Timor-Leste: preliminary assessment of a rapidly eroding landscape in the Coral Triangle
R. J. Wasson
A
B
C
D
E
F
G
H
I
Abstract
Sedimentation in the rivers and on the coasts of Timor-Leste has increased since deforestation, affecting floods and probably living aquatic resources.
To provide scientifically based estimates of long-term and short-term erosion rates to assess the apparent role of deforestation, a topic that has not received sufficient quantitative attention in the Coral Triangle.
Short-term erosion rates have been estimated from regional relationships between river sediment yield and catchment areas and cosmogenic nuclides for long-term rates. An attempt has also been made to estimate changes in rates of delta progradation to determine whether recent increases have occurred following deforestation.
The major scientific finding is that reduction in vegetation cover for agriculture and timber harvesting, particularly in the mountains, increased sediment yield by factors up to 120, mainly by landsliding. And there has been a large amount of sediment exported to the delta and offshore from river-channel change.
Impacts on living resources are likely both in rivers and on the coast, but require assessment. Revegetation of hillslopes is likely to reduce these impacts and improve the livelihoods of local people.
Keywords: Coral Triangle, deforestation, erosion, floods, land cover, living resources, sedimentation, Timor-Leste.
Introduction
The research question that will be partially answered in this paper is as follows: has land cover reduction in Timor-Leste increased rates of erosion and sediment transport, with impacts on rivers and the coastal zone, as a prelude to investigating the impacts on human livelihoods? To answer this question, a catchment to coast approach has been adopted, in which long-term rates of river catchment erosion, modern erosion rates, the sources of sediment reaching the river, delta and offshore, and impacts of increased erosion on river dynamics, delta growth, and mangroves were assessed. These phenomena are considered in relation to vegetation-cover change in Timor-Leste. It is acknowledged that in this country, where data are extremely limited, our methods produce uncertain estimates. Nonetheless, we contend that these estimates can guide not only future research but also catchment management targets.
As a contributor to both food insecurity and natural hazards, erosion and sediment transport have long been identified as major problems facing Timor-Leste (Ormeling 1955; Gonçalves 1963; Metzner 1977; Sandlund et al. 2001; National Directorate of Forestry and Water Resources and Ministry of Agricuture, Forestry and Fisheries 2004). But the combination of tectonically induced uplift, high and often intense rainfall, short and often steep rivers, major changes in land cover, and intensive land use in Timor-leste makes the identification of a role for land-cover change challenging, involving a broad range of investigative tools to provide sound results. This problem also applies to Indonesia, Malaysia, Philippines and Papua New Guinea, an area that produces some of the world’s highest rates of erosion and sediment discharge to the oceans (Milliman et al. 1999; Milliman and Farnsworth 2011; Alongi et al. 2013a).
Suárez-Castro et al. (2021) found that of the 82 countries with coral reefs, the vast majority of catchments with the highest sediment exports to the oceans are in South-east Asia. Catchments in central and eastern Indonesia and Timor-Leste account for 39% of sediment export to the Coral Triangle. As Earth warms, more intense rain is likely to accentuate erosion (Barnett et al. 2007; Asian Development Bank 2014) and increase the transport of sediment into rivers, to the coast and into the sea (see Neverman et al. 2023 for a modelling framework to estimate such fluxes, which could be applied in the future). Increased sediment transport will have impacts on deltas, coral reefs, sea-grass, mangroves and associated food resources important to local people. Sedimentation in the coastal zone is a major threat (and possibly a partial benefit by enlarging deltas) in the Coral Triangle (which includes Timor-Leste). This is a region of high marine biodiversity (Hughes et al. 2002; Coral Triangle Initiative 2009; Asian Development Bank 2014; Coral Triangle Initiative on Coral Reefs, Fisheries and Food Security Secretariat 2022). Burke et al. (2012) found that 45% of the coral reefs in the Coral Triangle are threatened from land-based pollution, including sediment, nutrients and pollutants, and 15% are highly threatened. The call by both the Coral Triangle Initiative (CTI) and the Arafura Timor Seas Ecosystem Action (ATSEA) Program Phase 2 (see https://atsea-program.com, accessed 20 July 2023) for evidence-based management is one to which this paper contributes.
Land-based sediment affects coral reefs by reducing coral cover, diversity, colony size and structural complexity, and increases bioerosion and coral disease (Carlson et al. 2019; Naciri et al. 2023). Bleaching can be reduced by sediment deposition but reductions in mortality by this process are outweighed by mortality from low-light periods (Fisher et al. 2019). Orlando and Yee (2017) found that high sediment delivery to coral reefs decreased most ecosystem services, including bioprospecting discovery, fisheries and recreational opportunities.
Restoration of coral reefs is in part dependent on restoration of adjacent catchments to reduce sediment output (Delevaux et al. 2019). The land-sea, ridge-to-reef or catchment-to-coast approach is clearly needed, given that forest cover in catchments has a positive statistical relationship with coral condition, showing that restoration of catchments will have a beneficial effect on coral (Carlson et al. 2019). The same conclusion may also apply to seagrass ecosystems (Al-Asif et al. 2022). Suárez-Castro et al. (2021) found that the greatest opportunity for catchment restoration worldwide is in the Coral Triangle.
The research by Suárez-Castro et al. (2021) is important but their erosion model calculates erosion rates only from rill and sheet processes, although they noted the possible importance as sediment sources of gully erosion and streambank erosion but did not mention landslides. Among many modelled catchments, their model underestimates the sediment yield from the measured loads in the Purari and Fly rivers in Papua New Guinea by factors of 8 and 33 respectively. Landslides are common in both catchments (Blong 1986; Pickup and Marshall 2008), which explains the underestimation of sediment yield. The same conclusion may apply to many catchments in the Coral Triangle, thereby underscoring the need not just for modelled estimates based on simplifying assumptions, but also empirical studies of sediment sources. Otherwise, restoration projects will not be able to target the major sediment sources and will be ineffective. The focus on land cover is important because it can be changed by land management, whereas many of the other phneomena that contribute to erosion and sedimentation, such as tectonic uplift, cannot be changed.
The study site and drivers of change
Timor-Leste is the eastern part of the island of Timor, part of the Lesser Sunda Islands. It has an area of nearly 15,000 km2, a mountainous backbone rising to 2963 m above sea level, and short steep rivers draining to either side of the mountains in the western half of the country, and lower gradient rivers in the eastern part where the relief is smaller. River deltas occur mainly on the southern coast. The climate is tropical with distinct wet and dry seasons and little temperature variation, strongly influenced by the West Pacific Monsoon. ENSO can vary annual rainfall by up to 50% (see https://climateknowledgeportal.worldbank.org/country/timor-leste/climate-data-historical, accessed 9 October 2024).
The main drivers of change in the erosion–sediment transport–sedimentation cascading system of Timor-Leste are uplift, the natural background erosion rate, vegetation-cover change, climate, streamflow, coastal currents, and waves.
The island of Timor (which includes West Timor in Indonesia) is the result of collision between the Australian continent and the Banda Volcanic Island Arc (Tate et al. 2017). The area from just west of Timor to near 135°E is a small tectonic plate, bounded to the south by the Timor Trough and to the north by the Flores–Wetar Thrust, which is a nascent subduction zone (Rangin et al 1999; Bird 2003; Nugroho et al. 2009). Collision occurred at c. 8 million years ago (Coudurier-Curveur et al. 2021), producing thrust slices from both Australian continental rocks and seafloor igneous and sedimentary rocks. Deformation of these rocks occurred between 9.8 and 5.5 × 106 years ago (Keep and Haig 2010). Uplift was by either isostatic adjustment of the northward-moving subduction slab from c. 4.5 million years ago (Kaneko et al. 2007) or by overlapping of Australian continental thrust sheets below Banda Arc rocks to produce crustal thickening (Tate et al. 2017).
Timor-Leste may have emerged from the sea ~3.1 × 106 years ago (Keep and Haig 2010) or earlier at c. 4.4 × 106 years ago (Tate et al. 2017). Given that the highest land is ~3 km above sea level and, if we assume that there has been no loss of rock from above the highest point, the uplift rate after emergence has been between 0.7 and 1 mm year−1, depending on when emergence occurred. However, it is almost certain that erosion has removed rock from above the highest point, and so the uplift rate has been >1 mm year−1. Standley and Harris (2009) calculated a rock uplift rate of 1.5–2 mm year−1 for parts of Timor. Given the young age of the uplift, it is unlikely that erosion is in equilibrium with uplift and erosion rates, and therefore cannot be calculated from uplift rates (Willett and Brandon 2002). Erosion is therefore most likely to be <1–2 mm year−1. To deal with this uncertainty, direct estimates of erosion are provided here on the basis of beryllium-10 (10Be) measurements in river sediments.
The uplift of Timor-Leste was accompanied by complex tectonics where high-angle faults have created large scarps, such as the northern side of Mount Cablaci, on Mount Perdido and Mount Laritame, and probably the steep northern coast opposite Ata’Uro Island where, just offshore, the Wetar Strait reaches a depth of 3 km (Keep and Haig 2010). Earthquakes occur in the vicinity of Timor-Leste and uplift is probably still occurring (Gageonnet and Lemoine 1958; Nugroho et al. 2009). The Timor Trough off the southern coast is a possible location for great earthquakes (Major et al. 2013; Harris and Major 2016; Coudurier-Curveur et al. 2021), which could have devastating effects on both Timor-Leste and northern Australia.
Information about more recent uplift and deformation is also available. Uplifted coral terraces are common along the northern coast of Timor-Leste and less common on the southern coast (Kaneko et al. 2007). On the northern coast, Chappell and Veeh (1978) showed that over the past ≤150,000 years, average uplift rates have been ~0.3 mm year−1 near Dili, and ~0.5 mm year−1 between Manatuto and Lautem. Cox (2009) showed that over the same period, a rate of ~0.5–0.6 mm year−1 occurred over a distance of ~170 km east of Dili, with local rates being as high as 1.6 mm year−1 over distances of <15 km. The rate of ~0.5 mm year−1 at the mouth of the Laclo River at Manatuto is higher than the catchment erosion rate of 0.12 mm year−1 (averaged over 3800 years: see Table 1), probably because of spatial variation in uplift rate or disequilibrium between uplift and erosion. On the southern coast, there are no estimates of uplift rate. However, in both the Laclo and Caraulun-1 catchments (Fig. 1), there are river terraces up to ~150 m above the modern river, suggesting uplift on both sides of the country.
Catchment | Area (km2) | Average catchment slope (°) | Long-term denudation (erosion) rate (mm year−1) | Averaging time (years) | Bedload (%) | Modern erosion rate (mm year−1) | |
---|---|---|---|---|---|---|---|
Laclo | 1369 | 13.7 | 0.16 ± 0.03 (0.12) | 3800 | 27 | 2.5 | |
Caraulun-1 | 579 | 15.2 | 0.53 ± 0.16 (0.41) | 1200 | 31 | 3.6 | |
Caraulun-2 | 36 | 9.0 | 0.11 ± 0.05 (0.09) | 5500 | 34 | 10.8 | |
Be Lulic-1 | 352 | 16.5 | 0.07 ± 0.02 (0.06) | 8600 | 31 | 4.4 | |
Be Lulic-2 | 8 | 14.0 | 0.23 ± 0.20 (0.18) | 2600 | 39 | 21.3 | |
Tafara | 339 | 13.6 | 0.58 ± 0.26 (0.45) | 1000 | 31 | 4.5 | |
Rai Ketan | 80 | 13.3 | 0.54 ± 0.21 (0.42) | 1100 | 30 | 7.6 |
Long-term erosion data were corrected for solution loss. Be Lulic-2 is the upper part of Be Lulic-1.
Location map showing the catchments of the Laclo, Aileu, Caraulun-1 and -2, Be Lulic-1 and -2, Tafara, and Rai Ketan rivers, the Tafara and Caraulun-1 deltas, and key towns. Be Lulic-2 is the upper part of the Be Lulic catchment, which is Be Lulic-1.
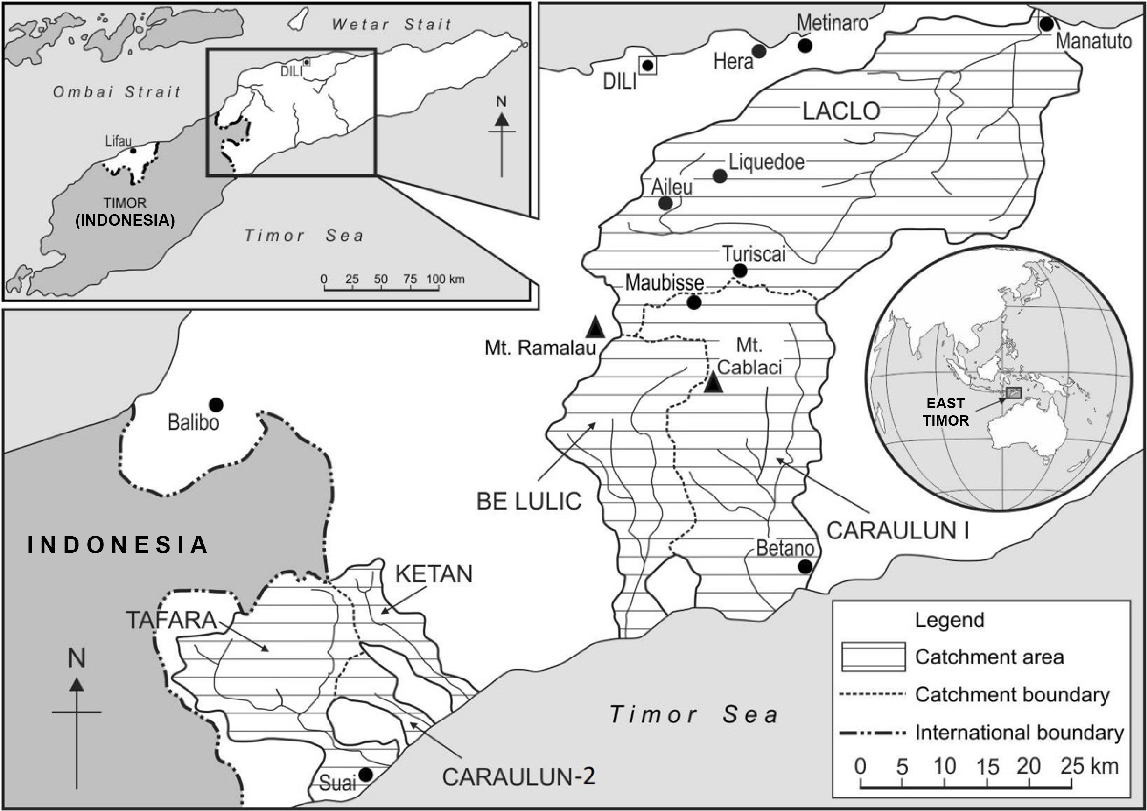
In 1989–1999, the areas of land-cover type in Timor-Leste were agriculture (36%), degraded woodland (22%), woodlands (19%), 12% forest and dense forest, heath or scrub 5% and other 6% (Bouma and Kobryn 2004). Shifting agriculture, settled agriculture, logging and fire have substantially altered the vegetation cover (Gageonnet and Lemoine 1958; Metzner 1977; Benevides 2003). Closed-canopy forests are now limited to hilltops and deep ravines where clearing and fire have had little effect.
Human land use has clearly had a profound effect on the vegetation of the country, the first evidence for which are the remains of pottery and domesticated animals that first appeared c. 3800–3600 years ago, after a long period of hunting and gathering (Spriggs et al. 2003; O’Connor 2006). The first use of cereals occurred after the introduction of pottery (Oliveira 2008). Clearing, burning and use of timber presumably increased after the introduction of agriculture. Maize was probably introduced by the Portuguese in the 16th century CE, and rice was already being grown at that time (Pigafetta 1969; Oliveira 2008), but it is not clear how widespread these crops were. Sandalwood was an important commodity for trade by 1436 CE when Chinese ships were travelling to Timor-Leste (McWilliam 2005), although the Chinese knew of Timor as early as 1250 CE (Ptak 1983). The quantities traded and effects of sandalwood removal on the landscape are unrecorded.
In 1861 CE, the English naturalist Alfred Russell Wallace (1869, chapt. 13) described the hills from the back of Dili to Balibar as ‘bare hills, whose surface was covered with small pebbles and scattered over with Eucalypti’. He went further to observe that:
…the Indigenous vegetation of Timor is poor and monotonous. The lower ranges of the hills are everywhere covered with scrubby Eucalypti, which only occasionally grow into lofty trees. Mingled with these in smaller quantities are acacias and the fragrant sandalwood, while the higher mountains, which rise to six or seven thousand feet [~1830–2125 m], are either covered with coarse grasses or are altogether barren. In the lower grounds are a variety of weedy bushes. In some of the valleys where the vegetation is richer, thorny shrubs and climbers are so abundant as to make the thickets quite impenetrable.
This a passable description of the present vegetation. When Forbes (1889) passed through Turiscai, in the upper Laclo River catchment, in the late 19th century, he observed that ‘The mountains of Turskain [sic] were everywhere covered with a rich carpet of green grass’. Some eucalypts grow there today, showing that the area is suitable for this genus. We can conclude therefore that deforestation of much of this area had already occurred by c. 130 years ago.
Coffee plants were introduced by Governor Celestino da Silva in the late 19th century CE after Portuguese control was secured following the final defeat of Dom Boaventura in Manufahi (Pelissier 1966). Significant areas were converted to coffee plantations thereafter, but the area and rate of development are unrecorded.
Erikstad et al. (2001) and Bouma and Kobryn (2004) quantified vegetation-cover change. Between 1983 and 1995, 40% of the existing forest cover of Timor-Leste was removed, woodland was reduced by 39%, degraded woodland increased by 214%, heath and shrub increased by 40% and agriculture decreased by 8%. Bouma and Kobryn (2004) attributed the changes to forest and woodland to commercial logging and policies of relocation of people during the Indonesian occupation, which increased pressure on timber resources by local people. Increased areas of degraded woodland are attributed to increased swidden agriculture as a consequence of increased population under the transmigration and relocation policies of the time.
McWilliam (2001) concluded ‘that inexorable encroachment and conversion of natural forest resources into swidden garden lands and secondary bushlands’ is the long-term history of forest in the island of Timor. The nature of the undisturbed vegetation is poorly known, except that the drier northern side of Timor-Leste was dominated by eucalyptus woodlands, the high peaks by heath, and the wetter southern side by mixed eucalyptus forest and broad-leaf rainforest. Away from villages, patches of dense closed-canopy forest and woodland in riparian zones still occur. Closer to villages, riparian forest has been replaced by swidden agriculture, settled agriculture, and shrubby secondary vegetation.
Climate is dominated by the Australian–Asian monsoon with a single wet season of 4–6 months beginning in December on the northern side of the country and two wet seasons on the southern side lasting in total for 7–9 months with peaks in December and May (Barnett et al. 2007). Annual rainfall is <1000 mm on the northern side, 1500–2000 mm in central and elevated areas, >2500 mm in the highest areas, and 1500–2000 mm on the southern slopes. Intense rainfall is common, and although tropical cyclones rarely affect Timor (Durand 2006) cyclone Seroja on 5 April 2021 produced many landslides and widespread flooding (Duffy et al. 2022). Variability of rainfall is largely related to the phases of ENSO with reduction of up to 50% in annual rainfall in El Niño years at some locations.
Trends in rainfall are difficult to detect because most records are short. The longest record is from 1952 to 2011 at Dili airport, with data missing for 1975, 1976, 2001–2003. At this station, there is no evidence for a trend in annual totals (Asian Development Bank 2014). For nine other stations in the period 2004–2012, rainfall was, on average, 19% lower than in the period 1954–1974 (from Portuguese and the records of Ministry of Agriculture and Fisheries, Government of Timor-Leste).
Portuguese records of streamflow are available for the period 1952–1974 (Alongi et al. 2012). For the Laclo River, the mean annual discharge was 29.3 m3 s−1 (range, 49.3–11.8), for the Be Lulic-1 River it was 11.85 m3 s−1 (range, 32.9–2.2), for the Caraulun-1 River 12.25 m3 s−1 (range, 22.5–5.8), and for the Tafara River 6.9 m3 s−1 (range, 20.4–0.3) (Fig. 1). Data are not available for the Caraulun-2, Be Lulic-2, or the Rai Ketan rivers. The mean annual suspended sediment concentrations for these rivers range from 12 to 20 kg m−3, calculated from the mean monthly discharges and the estimated sediment loads in Table 1; see below for explanation of these data. It is highly likely that much higher concentrations occur at or near peak flows that are not recorded in the summary data.
Coastal currents and waves that can interact with riverine flows to affect the growth of beaches and deltas, with impacts on coastal ecosystems, are also drivers of change. The recently increased erosion rates that are documented in Table 1 are likely to have increased the rate of coastal progradation, especially on the deltas of the Caraulun-1 and Tafara rivers that are symmetrical about their river mouths. In the absence of measured coastal currents or waves and their transport of sediment, the symmetrical deltas are taken as evidence of dominance by riverine input (Bhattacharya and Giosan 2003) rather than by alongshore transport of sediment. Also, the sandy beach ridges on the deltas are evidence that waves have transported sediment of riverine origin onto the delta fronts.
Materials and methods
Erosion rates and sediment yields
Most analysis has been in the following two river catchments: the Laclo on the northern coast, which enters the sea at Manatuto, and Caraulun-1 on the southern coast, which enters the sea a little west of Betano. Additional data have been obtained in the catchments of the rivers Be Lulic-1 and 2, Caraulun-2, Tafara and Rai Ketan (Fig. 1).
Natural rates of denudation on medium to long time scales have been determined from the 10Be content of quartz in the sediment of the rivers listed above. The concentration of 10Be in the quartz fraction of river sediment is a function of the ratio of 10Be production (in soil and bedrock in a catchment) and denudation (Granger and Riebe 2014). Cosmic ray production of 10Be by nuclear spallation and negative muon capture by oxygen nuclei essentially occurs in the upper few metres of rock and soil. Quartz has abundant O and is resistant to chemical alteration, and so is an ideal material for this analysis. The 10Be concentration (C10) is inversely proportional to the denudation rate (ε), as follows:
where Po is the surface production rate of 10Be, which depends on the intensity of secondary cosmic rays, which in turn is a function of latitude, altitude and topographic shielding (see Schaller et al. 2001); λ is the decay constant of 10Be, ρ is the density of rock, and Λ is the attenuation length, a measure of the exponential attenuation of cosmic rays with depth. For current purposes λ can be ignored. 10Be was extracted using the method of Kohl and Nishiizumi (1992) and measured by accelerator mass spectrometry (AMS) at the Australian National University (Fifield et al. 2010). Using geological maps and topographic data in a geographic information system, a correction was applied for the non-quartz-rich rock (limestone) in the catchments, but the calculated denudation rates are likely to apply to the entire catchments because the relief between the quartz-rich and quartz-poor areas is similar. The variation of 10Be production with altitude was taken into account from topographic data within a Geographical Information System, by using the altitude-scaling factors of Lal (1991). A potential complication is that alluvium stored in river terraces will have higher 10Be concentrations in the upper 1 m because of irradiation by cosmic rays during sediment storage. When released by erosion, these deposits could bias the denudation rate calculations. However, alluvial terraces occupy small areas in the catchments and are therefore unlikely to affect the calculations (see Granger and Riebe 2014). Extreme erosion events may have occurred because of rainfall or earthquakes and may bias the calculated long-term denudation rates by diluting cosmogenic nuclide concentrations in river sediments. This is an unlikely problem in Timor-Leste according to the analysis by Schide et al. (2022) because of high background erosion and long-term landsliding rates and high connectivity of hillslopes to river channels. Dilution of cosmogenic nuclides is therefore unlikely, meaning that cosmogenic tracers will not detect extreme events in this landscape. However, further analysis using the methods of Deng et al. (2021) could be beneficial.
Modern (i.e. decadal) rates of sediment transport have been determined from the following relationship for South-east Asia (Milliman et al. 1999):
where r2 = 0.77, y is mean annual suspended sediment load and x is catchment area (103 km2). The catchments from which this equation has been derived have sizes similar to those in Timor-Leste and the land-use impacts are also substantial. This is the only available method for this calculation because of a lack of measred river discharges and sediment concentrations.
In Timor-Leste, the bedload component is likely to be more than the usually assumed 10% because of landslides that entrain coarse sediment, incision of bedrock by upland channels, and erosion of river terraces that contain previously deposited bedload. Also, at the Caraulun-1 River irrigation headworks in the lowland reach, <5% of the sediment in the riverbed is silt and clay, and the remainder is sand and gravel with a maximum of 20 cm (Snowy Mountains Engineering Corporation 2002). Further upstream, boulders several metres in diameter are common in the riverbed, and in all other rivers examined. The suspended loads calculated from Eqn 2 have been converted to kilograms per second to enable estimation of the bedload percentages from the global compilation of data by Turowski et al (2010). The mean bedload fraction for all of the catchments in Table 1 is 32 ± 5%.
Sediment-source tracing
Sediment sources were determined by using the tracers 137Cs and 210Pb(ex) to estimate the proportion of the suspended load derived from sheet and rill erosion of hillslope topsoil (Wallbrink and Murray 1993; Wallbrink et al 1998) and, by difference, the proportion from other sources (landslides, gullies, roads, footpaths and riverbanks). The nuclides 137Cs, 210Pb, 226Ra were measured at the Environmental Research Institute of the Supervising Scientist (see https://www.dcceew.gov.au/science-research/supervising-scientist/ranger-mine/research) in Darwin in the <20-μm fraction by high-resolution gamma spectrometry (using the methods of Marten 1992). Although the activities are low, none was below the detection limit. Each sample from hillslopes consisted of ~100 subsamples over an area of ~100 m2 from the upper 1 cm of sediment recently mobilised by sheet erosion on hillslopes. Each riverbed sample also consisted of ~100 subsamples from pockets of mud between gravel particles taken over a distance of approximately five times the channel width to obtain a representative sample. Twenty samples were taken each from hillslopes and riverbeds.
On hillslopes, from near the coasts to the mountains in the two catchments, 80% of samples were from uncultivated and grazed sites, and 20% from cultivated sites; approximately in the proportions of uncultivated and cultivated areas in the two catchments. A more systematic sampling approach, such as that suggested by Wilkinson et al. (2015), was not possible because of access problems. That said, the sampling is believed to be representative of the major landscape and land-use types.
River-channel changes
Changes to the rivers in the Caraulun-1 catchment and delta were determined by comparison of Landsat thematic mapper 25- × 25-m moderate-resolution satellite images for 1986, 1996 and 2006. The images were orthorectified and an atmospheric correction applied to subtract dark objects, ocean water was masked out, and spectral enhancement applied by break-point analysis. The normalised difference vegetation index (NDVI) was calculated using density slicing and Erdas IMAGINE software (ver. 9.3.2, HEXAGON, Stockholm, Sweden) and checked using 70 ground points. The classes ‘riverbed’ and ‘bare’ were used to digitise the Caraulun River and tributaries to detect any changes of width and location.
Delta changes
Beach ridges on the Caraulun-1 delta at Betano (Fig. 2, 3) and on the delta of the Tafara River (Fig. 3) were sampled for optically stimulated luminescence (OSL) dating to derive estimates of changes to delta progradation rates, from which can be inferred changes in transport of riverine sediment to the coast. OSL ages for the Caraulun-1 delta samples were calculated from infrared stimulated luminescence (IRSL) signals from large aliquots of polymineral samples (90–180 μm). These samples unexpectedly showed no response to standard green-light OSL (indicating an absence of responsive quartz) by using the standard single aliquot regeneration (SAR) protocol (Murray and Roberts 1998). Anomalous fading is likely to be a small effect, given the low doses. However, the possibility of some laboratory-induced bleaching cannot be excluded because the samples were prepared under conditions appropriate for standard quartz OSL rather than feldspar IRSL. Burial doses were calculated using the probability density function (Gaussian) technique of Pietsch (2009). An internal dose rate from feldspars of 0.5 grays per thousand years has been assumed. Burial doses were calculated using the PDF (Gaussian) technique of Pietsch (2009). Dosimetry was based on high-resolution gamma spectrometry and calculated cosmic ray doses (Prescott and Hutton 1994). An external dose rate from feldspars of 0.5 grays per thousand years has been assumed. Given the unexpected lack of responsive quartz in these samples, and the uncertainty around the appropriateness of the laboratory conditions for feldspar, these results are indicative only.
Delta of the Caraulun-1 River. The Old River Channel is being reoccupied by the modern river through a breach in the riverbank.
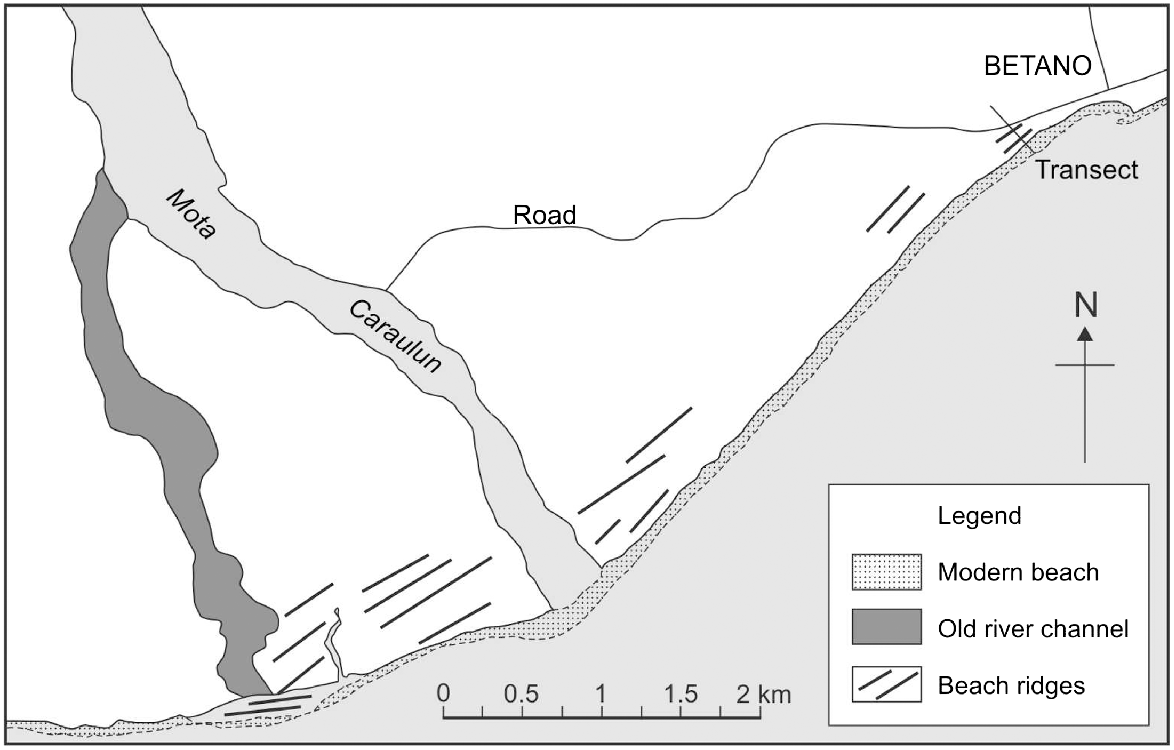
For the samples from the Tafara delta (Fig. 4, 5), carbonates, the organic fraction and heavy minerals were removed using hydrochloric acid (HCl) and hydrogen peroxide (H2O2), and by density separation with sodium polytungstate. Thereafter, the 150–210 μm fraction was used to obtain quartz grains. These were etched in 40% hydrofluoric acid (HF) for 80 min followed by a treatment with 12 N HCl and sieved again to get the fraction >150 μm. These quartz grains gave appreciable IRSL despite repeat HF etching and therefore a double-SAR protocol was used (Banerjee et al. 2001; Jain and Singhvi 2001) in a five point SAR protocol. Small aliquots of a few tens of grains each were used because the low sensitivity and the relatively young ages of the samples did not permit single-grain analysis. The typical recycling ratio was 1 ± 0.1 and the thermal transfer signal was negligible. Dose recovery tests gave values within 10% of the imparted dose. The regeneration growth curves were fit to a saturating exponential. The distribution of equivalent doses on histograms indicated that the samples were partially bleached, so the minimum equivalent doses (average of minimum paleodose and doses lying within a minimum ±2σ range) were used to derive the ages (Juyal et al. 2006). Some results have been derived from these dates (see below).
Results and discussion
Erosion rates
Distinguishing between natural and human-induced erosion and sedimentation in tectonically active landscapes of high relief is challenging (National Research Council 2010). The approach here is to use the only two data sources to make comparisons of erosion rates over different time scales.
The millennial denudation rates based on 10Be range from 0.07 ± 0.02 mm year−1 (Be Lulic-1) to 0.58 ± 0.26 mm year−1 (Tafara) (Table 1). These rates include both physical erosion and solute loss. The high total denudation rates in Table 1 are in a regime where weathering is limited by the kinetics of chemical reactions rather than the supply of weatherable minerals (Gabet and Mudd 2009). In this regime, solute loss is approximately constant and averages ~20% of the total denudation rate. In Table 1, the long-term denudation rates have been reduced by 20% to enable comparison with the modern rates, which are for sediment only. The long-term erosion rates are in parentheses after the long-term denudation rates in Table 1.
The millennial rates are averages over 1000–8600 years, known as integration times or the time for 60 cm of denudation to occur (Granger and Riebe 2014). Although there were undoubtedly some human influences in the catchments over these time periods, apparently land-cover change has been substantial only in the past ~100 years, a period representing an average of 5% of the integration times. These erosion rates are therefore either close to or at the natural rate and provide a baseline against which to compare modern rates. By using non-parametric Spearman correlation (see Zar 2005 for the background and method of calulation), statistically significant relationships between the natural and millennial denudation rates and catchment area, relief (not shown in Table 1), and catchment slope (calculated by the method of Montgomery and Brandon 2002) exist only between modern rates and catchment area because that relationship is the basis of the estimates of sediment yields (Eqn 1).
The average long-term erosion rate for the seven catchments in Table 1 is 0.25 ± 0.07 mm year−1. This value is lower than any of the estimated uplift rates, suggesting that erosion and uplift are not in equilibrium, a conclusion supported by a lack of relationship between long-term erosion rates and topographic measures, albeit based on few data.
Additional factors need to be considered. First, the millenial erosion rates are averaged over periods during which climate change has occurred in Timor-Leste (Haberle and David 2004; Bourke et al. 2007). Therefore, erosion rates have probably varied over the same periods. Second, the various estimates of uplift rate are insufficient to be definitive about their spatial variability, although it seems to be large (Tate et al. 2014).
The likely high spatial variability of uplift, relief and vegetation cover appears to be reflected in the difference between modern erosion rates and natural rates. Modern rates are higher than natural rates by factors between 9 and 120 (Table 1). The estimated specific sediment yields range from ~180 to 1400 Mg km−2 year−1.
Sediment sources
According to a qualitative account by Audley-Charles (1968), the major erosion forms in the Laclo and Caraulun-1 catchments are landslides, gullies, sheet erosion, stream bank failure and earthflows. Landslides, usually only a few metres deep, of various types are common, as found in a quantitative analysis by Soares (2007) who found that most landslides occurred on sedimentary rocks, and were infrequent on igneous, metamorphic (e.g. Aileu Formation) and volcanic rocks and associated soils. Soares et al. (2011) found, in two areas that include parts of the southern catchments in Fig. 1, that most landslides occurred in clay-rich rocks and soils on steep slopes and areas of cultivation. They also found that most landslide erosion occurs by infrequent large events. Duffy et al. (2022) mapped more than 2000 landslides across the country and found that they mostly occur on steep areas where weak schists, shales and mélange (blocks of rock in scaly clay) occur. Soares (2007) also found that most slope failures occurred on bare or grassed land, and least under woodland. Most failures occurred on slopes between 12 and 36°, with fewer occurring on slopes of 6–12°, and also on slopes of 36–48° where bedrock dominates. From the observations of the authors of this paper, gullies occur on all rock types but are rare, whereas streambank erosion of floodplains, river terraces and bedrock is common. Evidence of sheet erosion occurs on all formations except the Ainaro Gravels and Alluvial Terraces.
Quantitative estimates of the sources of modern river sediments were determined first by calculating the proportion of topsoil in the fine-grained fraction of deposited material in riverbeds. This fraction is derived mostly by sheet and rill erosion of hillslopes that are connected to the channel network. Of the 20 hillslope surface soil samples analysed for 137Cs and 210Pb(ex), 19 were from the Laclo and Caraulun-1 catchments, and one from a hillslope above Hera, a few kilometres east of Dili. The values for the sample from near Hera were within the range of the other samples and were therefore included in the analysis.
The weighted average value of 137Cs activity for the hillslope samples was 2.11 ± 0.26 Bq kg−1 for the <20μm fraction. The lowest value was 0.76 ± 0.09 Bq kg−1 on a grassed, grazed slope of ~9° on Mount Fleisha in the Caraulun-1 catchment. The highest value was 33.3 ± 3.0 Bq kg−1 on a scrub-covered slope of ~24° near Maubisse, also in the Caraulun-1 catchment. The concentration of 137Cs at a particular site appeared to depend on enrichment or depletion as a result of local erosion and deposition, rather than on rainfall and the orographic effect. This conclusion is based on the absence of a significant correlation (at P = 0.05, using a Spearman correlation) between nuclide concentration and either elevation or position relative to rainfall gradients. However, there was a weak positive correlation (P = ~0.05) between concentration and local slope, suggesting that most soil loss occurs on steep slopes but is not restricted to such slopes. The weighted average 210Pb(ex) value for the hillslopes was 247.5 ± 0.07 Bq kg−1 and had the same statistical relationships with slope and elevation as did the 137Cs results.
Of the 20 samples from riverbeds, 15 came from the Laclo and Caraulun-1 catchments and 5 from the Caraulun-2, Tafara, Be Lulic, Rai Ketan and Susane (near Hera) catchments. All the values from rivers other than the Laclo and Caraulun-1 catchments fell within the range of the values in these two catchments. The weighted average for 137Cs in the riverbed samples was 0.13 + 0.09 Bq kg−1 (<20 μm) and for 210Pb(ex) is 10.95 ± 0.09 Bq kg−1. Using the methods of Wallbrink and Murray (1993), the topsoil component of the fine-grained river sediment is therefore ~5% on the basis of 137Cs and ~4% based on 210Pb(ex). This estimate of 4–5% topsoil fraction of the fine-grained (suspended load) sediment in the rivers may be a slight overestimate of the sheet and rill erosion input because landslides and gullies produce sediment with a quantity of topsoil labelled by the two tracers. The best available estimate is that ~3% of the total sediment load is from topsoil, taking bedload into account, and therefore ~97% of the total load of the Laclo and Caraulun-1 rivers is derived by processes other than sheet and rill erosion, that is, from landslides, gullies, riverbank erosion, and roads and footpaths, each of which will now be considered. These calculations assume there is no 137Cs or 210Pb(ex) in the subsoils, a reasonable assumption given that most of the soils susceptible to erosion are Ultisols and Alfisols (Thompson 2011) that have dense clay B horizons into which the fallout nuclides are unlikely to penetrate. Most landslides are at most 2 m deep, as are gullies.
Landslides in Timor-Leste are produced by both earthquakes and rainfall. There are no measured rates of landsliding, therefore the earthquake-induced landslide quantity was estimated using the approach of Malamud et al (2004). Using their eqn 29:
where h is the erosion rate (mm year−1) triggered by landslides and b = (Mmax ± 1), an assumed seismic intensity (I4) of 1–2, and a maximum earthquake magnitude of 6 (see Das 2004; Ely and Sandiford 2010). The data on which this equation is based do not extend over millennia but probably relate to the past century, during which most deforestation occurred.
For the modern erosion rates, earthquake-induced landslides produce <1% of the total erosion rates in Table 1. These could be underestimates if great earthquakes can occur in the Timor Trough as suggested by Coudurier-Curveur et al. (2021). The United Nations Development Programme (2012) found that 50% of the country falls into a medium earthquake-landslide hazard zone and 1% in a high zone. The methods by which these estimates have been made are not clear, so will not be considered further.
From Rouwenhorst (2013), riverbank erosion in the Caraulun-1 catchment has been estimated from comparison of river areas between 1986 and 2006 by using satellite imagery. A differential analysis between Landsat images was combined with riverbank heights to estimate volumes of erosion. The average riverbank height was 2.8 ± 0.8 m on the basis of 48 measurements along both banks of the main river channel. Widening of tributaries is very small because they are in narrow steep-walled valleys, mostly in bedrock. They have therefore not been included in the estimate of channel erosion. The overall consumer’s accuracy for the land-cover classification on which this estimation was based was 88.6%. When calculating areas of erosion on the basis of pixels, there is inevitably a bias due to pixel size, methods used for classification, terrain (as reflected in the chosen DEM), and spectral resolution. Quantifying this bias is complex and requires detailed statistical modelling (Waldner and Defourny 2017), which requires another project. However, considering the river length and the size of the pixels, the error margin for the calculated areas of erosion is likely to be between ±10 and 30%, varying along the river network. The best estimate of riverbank erosion and, therefore, channel widening over the 20-year period is 2.9 ± 0.3–0.8% of the total annual average erosion rate of 3.6 mm year−1.
Roads and footpaths erode and some of the sediment reaches river channels. However, the spatial density of these sources is low, and therefore they are not considered further.
Sediment sources in the Caraulun-1 catchment
Sufficient data exist for a preliminary sediment-source budget for only one catchment, namely, Caraulun-1. The estimates apply to about the past century. The best estimates of the contributions to riverine sediment of different sources in this catchment are as follows: sheet and rill erosion produces ~3%, earthquake-induced landslides <1%, and the difference of 96% is mostly attributable to rainfall-induced landslides, gullies, roads and footpaths. As argued earlier, gullies, roads anf footpaths have low spatial density and it is therefore likely that rainfall-induced landslides are the major sediment source. Riverbank erosion contributes ~81 ± 10–33% of the total erosion in this catchment, most of the sediment from which has been deposited on the delta or transported offshore.
The assumption that all earthquake-induced landslide debris reaches channels, although unlikely, makes no substantial difference to the calculation given that this is a minor source, unless there have been great earthquakes in the Timor Trough in the past few millennia. From currently available data, ~96% of the river sediment is from rainfall-induced landslides, footpaths, roads and gullies. Gullies are rare and mostly in landslide scars and, therefore, can be thought of as part of the landslide contribution. Roads and footpaths are sparse and probably contribute very little sediment. Therefore, rainfall-induced landslides contribute most sediment to the river, a conclusion that probably applies to all of the high relief catchments in the western half of Timor-Leste. This conclusion is supported by clear evidence in the landscape of landslide scars. Duffy et al. (2022) produced a preliminary inventory and map of landslides from which an apparent inverse power law distribution appears to fit a graph of landslide area and frequency of occurrence (Soares et al. 2011), but not frequency in time. A major project would be required to estimate temporal frequency, on the basis of dated geomorphic evidence because there are no long-term documentary records. That river-channel change has contributed most of the erosion was unexpected, and calls for better documentation of the distribution of that material on the delta and offshore. It also invites an understanding of likely future changes to river width.
As a further step to refining the understanding of sediment sources, a slope map of the Laclo and Caraulun-1 catchments has been prepared (Fig. 5). The uppermost parts of the Caraulun-1 catchment and areas along the southern and south-eastern margin of the Laclo catchment have hillslopes of >30°. Such hillslopes are considered to be at the threshold of landsliding and controlled by the rate of uplift through the rate of vertical incision by rivers (Binnie et al. 2007), although Soares et al. (2011) argued that river incision is not a primary driver of landslide occurrence. Steep areas produce large quantities of sediment and may be the dominant sediment-source areas in both catchments (cf. Montgomery and Brandon 2002), particularly from slopes cleared of forest. Almost all this steep land occurs on the Lolotoi Formation, the most erodible part of which is weathered schist (Audley-Charles 1968).
The conclusion about increased sediment yield following deforestation in Timor-Leste could be tested by using dated fluvial deposits that record changes from before and after major land-cover change, similar to the approach of Wasson et al. (2008) in the central Himalaya. Similar deposits have not been found in Timor-Leste, but offshore deposits may provide an appropriate archive. The short, steep rivers, with gradients in the downstream 1 km of the studied rivers range from 0.0089 to 0.0105, probably transport sediment quickly from source to coast, and the time lag referred to earlier is likely to be small.
River-channel change
The results in Table 1 show that the modern erosion rates are 9–120 times the millennial rates. Because of the short averaging times for the millennial rates, adjustment for the different averaging times for the millennial and modern rates by using the method of Gardner et al. (1987) would produce little difference.
The increase is a factor of 21 in the Laclo catchment and 9 in the Caraulun-1. This increase should be reflected in both the rivers and at the coast because most of the sediment comes from landslides and therefore the sediment delivery from source to river is likely to be efficient (Knighton 1989; Sarker and Thorne 2006; cf. Madej et al. 2009). Alongi et al. (2006) showed that the Laclo River at Manatuto has shallowed and widened by erosion of riverbanks. The people living near the lower reaches of the Caraulun-1 River have also observed river-channel shallowing and widening, and the evidence for erosion of riverbanks is commonplace. Indeed, comparison of satellite images from 1986 to 2006 shows an increase of 31% in total river-channel area in the Caraulun-1 catchment from 8.2 to 10.7 km2, at an average rate of 0.13 km2 year−1. Some of this change has removed parts of floodplains that are used for cultivation and grazing. The shallowing and widening of these rivers is a common response to elevated rates of sediment input (see Wasson et al. 2022 for examples).
An attempt to estimate the rate of sediment accumulation on a flood plain of the Laclo River near Manatuto by 210Pb(ex) dating produced a result relevant to the question of riverbank erosion. There was no 210Pb(ex) in this deposit (sampled from the surface to a depth of 80 cm), showing that the deposit is ≥120 years old (5× the half-life of 210Pb) and the surface (which would have received direct atmospheric fallout even after decay of 210Pb in the older and deeper deposit) has probably been eroded. This conclusion is consistent with migration of the riverbank by lateral erosion of the floodplain into material ≥120 years old and scouring of the floodplain surface by more frequent and deeper overbank flows as the channel bed shallowed, all of which have been observed by the local people. An alternative explanation of the lack of 210Pb(ex) at this site is the addition of 226Ra in groundwater. Analysis of 226Ra, 228Ra, 230Th, and 232Th of two samples showed an excess of 226Ra and 228Ra over their Th parents, but the 228Ra excess was only 14% and there was no evidence of negative 210Pb(ex) (J. Pfitzner, pers. comm., 2018). Therefore, groundwater does not appear to have played a role.
Coastal impacts of increased sediment loads
River sediments add little to the northern coast because most of the sediment is transported directly to the ocean down a very steep gradient into the Wetar Strait. Small patches of mud occur on the coast near the mouth of the Laclo River, and to its west where mangroves are found (e.g. at Metinaro). There are a few symmetrical deltas on the northern coast, at the mouths of the Loes and Comoro rivers in particular. Sandy beaches are common, but their sediments probably come from the many small streams along the coastal ranges, not just from the large rivers, and are redistributed by longshore drift.
On the southern coast, deltas are common, many of which are symmetrical about river mouths whereas others are strongly asymmetrical showing evidence of preferential sediment transport to the east; particularly at Beco and coastward of Viqueque. Offshore of the delta front of the Caraulun-1 River, the seafloor is sandy to ~10 m, downslope of which it is muddy (Wayne 2004; Alongi et al. 2013b). Offshore ~5 km from Suai, the Mola-1 oil well intersected a 3-km-thick sequence of Pliocene–Quaternary sediments that are clay-dominated (Keep et al. 2005), showing that most sediment transported offshore is fine-grained. The well penetrated one of the small offshore sedimentary basins described by Crostella and Powell (1975).
There are no known comparable data from wells off the northern coast, but it is a reasonable assumption that the dominantly fine-grain sediment load of the Laclo River (Table 1) and other rivers in the area contribute to muddy deposits offshore. Observations by the authors after floods in this area showed that the suspended sediment plume extends several hundred metres offshore where it has a sharp edge, suggesting a plume plunge point (Lamb et al. 2010).
Mulder and Syvitski (1995) found that small mountain catchments with mean annual discharges of <460 m3 s−1 in the tropics are likely to produce hyperpycnal density currents offshore. Calculations using their eqn 6 indicated that all of the rivers of Timor-Leste, for which there are discharge estimates, are likely to produce hyperpycnal flows, with suspended sediment concentrations of >590 kg m−3, which is well above the critical concentration of 36 kg m−3. These density flows are likely during floods which, in the case of the Timor-Leste rivers, could be up to 1600 times the mean annual discharge based on eqn 1 of Mulder and Syvitski (1995). If convectional instability is present, much lower suspended sediment concentrations in river flows can generate hyperpycnal flows (Parsons et al. 2001).
The likely occurrence of hyperpycnal flows offshore from river mouths in Timor-Leste has several implications. The sediment plumes produced by hyperpycnal flows have a sharply defined outer edge. Beyond this is clear water with high light penetration, unlike in hypopycnal flows that spread turbid water much further from the coast. The N, P and dissolved organic matter in the clear water, only a few hundred metres offshore, enhances primary production of phytoplankton and secondary production of zooplankton and fish (Alongi et al. 2013b). Interviews with local people indicated greater fish catches in proximity to seasonal plumes. These fisheries are of major importance because of the small contribution to fisheries from mangroves along most of the northern and southern coasts. That is, the area of mangrove lagoon waters where fish communities ordinarily reside is small (Alongi 2013).
The fluvial transport of particulate organic carbon (POC) is strongly correlated with the transport of mineral sediment (Hilton et al. 2008). High-standing islands such as Timor in South-east Asia and Oceania produce ~33% of the mineral sediment transported to the world oceans (Milliman and Syvitski 1992), and a similar proportion of organic carbon comes from the area (Hilton et al. 2008). There are no measured loads of POC in the rivers of Timor-Leste, but applying the relationship between POC yield and catchment area for the region (Lyons et al. 2002, Fig. 1) to the Laclo, Caraulun-1 and 2, Be Lulic-1, Tafara and Rai Ketan rivers, the annual POC loads ranged from 6500 to 72,000 Mg year−1 (1.6–2.3% of suspended sediment). A surprising uniformity in estimates of the non-fossil component of POC in this area (Hilton et al. 2008) suggests that ~60% of POC from Timor-Leste rivers is non-fossil. Of this, a conservative estimate suggests that 10% would be deposited offshore in sediment protected from oxidation.
The offshore impacts of riverflows are not restricted to input of freshwater, sediments and POC. Dissolved and particulate nutrients must also affect offshore biogeochemical cycles on the adjacent shelf margin as identified on the southern coast (Alongi et al. 2013b). Measurements of nutrients in river water are restricted to low flows in the Laclo River (Alongi et al. 2006), with an N:P ratio between 17 and 22, within the range of both freshwater and seawater unpolluted by human or animal wastes or fertiliser (Alongi et al. 2014). This conclusion is also likely to apply to high flows.
The symmetrical deltas of the Caralaun-1 and Tafara rivers (Fig. 2, 4) consist at the surface of fine sandy beach ridges separated by muddy swales. If the increased sediment supply documented in Table 1 has increased the rate of progradation of the deltas, this should be detectable by age-dating the beach ridges. Beach ridges can be seen on aerial photos of the Caraulun-1 delta (Fig. 2), most of which could not be safely found in the crocodile-inhabited thick swamp forest. The only set of beach ridges that could be found on the ground occur at the eastern edge of the delta, near Betano. Here, the ridges consist of fine sandy loam, suggesting sandy sediment input both from offshore onto beaches and mud from floodwaters from inland.
OSL ages for the ridges are shown in Fig. 3. Pit 1 is in the modern beach and Pits 2 and 3 are in the first beach ridge inland with an age of 0 ± 50 years. Further inland, the only other detectable beach ridge is 2200 ± 300 years old. A sample from Pit 4, in alluvium that has probably buried other beach ridges, has an age of 900 ± 100 years at a depth of 40 cm. The rate of progradation from 2200 ± 300 to 0 ± 50 years of age is 0.02 m year−1. Between the ridge dated to 0 ± 50 years of age and the modern beach, the rate of progradation is 1.6 m year−1, a rate ~80 times faster than the other rate. Both calculations assume that the second ridge from the coast is 50 years old, given that mangroves at least 20–25 years old (Alongi et al. 2012; Alongi 2013) grow between this ridge and the modern beach. Beach ridges are easily found on the ground on the Tafara River delta (Fig. 4 and 5). However, the OSL ages (Fig. 5) have such large uncertainties that progradation-rate changes cannot be detected. The data are provided for the benefit of others who may wish to use this approach and further dating is warranted.
Comparison of satellite images shows a complicated pattern for the entire delta coast between 1986 and 2006. Some areas have eroded while others prograded. Some areas on the western side of the river have eroded by up to 65 m, and areas close to the river have prograded by up to 45 m. In the vicinity of the beach ridges dated by OSL, the beach has almost doubled in width at a rate of 2.5 m year−1, comparable with the rate from 50 years ago. Changes of the area of sedimentation at the river mouth suggest greater input of sediment between 1996 and 2006 than during 1986–1996 when erosion occurred.
At Betano, mangroves have been partially buried by beach deposits (Alongi et al. 2009; Alongi 2013), whereas further west, along the delta front, swamp forest and palm forest are in places being inundated by sand washed over the crest of the modern beach. Whereas the satellite imagery shows a complex pattern of change, the washover sand provides some evidence of increased sedimentation along the delta front within the past few decades. This additional sand is presumably a result of increased riverine inputs to the coast, given that, as argued earlier, the symmetrical shape of the delta is evidence of river sediment dominance.
The zonal patterns of mangroves along the southern coast are abrupt, in agreement with the supposition that increases in sedimentation are recent. For instance, the mangroves are either remnants of once more extensive forests or have remained stunted and marginalised as mid- or high-intertidal edge stands within a sandy lagoon (Alongi 2013). At Betano, mangrove peat was found at 1 m depth in cores taken seaward of the existing mangrove stands, suggesting smothering by modern riverine deposits. Further, the surface water of the mangroves is highly saline, but significant groundwater is tapped by mangroves with very deep roots, again a probable adaptation to either very rapid sand deposition in beach barriers or shifting, unstable sediments (Alongi 2013). These conditions have also led to physical conditions in which the ratio of forest area to total lagoonal area is small, with little ponding of seawater behind the beach barriers.
Extreme rainfall and riverflows are of great concern to the people of Timor-Leste (Alongi et al. 2009), triggering landslides and floods, washing away agricultural land, depositing sand and gravel on agricultural land, destroying houses and bridges, and causing death of both people and livestock. These extremes are likely to be the most important agents in erosion and sediment transport, although this has not been quantified. Future changes to hydrologic extremes (Bates et al. 2008) may therefore be of considerable concern (e.g. Ahmed et al. 2009), worsening soil erosion, sediment transport and flooding, and increasing hyperpycnal flows. Hyperpycnal flows may have positive effects by storing more POC offshore and, in combination with increased dissolved nutrient fluxes, increasing primary productivity offshore.
Conclusions
The question whether land-cover change has affected rates of erosion and sediment transport, with impacts on rivers and the coastal zone, can now be partially answered by taking as far as possible a catchment-to-coast approach.
The increase of erosion rates in the seven studied catchments by factors up to 120 is attributed mainly to land-cover change in the past century. This increase has led to aggradation of river channels that has removed agricultural and grazing land, and exacerbated flooding. Enough data exist only in the Caralulun-1 catchment to determine the main sediment source for the increased river sedimentation. Rainfall-induced landslides in steep areas cleared of native forest or areas of forest thinning produce the largest amount of sediment. Increased erosion and river sedimentation is therefore attributed to human factors. Channel aggradation has been accompanied by channel widening and riverbank erosion, a set of responses known to occur in braided rivers that have received a large input of sediment.
The increase of channel sedimentation is also reflected on the southern coast by increased delta progradation, although further research on this topic is required. This progradation has buried and partially extinguished mangroves and palm forests. The increased river-sediment loads may have increased the areas of turbid plumes that deposit sediment and POC offshore. The plumes appear to have increased primary productivity in the clear water beyond the plumes.
Future increases of extreme rainfall and discharge as climate changes may increase landsliding, channel change, and sediment input to the coast if revegetation does not occur. Limited revegetation efforts are underway, but they need to be accelerated.
The Coral Triangle Initiative (CTI) was designed to support people-centred biodiversity conservation, sustainable development, poverty reduction and equitable benefit sharing from natural resources. One of the principles of the CTI is that it should ‘be based on solid science’. Given that the phenomena and issues considered in this paper have received limited attention in the Coral Triangle, there is an urgent need to evaluate the role of erosion and sediment transport in ecosystem-based management for biodiversity conservation and sustainable riverine and coastal livelihoods over as much of the Coral Triangle as possiblely using a catchment-to-coast approach. The approach adopted here will produce results in years rather than decades if traditional monitoring methods are used.
Declaration of funding
The authors acknowledge the support and funding from Charles Darwin University, the Australian Institute of Marine Science and the Government of Timor-Leste for the original study that formed the basis of this paper.
Author contributions
R. J. Wasson provided conceptualisation, analysis, writing of original draft, and review and editing of the written text. J. Rouwenhorst added conceptualisation, analysis, and review and editing of the written text; A. Amaral provided analysis, logistics and supervision, and review and editing of the written text; K. Fifield, T. Pietsch, N. Chauhan, D. M. Alongi, F. Tirendi and A. K. Singhvi contributed analysis, and review and editing of the written text.
Acknowledgements
The project was inspired by His Excellency Estanislau da Silva, former Minister of Agriculture, Forestry and Fisheries, Government of Timor-Leste. The late Narcisso Almeida de Carvalho, formerly of the Ministry of Agriculture, Forestry and Fisheries, Government of Timor-Leste, facilitated fieldwork aspects of the study. This project was conducted under the auspices of ATSEF, the Arafura Seas Expert Forum (now known as ATSEA: https://atsea-program.com, accessed 18 December 2023). We thank the many people of Timor-Leste who provided information and hospitality, Myra Keep and David Haig for advice about the geology, John Pfitzner for advice about radionuclides, Jorge Ramos about reforestation schemes. We also thank the reviewers for their helpful comments.
References
Ahmed SA, Diffenbaugh NS, Hertel TW (2009) Climate volatility deepens poverty vulnerability in developing countries. Environmental Research Letters 4(3), 034004.
| Crossref | Google Scholar |
Al-Asif A, Kamal AHM, Hamli H, et al. (2022) Status, biodiversity, and ecosystem services of seagrass habitats within the Coral Triangle in the Western Pacific Ocean. Ocean Science Journal 57(2), 147-173.
| Crossref | Google Scholar |
Alongi DM, Carvalho NA, Silva MF, Geterres H, Inicio F, Amaral AT, Silva OA, McWilliam AR, Tirendi F, Trott LA, Wasson RJ (2006) River catchments and marine productivity in Timor Leste. Final report to the Ministry of Agriculture, Forestry and Fisheries, Government of Timor-Leste, from Charles Darwin University.
Alongi DM, de Carvalho NA, Amaral AL, et al. (2012) Uncoupled surface and below-ground soil respiration in mangroves: implications for estimates of dissolved inorganic carbon export. Biogeochemistry 109, 151-162.
| Crossref | Google Scholar |
Alongi DM, da Silva M, Wasson RJ, et al. (2013a) Sediment discharge and export of fluvial carbon and nutrients into the Arafura and Timor seas: a regional synthesis. Marine Geology 343, 146-158.
| Crossref | Google Scholar |
Alongi DM, Brinkman R, Trott LA, et al. (2013b) Enhanced benthic response to upwelling of the Indonesian Throughflow onto the southern shelf of Timor-Leste, Timor Sea. Journal of Geophysical Research: Biogeosciences 118(1), 158-170.
| Crossref | Google Scholar |
Asian Development Bank (2014) State of the Coral Triange: Timor-Leste. Available at https://www.adb.org/sites/default/files/publication/42394/state-coral-triangle-timor-leste.pdf [Verified 20 May 2024]
Banerjee D, Murray AS, Bøtter-Jensen L, et al. (2001) Equivalent dose estimation using a single aliquot of polymineral fine grains. Radiation Measurements 33(1), 73-94.
| Crossref | Google Scholar |
Barnett J, Dessai S, Jones RN (2007) Vulnerability to climate variability and change in East Timor. AMBIO: a Journal of the Human Environment 36(5), 372-378.
| Crossref | Google Scholar | PubMed |
Benevides FT (2003) Cropping systems in East Timor. In ‘Agriculture: new directions for a new nation East Timor (Timor-Leste). Proceedings of a Workshop’, 1–3 October 2002, Dili, Timor-Leste. (Eds H da Costa, C Piggin, CJ do Cruz, JJ Fox) ACIAR Proceedings 113, pp. 17–19. (Australian Centre for International Agricultural Research: Canberra, ACT, Australia)
Bhattacharya JP, Giosan L (2003) Wave-influenced deltas: geomorphological implications for facies reconstruction. Sedimentology 50(1), 187-210.
| Crossref | Google Scholar |
Binnie SA, Phillips WM, Summerfield MA, et al. (2007) Tectonic uplift, threshold hillslopes, and denudation rates in a developing mountain range. Geology 35(8), 743-746.
| Crossref | Google Scholar |
Bird P (2003) An updated digital model of plate boundaries. Geochemistry, Geophysics, Geosystems 4(3), 1027.
| Crossref | Google Scholar |
Blong RJ (1986) Natural Hazards in the Papua New Guinea Highlands. Mountain Research and Development 6(3), 233-246.
| Crossref | Google Scholar |
Bouma GA, Kobryn HT (2004) Change in vegetation cover in East Timor, 1989–1999. Natural Resources Forum 28(1), 1-12.
| Crossref | Google Scholar |
Bourke P, Brockwellm S, Faulkner P, et al. (2007) Climate variability in the mid to late Holocene Arnhem Land region, North Australia: archaeological archives of environmental and cultural change. Archaeology in Oceania 42(3), 91-101.
| Crossref | Google Scholar |
Burke L, Reytar K, Spalding M (2012) Reefs at risk revisited in the Coral Triangle. Report. (World Resources Institute: Washington, DC, USA) Available at https://www.wri.org/research/reefs-risk-revisited-coral-triangle
Carlson RR, Foo SA, Asner GP (2019) Land use impacts on coral reef health: a ridge-to-reef perspective. Frontiers in Marine Science 6, 562.
| Crossref | Google Scholar |
Chappell J, Veeh HH (1978) Late Quaternary tectonic movements and sea-level changes at Timor and Atauro Island. GSA Bulletin 89(3), 356-368.
| Crossref | Google Scholar |
Coral Triangle Initiative (2009) Regional plan of action: Coral Triangle Initiative on Coral Reefs, Fisheries and Food Security (CTI-CFF). (CTI-CFF Security Secretariat: Manado, Indonesia) Available at https://nicholasinstitute.duke.edu/sites/default/files/coral-reef-policies/CTI_RPA.pdf [Verified 29 May 2024]
Coral Triangle Initiative on Coral Reefs, Fisheries and Food Security Secretariat (2022) CTI-CFF Annual Report 2021. (CTI-CFF Security Secretariat: Manado, Indonesia) Available at https://coraltriangleinitiative.org/node/10009 [Verified 20 May 2024]
Coudurier-Curveur A, Singh SC, Deighton I (2021) Timor collision front segmentation reveals potential for great earthquakes in the western Outer Banda Arc, eastern Indonesia. Frontiers in Earth Science 9, 640928.
| Crossref | Google Scholar |
Crostella AA, Powell DE (1975) Geology and hydrocarbon prospects of the Timor area. In ‘Proceedings of the 4th Annual Convention of the Indonesian Petroleum Association’. (IPA: Jakarta, Indonesia) Available at https://www.ipa.or.id/en/publications/geology-and-hydrocarbon-prospects-of-the-timor-area [Abstract]
Das S (2004) Seismicity gaps and the shape of the seismic zone in the Banda Sea region from relocated hypocenters. Journal of Geophysical Research: Solid Earth 109, B12303.
| Crossref | Google Scholar |
Delevaux JMS, Stamoulis KA, Whittier R, et al. (2019) Place-based management can reduce human impacts on coral reefs in a changing climate. Ecological Applications 29(4), e01891.
| Crossref | Google Scholar | PubMed |
Deng K, Wittmann H, Yang S, et al. (2021) The upper limit of denudation rate measurement from cosmogenic 10Be(meteoric)/9Be ratios in Taiwan. Journal of Geophysical Research: Earth Surface 126(10), e2021JF006221.
| Crossref | Google Scholar |
Duffy B, De Jesus OVT, Quigley M, et al. (2022) Flooding and landsliding in Timor-Leste: linked hazards in a young mountain belt. Available at https://www.geo-down-under.org.au/timor-leste-flood-disaster/ [Verified 20 May 2024]
Ely KS, Sandiford M (2010) Seismic response to slab rupture and variation in lithospheric structure beneath the Savu Sea, Indonesia. Tectonophysics 483(1-2), 112-124.
| Crossref | Google Scholar |
Erikstad L, Bakkestuen V, Sandlund OT (2001) Deforestation in East Timor since 1972 as indicated by LANDSAT imagery. In ‘Assessing environmental needs and priorities in East Timor: issues and priorities’. (Eds OT Sandlund, I Bryceson, D de Carvalho, N Rio, J da Silva, MI Silva) Annex 6. (NINA-NIKU, Foundation for Nature Research and Cultural Heritage Research: Trondheim, Norway)
Fifield LK, Tims SG, Fujioka T, et al. (2010) Accelerator mass spectrometry with the 14UD accelerator at the Australian National University. Nuclear Instruments and Methods in Physics Research Section B: Beam Interactions with Materials and Atoms 268(7–8), 858-862.
| Crossref | Google Scholar |
Fisher R, Bessell-Browne P, Jones R (2019) Synergistic and antagonistic impacts of suspended sediments and thermal stress on corals. Nature Communications 10, 2346.
| Crossref | Google Scholar | PubMed |
Gabet EJ, Mudd SM (2009) A theoretical model coupling chemical weathering rates with denudation rates. Geology 37(2), 151-154.
| Crossref | Google Scholar |
Gardner TW, Jorgensen DW, Shuman C, et al. (1987) Geomorphic and tectonic process rates: effects of measured time interval. Geology 15(3), 259-261.
| Crossref | Google Scholar |
Haberle SG, David B (2004) Climates of change: human dimensions of Holocene environmental change in low latitudes of the PEPII transect. Quaternary International 118-119, 165-179.
| Crossref | Google Scholar |
Harris R, Major J (2016) Waves of destruction in the East Indies: the Wichmann catalogue of earthquakes and tsunami in the Indonesian region from 1538 to 1877. In ‘Geohazards in Indonesia: Earth Science for Disaster Risk Reduction, Vol. 44’. (Eds PR Cummins, I Meilano) Special publication, pp. 9–46. (Geological Society: London, UK) doi:10.1144/SP441.2
Hilton RG, Galy A, Hovius N, et al. (2008) Tropical-cyclone-driven erosion of the terrestrial biosphere from mountains. Nature Geoscience 1(11), 759-762.
| Crossref | Google Scholar |
Hughes TP, Bellwood DR, Connolly SR (2002) Biodiversity hotspots, centres of endemicity, and the conservation of coral reefs. Ecology Letters 5(6), 775-784.
| Crossref | Google Scholar |
Jain M, Singhvi AK (2001) Limits to depletion of blue-green light stimulated luminescence in feldspars: implications for quartz dating. Radiation Measurements 33(6), 883-892.
| Crossref | Google Scholar |
Juyal N, Chamyal LS, Bhandari S, et al. (2006) Continental record of the southwest monsoon during the last 130 ka: evidence from the southern margin of the Thar Desert, India. Quaternary Science Reviews 25(19–20), 2632-2650.
| Crossref | Google Scholar |
Kaneko Y, Maruyama S, Kadarusman A, et al. (2007) On-going orogeny in the outer-arc of the Timor–Tanimbar region, eastern Indonesia. Gondwana Research 11(1–2), 218-233.
| Crossref | Google Scholar |
Keep M, Haig DW (2010) Deformation and exhumation in Timor: distinct stages of a young orogeny. Tectonophysics 483(1–2), 93-111.
| Crossref | Google Scholar |
Keep M, Beck L, Bekkers P (2005) Complex modified thrust systems along the southern margin of East Timor. The APPEA Journal 45, 297-310.
| Crossref | Google Scholar |
Knighton AD (1989) River Adjustment to changes in sediment load: the effects of tin mining on the Ringarooma River, Tasmania, 1875–1984. Earth Surface Processes and Landforms 14(4), 333-359.
| Crossref | Google Scholar |
Kohl CP, Nishiizumi K (1992) Chemical isolation of quartz for measurement of in-situ-produced cosmogenic nuclides. Geochimica et Cosmochimica Acta 56(9), 3583-3587.
| Crossref | Google Scholar |
Lal D (1991) Cosmic ray labeling of erosion surfaces: in situ nuclide production rates and erosion models. Earth and Planetary Science Letters 104(2–4), 424-439.
| Crossref | Google Scholar |
Lamb MP, McElroy B, Kopriva B, et al. (2010) Linking river-flood dynamics to hyperpycnal-plume deposits: experiments, theory, and geological implications. GSA Bulletin 122(9–10), 1389-1400.
| Crossref | Google Scholar |
Lyons WB, Nezat CA, Carey AE, et al. (2002) Organic carbon fluxes to the ocean from high-standing islands. Geology 30(5), 443-446.
| Crossref | Google Scholar |
McWilliam A (2001) Prospects for the sacred grove. The Asia Pacific Journal of Anthropology 2(2), 89-113.
| Crossref | Google Scholar |
McWilliam A (2005) Haumeni, not many: renewed plunder and mismanagement in the Timorese sandalwood industry. Modern Asian Studies 39(2), 285-320.
| Crossref | Google Scholar |
Madej MA, Sutherland DG, Lisle TE, et al. (2009) Channel responses to varying sediment input: a flume experiment modeled after Redwood Creek, California. Geomorphology 103(4), 507-519.
| Crossref | Google Scholar |
Major J, Harris R, Chiang H-W, et al. (2013) Quaternary hinterland evolution of the active Banda Arc: surface uplift and neotectonic deformation recorded by coral terraces at Kisar, Indonesia. Journal of Asian Earth Sciences 73, 149-161.
| Crossref | Google Scholar |
Malamud BD, Turcotte DL, Guzzetti F, et al. (2004) Landslides, earthquakes, and erosion. Earth and Planetary Science Letters 229(1–2), 45-59.
| Crossref | Google Scholar |
Milliman JD, Syvitski JPM (1992) Geomorphic/Tectonic control of sediment discharge to the ocean: the importance of small mountainous rivers. The Journal of Geology 100(5), 525-544.
| Crossref | Google Scholar |
Milliman JD, Farnsworth KL, Albertin CS (1999) Flux and fate of fluvial sediments leaving large islands in the East Indies. Journal of Sea Research 41(1–2), 97-107.
| Crossref | Google Scholar |
Montgomery DR, Brandon MT (2002) Topographic controls on erosion rates in tectonically active mountain ranges. Earth and Planetary Science Letters 201(3–4), 481-489.
| Crossref | Google Scholar |
Mulder T, Syvitski JPM (1995) Turbidity currents generated at river mouths during exceptional discharges to the world oceans. The Journal of Geology 103(3), 285-299.
| Crossref | Google Scholar |
Murray AS, Roberts RG (1998) Measurement of the equivalent dose in quartz using a regenerative-dose single-aliquot protocol. Radiation Measurements 29(5), 503-515.
| Crossref | Google Scholar |
Naciri W, Boom A, Payne M, et al. (2023) Massive corals record deforestation in Malaysian Borneo through sediments in river discharge. Biogeosciences 20(8), 1587-1604.
| Crossref | Google Scholar |
National Directorate of Forestry and Water Resources and Ministry of Agricuture, Forestry and Fisheries (2004) Policy and strategy, forestry and watershed subsector. (NDFWR, MAFF) Available at https://faolex.fao.org/docs/pdf/tim203892.pdf [Verified 26 June 2004]
National Research Council (2010) Landscapes on the edge: new horizons for research on earth’s surface. (The National Academies Press: Washington, DC, USA). Available at https://doi.org/10.17226/12700
Neverman AJ, Donovan M, Smith HG, et al. (2023) Climate change impacts on erosion and suspended sediment loads in New Zealand. Geomorphology 427, 108607.
| Crossref | Google Scholar |
Nugroho H, Harris R, Lestariya AW, et al. (2009) Plate boundary reorganization in the active Banda Arc–continent collision: insights from new GPS measurements. Tectonophysics 479(1–2), 52-65.
| Crossref | Google Scholar |
O’Connor S (2006) Chapter 8. Unpacking the island Southeast Asian Neolithic cultural package, and finding local complexity. In ‘Uncovering Southeast Asia’s Past: selected papers from the 10th International Conference of the European Association of Southeast Asian Archaeologists’, 14–17 September 2004, London, UK. (Eds EA Bacus, IC Glover, VC Pigott) pp. 74–87. (The British Museum)
Oliveira NV (2008) Subsistence archaeobotany: food production and the agricultural transition in East Timor. PhD thesis, Australian National University, Canberra, ACT, Australia. Available at http://hdl.handle.net/1885/99968
Orlando JL, Yee SH (2017) Linking terrigenous sediment delivery to declines in coral reef ecosystem services. Estuaries and Coasts 40, 359-375.
| Crossref | Google Scholar | PubMed |
Parsons JD, Bush JWM, Syvitski JPM (2001) Hyperpycnal plume formation from riverine outflows with small sediment concentrations. Sedimentology 48(2), 465-478.
| Crossref | Google Scholar |
Pickup G, Marshall AR (2008) Geomorphology, hydrology, and climate of the fly river system. Developments in Earth and Environmental Sciences 9, 3-49.
| Crossref | Google Scholar |
Pietsch TJ (2009) Optically stimulated luminescence dating of young (<500 years old) sediments: testing estimates of burial dose. Quaternary Geochronology 4(5), 406-422.
| Crossref | Google Scholar |
Prescott JR, Hutton JT (1994) Cosmic ray contributions to dose rates for luminescence and ESR dating: large depths and long-term time variations. Radiation Measurements 23(2–3), 497-500.
| Crossref | Google Scholar |
Ptak R (1983) Some references to Timor in old Chinese records. Ming Studies 1983(1), 37-48.
| Crossref | Google Scholar |
Rangin C, Le Pichon X, Mazzotti S, et al. (1999) Plate convergence measured by GPS across the Sundaland/Philippine Sea Plate deformed boundary: the Philippines and eastern Indonesia. Geophysical Journal International 139(2), 296-316.
| Crossref | Google Scholar |
Rouwenhorst J (2013) A spatio-temporal analysis of sediment sources in the Caraulun catchment, East Timor. PhD thesis, Charles Darwin University, Casuarina, Darwin, NT, Australia. Available at https://researchers.cdu.edu.au/files/44114224/Thesis_CDU_55194_Rouwenhorst_J.pdf
Sarker MH, Thorne CR (2006) Morphological response of the Brahmaputra–Padma–Lower Meghna river system to the Assam earthquake of 1950. In ‘Braided rivers: process, deposits, ecology and management, Vol. 36’. (Eds S Smith, H Gregory) Special Publication, pp. 289–310. (The International Association of Sedimentologists)
Schaller M, von Blanckenburg F, Hovius N, et al. (2001) Large-scale erosion rates from in situ-produced cosmogenic nuclides in European river sediments. Earth and Planetary Science Letters 188(3–4), 441-458.
| Crossref | Google Scholar |
Schide K, Gallen SF, Lupker M (2022) Modelling the systematics of cosmogenic nuclide signals in fluvial sediments following extreme events. Earth Surface Processes and Landforms 47(9), 2325-2340.
| Crossref | Google Scholar |
Soares L (2007) Actual condition and characteristics of slope failure in East Timor by multivariate statistical analysis. MCEE thesis, Saitama University, Saitama City, Japan. Available at https://www.slideshare.net/hopffer2008/actual-condition-and-characteristics-of-slope-failure-in-east-timor-by-multivariate-statistical-analysis
Standley CE, Harris R (2009) Tectonic evolution of forearc nappes of the active Banda Arc–continent collision: origin, age, metamorphic history and structure of the Lolotoi Complex, East Timor. Tectonophysics 479(1–2), 66-94.
| Crossref | Google Scholar |
Suárez-Castro AF, Beyer HL, Kuempel CD, et al. (2021) Global forest restoration opportunities to foster coral reef conservation. Global Change Biology 27(20), 5238-5252.
| Crossref | Google Scholar | PubMed |
Tate GW, McQuarrie N, van Hinsbergen DJJ, et al. (2014) Resolving spatial heterogeneities in exhumation and surface uplift in Timor-Leste: constraints on deformation processes in young orogens. Tectonics 33(6), 1089-1112.
| Crossref | Google Scholar |
Tate GW, McQuarrie N, Tiranda H, et al. (2017) Reconciling regional continuity with local variability in structure, uplift and exhumation of the Timor orogen. Gondwana Research 49, 364-386.
| Crossref | Google Scholar |
Thompson SJ (2011) Geology and soils of Timor-Leste. (Seeds of Life–Fini ba Moris) Available at http://seedsoflifetimor.org/wp-content/uploads/2012/12/Geology-and-Soils-in-Timor-LesteA4.pdf
Turowski JM, Rickenmann D, Dadson SJ (2010) The partitioning of the total sediment load of a river into suspended load and bedload: a review of empirical data. Sedimentology 57(4), 1126-1146.
| Crossref | Google Scholar |
United Nations Development Programme (2012) Comprehensive national hazard assessment and mapping in Timor-Leste. National Disaster Management Directorate, Ministry of Social Solidarity, Democratic Republic of Timor Leste; and Asian Disaster Preparedness Center, National Disaster Management Directorate, UNDP.
Waldner F, Defourny P (2017) Where can pixel counting area estimates meet user-defined accuracy requirements? International Journal of Applied Earth Observation and Geoinformation 60, 1-10.
| Crossref | Google Scholar |
Wallbrink PJ, Murray AS (1993) Use of fallout radionuclides as indicators of erosion processes. Hydrological Processes 7(3), 297-304.
| Crossref | Google Scholar |
Wallbrink PJ, Murray AS, Olley JM, Olive LJ (1998) Determining sources and transit times of suspended sediment in the Murrumbidgee River, New South Wales, Australia, using fallout 137Cs and 210Pb. Water Resources Research 34(4), 879-887.
| Crossref | Google Scholar |
Wasson RJ, Juyal N, Jaiswal M, et al. (2008) The mountain-lowland debate: deforestation and sediment transport in the upper ganga catchment. Journal of Environmental Management 88(1), 53-61.
| Crossref | Google Scholar | PubMed |
Wasson R, Acharjee S, Rakshit R (2022) Towards identification of sediment sources, and processes of sediment production, in the Yarlung–Tsangpo–Brahmaputra River catchment for reduction of fluvial sediment loads. Earth-Science Reviews 226, 103932.
| Crossref | Google Scholar |
Wilkinson SN, Olley JM, Furuichi T, et al. (2015) Sediment source tracing with stratified sampling and weightings based on spatial gradients in soil erosion. Journal of Soils and Sediments 15, 2038-2051.
| Crossref | Google Scholar |
Willett SD, Brandon MT (2002) On steady states in mountain belts. Geology 30(2), 175-178.
| Crossref | Google Scholar |
Zar JH (2005) Spearman rank correlation. In ‘Encyclopedia of biostatistics’. (Eds P Armitage, T Colton) Chapt. 7. (Wiley) doi:10.1002/0470011815.b2a15150