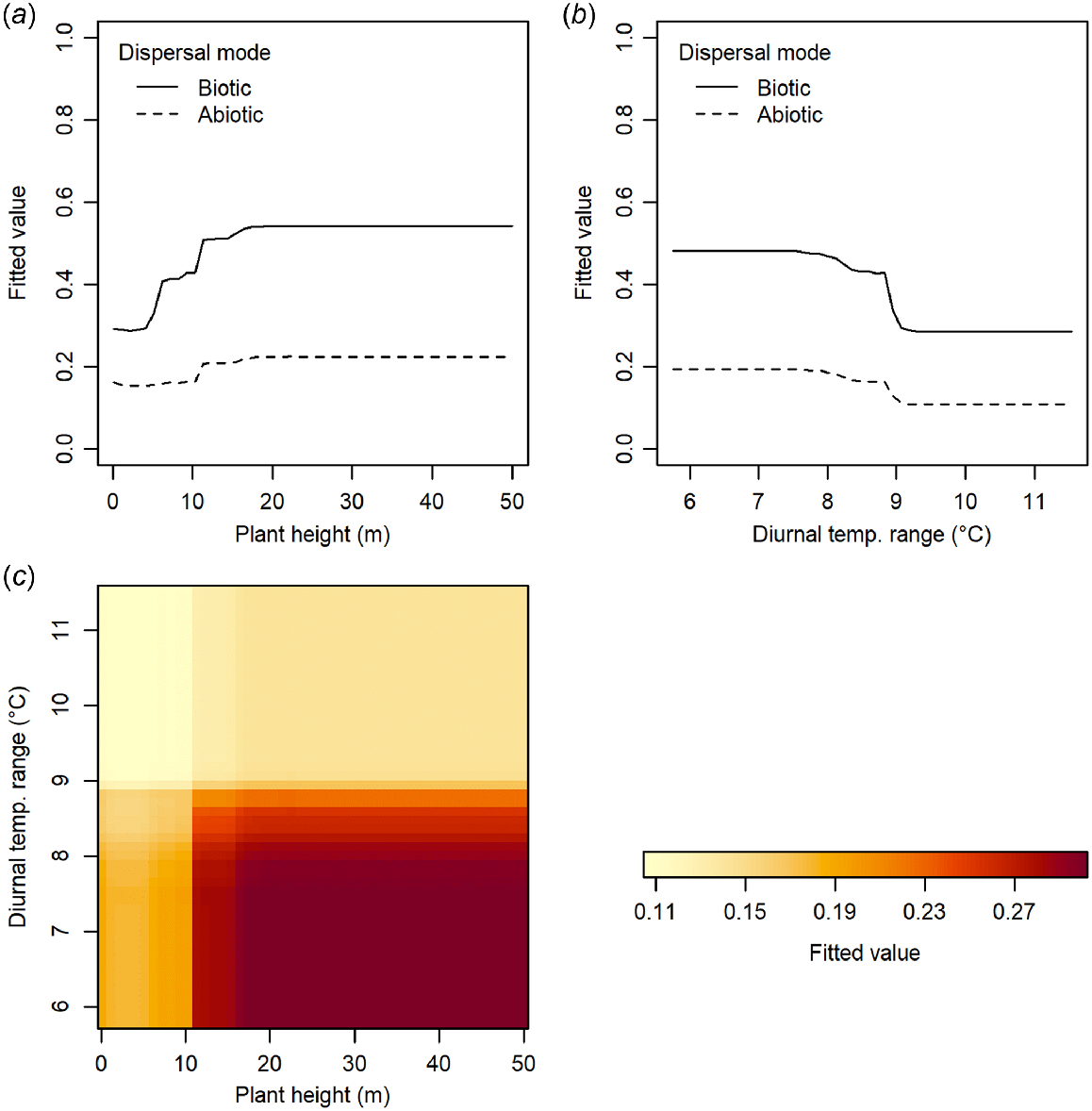
When we predicted the likely seed storage behaviour for woody species for which this trait is currently unknown, we found that 81% of the New Zealand woody flora are likely to produce orthodox seeds, 17% are likely to produce intermediate or recalcitrant seeds, and only 1% have insufficient information to allow us to predict this trait (Fig. 6). Given the bias in the dataset towards tree species, which had a higher incidence of recalcitrant seed behaviour than shrub species (Fig. 4a), this predicted value of 81% may be more realistic for the proportion of orthodox species within the entire New Zealand woody flora than the 73% observed amongst woody species with known storage behaviour (Table 1). There was some variation among regions in the proportion of the region’s woody flora that produce, or are predicted to produce, orthodox seeds. This regional variation largely followed a latitudinal gradient, and ranged from 81% of woody species in cool temperate regions (Otago and Canterbury) to 67% in a warm temperate region (Northland; Fig. 6). Approximately 31% of all indigenous woody species in the warm temperate Auckland and Bay of Plenty regions are predicted to produce non-orthodox seeds that are unlikely to be able to be conserved following conventional seed banking methodologies.
Predicted proportions of species in regional woody flora that produce orthodox compared to non-orthodox (recalcitrant and intermediate) seeds. Inset: the predicted seed storage behaviours for all New Zealand woody species (620 species). Insufficient information refers to species where there was insufficient information for a species on its traits, habitat, or seed storage behaviour of near relatives and thus, reliable predictions could not be made.
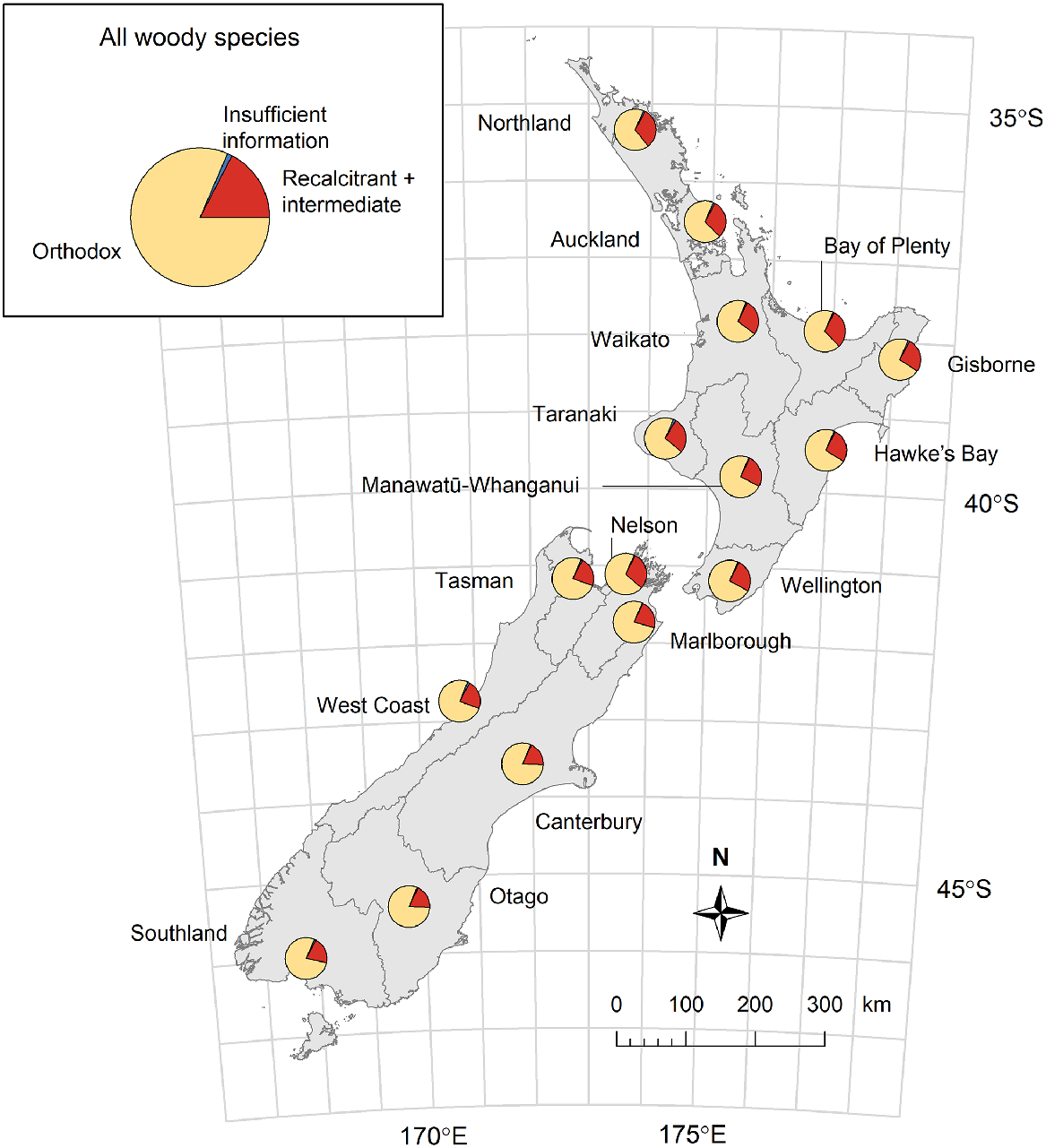
Discussion
Seed ecology and conservation of the New Zealand flora
Here, we have compiled all available seed storage data for the highly endemic New Zealand flora, to our knowledge an unprecedented exercise for a national flora (although see Crawford et al. (2007) for a compilation of data on the survival of Australian species within a seed bank). The proportion of recalcitrant species in the New Zealand flora (11% of all known species) is considerably higher than the estimated value of 3% of all plants for the global biome ‘temperate broadleaf and mixed forests’ (Wyse and Dickie 2017), in which much of the New Zealand landscape occurs (Olson et al. 2001). The temperate broadleaf and mixed forests biome is predominantly distributed in the Northern Hemisphere and data from it are biased towards deciduous forests, including those of Great Britain and Ireland (Olson et al. 2001), where recalcitrant species may make up just 1% of the flora (Wyse and Dickie 2018). In contrast, the New Zealand woody flora occur in evergreen rain forests (Grubb et al. 2013; McGlone et al. 2016).
The higher incidence of orthodox seed behaviour among New Zealand indigenous herbaceous species compared to woody species was in line with global trends (Tweddle et al. 2003; Wyse and Dickie 2017). However, the incidence of recalcitrant and intermediate herbaceous species found here in the New Zealand flora was much higher than a previous global estimate based on the available data at the time. In their study, Tweddle et al. (2003) found non-orthodox seeds to be exceedingly rare amongst herbaceous species, as 99.8% of the 517 herbaceous species in their dataset were orthodox, and just 0.2% of species (equates to a single species) were recalcitrant. Tweddle et al. (2003) discuss that their finding was likely due to recalcitrant seeds being incompatible with the regeneration of annual and biennial species. Data are not available on the proportion of their herbaceous species that were annuals, however in the New Zealand flora the majority of herbs are perennial, with just 5.7% comprising annual species (data from McGlone and Richardson 2022). For comparison 37% of herbaceous species in the indigenous flora of California (Carl Freeman et al. 1979), 25% of the herbaceous species from North and South Carolina (Conn et al. 1980), and 27% of the herbaceous species of Britain and Ireland (Hill et al. 2004), are annuals. This low proportion of annual herbs may explain the higher incidence of recalcitrant herbs observed here.
The New Zealand flora largely originated from post-25 Ma immigration either down the northern Pacific island chains, or via dispersal from Australia, with few of the vicariant Gondwanan species surviving the Oligocene marine transgression (McGlone and Richardson 2022). The flora is comparatively young, with 90% of extant lineages younger than 15 million years and 50% of lineages evolving during the last 5 Ma (Heenan and McGlone 2019). Structurally, New Zealand forests are complex, resembling tropical forests and with high numbers of biotically-dispersed species (Cockayne 1921; Dawson and Sneddon 1969; McGlone et al. 2016). Of the lowland woody genera, 60% also have taxa in tropical or subtropical regions (McGlone and Richardson 2022). The high proportion of species with tropical affinities, particularly in warm temperate ‘podocarp-broadleaf’ forests (forests with a broadleaved-angiosperm canopy and emergent Podocarpaceae trees) in the north of the country, may explain the comparatively high proportion of recalcitrant species in the New Zealand flora relative to other temperate broadleaf and mixed forests globally. We found a higher incidence of recalcitrant species in the warm temperate northern regions, consistent with the finding that temperature was the strongest environmental predictor of seed storage behaviour as non-orthodox species were more likely to occur in habitats with lower diurnal temperature ranges and higher mean annual temperatures. The dominant tree species of New Zealand have different southern range limits depending on their individual environmental tolerances (Wardle 1991), which may explain the gradual rather than abrupt differences in the incidence of recalcitrant species among regions moving south. The relationship between the incidence of recalcitrant species and temperature follows patterns observed globally (Tweddle et al. 2003; Wyse and Dickie 2018), however, unlike global trends, rainfall was not an important predictor of seed storage behaviour despite the strong rainfall gradients within New Zealand. Thus, the primary environmental correlate for seed storage behaviour in the New Zealand woody flora appears to be temperature, via latitude and elevation gradients.
Plant traits were more important correlates of seed storage behaviour than environmental variables. While previous work indicates that tree species globally have a higher incidence of recalcitrant seeds relative to all plants, potentially driven by the high diversity of tree species in tropical moist forest and mangrove habitats (Wyse et al. 2018), this is the first study to explicitly detect a trend of increasing likelihood for recalcitrant seeds with plant height. The seed trait relationships observed here were consistent with global trends, with the finding that recalcitrant species had seeds that were larger, and more likely to be animal-dispersed (Daws et al. 2005, 2006; Wyse and Dickie 2018). We do not have the data to examine whether the seed coat ratio, previously reported to predict seed storage behaviour alongside seed mass (Daws et al. 2006), relates to seed storage behaviour in the New Zealand flora. However, contrary to the predictive model derived from seed traits of the flora of Panamá indicating that recalcitrant seeds are large and with thin seed coats, which also successfully predicted seed storage behaviours of British species, numerous large-seeded New Zealand trees have unusually thick-walled (2–3 mm) endocarps (Carpenter et al. 2018b) including the recalcitrant Elaeocarpus dentatus (Elaeocarpaceae). Daws et al. (2005) suggest that, for large-seeded species, the recalcitrant seed trait is advantageous as the swift germination correlated with the trait may confer a selective advantage by reducing the amount of time during which the seed is vulnerable to predation. Yet, the recalcitrant E. dentatus can take up to 7 years to germinate (Carpenter et al. 2018b). Before faunal changes associated with human colonisation of New Zealand, vertebrate seed predators were avian rather than mammalian (Carpenter et al. 2020). Thus, seed trait relationships could potentially differ to those from other flora where mammalian seed predators predominate. Further research should examine these relationships within the New Zealand flora. However, it is certainly evident that some dominant, non-orthodox trees such as Agathis australis (Araucariaceae) and Podocarpaceae species germinate rapidly after being shed, forming a seedling bank (Ogden 1985).
Our finding of a prevalence of recalcitrant species among bird-dispersed, lowland, dominant canopy trees raises concerns for conservation. Lowland habitats have seen a disproportionate level of habitat loss due to clearance for agriculture (Ewers et al. 2006), and with the exception of the West Coast region (South Island) remaining lowland forest largely occurs on private land, where there is less legal protection (Pannell et al. 2021). In situ conservation options for species in these forests are therefore more limited than they are in higher elevation forests. Beech (Nothofagaceae) forests are most common at high elevation and are likely to contain a high proportion of species suitable for conservation in a conventional seed bank, whilst the more diverse conifer-angiosperm forests (including podocarp-broadleaf forest and kauri forest) present more challenges. However, in contrast to global trends (Wyse et al. 2018) we did not find a relationship between threat status and seed storage behaviour, suggesting that the species most threatened in situ are no less likely to be conserved ex situ within a conventional seed bank than non-threatened species; a promising finding with respect to their conservation. However, it is certainly evident that further research is required for many species.
Future directions
Our compilation of seed storage information for the New Zealand flora has highlighted many knowledge gaps that future research must aim to fill. Herbs and shrubs are the plant growth forms with the most limited seed storage data, and should therefore be a focus of further research. Given the low proportion of annuals in the New Zealand herbaceous flora relative to other regions, and the higher incidence of recalcitrant species in the New Zealand herbaceous flora than amongst herbs globally as indicated by the currently available evidence, deepening our understanding of seed storage behaviour of New Zealand herbaceous species should be a priority. Models derived from global data may fail to accurately predict the seed storage traits of these species. Further, our results illustrate the woody plant families for which very limited, or no, information is available in the New Zealand flora. The seed storage behaviour of a given species has been shown to be strongly correlated with those of its close relatives (Wyse and Dickie 2018). Therefore, to better understand seed storage behaviour of the New Zealand flora it will be more immediately beneficial to survey species from families and genera for which there is currently little information, rather than gaining deeper insights into genera were there are already many species with known storage information. The exceptions are genera such as Pittosporum and Coprosma, or families such as Podocarpaceae, in which there is variability in seed storage behaviour among species. For such taxa, it would be useful to gain further insights to understand plant or habitat traits that may predict seed storage traits. Loranthaceae and Santalaceae are families with no seed storage data for New Zealand species, and globally very little additional information. The Loranthaceae, in particular, is a family of concern in New Zealand as many of these mistletoe species are classed as ‘at risk – declining’ due to threats including mammalian herbivores (Australian brushtail possum; Sweetapple et al. 2002), and reductions in avian pollinators (Kelly et al. 2007). We have been unable to find any data on seed storage behaviour for Loranthaceae globally, whilst the available information for Santalaceae is only marginally better. Within Santalaceae, many species are intermediate (Thomson 2006), while Osyris lanceolata is recalcitrant (Mwang’ingo et al. 2004).
Another substantial knowledge gap in New Zealand seed conservation is that of seed longevity in an ex situ seed bank. This refers to the length of time an orthodox seed can remain deep-frozen in storage before viability is significantly lost. Information on seed longevity in an ex situ seed bank is critical for effective collection management, as it will set the seed viability re-test intervals for the collections, determine re-collection strategies (Liu et al. 2020), and inform any adaptation of current conventional protocols that may be required to suit short-lived seeds. Accelerated ageing tests have been found to correlate with real time data of seed survival in storage, and can augment the real time data to aid understanding of potential seed lifespan in storage (Davies et al. 2020). Data suggest that there is considerable variability among, and within, orthodox species in their longevity in an ex situ seed bank, with indications that species from hot dry environments may store longer than those from cool, wet habitats (Probert et al. 2009; Davies et al. 2020; Liu et al. 2020). For example, among the Hawaiian indigenous flora, 63% of 295 species examined by Chau et al. (2019) retained at least 70% viability after 10 years in storage, while 23% declined to less than 70% of the initial viability within 5 years of storage.
Finally, urgent work is also required on developing protocols for alternative ex situ conservation methods for threatened recalcitrant species in the New Zealand flora. To date, extensive work has been undertaken for two rain forest trees, Didymocheton spectabilis and the critically threatened Syzygium maire, to determine appropriate cryopreservation protocols (Park 2013; van der Walt et al. 2021a, 2021b, 2022). For both species, research has examined optimal processing of excised embryos and tissue culture methodologies; however, further work is required to establish viable approaches. We suggest that, in addition to continuing work on these species, at risk recalcitrant tree species such as Meryta sinclairii and Pseudopanax chathamicus should be future priorities for research. Developing seed storage protocols for recalcitrant, dominant canopy tree species such as Podocarpus totara, Dacrycarpus dacrydioides, Beilschmiedia tawa, and Elaeocarpus dentatus would also aid in the supply of seedlings of these species for restoration programmes, particularly species such as B. tawa, P. totara, and D. dacrydioides that undergo mast seeding (Webb and Kelly 1993; Yukich Clendon et al. 2023), and thus for which seed availability fluctuates annually.
Conclusions
A high proportion of the New Zealand seed plant flora is likely to be suitable for ex situ conservation in a conventional seed bank, and thus increased investment in the technologies and seed collection efforts will have positive conservation outcomes. However, considerable work must be done to further our understanding of seed storage behaviours of New Zealand species and to develop protocols for alternative ex situ conservation strategies for the many recalcitrant and intermediate species within the flora, especially for non-orthodox rain forest species such as Agathis australis and Syzygium maire that are acutely threatened by the novel pathogens Phytophthora agathidcida and A. psidii, respectively. Ex situ collections can provide valuable material for restoration programmes, and act as an important ‘back-up’ for those species that remain critically threatened in situ due to habitat loss, pathogens, and invasive species.
Data availability
Data obtained from Seed Information Database are available via the TRY Plant Trait Database (https://www.try-db.org) and from https://ser-sid.org/. Data obtained via literature review are available on FigShare (https://figshare.com/articles/dataset/Data_Can_seed_banking_assist_in_conserving_the_highly_endemic_New_Zealand_indigenous_flora_/24439102; doi:10.6084/m9.figshare.24439102.v1).
Declaration of funding
This research was funded by the New Zealand Ministry of Business, Innovation and Employment (Ngā Rākau Taketake – Myrtle Rust and Kauri Dieback Research, grant number C09X1817) through New Zealand’s Biological Heritage National Science Challenge. The Royal Botanic Gardens, Kew is part-funded by Grant in Aid from the United Kingdom’s Department for Environment Food and Rural Affairs.
Author contributions
SVW and PJB conceived the ideas. SVW conducted the analyses and led the writing of the manuscript. TFC undertook the literature review and data compilation. TRE designed and undertook the analyses to produce regional flora lists, and to obtain estimates of woody species environmental habitat parameters. AF and JBD provided data. PJB obtained the funding. All authors contributed to writing the manuscript.
Acknowledgements
We acknowledge the use of data drawn from the Natural Forest plot data collected between January 2009 and March 2014 by the LUCAS programme for the Ministry of the Environment. We are grateful to Sarah Richardson for the provision of the mean basal area data generated from the LUCAS plots, and for helpful and stimulating discussions.
References
Bellingham PJ, Richardson SJ, Gormley AM, Allen RB, et al. (2020) Implementing integrated measurements of essential biodiversity variables at a national scale. Ecological Solutions and Evidence 1, e12025.
| Crossref | Google Scholar |
Berjak P, Pammenter NW (2008) From Avicennia to Zizania: seed recalcitrance in perspective. Annals of Botany 101, 213-228.
| Crossref | Google Scholar | PubMed |
Brandt AJ, Bellingham PJ, Duncan RP, Etherington TR, et al. (2021) Naturalised plants transform the composition and function of the New Zealand flora. Biological Invasions 23, 351-366.
| Crossref | Google Scholar |
Carl Freeman D, Harper KT, Kent Ostler W (1979) Ecology of plant dioecy in the intermountain region of Western North America and California. Oecologia 44, 410-417.
| Crossref | Google Scholar |
Carpenter JK, Kelly D, Moltchanova E, O’Donnell CFJ (2018a) Introduction of mammalian seed predators and the loss of an endemic flightless bird impair seed dispersal of the New Zealand tree Elaeocarpus dentatus. Ecology and Evolution 8, 5992-6004.
| Crossref | Google Scholar | PubMed |
Carpenter JK, Wood JR, Wilmshurst JM, Kelly D (2018b) An avian seed dispersal paradox: New Zealand’s extinct megafaunal birds did not disperse large seeds. Proceedings of the Royal Society B: Biological Sciences 285, 20180352.
| Crossref | Google Scholar |
Carpenter JK, Wilmshurst JM, McConkey KR, Hume JP, Wotton DM, Shiels AB, Burge OR, Drake DR (2020) The forgotten fauna: native vertebrate seed predators on islands. Functional Ecology 34, 1802-1813.
| Crossref | Google Scholar |
Chamberlain SA, Boettiger C (2017) R, Python, and Ruby clients for GBIF species occurrence data. PeerJ Preprints 5, e3304v3301.
| Crossref | Google Scholar |
Chau MM, Chambers T, Weisenberger L, Keir M, Kroessig TI, Wolkis D, Kam R, Yoshinaga AY (2019) Seed freeze sensitivity and ex situ longevity of 295 species in the native Hawaiian flora. American Journal of Botany 106, 1248-1270.
| Crossref | Google Scholar | PubMed |
Conn JS, Wentworth TR, Blum U (1980) Patterns of dioecism in the flora of the Carolinas. The American Midland Naturalist 103, 310-315.
| Crossref | Google Scholar |
Coomes DA, Allen RB, Scott NA, Goulding C, Beets P (2002) Designing systems to monitor carbon stocks in forests and shrublands. Forest Ecology and Management 164, 89-108.
| Crossref | Google Scholar |
Craig J, Anderson S, Clout M, Creese B, Mitchell N, Ogden J, Roberts M, Ussher G (2000) Conservation issues in New Zealand. Annual Review of Ecology and Systematics 31, 61-78.
| Crossref | Google Scholar |
Crawford AD, Steadman KJ, Plummer JA, Cochrane A, Probert RJ (2007) Analysis of seed-bank data confirms suitability of international seed-storage standards for the Australian flora. Australian Journal of Botany 55, 18-29.
| Crossref | Google Scholar |
Davies RM, Hudson AR, Dickie JB, Cook C, O’Hara T, Trivedi C (2020) Exploring seed longevity of UK native trees: implications for ex situ conservation. Seed Science Research 30, 101-111.
| Crossref | Google Scholar |
Daws MI, Garwood NC, Pritchard HW (2005) Traits of recalcitrant seeds in a semi-deciduous tropical forest in Panama: some ecological implications. Functional Ecology 19, 874-885.
| Crossref | Google Scholar |
Daws MI, Garwood NC, Pritchard HW (2006) Prediction of desiccation sensitivity in seeds of woody species: a probabilistic model based on two seed traits and 104 species. Annals of Botany 97, 667-674.
| Crossref | Google Scholar | PubMed |
Dawson JW, Sneddon BV (1969) The New Zealand rain forest: a comparison with tropical rain forest. Pacific Science 23, 131-147.
| Google Scholar |
Elith J, Leathwick JR, Hastie T (2008) A working guide to boosted regression trees. Journal of Animal Ecology 77, 802-813.
| Crossref | Google Scholar | PubMed |
Etherington TR (2021) Mahalanobis distances for ecological niche modelling and outlier detection: implications of sample size, error, and bias for selecting and parameterising a multivariate location and scatter method. PeerJ 9, e11436.
| Crossref | Google Scholar | PubMed |
Ewers RM, Kliskey AD, Walker S, Rutledge D, Harding JS, Didham RK (2006) Past and future trajectories of forest loss in New Zealand. Biological Conservation 133, 312-325.
| Crossref | Google Scholar |
Fick SE, Hijmans RJ (2017) WorldClim 2: new 1-km spatial resolution climate surfaces for global land areas. International Journal of Climatology 37, 4302-4315.
| Crossref | Google Scholar |
GBIF.org (2022) GBIF occurrence download. Available at https://doi.org/10.15468/dl.ywg23n [Accessed 7 November 2022]
Grubb PJ, Bellingham PJ, Kohyama TS, Piper FI, Valido A (2013) Disturbance regimes, gap-demanding trees and seed mass related to tree height in warm temperate rain forests worldwide. Biological Reviews 88, 701-744.
| Crossref | Google Scholar | PubMed |
Heenan PB, McGlone MS (2019) Cenozoic formation and colonisation history of the New Zealand vascular flora based on molecular clock dating of the plastid rbcL gene. New Zealand Journal of Botany 57, 204-226.
| Crossref | Google Scholar |
Hijmans RJ (2002) terra: spatial data analysis. R package version 1.6-47. Available at https://CRAN.R-project.org/package=terra
Hijmans RJ, Phillips S, Leathwick J, Elith J (2017) dismo: species distribution modeling. R package version 1.1-4. Available at http://CRAN.R-project.org/package=dismo
Husheer SW, Coomes DA, Robertson AW (2003) Long-term influences of introduced deer on the composition and structure of New Zealand Nothofagus forests. Forest Ecology and Management 181, 99-117.
| Crossref | Google Scholar |
Kattge J, Bönisch G, Díaz S, Lavorel S, et al. (2020) TRY plant trait database – enhanced coverage and open access. Global Change Biology 26, 119-188.
| Crossref | Google Scholar | PubMed |
Kelly D, Ladley JJ, Robertson AW (2007) Is the pollen-limited mistletoe Peraxilla tetrapetala (Loranthaceae) also seed limited? Austral Ecology 32, 850-857.
| Crossref | Google Scholar |
Land Information New Zealand (2011) NZ Coastlines (Topo, 1:500k). Available at https://data.linz.govt.nz/layer/50204-nz-coastlines-topo-1500k/ [Accessed 10 July 2019]
Li D-Z, Pritchard HW (2009) The science and economics of ex situ plant conservation. Trends in Plant Science 14, 614-621.
| Crossref | Google Scholar | PubMed |
Liu U, Cossu TA, Davies RM, Forest F, Dickie JB, Breman E (2020) Conserving orthodox seeds of globally threatened plants ex situ in the Millennium Seed Bank, Royal Botanic Gardens, Kew, UK: the status of seed collections. Biodiversity and Conservation 29, 2901-2949.
| Crossref | Google Scholar |
McGlone MS (1983) Polynesian deforestation of New Zealand: a preliminary synthesis. Archaeology in Oceania 18, 11-25.
| Crossref | Google Scholar |
McGlone MS, Richardson SJ (2022) Sexual systems in the New Zealand angiosperm flora. New Zealand Journal of Botany
| Crossref | Google Scholar |
McGlone MS, Buitenwerf R, Richardson SJ (2016) The formation of the oceanic temperate forests of New Zealand. New Zealand Journal of Botany 54, 128-155.
| Crossref | Google Scholar |
McGlone MS, Heenan PB, Perry GLW (2024) Eco-evolutionary priority and the assembly of the New Zealand flora. Journal of the Royal Society of New Zealand 54(1), 124-143.
| Crossref | Google Scholar |
Mounce R, Smith P, Brockington S (2017) Ex situ conservation of plant diversity in the world’s botanic gardens. Nature Plants 3, 795-802.
| Crossref | Google Scholar | PubMed |
Mwang’Ingo PL, Teklehaimanot Z, Maliondo SM, Msanga HP (2004) Storage and pre-sowing treatment of recalcitrant seeds of Africa sandalwood (Osyris lanceolata). Seed Science and Technology 32, 547-560.
| Crossref | Google Scholar |
Nadarajan J, van der Walt K, Lehnebach CA, Saeiahagh H, Pathirana R (2021) Integrated ex situ conservation strategies for endangered New Zealand Myrtaceae species. New Zealand Journal of Botany 59, 72-89.
| Crossref | Google Scholar |
Nic Lughadha E, Bachman SP, Leão TCC, Forest F, et al. (2020) Extinction risk and threats to plants and fungi. Plants, People, Planet 2, 389-408.
| Crossref | Google Scholar |
Nugent G, Fraser W, Sweetapple P (2001) Top down or bottom up? Comparing the impacts of introduced arboreal possums and ‘terrestrial’ ruminants on native forests in New Zealand. Biological Conservation 99, 65-79.
| Crossref | Google Scholar |
Ogden J (1985) An introduction to plant demography with special reference to New Zealand trees. New Zealand Journal of Botany 23, 751-772.
| Crossref | Google Scholar |
Ogden J (1995) The long-term conservation of forest diversity in New Zealand. Pacific Conservation Biology 2, 77-90.
| Crossref | Google Scholar |
Olson DM, Dinerstein E, Wikramanayake ED, Burgess ND, et al. (2001) Terrestrial ecoregions of the world: a new map of life on earth. BioScience 51, 933-938.
| Crossref | Google Scholar |
Pannell JL, Buckley HL, Case BS, Norton DA (2021) The significance of sheep and beef farms to conservation of native vegetation in New Zealand. New Zealand Journal of Ecology 45, 3427.
| Crossref | Google Scholar |
Pebesma E (2018) Simple features for R: standardized support for spatial vector data. The R Journal 10, 439-446.
| Crossref | Google Scholar |
Probert RJ, Daws MI, Hay FR (2009) Ecological correlates of ex situ seed longevity: a comparative study on 195 species. Annals of Botany 104, 57-69.
| Crossref | Google Scholar | PubMed |
R Core Team (2021) ‘R: a language and environment for statistical computing.’ (R Foundation for Statistical Computing: Vienna, Austria) Available at https://www.R-project.org/
Richardson SJ, Holdaway RJ, Carswell FE (2014) Evidence for arrested successional processes after fire in the Waikare River catchment, Te Urewera. New Zealand Journal of Ecology 38, 221-229.
| Google Scholar |
Royal Botanic Gardens Kew (2017) Seed Information Database (SID). Version 7.1. Available at https://ser-sid.org/ [May 2017]
Sommerville KD, Clarke B, Keppel G, McGill C, Newby Z-J, Wyse SV, James SA, Offord CA (2018) Saving rainforests in the South Pacific: challenges in ex situ conservation. Australian Journal of Botany 65, 609-624.
| Crossref | Google Scholar |
Stats NZ (2019) Regional council 2019 (generalised). Available at https://datafinder.stats.govt.nz/layer/98763-regional-council-2019-generalised/ [Accessed 24 July 2019]
Sweetapple PJ, Nugent G, Whitford J, Knightbridge PI (2002) Mistletoe (Tupeia antarctica) recovery and decline following possum control in a New Zealand forest. New Zealand Journal of Ecology 26, 61-71.
| Google Scholar |
Thorsen MJ, Dickinson KJM, Seddon PJ (2009) Seed dispersal systems in the New Zealand flora. Perspectives in Plant Ecology, Evolution and Systematics 11, 285-309.
| Crossref | Google Scholar |
Tweddle JC, Dickie JB, Baskin CC, Baskin JM (2003) Ecological aspects of seed desiccation sensitivity. Journal of Ecology 91, 294-304.
| Crossref | Google Scholar |
van der Walt K (2023) Seed storage physiology of Lophomyrtus and Neomyrtus, two threatened Myrtaceae genera endemic to New Zealand. Plants 12, 1067.
| Crossref | Google Scholar | PubMed |
van der Walt K, Kemp P, Sofkova-Bobcheva S, Burritt DJ, Nadarajan J (2021a) Seed development, germination, and storage behaviour of Syzygium maire (Myrtaceae), a threatened endemic New Zealand tree. New Zealand Journal of Botany 59, 198-216.
| Crossref | Google Scholar |
van der Walt K, Peter K, Sofkova-Bobcheva S, Burritt D, Nadarajan J (2021b) Evaluation of droplet-vitrification, vacuum infiltration vitrification and encapsulation-dehydration for the cryopreservation of Syzygium maire zygotic embryos. CryoLetters 42, 202-209.
| Google Scholar |
van der Walt K, Burritt DJ, Nadarajan J (2022) Impacts of rapid desiccation on oxidative status, ultrastructure and physiological functions of Syzygium maire (Myrtaceae) zygotic embryos in preparation for cryopreservation. Plants 11, 1056.
| Crossref | Google Scholar | PubMed |
Varela S, Anderson RP, García-Valdés R, Fernández-González F (2014) Environmental filters reduce the effects of sampling bias and improve predictions of ecological niche models. Ecography 37, 1084-1091.
| Crossref | Google Scholar |
Walters C (2015) Orthodoxy, recalcitrance and in-between: describing variation in seed storage characteristics using threshold responses to water loss. Planta 242, 397-406.
| Crossref | Google Scholar | PubMed |
Webb CJ, Kelly D (1993) The reproductive biology of the New Zealand Flora. Trends in Ecology & Evolution 8, 442-447.
| Crossref | Google Scholar | PubMed |
Wilmshurst JM, Anderson AJ, Higham TFG, Worthy TH (2008) Dating the late prehistoric dispersal of Polynesians to New Zealand using the commensal Pacific rat. Proceedings of the National Academy of Sciences 105, 7676-7680.
| Crossref | Google Scholar |
Wyse SV, Dickie JB (2017) Predicting the global incidence of seed desiccation sensitivity. Journal of Ecology 105, 1082-1093.
| Crossref | Google Scholar |
Wyse SV, Dickie JB (2018) Taxonomic affinity, habitat and seed mass strongly predict seed desiccation response: a boosted regression trees analysis based on 17 539 species. Annals of Botany 121, 71-83.
| Crossref | Google Scholar |
Wyse SV, Dickie JB, Willis KJ (2018) Seed banking not an option for many threatened plants. Nature Plants 4, 848-850.
| Crossref | Google Scholar | PubMed |
Yukich Clendon OMM, Carpenter JK, Kelly D, Timoti P, Burns BR, Boswijk G, Monks A (2023) Global change explains reduced seeding in a widespread New Zealand tree: indigenous Tūhoe knowledge informs mechanistic analysis. Frontiers in Forests and Global Change 6, 1172326.
| Crossref | Google Scholar |
Appendix 1.List of dominant canopy tree species in New Zealand forests
Family | Species | Common name | Threat status | Seed storage behaviour | |
---|---|---|---|---|---|
Araliaceae | Pseudopanax arboreus | Whauwhaupaku, five finger | Not threatened | Recalcitrant | |
Araucariaceae | Agathis australis | Kauri | Threatened – nationally vulnerable | Intermediate | |
Arecaceae | Rhopalostylis sapida | Nīkau | Not threatened | Orthodox | |
Asteraceae | Brachyglottis rotundifolia | Pūwharetāiko, muttonbird scrub | Not threatened | Unknown | |
Asteraceae | Macrolearia colensoi | Tūpare, leatherwood | At risk – naturally uncommon | Unknown | |
Asteraceae | Olearia avicenniifolia | Mountain akeake | Not threatened | Unknown | |
Atherospermataceae | Laurelia novae-zelandiae | Pukatea | Not threatened | Recalcitrant | |
Coriariaceae | Coriaria arborea | Tutu | Not threatened | Orthodox | |
Cunoniaceae | Ackama rosifolia | Makamaka | Not threatened | Intermediate | |
Cunoniaceae | Pterophylla racemosa | Kāmahi | Not threatened | Orthodox | |
Cunoniaceae | Pterophylla silvicola | Towai | Not threatened | Intermediate | |
Cupressaceae | Libocedrus bidwillii | Kaikawaka | Not threatened | Orthodox | |
Elaeocarpaceae | Elaeocarpus dentatus | Hīnau | Not threatened | Recalcitrant | |
Fabaceae | Sophora chathamica | Kōwhai | Not threatened | Orthodox | |
Griseliniaceae | Griselinia littoralis | Kāpuka | Not threatened | Recalcitrant | |
Lamiaceae | Vitex lucens | Pūriri | Not threatened | Orthodox | |
Lauraceae | Beilschmiedia tarairi | Taraire | Not threatened | Unknown | |
Lauraceae | Beilschmiedia tawa | Tawa | Not threatened | Recalcitrant | |
Meliaceae | Didymocheton spectabilis | Kohekohe | Not threatened | Recalcitrant | |
Myrtaceae | Kunzea ericoides s.l. | Kānuka | Threatened – nationally vulnerable | Orthodox | |
Myrtaceae | Leptospermum scoparium | Mānuka | Threatened – nationally vulnerable | Orthodox | |
Myrtaceae | Metrosideros robusta | Northern rātā | Threatened – nationally vulnerable | Orthodox | |
Myrtaceae | Metrosideros umbellata | Southern rātā | Threatened – nationally vulnerable | Orthodox | |
Nothofagaceae | Fuscospora cliffortioides | Tawhairauriki, mountain beech | Not threatened | Orthodox | |
Nothofagaceae | Fuscospora fusca | Tawhairaunui, red beech | Not threatened | Orthodox | |
Nothofagaceae | Fuscospora solandri | Tawhairauriki, black beech | Not threatened | Orthodox | |
Nothofagaceae | Fuscospora truncata | Tawhairaunui, hard beech | Not threatened | Unknown | |
Nothofagaceae | Lophozonia menziesii | Tawai, silver beech | Not threatened | Orthodox | |
Onagraceae | Fuchsia excorticata | Kōtukutuku | Not threatened | Orthodox | |
Paracryphiaceae | Quintinia serrata | Tāwheowheo | Not threatened | Unknown | |
Phyllocladaceae | Phyllocladus toatoa | Toatoa | Not threatened | Unknown | |
Podocarpaceae | Dacrycarpus dacrydioides | Kahikatea | Not threatened | Recalcitrant | |
Podocarpaceae | Dacrydium cupressinum | Rimu | Not threatened | Intermediate | |
Podocarpaceae | Halocarpus biformis | Pink pine | Not threatened | Unknown | |
Podocarpaceae | Lepidothamnus intermedius | Yellow silver pine | Not threatened | Unknown | |
Podocarpaceae | Manoao colensoi | Manoao, silver pine | Not threatened | Unknown | |
Podocarpaceae | Pectinopitys ferruginea | Miro | Not threatened | Orthodox | |
Podocarpaceae | Podocarpus totara | Tōtara | Not threatened | Recalcitrant | |
Podocarpaceae | Prumnopitys taxifolia | Mataī | Not threatened | Orthodox | |
Proteaceae | Knightia excelsa | Rewarewa | Not threatened | Orthodox | |
Rubiaceae | Coprosma arborea | Māmāngi | Not threatened | Unknown | |
Strasburgeriaceae | Ixerba brexioides | Tāwari | Not threatened | Recalcitrant | |
Violaceae | Melicytus ramiflorus | Māhoe | Not threatened | Orthodox |
Note: Taxonomy follows New Zealand Plant Conservation Network (www.nzpcn.org.nz).