Managing flows for frogs: wetland inundation extent and duration promote wetland-dependent amphibian breeding success
J. F. Ocock
A
B
C
D
E
F
Abstract
River regulation is a key threat to amphibian communities that continue to face global declines. Prior work in regulated river catchments has indicated that wetland inundation from connecting river flows supports breeding in some frog species more than others.
Our aim was to identify flow metrics influencing breeding and recruitment success in flow-dependent frogs, to derive flow–ecology relationships.
We conducted repeat surveys of frog communities between 2015 and 2020 across two important wetlands in the northern Murray–Darling Basin, Australia. Using random forest analyses, we investigated the influence of 22 explanatory variables on breeding activity and success.
Inundation extent was the most important driver of calling activity by flow-dependent species (increasing breeding attempts), whereas the volume of river flows in the preceding months was most important for increasing breeding success.
Two key features of the flow pulse are linked to breeding success for amphibians in floodplain wetland systems: (1) the size of the pulse as it relates to area of wetland inundated; and (2) the duration of connecting flows as a measure of wetland duration.
By establishing amphibian flow–ecology relationships, these species can be included in governmental environmental water objectives and management.
Keywords: environmental water, flow-dependent, frog conservation, Limnodynastes, Litoria, monitoring, Murray–Darling Basin, random forests.
Introduction
Natural river flows have been disrupted around the world, causing devastating impacts for downstream ecosystems (Nilsson et al. 2005). Regulation of river flows by dams and storages, weirs and regulators have altered flow magnitude, timing, duration and frequency, which characterise the flow regime of a river (Vörösmarty et al. 2010). For flows that variably inundate floodplain wetlands, this alteration has decreased wetland flooding extent, duration and frequency, subsequently lengthening dry periods between floods to the detriment of wetland habitats and wetland-dependent fauna (Bunn and Arthington 2002; Tockner et al. 2010; Thompson et al. 2018; Quentin Grafton et al. 2022). The large floodplain wetlands in semiarid and dryland regions reliant on highly variable upstream river flows to inundate low-lying marshes, creeks and waterholes have been particularly affected.
Frogs are a conspicuous and important component of riparian habitat and wetlands providing a critical energy link in the food web by allowing resource exchange between freshwater and terrestrial ecosystems, which can be substantial (Pough 1980; Earl et al. 2022). The effects of river regulation on rivers and wetlands are recognised as a threat to amphibian communities and individual species (Eskew et al. 2012; Naniwadekar and Vasudevan 2014; Peek et al. 2021; Mathwin et al. 2023). For example, population declines in the southern bell frog (Litoria raniformis) up to the early 2000s were attributed to changes in flow regimes and subsequent impacts on wetland habitat availability in south-eastern Australia (Ocock and Wassens 2018). Increased flow fluctuations, asynchronous flows and disconnected streams due to dam operations in northern America has reduced larval movement, distribution, abundance and genetic diversity of river-breeding frogs (Rana boyii, Rana draytonii and Ascaphus truei) (Kupferberg et al. 2012; Dare et al. 2020; Peek et al. 2021). In other areas, the removal of flows sustaining riparian wetlands downstream of dams has reduced breeding opportunities and removed species (Bateman et al. 2008; Guzy et al. 2018).
In response to the globally recognised negative effects of flow regulation on rivers, riparian and floodplain wetland habitats, including dependent frog communities, jurisdictions around the world have implemented policies and plans to manage river flows specifically for environmental benefits such as in the UK (Acreman et al. 2014), in California and Texas in the USA (Yarnell et al. 2016; Wineland et al. 2022), Mexico (Salinas-Rodríguez et al. 2021) and South Africa (Brown et al. 2020). In Australia, large and important wetlands in the Murray–Darling Basin (MDB) are reliant on upstream river flows for flooding but have suffered widespread ecological degradation due to river regulation and over allocation to consumptive use (Arthington and Pusey 2003; Kingsford et al. 2015). Major water reforms by governments, culminating in the 2012 Basin Plan (Australian Government 2012), legislated the commitment to recover water from extractive uses to meet the needs of the environment (Hart 2016; Swirepik et al. 2016). However, for any targeted management actions to also benefit frog species, there needs to be a clear understanding of flow–ecology relationships for species dependent on river flows that inundate floodplain wetlands (Acreman et al. 2014; Poff et al. 2017).
Wetlands, floodplains, riverine and riparian habitats support a variety of amphibian species (Eskew et al. 2012; Ocock et al. 2016; Holgerson et al. 2019). Alteration of flows in these systems disrupt natural flow cues and inundation patterns, and so are likely to disproportionally affect species with synchrony to the pre-disturbance regime (Kupferberg et al. 2012). Managed flows targeting wetland species therefore need to consider behavioural, physical or life-history-related links to the flow regime. Developing suitable flow requirements for a guild or group of species with similar responses and traits is useful where there is an assemblage of potentially affected species with poorly known habitat requirements. In ecological studies, functional groups have been assigned to wetland vegetation (Casanova and Brock 2000; Lytle et al. 2017), freshwater fish (Mims and Olden 2013; Stocks et al. 2021), waterbirds (Kingsford et al. 2020) and more recently freshwater mussels (Gates et al. 2015) but, to date, there has been limited application of this approach for frog species.
The advantages of identifying functional groups are that species in the same group respond similarly to environmental drivers. Prior work on amphibians in regulated river catchments has indicated that not all species have a similar response to rainfall or warm temperatures alone and some have a more positive association with wetlands when inundated from river flows than other species (Ocock et al. 2014, 2016; Littlefair et al. 2021). These differences allow species to be grouped into ecological guilds according to common traits, life history strategies and their hydrological dependence (Chen and Olden 2018). However, for amphibians demonstrating dependence on wetland conditions and responses to flooding river flows, these relationships are ill-defined as studies have mainly focused on the influence of aspects of the flow regime on species occurrence, abundance or community composition (Littlefair et al. 2021; Sarker et al. 2022). The nature of the relationship between wetland-dependent amphibians and flow with regards to demographic parameters (e.g. breeding behaviour) or factors promoting population persistence (e.g. reproductive success) has not been well explored.
In our study, we aimed to determine the important flow and habitat characteristics driving reproductive activity and recruitment success in frog species positively associated with the flooding patterns of floodplain wetlands. We used a simple grouping to focus our analysis on species we were confident have a breeding response to wetland inundation from river flows rather than responding to local rainfall or temperature. To do this, we analysed frog and habitat survey data collected as part of repeat annual austral spring–summer monitoring between 2015 and 2020 at 29 sites across two large floodplain wetlands, the Gwydir Wetlands and Macquarie Marshes in the semiarid region of the northern MDB (Fig. 1). Our survey period covered a range of flow conditions in these wetland systems including the driest year on record (Bureau of Meterology 2020) and small and large managed and natural wetland inundating flow events.
Location of frog surveys sites within two wetland systems (a) Gwydir Wetlands and (b) Macquarie Marshes in relation to: the number of times inundated (0–10) in 10 years (July 2011–June 2021) as a surrogate of habitat availability over the study period and prior 5 years; the NSW reserve estate; and Ramsar sites within (c) respective Gwydir River and Macquarie River catchments in the northern portion of the Murray–Darling Basin (MDB), Australia. The inundation mapping for the number of time inundated in 10 years was derived from satellite imagery analysis.
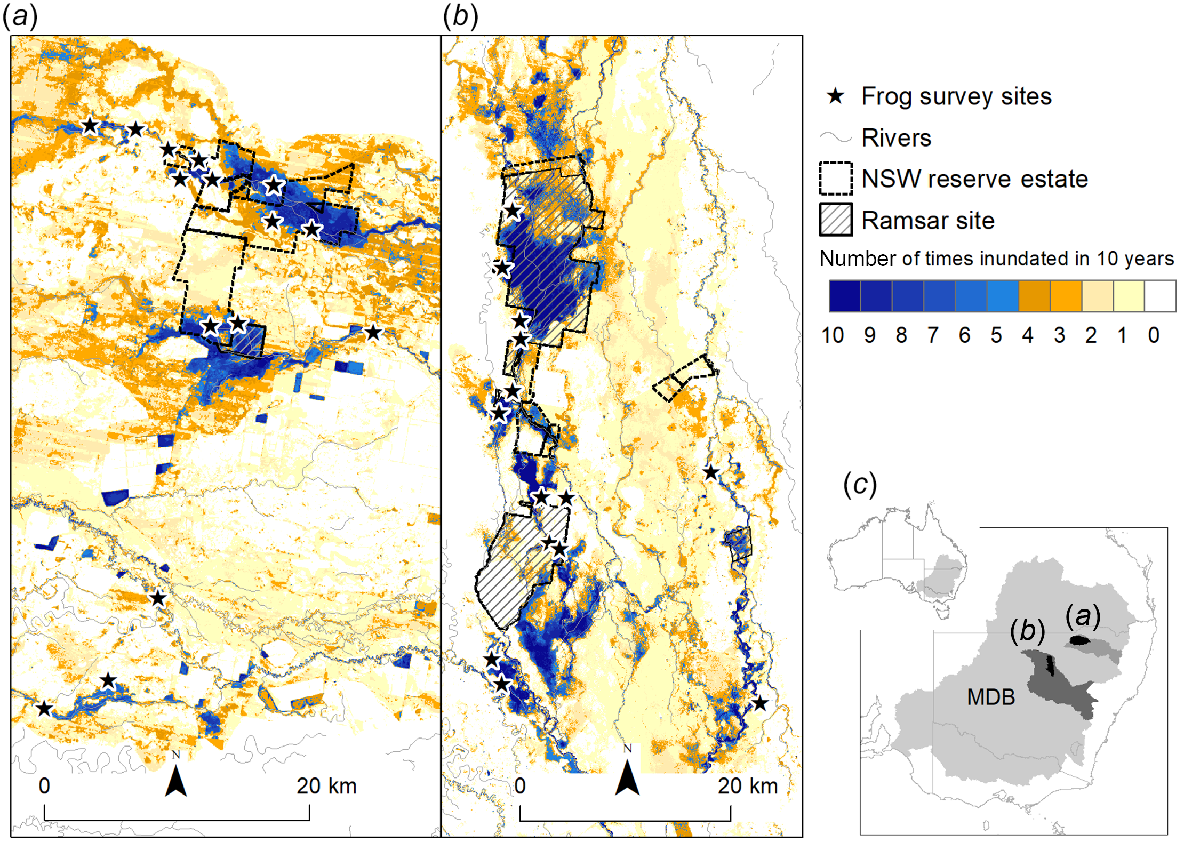
We focused on two stages of life history needed for successful recruitment in the species identified by our grouping: the suitable conditions for breeding behaviour (male calling and egg-laying by females) and suitable conditions for tadpoles to complete metamorphosis and move to their terrestrial life stage. We expected breeding activity, evidenced by calling males, to be positively influenced by extent and timing of wetland inundation. Breeding success, evidenced by higher numbers of recently metamorphosed frogs (metamorphs), was expected to be positively influenced by longer wetland inundation duration. We used our results to develop principles to guide water delivery aimed at supporting outcomes for frog species reliant on wetland inundation to maintain populations and to highlight key considerations for evaluating those management actions.
Methods
Study area
The Gwydir Wetlands and Macquarie Marshes are located in the northern portion of the MDB in south-eastern Australia (Fig. 1), a semiarid region characterised by low average rainfall and high evaporation rates (Quentin Grafton et al. 2022). The Gwydir Wetlands are formed on the floodplains of the lower Gwydir River catchment and the Macquarie Marshes comprise a large floodplain wetland system formed by the breakdown of the Macquarie River in the lower catchment (Fig. 1). Both wetland systems are examples of mosaic landscapes consisting of diverse vegetation types that provide habitat for dependent fauna at critical life stages, sometimes in high abundance, when they are variably inundated from river flows (Fig. 1). Recognised for their high biodiversity values, both wetlands include protected areas under the NSW reserve estate, with parts recognised as internationally important under the Ramsar Convention. Owing to water resource development, both wetlands have been substantially affected by alteration to the natural flow regime and changes in land use that has caused declines in the health and extent of wetland habitat (Kingsford 2000). To mitigate these impacts, both wetlands have a long history of environmental water management targeting the inundation of wetland habitats (Foerster 2008; Berney and Hosking 2016).
Study species
Fifteen frog taxa have been recorded across different wetland habitats in the study area. All have wide distributions and are not considered threatened. The species display a range of morphological and behavioural traits, with prior work identifying three broad groupings based on those traits that may inform their flow–ecology relationship (see Ocock et al. 2014, 2016; Sarker et al. 2022). Non-burrowing ground frogs, such as the barking marsh frog (Limnodynastes fletcheri), have limited ability to withstand drying and are reliant on floodplain habitats, including wetlands, waterholes and creeks, for refuge. They also exhibit breeding behaviour in response to inundation of wetlands by river flows in the absence of rainfall. Arboreal species and burrowing species have various adaptations that reduce cutaneous water loss, including forming a cocoon after burrowing underground (e.g. water-holding frog, Cyclorana platycephala) and adopting water-conserving postures (e.g. green tree frog, Litoria caerulea). General activity and breeding behaviour in these species is primarily in response to local rainfall and temperatures, and unrelated to flow characteristics, such as timing, extent and duration of wetland inundation, though inundated floodplain wetlands may provide refuge and breeding habitat.
Frog and tadpole surveys
Frog surveys were completed at 15 wetland sites across the Gwydir Wetlands system and at 14 sites in the Macquarie Marshes (Fig. 1) between austral spring 2015 to summer 2020. The survey sites covered a range of habitat types including vegetated mixed marsh lagoons, open waterholes and riverine habitats. All sites were surveyed each September (early spring) and November (late spring) from 2015 to 2020 with some exceptions due to site access constraints. In most years, the early spring surveys coincided with the initial arrival of flows filling the wetlands (Fig. 2 and 3). The late spring survey provided opportunity for detection of breeding success such as tadpoles or recently metamorphosed frogs and coincided with the main activity window for most frog species considered flow-dependent. Additional late summer surveys were conducted in the Gwydir Wetlands (only) to capture outcomes of managed events that inundated wetlands from late spring into late summer in February 2017 (all sites) and March 2019 (six sites only), and to capture responses to the first significant flows after a period of extended drought in March 2020 (seven sites only) (Fig. 2).
Daily rainfall (a) at Moree Airport and daily flow rates (b) from an upstream gauging station (gauge number 418004) indicative of wetland inundation extent in the Gwydir Wetlands over the study period showing timing of surveys (closed circles).
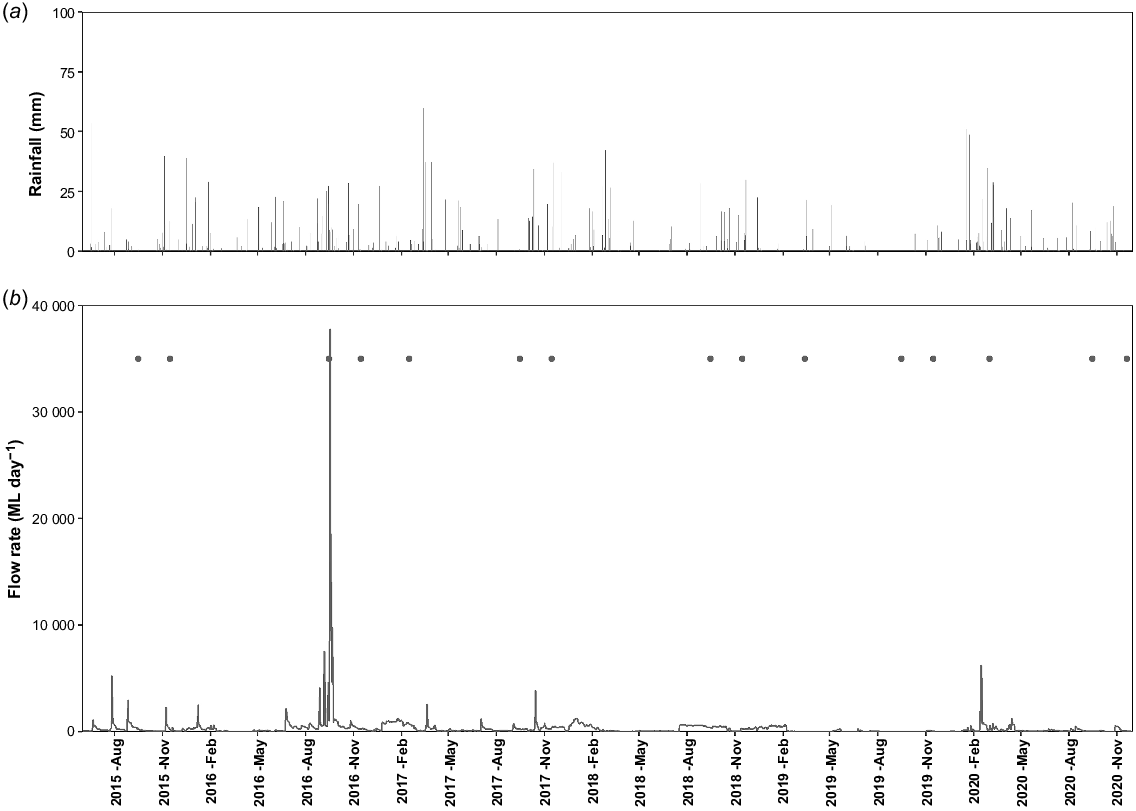
Daily rainfall (a) at Quambone Station and daily flow rates (b) from upstream gauging stations (gauge numbers 421088 and 421090 combined) indicative of wetland inundation extent in the Macquarie Marshes over the study period, showing timing of surveys (closed circles).
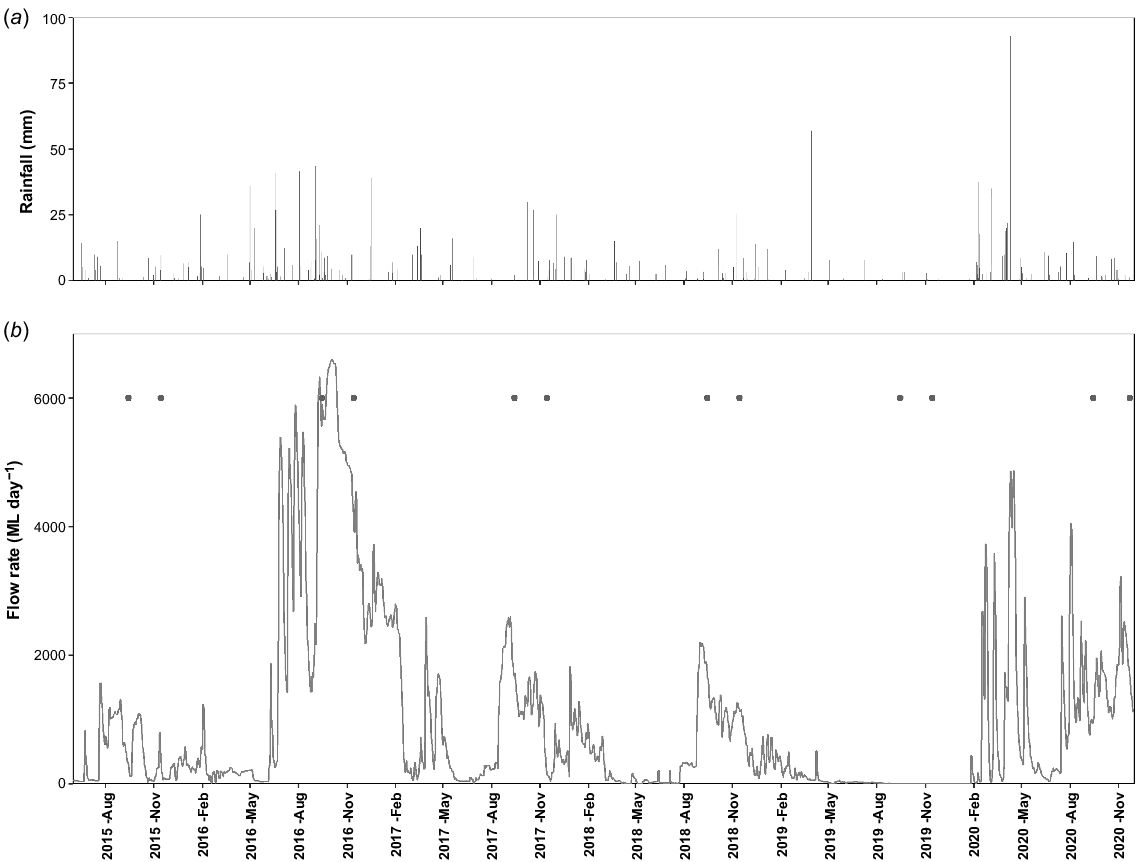
All field surveys were approved under NSW National Parks and Wildlife Service scientific licence SL101491 and NSW Department of Planning and Environment, Environment & Heritage Group animal research authority 141209-03.
We completed a daytime habitat assessment and tadpole survey, followed by a night-time frog survey at each site during each survey period with all surveys completed within 4 days and nights. Nocturnal transect surveys for frogs began at least 30 min after sunset. At each site, we used two approaches. We first completed a 5-min audio survey to record each frog species heard calling. Each species calling was assigned a category based on an index of calling abundance as we could not reliably discern the precise number of individuals calling particularly as the chorus size increased, with ‘rare’, 1–5 individuals calling; ‘common’, 6–10; ‘abundant’, 11–20; and ‘very abundant’, greater than 20 individuals calling (Royle 2004). We then did a visual and auditory encounter survey (VAES) equating to a total of 1-person h where two experienced field staff searched across the wetland area for a duration of 30 min. Spotlights (with a maximum of 1000 lumens) were used to search for frogs in inundated areas, along the wetland edge and into the surrounding terrestrial habitat. For each individual observed, we recorded species, method of detection (seen only, heard calling in close proximity to the surveyor, or seen and heard), and life stage (adult or recently metamorphosed). When needed, individuals were caught by hand with dampened plastic gloves and measured with a soft ruler or callipers. Hygiene protocols were followed, and all gear disinfected between survey areas.
We used two methods to distinguish metamorphs from adult frogs. First, the individual was classified as a metamorph if it had a visible residual tail stub. Second, for flow-dependent frog species only, if the individual did not have a tail stub but was equal to or smaller than the size recorded for metamorphs, namely less than ~26 mm for barking marsh frogs and salmon-striped frogs (Limnodynastes salmini), ~23 mm or less for striped marsh frogs (Limnodynastes tasmaniensis) and 12 mm or less for eastern sign-bearing froglets (Crinia parinsignifera) (following Anstis 2013 and J. F. Ocock, unpubl. data). Species taxonomy follow Anstis (2013).
Habitat assessment
A rapid assessment of habitat structure, inundation extent and water depth at each site, was completed during each daytime site survey. Three vegetation quadrats were located at each site. For each quadrat, the percentage open water, percentage terrestrial vegetation, percentage bare ground and percentage cover of each of the aquatic vegetation categories (submerged, floating, short and tall emergent) was estimated. Estimates of the vegetation categories could total more than 100% as layers of different aquatic vegetation types can overlap each other. Water depth was estimated (cm) in the centre of each quadrat. Data were then averaged for the eight habitat covariates (Table 1).
Variable (code name) | Description of measurement or estimation | |
---|---|---|
Satellite-derived inundation map data | ||
Proportion of site inundated during survey (Inun.area) | Proportion of site inundated, calculated by comparing inundation maps using closest satellite image available to each survey (Thomas et al. 2015) compared with polygons of each survey area and cross-checked with data collected during on-ground habitat assessments. | |
Consecutive days inundated (Days.inun.consec) | Total number of days each site was consecutively inundated prior to each survey (inundation duration). Calculated by subtracting the frog survey date from the date when the site first appeared dry prior to the survey (as per Thomas 2019). | |
WaterNSW real-time river flow gauge data (available at https://realtimedata.waternsw.com.au/) | ||
30-day flows preceding survey (ML) (flow_30day) | Standardised cumulative river discharge 30 days prior to survey date from nearest gauge. Gwydir Wetlands: Gingham watercourse (Gingham Channel at Tillaloo, gauge number 418076 and Gingham Channel at Gingham Bridge, number 418079), Lower Gwydir watercourse (Gwydir at Allambie Bridge, number 418078), Mehi River (Mehi downstream of Gundare Regulator, number 418085) and Mallowa watercourse (Mallawa Creek at Regulator, number 418049). Macquarie Marshes: North and South Marsh area, (Marebone Weir numbers 421088 and downstream of Marebone Brook, 421090) combined; East Marsh, (Gum Cowal at Bifurcation, number 421146). | |
60-day flows preceding survey (ML) (flow_60day) | Standardised cumulative river discharge 60 days prior to survey date from nearest gauge (see above). | |
90-day flows preceding survey (ML) (flow_90day) | Standardised cumulative river discharge 90 days prior to the survey date from nearest gauge (see above). Variable used in metamorph model only. | |
120-day flows preceding survey (ML) (flow_120day) | Standardised cumulative river discharge 120 days prior to survey date from nearest gauge (see above for relevant gauges). Variable used in metamorph model only. | |
Bureau of Meteorology rainfall gauge data (available at http://www.bom.gov.au/climate/data/) | ||
1-day rainfall (Rainfall.1day) | Rainfall recorded on day of survey from nearest gauge. Gwydir Wetlands – Moree Airport, number 53115; Macquarie Marshes – Quambone Station, number 051042. | |
7-day rainfall (Rainfall.7day) | Rainfall recorded in the 7 days prior to survey. | |
15-day rainfall (Rainfall.15day) | Rainfall recorded in the 15 days prior to survey. | |
Field survey data: | ||
Survey month (MonthUpdated) | Month of survey occurred (September, November, March). Variable used in calling model only. | |
Inundation timing (FloodTiming) | Broad categories to describe timing of flows to each site: Start (during initial September survey), Middle (between September and November trips), End (November or later in summer before February–March surveys) and None (no flows). | |
24-h rainfall (Rain24hr) | True or false if rain observed in the preceding 24 h. | |
Survey air temperature (SurveyTemp) | Ambient air temperature (°C) recorded at time of survey using a handheld kestrel meter. | |
Rapid habitat assessment: average proportion of three habitat quadrats | ||
Average water depth (WaterDepth) | Water depth measured at three points during site habitat assessments. | |
Average short emergent aquatic vegetation (ShortEmergVeg) | Average proportion of short emergent vegetation. | |
Average total aquatic vegetation (TotalAquaticVeg) | Average proportion of all aquatic vegetation types. | |
Average tall emergent aquatic vegetation (TallEmergeVeg) | Average proportion of tall emergent vegetation. | |
Average low growing aquatic vegetation (LowGrowVeg) | Average proportion of low growing aquatic vegetation. | |
Average floating aquatic vegetation (FloatingVeg) | Average proportion of floating aquatic vegetation. | |
Average submerged aquatic vegetation (SubmergedVeg) | Average proportion of submerged aquatic vegetation. | |
Average open water (OpenWater) | Average proportion of open water. | |
Catchment name | ||
Catchment (Catchment) | Macquarie, Gwydir |
Environmental predictors
We derived a set of environmental predictors based on known or suspected influence on breeding attempts and success of flow-dependent frogs (see Table 1 for details on how each was calculated). Air temperature during the survey, rainfall (immediate and in the preceding 1, 7 and 15 days), seasonality, vegetation structure and water depth are known to influence frog calling and success (Ocock et al. 2016; Littlefair et al. 2021). We represented wetland inundation extent and inundation duration with metrics based on antecedent conditions using satellite-derived inundation maps and river flow gauge data (Thomas et al. 2015). The river gauge data were standardised for each gauge across the whole period of the study by converting all values to a relative rank (i.e. percentile, range 0–1) to allow comparison between the catchments in the global model. The data pre-processing was performed using R package dplyr (ver. 1.1.4, H. Wickham, R. François, L. Henry, K. Müller, D. Vaughan and Posit Software, see https://CRAN.R-project.org/package=dplyr). To test if breeding responses varied geographically, we included catchment (Gwydir and Macquarie) as predictors. Overall, 22 explanatory covariates were derived (Table 1).
Analyses
We first collated the VAES data to calculate the total abundance for the three guilds: non-burrowing ground frogs (‘flow-dependent’), predominantly arboreal frogs and burrowing frogs. We also collated the number of species heard calling and number of surveys where metamorphs were recorded.
We undertook detailed analyses to investigate the importance of the various hydrological and habitat covariates as drivers on breeding attempts (calling abundance index) and breeding success (number of metamorphs) in flow-dependent frog species using a random forest machine-learning algorithm (Fig. 4). The Random Forest is an ensemble learning method and details of the algorithm is provided in Breiman (2001). This analysis approach can handle both linear and non-linear relationships and the presence of multi-collinearity among the input covariates. We used the ‘ranger’ package (ver. 0.16.0, see https://cran.r-project.org/package=ranger; Wright et al. 2017) in the software program R, which allows fast implementation of the random forest.
Data analysis approach for identifying key drivers of flow-dependent frog breeding attempts (male calling) and breeding success (number of recently metamorphosed frogs).
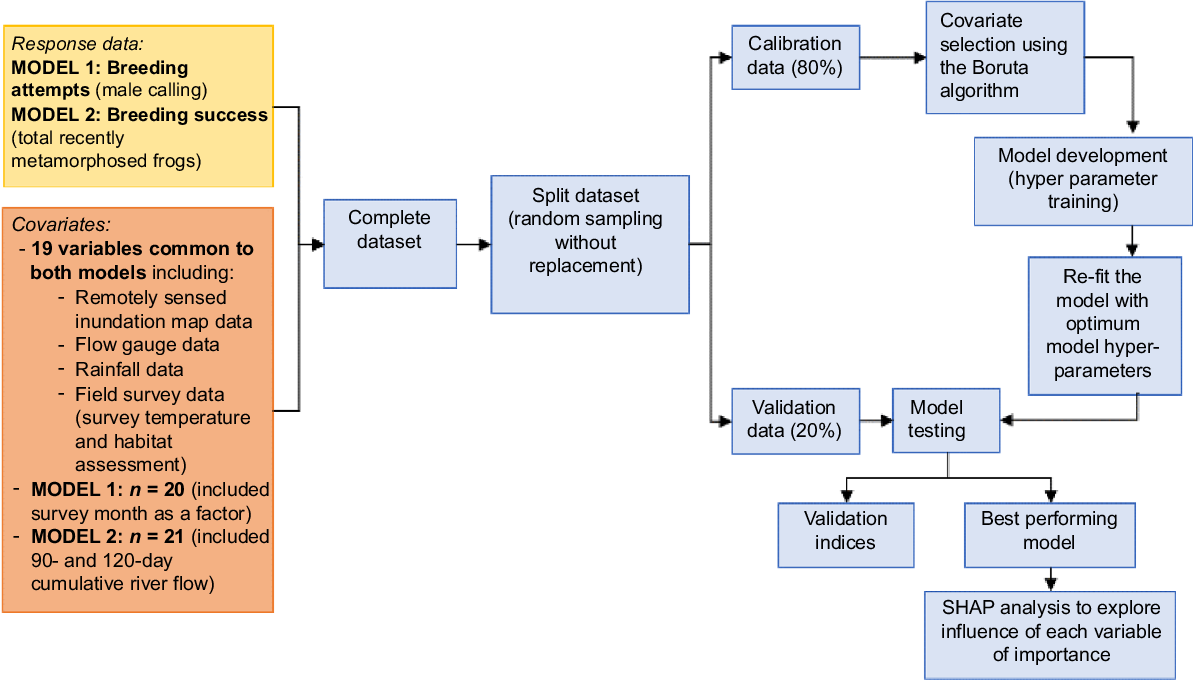
We ran separate models for breeding attempts and breeding success as we had hypothesised suitable conditions for male frogs to call and attempt breeding may be different to factors that increased breeding success (e.g. number of individuals successfully completing larval development to the terrestrial stage). We modelled breeding attempts using a calling abundance index of the flow-dependent species as the response variable (see Table 2 for the list of species). To do this, we converted the index categories for each flow-dependent species to a number where we used the minimum number of the range the index represented (i.e. ‘rare’ was converted to 1, ‘common’ to 6, ‘abundant’ to 11 and ‘very abundant’ to 20) and then summed the converted number for all flow-dependent species to obtain one abundance total for each site survey (potential max. value = 120). To evaluate the important drivers of breeding success, we modelled total abundance of metamorphs from all flow-dependent species as the response. We combined counts from the two catchments to create a global dataset (calling: Global and metamorphs: Global). Nineteen covariates were common to both models. The breeding attempts model (20 covariates in total) included survey month (i.e. September, November or March) as we were interested if season was an important influence on calling. The breeding success models (21 covariates total) included 90- and 120-day cumulative river flow metrics as we hypothesised these longer time periods, namely lagged dataset, would be relevant to tadpoles completing metamorphosis but not to conditions for calling males.
Scientific name | Common name | Average of all individuals (±s.e., range) | Average of recently developed individuals (±s.e., range) | Number of sites calling recorded | |
---|---|---|---|---|---|
Flow-dependent | |||||
Crinia parinsignifera | Eastern sign-bearing froglet | 18.7 (2.4, 1–213) | 2.8 (0.66, 1–13) | 27 | |
Limnodynastes fletcheri | Barking marsh frog | 12.9 (2.0, 1–289) | 2.7 (1.1, 1–18) | 27 | |
Limnodynastes salmini | Salmon striped frog | 3.2 (0.49, 1–15) | 3.4 (0.8, 1–10) | 14 | |
Limnodynastes tasmaniensis | Spotted marsh frog | 19.7 (1.7, 1–222) | 25.3 (4.7, 1–222) | 29 | |
Litoria latopalmata | Broad-palmed frog | 4.3 (0.7, 1–33) | 1 (N/A, 1–1) | 18 | |
Litoria peronii | Peron’s tree frog | 2.7 (0.3, 1–14) | Nil | 26 | |
Arboreal-dominant | |||||
Litoria caerulea | Green tree frog | 2.0 (0.3, 1–5) | Nil | 5 | |
Litoria rubella | Desert tree frog | 2.1 (0.4, 1–4) | 1 (N/A, 1–1) | 4 | |
Burrowing-dominant | |||||
Cyclorana alboguttata | Striped burrowing frog | 2.1 (0.4, 1–4) | Nil | 5 | |
Cyclorana cultripes | Knife-footed frog | 1 (N/A, 1–1) | 1 (N/A, 1–1) | 1 | |
Cyclorana platycephala | Water-holding Frog | 1 (N/A, 1–1) | 1 (N/A, 1–1) | Not heard | |
Neobatrachus sudelli | Sudell’s frog | 1.7 (0.3, 1–2) | 2 (N/A, 2–2) | Not heard | |
Notaden bennettii | Crucifix frog | Nil | Nil | 1 |
The average (±s.e., min.–max.) number of all individuals and the average number of recently metamorphosed individuals only recorded during VAES at each site survey is presented, with the proportion of all sites the species was heard calling during the 5-min aural surveys. N/A, not available.
The dataset was split into calibration (training data 80%) and validation (test data 20%) using a conditional Latin hypercube sampling (cLHC) strategy. Latin hypercube sampling is a procedure that provides an efficient way of sampling covariates from their multivariate distributions using a stratified random approach. It enables a full coverage of the range of each feature to be selected by maximally stratifying the marginal distribution (Minasny and McBratney 2006). Prior to the model fitting process, covariate selection was performed using the Boruta algorithm, a wrapper function built with the random forest algorithm (Wimalathunge and Bishop 2019). Boruta trains a random forest classifier on the extended dataset and applies an importance measure to evaluate the importance of each covariate where a higher value means the covariate (predictor) is more important (see Fig. 4).
Once the optimum covariates were identified, each model was optimised for key random forest model hyperparameters (mtry, n-nodes, variance type) through 10-fold cross-valuation techniques. Once the optimum model hyperparameters were obtained, those models were validated using leave-one-out cross-validation (LOOCV). Model quality was assessed using: (1) root mean squared error (RMSE) as a measure of model accuracy; (2) normalised RMSE; and (3) Lin’s concordance correlation (LCC), which measures the agreement between observation and predictions of the same variable (the 1:1 line indicates perfect agreement in a validation plot), where low RMSE and high LCC indicate better quality model. This is also indicated by a low value in the variation in the magnitude of errors. Additionally, we calculated mean error (ME) as a measure of model bias, Pearson’s correlation (r) and R2 as most published work reports on model evaluation based on coefficient of determination (R2). The variable importance plots (VIP) were used to determine the explanatory variables with the greatest influence on each response variable. The relationship between the response variable and input features is not directly explainable in complex machine-learning models. We used Shapley Additive Explanations (SHAP), an interpretive machine-learning (IML) approach based on game theory, to identify the relationship between the final features included in the random forest model and the considered response variable. The use of SHAP values allowed us to unlock the ‘black-box’ nature machine-learning algorithm when explaining how the considered covariates influenced the two response variables. We visualised the direction of the relationships between the five most important factors and the two responses variables using the ‘fastshap’ package (ver. 0.1.0, B. M. Greenwell, see https://cran.r-project.org/package=fastshap) in the software program R.
Results
Climatic conditions
River flows and rainfall varied considerably over the study period in both catchments (Fig. 2 and 3). The highest flows into the wetlands were in late 2016 leading to widespread inundation until early 2017, which was followed by severe drought conditions from spring 2017 until early 2020, with 2019 as the driest and warmest year on record (Bureau of Meterology 2020). Water managed for the environment was delivered to the wetlands in both catchments during the study period, though the volumes and flow release timing differed among the years depending on condition of the wetland and water availability (NSW Office of Environment and Heritage 2018, 2019, 2020; NSW Department of Planning, Industry and Environment – Environment, Energy and Science 2021, 2022).
Species abundances
Over the 6 years, 13 frog species were observed from 343 site surveys across 95 survey nights (148 and 195 site surveys in the Marshes and Gwydir, respectively) and over 11 000 individual frogs were recorded during the VAES (sum of heard-only, seen-only and heard-and-seen) (Table 2). Six species comprised 99% of all observations and were all non-burrowing species (n = 11 304). Each of these species were recorded widely across the sites and survey nights and were heard calling at all 29 sites (5-min audio survey). Recently metamorphosed frogs from five of these species were recorded at 28 sites across 81 site surveys (Table 2).
The remaining seven species were rarely recorded, comprising less than 1% of all counted frogs (Table 2). This group consisted of two arboreal and five burrowing species. Overall, these species were recorded (seen or heard) at 18 sites, on 30 survey nights and 41 site surveys, and heard only at 11 sites on 15 different survey nights, nearly all when at least 5 mm of rain had fell in the preceding 7 days. Recently metamorphosed frogs from four of these species were seen in five individual surveys.
Random forests: model section and validation
Boruta selected 12 and 11 variables as the most important for breeding attempts and breeding success respectively. Model validation suggested the breeding attempts model was slightly better than breeding success though both models performed reasonably well, indicating the predictors were suitable for predicting the observed values (Table 3, Fig. 5). The LCC was closer to 1 than 0 and the RMSE was low in both models, though both showed some bias (ME and MAE, Table 3). The RMSE, which shows how far predictions are from the true values, was relatively low for both models indicating a small deviation between residuals and observed data. The higher RMSE in the breeding success model is due to the outliers (Fig. 5). The breeding attempts model also had lower variation in the magnitude of errors (difference between RMSE and ME).
Model | LCC | ME | MAE | RMSE | NRMSE | r | R2 | Total samples | Number of variables | |
---|---|---|---|---|---|---|---|---|---|---|
Breeding attempts | 0.692 | 3 | 10 | 16 | 153.2 | 0.69 | 0.48 | 303 | 12 (20) | |
Breeding success | 0.686 | 2 | 8 | 26 | 149 | 0.69 | 0.47 | 325 | 11 (21) |
‘Total samples’ is the total number of complete observations and ‘Number of variables’ is the number selected using Boruta algorithm as the most important variables (total number of variables in the model). LCC, Lin’s concordance correlation; ME, mean error; MAE, measure of absolute error; RMSE, root mean squared error; NRMSE, normalised root mean squared error.
Influence of flow and habitat on breeding activity and breeding success
The covariate representing inundation extent was the most important influence on the calling abundance index response variable, followed by inundation timing, total volume of flows in the preceding 30 days and water depth (Fig. 6a). The seven other variables included in the important factors had comparatively low influence on breeding responses (Fig. 6a). Inundated area at the time of the survey positively corresponded with calling activity, with the SHAP calling response value increasing linearly 10-fold as wetland inundation increased from 0.5 to 1.0 (Fig. 7). For the covariates relating to timing, calling was higher in September compared to November, and at the start of a flood event compared to other stages (Fig. 7). Increasing water depths up to ~15 cm corresponded with increasing calling, but for further increases in water depth, calling activity decreased. Flow volumes exceeding 0.7 of peak flows (standardised for the catchment over the survey period) had a strongly linear positive influence on calling response (Fig. 7).
The variable importance plots generated from the best performing random forest model developed from the data generated on (a) breeding activity and (b) breeding success showing the top selected variables (see Table 2 for full explanation of the variable abbreviations).
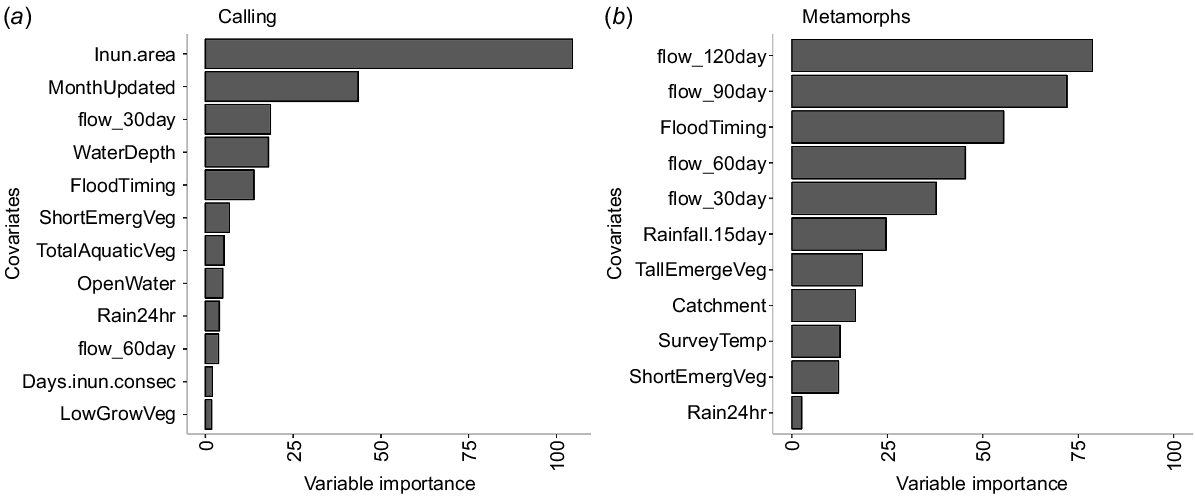
SHAP dependence plots demonstrating the influence of the top five important variables on (a) breeding activity and (b) breeding success.
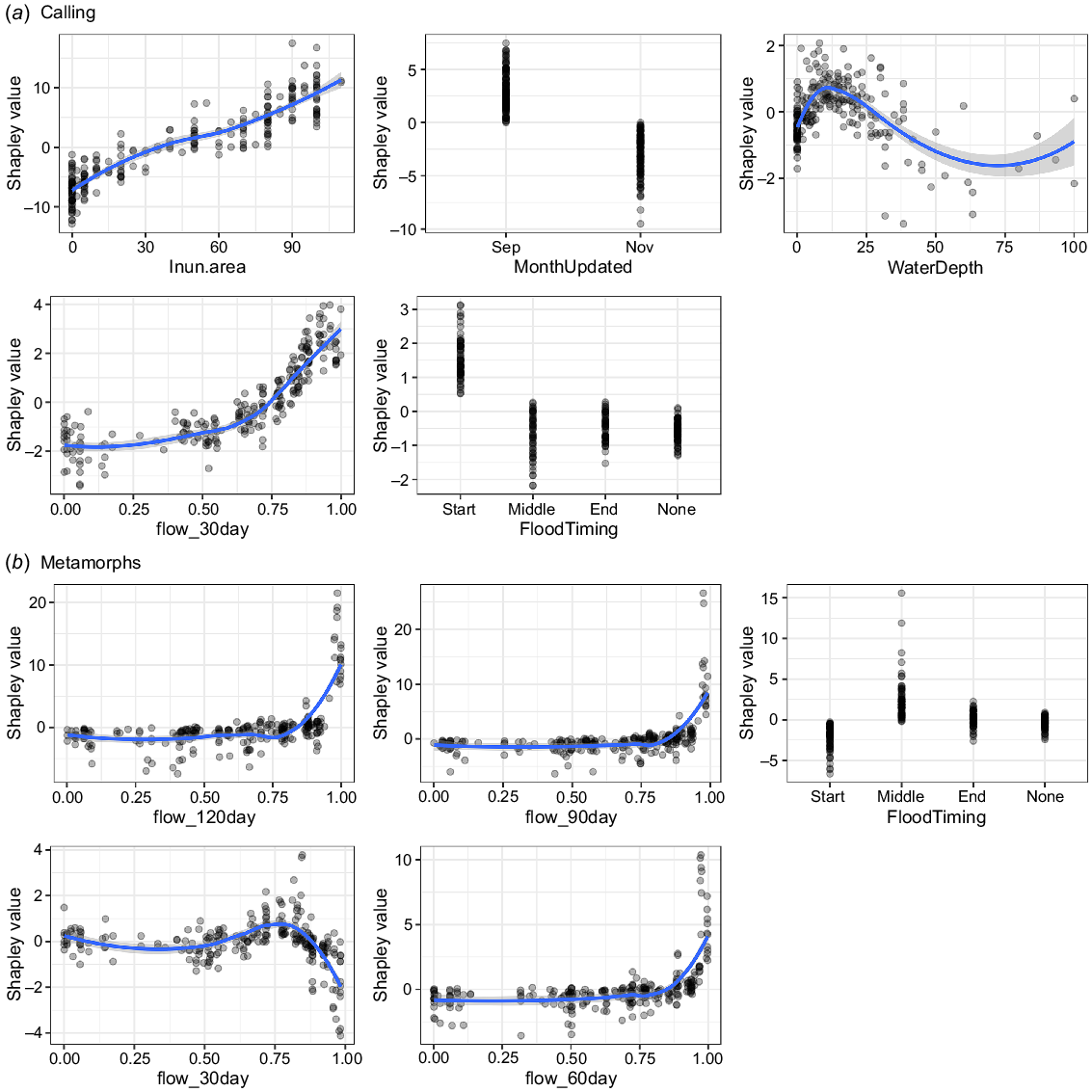
All four covariates representing total flows in the preceding months and timing of the flows were an important influence on the total number of metamorphs (Fig. 6b). The relationships for three of the four flow totals (60, 90 and 120 days preceding the surveys) were positive, where the SHAP value for the number of metamorphs increased sharply, from 1 to 20, when flows increased above 0.75 of the peak standardised flow (Fig. 7). In contrast, flows above 0.8 of standardised flows in the preceding 30 days corresponded with decreasing numbers of metamorphs. The timing of flow events (FloodTiming) was the third most important variable, with the highest numbers of recently metamorphosed frogs associated with the middle stage of a flow event. Although catchment was included in the final set of important factors, there was no clear difference in frog responses between the Macquarie and Gwydir systems.
Discussion
The ability to recreate natural flow variability and support flow-dependent species is constrained by several competing anthropogenic factors, particularly limited water availability and water delivery constraints. Therefore, when making decisions about what environmental benefits can be achieved with the limited water available, water managers need a clear understanding of the flow–ecology relationship, namely ecological links to flow for various fauna and flora groups (Davies et al. 2014; Arthington et al. 2018). Our study identified two key features of the flow pulse linked to recruitment success for amphibians in floodplain wetland systems, the size of the pulse as it relates to area of wetland inundated and the duration of high river flows as a measure of wetland duration, and provides clear advice for managing environmental water to support the persistence of flow-dependent frog species.
In the large inland wetlands of the northern MDB, this current work has confirmed the species that dominate diversity and abundance during both small and large natural and managed wetland filling events identified in previous studies (Ocock et al. 2016; Littlefair et al. 2021; Sarker et al. 2022). We present firm evidence that not all frog species inhabiting floodplain wetlands have the same relationship with flow and wetland conditions and these differences form the basis for assigning species to relevant flow-response guilds. We suggest environmental flow event monitoring and evaluation targets should prioritise reporting on flow-dependent species and metrics of calling abundance and recruitment success as these are meaningfully linked to flow characteristics (Davies et al. 2014; King et al. 2015; Thompson et al. 2018). However, further exploration on temporal abundance trends in relation to the sequence of flow pulses is required. Arboreal and burrowing frogs appear to lack a significant relationship with wetland flows, instead exhibiting increased activity and explosive breeding behaviour in response to rainfall, barometric pressures changes and high night-time temperatures, which were rare during the survey visits. Consequently, breeding success in these species is unlikely to be associated with managed flow releases without concurrent rainfall.
Our results suggest ‘designer’ flows for frogs should prioritise flow events that inundate as large a wetland area as possible during spring and can maintain duration of more than 3 months before drying back (see also Bino et al. 2018). This approach should apply to all management scenarios including when only small volumes of water are available, if they are sufficient to inundate core wetland areas and allow completion of larval development. The higher number of calling males in larger areas of inundation indicates the calling behaviour is limited by the availability of breeding habitat and increasing the extent of this suitable habitat (i.e. increasing the area of wetland inundated) releases this limitation. The limit on calling males may be due to competition; for example, inter-male spacing enforced by aggressive behaviour has been observed in both Limnodynastidae and Litoria males while in calling aggregations (Payne 2014). Flows that arrive in early austral spring (September) provide optimal conditions for calling by most of the flow-dependent species as this is considered a key period for breeding activity (Anstis 2013). Breeding and egg-laying during the early stages of a wetland filling confers benefits for those species as the eggs and tadpoles are less likely to be predated by odonate larvae or fish that have limited abundance in the early stages of the wetland hydroperiod (Pilliod et al. 2010). Tadpole development is also timed to the pulse of available food resources, where items highly nutritional to tadpoles become increasingly abundant soon after the initial flooding (Catenazzi and Kupferberg 2013; Ocock et al. 2018).
High flow volumes in the preceding months were critical to breeding success in our study. Although we expected wetland duration to be strongly linked with increasing number of metamorphs, the site-specific measure of wetland duration (Days.inun.consec) was not included in the best model. Instead, it is likely higher flow volumes were a proxy for longer inundation duration across the whole wetland complex (Thomas et al. 2015). The top two time periods for high flow identified in the model (3 and 4 months) aligned with the expected development time of ~3 months for all species in the flow-dependent guild of our study (Anstis 2013). Tadpole development in these species is largely synchronous, with most individuals emerging from the aquatic habitat at a similar time relative to the wetland being inundated (Hoffmann 2018). Once emerged, the recently metamorphosed frogs are still reliant on wetland habitat for foraging and to prevent dehydration while they increase body size and improve condition sufficiently to survive the terrestrial environment.
By establishing flow–ecology relationships, these species can be included in environmental flow assessments and management. In California, breeding success of river-breeding frogs was clearly linked with specific flow conditions (Kupferberg et al. 2012; Peek et al. 2021); and in recent years, flow recommendations to protect aquatic fauna including threatened river-breeding frog species have been successfully embedded in regulation (Yarnell et al. 2016). Dams require a licence to operate and many now carry conditions for the flow schedule to minimise the risk to recruitment success (e.g. planning releases to avoid stranding egg masses and tadpoles). In Australia, environmental water is held under a legislative framework, though the annual priority targets of that water often are not legislated. Frogs are not a key theme in the Commonwealth government’s Basin-wide Environmental Watering Strategy that supports planning and management of environmental water (Murray–Darling Basin Authority 2019) despite the demonstrated importance of environmental water for sustaining populations of threatened southern bell frog in the southern Murray–Darling Basin (Ocock and Wassens 2018). Environmental flows have been delivered for specific purposes, such as ensuring sufficient water depths are maintained to support successful chick-rearing by colonially-nesting waterbirds (Brandis et al. 2011), providing drought refuge and connectivity (Department of Agriculture, Water and the Environment 2018), and mitigating hypoxic blackwater events (Watts et al. 2018). However, often environmental flow planning aims to maximise multiple environmental outcomes by designing an event hydrograph (flow timing, river heights, duration) to create the wetland inundation extent and duration required for a range of ecological objectives.
In the northern MBD, the timing and duration requirements for breeding success in flow-dependent amphibian species (i.e. spring inundation that maintains extent for at least 3–4 months) are broadly encompassed by the water requirements for growth and germination of low-growing wetland plants and river red-gum forests (Bren 1988), the breeding requirements of many waterbird species (Kingsford and Norman 2002) and the lifecycle of microinvertebrates (Jenkins and Boulton 2003), seen as important for driving wetland productivity and food webs. However, specific river temperature and flow heights linked to spawning and egg-hatching in some native fish including threatened species, which require stable flows of a certain duration (Stocks et al. 2021), may conflict with the ideal hydrograph for pulsed wetland inundation accommodating frogs, vegetation and productivity. Salmonid habitat restoration and protection has driven river management decisions across much of its range in California, but in some instances has negatively affected the threatened foothill yellow-legged frog (Rana boylii) because of differences in the two species’ flow requirements (Railsback et al. 2016). Where combined outcomes cannot be achieved, a priority target for a specific flow event may need to be identified. Accommodating diverse conservation priorities in regulated river systems will require considering the outcomes of multi-year sequence of flow events and further work is needed to identify which particular ‘shaped’ events are suitable for which priority targets and how they could be accommodated in the flow regime.
Our study focussed on determining the important characteristics of single flow events for successful breeding by flow-dependant amphibians. A third crucial factor in the flow–ecology relationship that needs greater investigation is the impact of different time intervals between suitable flow events on amphibian reproductive success. It will be imperative that this return frequency does not extend beyond the expected reproductive life span of flow-dependent amphibians in wetlands subject to periodic drying that has increased in some areas due to river regulation (Mathwin et al. 2023). Gaining an understanding of demographic parameters, specifically adult longevity for all relevant species, would help inform water planning during extended dry periods to ensure sufficient wetland inundation is provided to secure population persistence.
Data availability
Frog survey records are available through NSW Bionet; ancillary data are available on request.
Declaration of funding
This research was funded by the NSW Department of Climate Change, Energy, the Environment and Water – Environment and Heritage Group (and former agencies) and by the Australian Government through Basin Plan Implementation funding.
Acknowledgements
We acknowledge the traditional custodians of the lands and waterways of our study areas, the Gomeroi–Kamilaroi people of the Gwydir Wetlands and the Wailwan people of the Macquarie Marshes, and we pay our respect to elders past, present and emerging. Our thanks to landholders in the Gwydir Wetlands and Macquarie Marshes for supporting our surveys and access to their wetlands. Field surveys would not have been possible without a dedicated team, and we thank those who participated in the frog surveys: Jane Humphries, David Coote, Emma Wilson, Vanessa Cain, Kieran Fitzpatrick, Matt Miles, Stephanie Suter, Bradley Clarke-Wood, Steve Eke, Nicola Brookhouse, Joel Honeysett and John Boyd (NSW Department of Planning and Environment, Environment & Heritage Group), Razzaq Sarker, Kyia Eveleigh (University of New England) and Daniel McKenzie (Eco Logical Australia).
References
Acreman MC, Overton IC, King J, Wood PJ, Cowx IG, Dunbar MJ, Kendy E, Young WJ (2014) The changing role of ecohydrological science in guiding environmental flows. Hydrological Sciences Journal 59, 433-450.
| Crossref | Google Scholar |
Arthington AH, Pusey BJ (2003) Flow restoration and protection in Australian rivers. River Research and Applications 19, 377-395.
| Crossref | Google Scholar |
Arthington AH, Kennen JG, Stein ED, Webb JA (2018) Recent advances in environmental flows science and water management – innovation in the Anthropocene. Freshwater Biology 63, 1022-1034.
| Crossref | Google Scholar |
Australian Government (2012) Basin Plan 2012: made under subparagraph 44(3)(b)(i) of the Water Act 2007. Available at https://www.legislation.gov.au/Details/F2012L02240
Bateman HL, Harner MJ, Chung-MacCoubrey A (2008) Abundance and reproduction of toads (Bufo) along a regulated river in the southwestern United States: importance of flooding in riparian ecosystems. Journal of Arid Environments 72, 1613-1619.
| Crossref | Google Scholar |
Berney P, Hosking T (2016) Opportunities and challenges for water-dependent protected area management arising from water management reform in the Murray–Darling Basin: a case study from the Macquarie Marshes in Australia. Aquatic Conservation: Marine and Freshwater Ecosystems 26, 12-28.
| Crossref | Google Scholar |
Bino G, Wassens S, Kingsford RT, Thomas RF, Spencer J (2018) Floodplain ecosystem dynamics under extreme dry and wet phases in semi-arid Australia. Freshwater Biology 63, 224-241.
| Crossref | Google Scholar |
Brandis KJ, Kingsford RT, Ren S, Ramp D (2011) Crisis water management and ibis breeding at Narran Lakes in arid Australia. Environmental Management 48, 489-498.
| Crossref | Google Scholar | PubMed |
Breiman L (2001) Random forests. Machine Learning 45, 5-32.
| Crossref | Google Scholar |
Bren LJ (1988) Effects of river regulation on flooding of a riparian red gum forest on the River Murray, Australia. Regulated Rivers: Research & Management 2, 65-77.
| Crossref | Google Scholar |
Brown C, Campher D, King J (2020) Status and trends in EFlows in southern Africa. Natural Resources Forum 44, 66-88.
| Crossref | Google Scholar |
Bunn SE, Arthington AH (2002) Basic principles and ecological consequences of altered flow regimes for aquatic biodiversity. Environmental Management 30, 492-507.
| Crossref | Google Scholar | PubMed |
Bureau of Meterology (2020) Annual climate statement 2019. (BOM) Available at http://www.bom.gov.au/climate/current/annual/aus/2019/ [Verified 7 September 2023]
Casanova MT, Brock MA (2000) How do depth, duration and frequency of flooding influence the establishment of wetland plant communities? Plant Ecology 147, 237-250.
| Crossref | Google Scholar |
Catenazzi A, Kupferberg SJ (2013) The importance of thermal conditions to recruitment success in stream-breeding frog populations distributed across a productivity gradient. Biological Conservation 168, 40-48.
| Crossref | Google Scholar |
Chen W, Olden JD (2018) Evaluating transferability of flow–ecology relationships across space, time and taxonomy. Freshwater Biology 63, 817-830.
| Crossref | Google Scholar |
Dare GC, Murray RG, Courcelles DMM, Malt JM, Palen WJ (2020) Run-of-river dams as a barrier to the movement of a stream-dwelling amphibian. Ecosphere 11, e03207.
| Crossref | Google Scholar |
Davies PM, Naiman RJ, Warfe DM, Pettit NE, Arthington AH, Bunn SE (2014) Flow–ecology relationships: closing the loop on effective environmental flows. Marine and Freshwater Research 65, 133-141.
| Crossref | Google Scholar |
Department of Agriculture, Water and the Environment (2018) Final report on the northern connectivity event (April–July 2018). (DAWE) Available at https://www.dcceew.gov.au/sites/default/files/documents/final-report-northern-connectivity-event-2018.pdf [Verified 18 August 2023]
Earl JE, Blomquist SM, Harper EB, Hocking DJ, Hunter ML, Jr, Johnson JR, Osbourn MS, Patrick DA, Popescu VD, Rittenhouse TAG, Rothermel BB (2022) Amphibian biomass export from geographically isolated wetlands: temporal variability, species composition, and potential implications for terrestrial ecosystems. Diversity 14, 163.
| Crossref | Google Scholar |
Eskew EA, Price SJ, Dorcas ME (2012) Effects of river-flow regulation on anuran occupancy and abundance in riparian zones. Conservation Biology 26, 504-512.
| Crossref | Google Scholar | PubMed |
Foerster A (2008) Managing and protecting environmental water: lessons from the Gwydir for ecologically sustainable water management in the Murray Darling Basin. Environmental and Planning Law Journal 25, 49-72.
| Google Scholar |
Gates KK, Vaughn CC, Julian JP (2015) Developing environmental flow recommendations for freshwater mussels using the biological traits of species guilds. Freshwater Biology 60, 620-635.
| Crossref | Google Scholar |
Guzy JC, Eskew EA, Halstead BJ, Price SJ (2018) Influence of damming on anuran species richness in riparian areas: a test of the serial discontinuity concept. Ecology and Evolution 8, 2268-2279.
| Crossref | Google Scholar | PubMed |
Hart BT (2016) The Australian Murray–Darling Basin plan: challenges in its implementation (part 1). International Journal of Water Resources Development 32, 819-834.
| Crossref | Google Scholar |
Hoffmann EP (2018) Environmental watering triggers rapid frog breeding in temporary wetlands within a regulated river system. Wetlands Ecology and Management 26, 1073-1087.
| Crossref | Google Scholar |
Holgerson MA, Duarte A, Hayes MP, Adams MJ, Tyson JA, Douville KA, Strecker AL (2019) Floodplains provide important amphibian habitat despite multiple ecological threats. Ecosphere 10, e02853.
| Crossref | Google Scholar |
Jenkins KM, Boulton AJ (2003) Connectivity in a Dryland River: short-term aquatic microinvertebrate recruitment following floodplain inundation. Ecology 84, 2708-2723.
| Crossref | Google Scholar |
King AJ, Gawne B, Beesley L, Koehn JD, Nielsen DL, Price A (2015) Improving ecological response monitoring of environmental flows. Environmental Management 55, 991-1005.
| Crossref | Google Scholar | PubMed |
Kingsford RT (2000) Ecological impacts of dams, water diversions and river management on floodplain wetlands in Australia. Austral Ecology 25, 109-127.
| Crossref | Google Scholar |
Kingsford RT, Norman FI (2002) Australian waterbirds – products of the continent’s ecology. Emu - Austral Ornithology 102, 47-69.
| Crossref | Google Scholar |
Kingsford RT, Mac Nally R, King A, Walker KF, Bino G, Thompson R, Wassens S, Humphries P (2015) A commentary on ‘Long-term ecological trends of flow-dependent ecosystems in a major regulated river basin’, by Matthew J. Colloff, Peter Caley, Neil Saintilan, Carmel A. Pollino and Neville D. Crossman. Marine and Freshwater Research 66, 970-980.
| Crossref | Google Scholar |
Kingsford RT, Porter JL, Brandis KJ, Ryall S (2020) Aerial surveys of waterbirds in Australia. Scientific Data 7, 172.
| Crossref | Google Scholar | PubMed |
Kupferberg SJ, Palen WJ, Lind AJ, Bobzien S, Catenazzi A, Drennan J, Power ME (2012) Effects of flow regimes altered by dams on survival, population declines, and range-wide losses of California river-breeding frogs. Conservation Biology 26, 513-524.
| Crossref | Google Scholar | PubMed |
Littlefair ME, Nimmo DG, Ocock JF, Michael DR, Wassens S (2021) Amphibian occurrence and abundance patterns across a modified floodplain ecosystem. Austral Ecology 46, 1343-1355.
| Crossref | Google Scholar |
Lytle DA, Merritt DM, Tonkin JD, Olden JD, Reynolds LV (2017) Linking river flow regimes to riparian plant guilds: a community-wide modeling approach. Ecological Applications 27, 1338-1350.
| Crossref | Google Scholar | PubMed |
Mathwin R, Wassens S, Gibbs MS, Young J, Ye Q, Saltré F, Bradshaw CJA (2023) Modeling the effects of water regulation on the population viability of a threatened amphibian. Ecosphere 14, e4379.
| Crossref | Google Scholar |
Mims MC, Olden JD (2013) Fish assemblages respond to altered flow regimes via ecological filtering of life history strategies. Freshwater Biology 58, 50-62.
| Crossref | Google Scholar |
Minasny B, McBratney AB (2006) A conditioned Latin hypercube method for sampling in the presence of ancillary information. Computers & Geosciences 32, 1378-1388.
| Crossref | Google Scholar |
Murray–Darling Basin Authority (2019) Basin-wide watering strategy. (MDBA) Available at https://www.mdba.gov.au/publications-and-data/publications/basin-wide-environmental-watering-strategy [Verified 10 September 2023]
Naniwadekar R, Vasudevan K (2014) Impact of dams on riparian frog communities in the Southern Western Ghats, India. Diversity 6, 567-578.
| Crossref | Google Scholar |
Nilsson C, Reidy CA, Dynesius M, Revenga C (2005) Fragmentation and flow regulation of the world’s large river systems. Science 308, 405-408.
| Crossref | Google Scholar | PubMed |
NSW Department of Planning, Industry and Environment – Environment, Energy and Science (2021) Use of water for the environment in NSW: outcomes 2019–20. (DPIE-EES, NSW Department of Planning and Environment) Available at https://www.environment.nsw.gov.au/topics/water/water-for-the-environment/planning-and-reporting/water-for-environment-outcomes-2019-20 [Verified 4 May 2023]
NSW Department of Planning, Industry and Environment – Environment, Energy and Science (2022) Use of water for the environment in NSW: outcomes 2020–21. (DPIE-EES, NSW Department of Planning and Environment) Available at https://www.environment.nsw.gov.au/topics/water/water-for-the-environment/planning-and-reporting/water-for-environment-outcomes-2020-21 [Verified 4 May 2023]
NSW Office of Environment and Heritage (2018) Use of water for the environment in New South Wales outcomes 2016–17. (NSW OEH) Available at https://www.environment.nsw.gov.au/-/media/OEH/Corporate-Site/Documents/Water/Water-for-the-environment/environmental-water-outcomes-report-2016-17-180191.pdf
NSW Office of Environment and Heritage (2019) Use of water for the environment in NSW: outcomes 2017–18. (NSW OEH) Available at https://www.environment.nsw.gov.au/topics/water/water-for-the-environment/planning-and-reporting/water-for-environment-outcomes-2017-18
NSW Office of Environment and Heritage (2020) Use of water for the environment in NSW: outcomes 2018–19. (NSW OEH) Available at https://www.environment.nsw.gov.au/topics/water/water-for-the-environment/planning-and-reporting/water-for-environment-outcomes-2018-19
Ocock JF, Kingsford RT, Penman TD, Rowley JJL (2014) Frogs during the flood: differential behaviours of two amphibian species in a dryland floodplain wetland. Austral Ecology 39, 929-940.
| Crossref | Google Scholar |
Ocock JF, Kingsford RT, Penman TD, Rowley JJ (2016) Amphibian abundance and detection trends during a large flood in a semi-arid floodplain wetland. Herpetological Conservation and Biology 11, 408-425.
| Google Scholar |
Ocock JF, Brandis KJ, Wolfenden BJ, Jenkins KM, Wassens S (2018) Gut content and stable isotope analysis of tadpoles in floodplain wetlands. Australian Journal of Zoology 66, 261-271.
| Crossref | Google Scholar |
Payne A (2014) Observations on the calling behaviour of the Jervis Bay tree frog Litoria jervisiensis. Australian Zoologist 37, 263-266.
| Crossref | Google Scholar |
Peek RA, O’Rourke SM, Miller MR (2021) Flow modification associated with reduced genetic health of a river-breeding frog, Rana boylii. Ecosphere 12, e03496.
| Crossref | Google Scholar |
Pilliod DS, Hossack BR, Bahls PF, Bull EL, Corn PS, Hokit G, Maxell BA, Munger JC, Wyrick A (2010) Non-native salmonids affect amphibian occupancy at multiple spatial scales. Diversity and Distributions 16, 959-974.
| Crossref | Google Scholar |
Pough FH (1980) The advantages of ectothermy for tetrapods. The American Naturalist 115, 92-112.
| Crossref | Google Scholar |
Quentin Grafton R, Chu L, Kingsford RT, Bino G, Williams J (2022) Resilience to hydrological droughts in the northern Murray–Darling Basin, Australia. Philosophical Transactions of the Royal Society A: Mathematical, Physical and Engineering Sciences 380, 20210296.
| Crossref | Google Scholar |
Railsback SF, Harvey BC, Kupferberg SJ, Lang MM, McBain S, Welsh HH, Jr (2016) Modeling potential river management conflicts between frogs and salmonids. Canadian Journal of Fisheries and Aquatic Sciences 73, 773-784.
| Crossref | Google Scholar |
Royle JA (2004) Modeling abundance index data from anuran calling surveys. Conservation Biology 18, 1378-1385.
| Crossref | Google Scholar |
Salinas-Rodríguez SA, Barba-Macías E, Infante Mata D, Nava-López MZ, Neri-Flores I, Domínguez Varela R, González Mora ID (2021) What do environmental flows mean for long-term freshwater ecosystems’ protection? Assessment of the Mexican water reserves for the environment program. Sustainability 13, 1240.
| Crossref | Google Scholar |
Sarker MAR, McKnight DT, Ryder D, Walcott A, Ocock JF, Spencer JA, Preston D, Brodie S, Bower DS (2022) The effect of inundation on frog communities and chorusing behaviour. Ecological Indicators 145, 109640.
| Crossref | Google Scholar |
Stocks JR, Davis S, Anderson MJ, Asmus MW, Cheshire KJM, van der Meulen DE, Walsh CT, Gilligan DM (2021) Fish and flows: abiotic drivers influence the recruitment response of a freshwater fish community throughout a regulated lotic system of the Murray-Darling Basin, Australia. Aquatic Conservation: Marine and Freshwater Ecosystems 31, 3228-3247.
| Crossref | Google Scholar |
Swirepik JL, Burns IC, Dyer FJ, Neave IA, O’Brien MG, Pryde GM, Thompson RM (2016) Establishing environmental water requirements for the Murray–Darling Basin, Australia’s largest developed river system. River Research and Applications 32, 1153-1165.
| Crossref | Google Scholar |
Thomas RF, Kingsford RT, Lu Y, Cox SJ, Sims NC, Hunter SJ (2015) Mapping inundation in the heterogeneous floodplain wetlands of the Macquarie Marshes, using Landsat Thematic Mapper. Journal of Hydrology 524, 194-213.
| Crossref | Google Scholar |
Thompson RM, King AJ, Kingsford RM, Mac Nally R, Poff NL (2018) Legacies, lags and long-term trends: effective flow restoration in a changed and changing world. Freshwater Biology 63, 986-995.
| Crossref | Google Scholar |
Tockner K, Pusch M, Borchardt D, Lorang MS (2010) Multiple stressors in coupled river–floodplain ecosystems. Freshwater Biology 55, 135-151.
| Crossref | Google Scholar |
Vörösmarty CJ, McIntyre PB, Gessner MO, Dudgeon D, Prusevich A, Green P, Glidden S, Bunn SE, Sullivan CA, Liermann CR, Davies PM (2010) Global threats to human water security and river biodiversity. Nature 467, 555-561.
| Crossref | Google Scholar | PubMed |
Watts RJ, Kopf RK, McCasker N, Howitt JA, Conallin J, Wooden I, Baumgartner L (2018) Adaptive management of environmental flows: using irrigation infrastructure to deliver environmental benefits during a large hypoxic blackwater event in the Southern Murray–Darling Basin, Australia. Environmental Management 61, 469-480.
| Crossref | Google Scholar | PubMed |
Wimalathunge NS, Bishop TFA (2019) A space–time observation system for soil moisture in agricultural landscapes. Geoderma 344, 1-13.
| Crossref | Google Scholar |
Wineland SM, Bașağaoğlu H, Fleming J, Friedman J, Garza-Diaz L, Kellogg W, Koch J, Lane BA, Mirchi A, Nava LF (2022) The environmental flows implementation challenge: insights and recommendations across water-limited systems. Wiley Interdisciplinary Reviews: Water 9, e1565.
| Crossref | Google Scholar |
Wright MN, Dankowski T, Ziegler A (2017) Unbiased split variable selection for random survival forests using maximally selected rank statistics. Statistics in Medicine 36, 1272-1284.
| Crossref | Google Scholar | PubMed |
Yarnell S, Peek R, Epke G, Lind A (2016) Management of the spring snowmelt recession in regulated systems. JAWRA Journal of the American Water Resources Association 52, 723-736.
| Crossref | Google Scholar |