Age and growth of Pomatomus saltatrix in the south-western Pacific Ocean (eastern Australia), with a global comparison
Hayden T. Schilling


A Centre for Marine Science & Innovation, University of New South Wales, Sydney, NSW 2052, Australia.
B Sydney Institute of Marine Science, Building 19, Chowder Bay Road, Mosman, NSW 2088, Australia.
C New South Wales Department of Primary Industries, Sydney Institute of Marine Science, Chowder Bay Road, Mosman, NSW 2088, Australia.
D Fishery Monitoring, Fisheries Queensland, Department of Agriculture and Fisheries, Ecosciences Precinct, Dutton Park, Qld 4102, Australia.
E Institute of Marine Sciences, University of California, Santa Cruz, CA 95064, USA.
F Present address: New South Wales Department of Primary Industries, Port Stephens Fisheries Institute, Taylors Beach, NSW 2316, Australia.
Marine and Freshwater Research 74(6) 463-478 https://doi.org/10.1071/MF22216
Submitted: 13 October 2022 Accepted: 22 February 2023 Published: 14 March 2023
© 2023 The Author(s) (or their employer(s)). Published by CSIRO Publishing. This is an open access article distributed under the Creative Commons Attribution-NonCommercial 4.0 International License (CC BY-NC)
Abstract
Context: Pomatomus saltatrix is one of few globally distributed pelagic mesopredators that is exploited heavily throughout its range. Despite the implementation of management strategies, the south-western Pacific Ocean (eastern Australian) population has few published estimates of the key life-history parameters including growth.
Aims: To estimate the age and growth of P. saltatrix in the south-western Pacific and compare these with the age and growth in other populations.
Methods: Age estimates were made using whole otolith readings and an age–length key was used with a length frequency distribution to estimate the age structure of the population. Eight different growth models were compared within a Bayesian framework for both juvenile and overall growth.
Key results: The Schnute growth equation provided the best fit for overall growth and yielded parameter values of a = −0.15, b = 2.56, Size-at-age 1 = 24.38-cm fork length (FL) and Size-at-age 4 = 47.36 cm FL.
Conclusions: P. saltatrix in the south-western Pacific has a growth rate similar to that in other populations of P. saltatrix.
Implications: Despite its geographically and genetically distinct populations, P. saltatrix demonstrates a generally consistent life-history strategy of fast growth and high mortality, except for the north-western Atlantic population, which has lower mortality.
Keywords: age validation, Australian, Bayesian growth models, bluefish, life history, mortality, recreational fishing, Schnute growth curve, tailor, whole otolith.
Introduction
Pomatomus saltatrix (Linnaeus) has many common names throughout its global distribution, including bluefish, tailor, elf, shad, tassergal and anchova. It is a mobile pelagic mesopredatory fish found throughout subtropical and temperate latitudes (Fig. 1). There are eight major populations, located in the western Mediterranean Sea, eastern Mediterranean–Black Sea, the north-western Atlantic, eastern Atlantic, south-western Atlantic, western Indian, eastern Indian and south-western Pacific oceans (Juanes et al. 1996). Their circumglobal distribution has been shaped by paleoclimate, water temperatures and water depths, and has resulted in extremely limited genetic mixing among populations (Goodbred and Graves 1996; Miralles et al. 2014).
![]() |
As a traditional food source and artisanal fishery species (Silvano and Begossi 2005), P. saltatrix is targeted around the world primarily by recreational fishers for sport and food, although commercial operations continue to harvest significant quantities (14 731 tonnes in 2015; Food and Agriculture Organization of the United Nations 2017). P. saltatrix is typically found around surf beaches, headlands, bays and estuaries (Pollock 1984; Zeller et al. 1996; Potts et al. 2016; Schilling et al. 2017). During the 20th century, many populations of P. saltatrix were noted to be in decline, most likely because of overexploitation (Northeast Fisheries Science Center 1997; Maggs et al. 2012; Nieto et al. 2015; Lovett et al. 2020). This prompted the introduction of regulations and management plans, including seasonal closures, size limits and bag limits, and commercial quotas, which resulted in most stocks showing signs of stabilisation or improvement (Maggs et al. 2012; Northeast Fisheries Science Center 2015; Lovett et al. 2020).
Across populations, P. saltatrix shows similarities in migration (Shepherd et al. 2006; Brodie et al. 2018), spawning patterns (Sabatés and Martin 1993; Schilling et al. 2019), and early life history or recruitment (Juanes et al. 1996; Schilling et al. 2018, 2020). Although their life history is broadly similar globally, P. saltatrix may have different growth and mortality rates across different populations (Juanes et al. 1996). These differences are evident in the maximum ages observed in each population. For example, in the north-western Atlantic, fish aged 12 years or older occur regularly (Robillard et al. 2009); however, the other populations rarely contain fish older than 6–8 years (van der Elst 1976; Ceyhan et al. 2007; Smith et al. 2013; Leigh et al. 2017).
Given the potential for such large variability in life-history parameters among populations, it is necessary to estimate population-specific parameters. The eastern Indian Ocean, south-western Pacific Ocean and eastern Atlantic Ocean P. saltatrix populations are the only stocks without peer-reviewed studies on their growth and mortality rates. The two Australian stocks (eastern Indian Ocean and south-western Pacific Ocean) are genetically distinct (Nurthen et al. 1992), and stock assessments have been conducted (Smith et al. 2013; Leigh et al. 2017; Lovett et al. 2020).
A key aspect of stock assessment is age estimation, which defines the important parameters of growth and mortality, the two most influential demographic characteristics controlling the productivity of fish populations (Campana and Thorrold 2001). If these parameters are not accurately understood, fished populations may be at risk of becoming overfished. In Australia, P. saltatrix age composition, growth and mortality are underpinned by ages estimated from counts in annuli in whole otoliths (Leigh et al. 2017). However, the use of whole otoliths has not been fully accepted as an ageing method for P. saltatrix in Australia, so some uncertainty still surrounds the growth, mortality and age structure of Australian P. saltatrix populations. Hoyle et al. (2000) and Brown et al. (2003) validated the annual periodicity of growth checks in whole otoliths in eastern Australia by using fluorescent marking methods (Brown et al. 2003) and marginal increment analysis (Hoyle et al. 2000; although the results were conclusive only for otoliths with one translucent ring), but comparisons of whole and sectioned otoliths were limited. This is despite sectioned otoliths being the preferred method of age estimation in the north-western Atlantic population, where annuli have been validated with high precision (Robillard et al. 2009).
In eastern Australia, P. saltatrix has historically supported important commercial and recreational fisheries (Lovett et al. 2020). Total commercial catch taken from eastern Australia was ~120 tonnes in 2020, with recreational harvest being estimated to be of a similar magnitude (Murphy et al. 2020; Teixeira et al. 2021). Owing to the importance of P. saltatrix to commercial and recreational fisheries in eastern Australia, together with past concerns for the stock, it is important to understand its key life-history parameters to accurately assess and sustainably manage the south-western Pacific (eastern Australia) population. Fishery-dependent monitoring of the length and age structure of the recreational and commercial harvest is routinely undertaken by state fisheries agencies. To complement this, the aims of this study were to (1) validate the use of whole otoliths as an appropriate ageing tool, (2) estimate growth and mortality rates, and (3) compare these key life-history parameters with those in other global populations of P. saltatrix, so as to identify key differences and potential drivers of differences in life history.
Materials and methods
Fish collection
To model growth using size-at-age data, otoliths were collected from 4049 P. saltatrix individuals in eastern Australia (25–37°S) that were sourced from both the commercial and recreational fishing sectors in conjunction with targeted sampling of juvenile fish (Table 1). In New South Wales (NSW), fish from the commercial sector were sampled from ports of landing (the Sydney Fish Market and regional fishermen’s co-operatives). NSW recreationally harvested fish were sampled through surveys of beach fishers and donations of biological material from a recreational citizen science program (NSW Department of Primary Industries Research Angler Program https://www.dpi.nsw.gov.au/fishing/recreational/resources/fish-tagging/researchangler). Where possible, all fish were measured for fork length (FL) and total length (TL) to the nearest 0.1 cm, and weight to the nearest gram, and sex was determined by macroscopic examination of the gonads (Schilling et al. 2019). Otoliths were removed by dissection in the laboratory, cleaned in fresh water and stored dry.
![]() |
In Queensland (Qld), fish were sampled between the NSW–Qld border (28.1°S) and Baffle Creek (24.5°S) through the Fisheries Queensland Fishery Monitoring Program (Fisheries Queensland 2013). Commercial catches, to be representative of the Qld harvest, were routinely sampled year-round from fishers’ residences and seafood processors. Recreational catches, to be representative of the Qld harvest, were routinely sampled year-round using a variety of sampling strategies, including boat-ramp surveys, roving beach surveys, competition sampling and the Keen Angler Program. Whole fish or fish frames were measured for FL to the nearest 1 cm, and, where possible, sex was recorded. Otoliths were removed from a subset of fish (with an annual calendar year cap of 10 otoliths per each 1-cm-FL length class), cleaned in fresh water and stored dry.
This research includes computations using the computational cluster Katana supported by Research Technology Services at UNSW Sydney (https://doi.org/10.26190/669x-a286). This research was approved by the NSW Department of Primary Industries Fisheries Ethics Committee (ACEC# 14/14) and fish were collected under NSW DPI Scientific Collection Permit (P03/0086(F)-8.1).
Comparison between whole and sectioned otoliths
To validate the use of whole otoliths as an ageing method for P. saltatrix, increment counts from whole otoliths were compared to increment counts from sections of the same otoliths in a blind reading (randomised order), by first reading whole otoliths then sectioning the same otoliths. Increments were defined as opaque rings on the otoliths. Age estimates from sectioned otoliths have been fully validated and show high precision in the north-western Atlantic P. saltatrix stock (Robillard et al. 2009). Using the NSW Department of Primary Industries (DPI) Fisheries database, five fish were randomly selected from each 5-cm-FL size bin (16 bins; 0–80 cm). All available otoliths were used for size bins with fewer than five otoliths (total n = 71). The left otolith was used for consistency, except when it was broken. Whole otoliths were read by immersing the otolith in water and illuminating them by using reflected light.
Interpretation of otolith increment macrostructure was undertaken according to the fish ageing protocols for the Fisheries Queensland Fishery Monitoring Program Ageing protocols (Fisheries Queensland 2017, 2018), with some minor modifications such as immersion in water instead of oil, a variation to the classification of edge type and a variation in the reader training and qualification procedure. Briefly, the methods used here involved counting the number of annual increments between the primordium and the otolith edge, starting with the first annual increment approximately forming near the anterior edge of the antirostrum (Brown et al. 2003), and an edge classification assigned as ‘thin’, ‘medium’ or ‘wide’. A ‘thin’ edge was defined as <20% width of the previous translucent zone or increment not fully visible around the whole edge of the otolith; a ‘medium’ edge was defined as 20–70% width of the previous translucent zone; and a ‘wide’ edge was defined as >70% width of the previous translucent zone. Images were taken using a dissecting microscope (Leica M80, Switzerland) with HD camera at 7.5× magnification. For each otolith, two whole otolith readings were performed without knowledge of fish length or sample data.
To prepare a sectioned sample, an otolith was embedded in resin and sectioned transversely across the primordium to 30–35-μm thickness by using a low-speed saw. The section was polished to remove saw marks, and covered with mounting medium (Safety Mount #4, Fronine, Australia) and a coverslip. Sectioned otoliths were read using the procedure described by Robillard et al. (2009); however, reflected light was used instead of transmitted light and the sections were not burnt. For each sectioned otolith, two counts were made without knowledge of fish length or sample data. An example of an otolith read using both methods is included in the Supplementary material (Supplementary Fig. S1).
The two whole otolith reads of each otolith and two sectioned otolith reads of each otolith were averaged for use in the comparison. Within-method and among-method comparisons were conducted using age-bias plots and average percentage error (APE). As recommended by McBride (2015), bias was assessed using a combination of a maximally pooled method (McNemar 1947), a diagonally pooled method (Evans and Hoenig 1998) and an unpooled method (Bowker 1948).
APE was defined as:

where xij is the ith age for the jth fish, is the mean age for the jth fish, R is the number of times that each fish was aged (assumed to be the same for all fish, here R = 2), and n is the number of aged fish in the sample (Beamish and Fournier 1981), here n = 71. Bias and precision calculations were conducted using the ‘FSA’ package (ver. 0.8.20, D. H. Ogle, see https://cran.r-project.org/package=FSA) in R (ver. 3.4.3, R Foundation for Statistical Computing, Vienna, Austria, see https://www.R-project.org/).
Age and growth-rate determination
Age estimates were collated for two otolith collections taken from NSW and Qld (Table 1), the two eastern Australian states where P. saltatrix is most abundant. All otoliths were read using whole otoliths, following the Fishery Monitoring Fish Ageing Protocols (Fisheries Queensland 2017, 2018), except for three otoliths that were broken but had intact cores, and so were read from sectioned otoliths. For the Qld collection, otoliths are read annually, immediately after a reader passes a competency test on a reference collection. Readers must exceed a set level of precision and bias for increment count and edge type (Fisheries Queensland 2017, 2018). To pass the competency test, a reader must not show unacceptable bias or precision. Unacceptable bias for this species occurs when the calculated bias is ≥1 increment for all groups of increment counts or when the calculated bias shows a divergent trend across increment counts. Unacceptable precision occurs when the index of average percentage error (IAPE) for increment counts is ≥8%. Readers must also obtain an average agreement on edge classifications (New 50%, Intermediate 50%, Wide 70%). After reading the annual sample, a reader must immediately re-read 200 otoliths and pass the same prescribed level of precision and bias for increment count and edge type before the data are added to the database. For the NSW collection, otolith reading was conducted in batches over 2 months by a single qualified reader (having recently passed the Qld competency test described above). After every batch of otolith readings, 200 random otoliths were re-read and tested for precision and bias to ensure consistent interpretation before the data were entered into the database.
Annual formation of increments has been validated for sectioned P. saltatrix otoliths in the north-western Atlantic by using marginal increment analysis (MIA) up to Age 8 (MIA; Robillard et al. 2009), and previously in eastern Australia by using otolith fluorescence marking with a tag–recapture program (Brown et al. 2003) and MIA (Hoyle et al. 2000). The previous MIA from this region showed only clear results for otoliths with a single translucent ring. To further validate annual increment formation in eastern Australia in this study, MIA and assessment of the otolith edge were conducted on the whole otoliths in the NSW dataset. Measurements of the distance from the most recently formed increment to the otolith edge and between the most recently formed increment and the previous increment in whole otoliths, were taken to calculate the marginal increment ratio (MIR). The otolith edge was also classified as being translucent or opaque. For otoliths that displayed translucent edges, the width of the translucent edge was recorded. The translucent-edge widths were defined relative to the width of the previous translucent increment as narrow (5–30%), medium (30–60%) or wide (>60%). MIA and edge-type analysis were restricted to fish with one or two visible increments, to ensure sufficient sample sizes each month. All otoliths were examined using the camera and microscope setup described above. The MIA classification rules were slightly different from the edge-classification rules used in the ageing analysis (to conform to local Fisheries standards); however, as the sole purpose of the MIA was to confirm the annual formation of otolith increments, this did not affect the ageing process.
To provide a better estimate of juvenile growth rates in eastern Australia, a subset of 61 otoliths from Age-0 fish were hand-polished with fine lapping paper until daily increments could be counted. These fish ranged in size from 3.9 to 19.5 cm FL. Daily increments in young-of-the-year P. saltatrix have previously been validated (Nyman and Conover 1988), but it has been difficult to identify daily growth rings in older fish (Brown et al. 2003).
The ageing method (Fisheries Queensland 2017) advises that to assign an age class (number of birthdays a fish is assumed to have), otoliths with a ‘wide’ edge observed during the months of increment formation have one increment added to their increment count to account for the delay in the increment formation in that individual. This adjustment corrects the age of fish in which the otolith increment should have formed in the last month, but is not yet visible on the very edge of the otolith (Fisheries Queensland 2017, 2018). To assign each fish to an age class, we followed the adjustment procedure in Fisheries Queensland (2017), whereby, according to the month of capture and type of otolith edge, the increment count was adjusted to generate an age class. If the edge type was ‘new’ and the capture month was August, then the age class was equal to the increment count – 1. If the edge type was wide and the capture month was September–December, the age class was equal to the increment count + 1. In all other scenarios, the age class was equal to the increment count. These adjustments account for the fact that the marginal opaque zones (new increments) do not become visible in all fish at the same time and that all cohorts of tailor move from one age class to the next on the nominal birthdate of 1 September. A biological age (months) for each fish was calculated using the age class of each fish and the following formulae:


where Am is biological age (months), Cm is capture month, and Bm is biological birth month (9 = September in our circumstances).
Before calculating growth rates, the biological age in months or daily increments was converted to decimal years. Fish of Age-class 0 (years) that did not have their age in days estimated were excluded from growth model analyses (n = 659).
Because of the heteroscedastic error structure of the data, owing to the Age-0 fish being aged on a scale (days) different from the annuli-aged fish (years), multiple growth models were fitted to the size-at-age data within a Bayesian framework, which allowed the variance parameter for the Age-0 (daily aged) and adult (annually aged) fish to vary. This process ensured more accurate parameter and error estimations than a shared variance. Eight growth models were fitted, including von Bertalanffy, linear, logistic, power, Schnute Variation 1, Schnute Variation 2 (equivalent to Gompertz), Schnute Variation 3 and Schnute Variation 4 (Supplementary Table S1). Model selection was undertaken on the basis of the-leave-one-out information criterion and the difference in expected log pointwise predictive density from the best model. The Bayesian model-fitting process involved four chains using Markov-chain Monte Carlo sampling with a 25 000 iteration burn-in (warm up) and 25 000 iteration sampling period with a thinning parameter of 5. We used semi-informative priors to assist in model convergence (Supplementary Table S2). Model convergence was assessed by visually inspecting the chains to ensure mixing and checking the values were ≤1.01. This was undertaken using the ‘brm’ function in the ‘brms’ R package (ver. 2.16.3, see https://github.com/paul-buerkner/brms; Bürkner 2017), with models fit using a High Performance Computing system (Katana, University of New South Wales, see https://doi.org/10.26190/669x-a286).
Juvenile growth rates were calculated using the same model selection process as described above, but using only otoliths that were daily aged. We present the best-fitting model in addition to the mean growth rate from a linear model. Using this fitted juvenile growth curve, the predicted birth dates of all small (5–20 cm FL) P. saltatrix individuals collected as part of this study were estimated. It is worth nothing that the juvenile collection may not be representative of the population.
Using the best-performing overall growth model, we refit sex-specific growth curves. The daily aged juveniles (unknown sex) were included in both male and female models to help anchor the growth curves. Differences between sexes were assessed by comparing the 95% credible intervals for the parameters in the growth model.
Total mortality estimation from length and age compositions
The fishery for P. saltatrix in eastern Australia comprises both recreational and commercial harvests in multiple management jurisdictions. To estimate instantaneous total mortality (Z), an overall catch composition was compiled by combining weighted length frequencies representative of annual commercial and recreational catches from both NSW and Qld (Litherland et al. 2016).
To generate representative length frequencies for the Qld commercial P. saltatrix fishery, gill-net, tunnel-net and ocean beach-net catches were sampled year-round as described above. Commercial catch records were used to post-weight the length samples by using spatial and temporal stratification and are considered representative of the harvest (Fisheries Queensland 2013).
To generate representative length frequencies for the Qld recreational P. saltatrix fishery, line catches were sampled year-round as described above. Recreational catch estimates from the state-wide recreational fishing survey (Webley et al. 2015) were used to post-weight the length samples by using spatial stratification and are considered representative of the harvest (Fisheries Queensland 2013). Sampling was undertaken to be representative of the harvest. All fish were measured for FL to the nearest 1 cm, and the percentage of catch generated for each 1-cm-FL bin (Table 2). The length frequency was supplied as the percentage of catch per 2-cm-TL-class bin length. These TL bin percentages were back-converted to FL by using our FL and TL relationship (Eqn 3) and the proportion method of Booth and Isted (1997). Briefly, this method involves all possible TL measurements between 0 and 90 cm (to nearest 0.1 cm) for P. saltatrix being converted into FL by using Eqn 3. The proportion of these TL measurements in each FL centimetre size class was noted and used to construct a TL–FL key in the form of a matrix, which was then multiplied by the number of measurements within each TL size class, generating the predicted FL length frequency data.
![]() |
To generate a NSW commercial harvest length frequency, samples of NSW commercial fishing catches were measured to the nearest 1 cm rounded down (FL) in various locations along the coast (Table 2). The state-wide commercial-catch records and gradings were used to scale up length–frequency samples on the basis of latitude, sample grade and month, similar to the Qld data (Stewart et al. 2018). The recreational length frequencies for NSW were determined by measuring fish caught by recreational fishers (Table 2). These measured fish came from a combination of boat ramp surveys, walking beach surveys and fish donated by recreational fishers through the NSW DPI Research Angler Program.
Age compositions were generated using a combined age–length key that was created using all the aged fish from both NSW and Qld otolith collections (NSW and Qld) and the combined representative harvest (weighted by relative harvest estimates) length frequency (Supplementary Table S3). All length frequencies were binned to 2-cm-FL bins to correspond to the coarsest bins in any dataset (the Qld datasets). Ages were assigned to fish using the method described in Isermann and Knight (2005). The length composition of the population was assumed to be captured by the length composition of the harvest above a certain size (see Supplementary Table S4) and was used to estimate Z by using the Chapman–Robson method (Chapman and Robson 1960). The first age group included was 1 year older than the age of peak abundance (see Supplementary Table S5), as recommended by Smith et al. (2012). This accounts for the truncated size distributions resulting from the minimum legal lengths. The Chapman–Robson estimator presented by Hoenig et al. (1983) is:
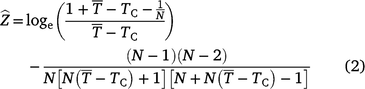
where is the mean age of fish in the sample of fish that are greater than or equal to age TC, TC is the age of full recruitment, and N is the sample size of fish greater than or equal to age TC. The first term transforms the Chapman–Robson estimate of survival into an estimate of Z, and the second term reduces the bias that is induced by the transformation. Age compositions and Z were calculated using the ‘FSA’ package (ver. 0.8.20, D. H. Ogle) in R (ver. 3.4.3, R Foundation for Statistical Computing).
Global life-history comparison
Comparing the life-history parameters of the eight major global populations of P. saltatrix required a compilation of the most recent estimates of growth and mortality from the five populations from the eastern Atlantic, north-western Atlantic, south-western Atlantic, western Indian and eastern Indian oceans. The two populations (eastern and western) of the Mediterranean Sea showed the same life history and so were considered together (Ceyhan et al. 2007; Sabatés et al. 2012). The collected data from the literature were combined with the estimates in this study for the south-western Pacific Ocean population.
In assembling the data, peer-reviewed literature was used when available; however, there are no estimates of total or fishing mortality available for the eastern Atlantic population. The growth model provided for the eastern Atlantic population (Champagnat 1983) did not match the size–at-age data or the fitted curve; therefore, parameters for this equation were recalculated using the size-at-age data in Champagnat (1983). A von Bertalanffy growth curve was fitted using the ‘FSA’ package (ver. 0.8.20, D. H. Ogle) in R (ver. 3.4.3, R Foundation for Statistical Computing).
All reported growth models for global P. saltatrix populations took the form of the von Bertalanffy growth function (VBGF); however, the parameters of the VBGF were not compared because some of these growth models had large |t0| values (>1). This suggests poor modelling of juvenile growth and therefore a questionable k growth parameter (Supplementary Table S6). This is because if the t0 value is not accurate, the slope of the growth curve (the rate of growth) is likely to be biased (either too shallow or too steep). Thus, comparisons of size-at-age and growth rates were made among populations. Comparisons of growth rates among populations therefore consisted of (1) comparing Sizes-at-age 1–5 years, and (2) comparing growth in the year preceding each of these ages. These ages were chosen because the growth equations for each population fit the data reasonably well across this age range. Size-at-age was calculated directly from the reported growth equations. All populations were constrained to a biologically relevant size 0 cm FL at Age 0 to overcome the poor fits to juvenile growth in the reported studies by using the VBGF to provide an estimate of growth rate in the first year.
An individual outlier
A single large P. saltatrix individual (90 cm FL, 103 cm TL, 7.8 kg, female) was donated to the NSW DPI Research Angler Program in May 2018, after the sampling period of this study had finished. This fish was captured during November 2017 in the atypical environment of an intermittently closed and open lagoon (ICOLL) on the NSW south coast (St George’s Basin; 35.12°S, 150.60°E). This fish was by far the largest individual recorded in the current study and was estimated to be double the maximum biological age for the other 4049 aged fish (14 years; Supplementary Fig. S2) and larger than all 31 778 fish measured as part of the length–frequency sampling. The exceptional size and longevity of this individual may be due to its atypical collection environment where it may have been trapped. As an outlier, this individual was not included in the statistical comparisons of whole and sectioned otoliths or growth models.
Results
Morphometric relationships
Using the sampled fish from the NSW portion of the south-western Pacific population, the relationship between TL (cm) and FL (cm) was described by (r2 = 0.997, P < 0.001):

and the relationship between FL (cm) and weight (W; g) was described by (r2 = 0.998, P < 0.001):

Comparison between whole and sectioned otoliths
No significant bias was observed between the mean whole increment counts and mean sectioned otolith increment counts for up to six increments (Fig. 2, Table 3). The differences in mean increment counts between both methods were all ≤1 increment and spread evenly across predicted ages up to Age 6 (Fig. 2a) and sizes up to 80 cm (Fig. 2b). Five otoliths were available in all length classes except for the following: 60.1–65 cm FL (four otoliths), 65.1–70 cm FL (three otoliths), 70.1–75 cm FL (three otoliths) and 75.1–80 cm FL (three otoliths).
![]() |
The variable appearance of the whole otoliths was reflected by a medium precision when re-reading the otoliths (APE = 4.51; Table 3). Nonetheless, there was agreement in increment counts for 92.6% of otoliths. For the otoliths with inconsistent counts, there was a maximum difference of one between the first and second readings. The APE for sectioned otoliths was also acceptable, although greater than for whole otoliths (APE = 6.678; Table 3).
For the exceptionally large P. saltatrix, eight increments were observed in the whole otolith, but when sectioned, there were 13 increments clearly visible (Fig. S2). The otolith was also considerably more opaque than were otoliths from all other sampled fish and was of an irregular shape compared with otoliths from most large P. saltatrix individuals.
Growth modelling
Using whole otoliths, both MIA and assessment of otolith edge-type analysis throughout the year confirmed that one annulus was formed per year, which is consistent with other global populations. For the south-western Pacific population, this annulus was shown to form between September and January (Fig. 3).
![]() |
The best-fitting growth model was the Schnute growth model Variation 1 (Table S1). This model is expressed as:

where a = −0.15 (−0.24 to −0.08), b = 2.56 (2.35–2.87), Age 1 (T1) = 1, Age 2 (T2) = 4, size at Age 1 (y1) = 24.38 (23.99–24.89) cm FL, size at Age 2 (y2) = 47.36 (47.00–47.71) cm FL (Fig. 4; estimates are the median and 95% credible intervals for the parameter estimates). Unlike the VBGF, this model has no asymptotic length. The sex-specific growth models (Schnute Variation 1) showed that female fish grow faster than male fish, although this difference is minor (female being 1.2 cm larger, on average, at Age 1 and 2.2 cm larger at Age 4, Table 4, Supplementary Fig. S3).
![]() |
The VBGF did not accurately describe the growth of smaller or large fish (Fig. 4). We report the fit because the VBGF is the standard growth curve in all fish growth literature; however, we do not recommend the use of these values. There was large variance in the parameter estimates; the best-fitting parameters (with 95% CI) were L∞ = 141.7 cm FL (116.7, 169.8), k = 0.07 year−1 (0.05, 0.09) and t0 = −2.02 (−2.24, −1.79).
Using only the data from fish that had their daily ages estimated (sizes: 3.9–19.5 cm; ages: 32–236 days, n = 61), juvenile P. saltatrix grew ~0.09 cm day−1 (Bayesian linear model; 95% CI 0.08–0.09, size at Age 0 = 1.88 mm (95% CI: 0.97–2.77) r2 = 0.87). The best-fitting growth curve between FL (cm) and age (d; days) is given by the logistic equation (n = 61, P < 0.01):

where L∞ = 20.50 (95% CI: 20.31–20.69) cm FL, k = 0.02 (95% CI: 0.02–0.02) and t0 = 94.31 (95% CI: 0.02–0.02; Fig. 5) days. Using this equation, the estimated birth dates for 659 juvenile fish suggested that some spawning may be occurring in all months except March and June (Supplementary Fig. S4).
Age structure and mortality estimations
In total, 95% of the harvest in eastern Australia (south-western Pacific Ocean population) was between 28 and 46 cm FL, which represents fish aged 1–3 years old, with the majority of the harvest being 2 and 3 years of age (Fig. 6, Tables S5, S6). Because of the truncation of sizes owing to the larger minimum legal length (MLL) in Qld (35 cm TL) than in NSW (30 cm TL), the Qld harvest had proportionally more 3-year-old and fewer 1-year-old fish than did NSW. Very few large old fish were collected from eastern Australia in the present study, with just 35 individuals sampled being >60 cm FL and 11 fish being >5 years of age (Fig. 4, 6). Excluding the outlier described above, the largest (and oldest) P. saltatrix individual sampled was 80.7 cm FL and 7 years old (Fig. 4, 6). Using Ages 3–7, total mortality (Z) was estimated to be 1.62 (95% CI 1.43–1.82).
![]() |
Global life-history comparison
Except for the P. saltatrix population in the north-western Atlantic, which showed a low estimate of Z, all other global P. saltatrix populations had moderate to high estimates of Z (0.90–1.62; Table 5). This pattern is reflected in the maximum observed age in each population, with the north-western Atlantic population containing the oldest fish. Growth was similar between all populations up to approximately Age 3. After this age, the north-western Atlantic and eastern Atlantic populations showed larger sizes at each subsequent age (Fig. 7, 8). All populations, except the south-western Atlantic and eastern Atlantic, showed that fast growth reached a plateau after Age 2 (Fig. 7). Juvenile growth (<1 year) was poorly described by the VBGF with |t0| values being >1 in all populations except for the eastern Indian, eastern Atlantic and north-western Atlantic ocean populations (Fig. 7, Table S6).
![]() |
![]() |
![]() |
Discussion
The south-western Pacific Ocean (eastern Australian) P. saltatrix population is characterised by fast, non-asymptotic growth up to the normal maximum reported age (7 years). Modelled growth of the species likely fails to reach an asymptotic length because of high mortality rates (i.e. low numbers of old, large fish). Growth and mortality in P. saltatrix shows two main patterns among global populations. All populations have fast growth and high mortality rates, except for the north-western and eastern Atlantic ocean populations. The north-western Atlantic population has fast growth but considerably lower mortality rates, which is reflected by higher proportions of large, old fish not found elsewhere. The general similarity in life histories over such a broad geographical range indicate that P. saltatrix represents a key example of the pelagic mesopredator niche, but one that may be strongly influenced by fishing pressure as demonstrated by the potential for high mortality rates.
Age determination
Our study has validated the use of whole otoliths for accurate age estimation of P. saltatrix for fish routinely found in catches in eastern Australia, with sections being better for atypical exceptionally old P. saltatrix. Increments counted in whole otoliths showed close agreement with those counted in sectioned otoliths up to estimated Age 6. Whole otoliths are used for ageing P. saltatrix in the Mediterranean, eastern Indian, western Indian and the south-western Pacific populations (Govender 1999; Ceyhan et al. 2007; Leigh et al. 2017), which are regions where large, old individuals are scarce.
Marginal increment and edge-type analyses both showed one annulus forming per year. This agrees with previous validation using sectioned otoliths reported from the north-western Atlantic (Robillard et al. 2009) and south-western Pacific (Hoyle et al. 2000). Our study found that formation of increments occurred earlier in the year (c. November) than in a previous marginal increment analysis (December–January), which is likely to be due to the previous use of sectioned otoliths (Hoyle et al. 2000).
In the current study, we conclude that whole otoliths are a valid method of age determination in the south-western Pacific up to Age 6. Our conclusion is based on validating only the first two increments, although there was high agreement with sectioned otolith counts, which have been validated up to 8 years in the north-western Atlantic (Robillard et al. 2009). The current validation agrees with the previous validation work of the first increment and increment periodicity in the south-western Pacific (Hoyle et al. 2000; Brown et al. 2003), and confirms the high agreement (>95%) between increment counts in sectioned and whole otoliths up to Age 4 in the north-western Atlantic (Sipe and Chittenden 2002). Despite the close agreement between whole and sectioned otoliths up to six increments, there was a large difference in increment counts between whole and sectioned otoliths in the largest fish. This was due to the difficulty in interpreting whole otoliths of large fish, particularly increments near the otolith edge. Therefore whole otoliths can be used as an alternative to sectioned otoliths for routine ageing of P. saltatrix in eastern Australia; however, the otoliths from fish larger than 70 cm FL should be sectioned to ensure unbiased age assignment.
The daily growth analysis showed a fast juvenile growth rate of ~0.09 cm day−1, which is within the reported juvenile growth rate in the north-western Atlantic (0.09–0.21 cm day−1; Juanes et al. 1996), faster than juvenile growth in the eastern Indian Ocean based upon tagging (0.02–0.04 cm day−1; Young et al. 1999), and aligns with previous estimates for the western Pacific (0.075–0.13 cm day−1) based upon length frequencies (NSW SPCC 1981). The differences among these populations could potentially be due to the relatively low productivity of the eastern Indian Ocean, in comparison to the high productivity of the north-western Atlantic and western Pacific (Longhurst et al. 1995; Reygondeau et al. 2013). Using our estimated growth rates, we calculated birth dates that span most of the year. Although we saw an obvious peak in births between August and December in our samples, this may be due to biased sampling for juveniles conducted mainly between February and May in NSW. This extended spawning period aligns with previous research, which showed that spawning was not restricted to August–December as originally thought, and confirmed that reproduction and recruitment may occur over a prolonged time period (Schilling et al. 2019).
Growth modelling
The model selection process used in our current study found that the classic VBGF was not well suited to describing growth for the south-western Pacific Ocean (eastern Australian) population. This is because it did not accurately describe juvenile growth and our data did not show a clear asymptotic length. We found that a model without an asymptotic length (Schnute Variation 1) was more accurate. Although few studies of growth in fish suggest models with no asymptotic lengths (e.g. Stewart et al. 2004), this case is consistent with the high mortality rates estimated for the south-western Pacific population, whereby few fish survive long enough to provide size-at-age data from large old fish. Despite the overall better fit provided by the Schnute Variation 1 growth model, this model still underestimated growth of larger, older classes of fish. Because biological theory suggests that there should be an asymptotic length, we caution about extrapolating our given growth rates beyond the ages presented in this study. For example, our growth rate if extrapolated would estimate a 14-year-old fish to be 112 cm FL; however, our large 14-year-old outlier was only 90 cm FL. By including the outlier fish in the growth models, this may have slightly changed the shape of the curve, but this fish may be the outcome of highly atypical growth conditions not experienced by the rest of the population. We found that female P. saltatrix individuals grew slightly faster than did males, which is consistent with the fact that females mature at slightly larger sizes than males (Schilling et al. 2019). Sex-specific differences in growth rates have not been observed in the north-western Atlantic population (Robillard et al. 2009), but have been observed in the Mediterranean (Ceyhan et al. 2007).
All previous studies of P. saltatrix growth have provided estimates of the VBGF parameters; however, these model parameters are likely to be biased by poorly modelled juvenile growth (Fig. 7, Table S6), and therefore should not be compared. Our comparison of growth rates shows that all populations except the south-western Atlantic share an initial fast growth rate (20–30 cm in the first year) before slowing in the second year. The different growth rate observed in the south-western Atlantic population (Haimovici and Krug 1996) may be due to ageing errors, with the first annual increment potentially being misidentified because of the use of scales instead of otoliths to derive age estimates. If this were the case, then the growth patterns for this population would be likely to match the pattern observed in most other populations. The north-western Atlantic population has rapid initial growth that is maintained longer than in the other populations, potentially owing to delayed investment in reproduction, because this population has a larger size at maturity than do the other populations (van der Elst 1976; Champagnat 1983; Robillard et al. 2008; Schilling et al. 2019).
Differences in growth rates among and within populations of other species have previously been linked to temperature, with faster growth occurring in higher-temperature environments because of increased metabolic rate (Morrongiello and Thresher 2015; Nicolle et al. 2022). Given that all global P. saltatrix populations experience similar average annual water temperature (Goodbred and Graves 1996; Fig. 1), it is considered unlikely that variations in environmental temperature are driving the difference in growth between the north-western Atlantic population and the other populations of P. saltatrix. However, migrations are not considered when simply looking at average annual sea-surface temperature over the whole species distribution, and all populations of P. saltatrix migrate. For example, the south-western Pacific P. saltatrix population is heavily influenced by sea-surface temperature, with abundance peaking at 21.5°C (Brodie et al. 2018). Population-specific studies may be required to fully understand the importance of environmental temperature for driving basic life-history parameters, such as growth, in P. saltatrix populations.
It is also possible that growth rates are not a response to temperature, but rather regional productivity, although there are still mismatches in productivity and growth rate. The north-western Atlantic and eastern Atlantic populations, which have the highest growth rates, are not the regions with the highest regional productivity, whereas the south-western Atlantic population also occurs in an area with high regional productivity and does not have a higher growth rate (Antoine et al. 1996; Behrenfeld et al. 2006; Stock et al. 2017). Despite the variations in temperature and productivity, growth rates are remarkably consistent among all the populations. This suggests that neither productivity nor temperature are the primary driver of growth rate differences among different populations of P. saltatrix.
We propose the most likely cause of these differing growth rates is fishery-induced evolution, with the populations with higher levels of Z (either from M or F) investing in reproduction earlier and therefore slowing growth (Kuparinen and Merilä 2007; Quince et al. 2008a, 2008b). This is highlighted by the north-western Atlantic and eastern Atlantic populations having the lowest mortality and the fastest growth rates. Indeed a reduction of fishing pressure has been linked to a larger reproductive size in the south-western Pacific population (Schilling et al. 2019).
Mortality
Although we did collect data from a single, exceptionally large individual, this fish was an outlier from the population because of its size (far exceeding the length of 31 778 fish sampled) and age (14 years estimated by sectioned otolith, which made it the oldest of the 4049 fish sampled). The exceptional size and longevity of this individual was attributed to its atypical collection environment of an intermittently closed estuary system on the NSW southern coast, despite the typical habitat for adult P. saltatrix being coastal marine waters. Despite the unusual nature of this fish, it does provide evidence that P. saltatrix in the south-western Pacific, under some circumstances, have potential longevity similar to that observed in the north-western Atlantic population (Robillard et al. 2009). It is worth noting that the most recent stock assessment of the population did not include this individual because it was an atypical individual not representative of the population.
Estimates of total mortality (Z) are similar among global populations (0.90–1.62), with the exception of the north-western Atlantic population, which has by far the lowest Z estimate of 0.34. The south-western Pacific (eastern Australian) population has the highest Z, which is likely to be driven by continued heavy recreational fishing pressure on annual spawning aggregations in the north of their distribution and the combination of variable recruitment and a population consisting of very few age classes (Pollock 1984; Zeller et al. 1996; Leigh et al. 2017). It should be noted that catch-curve analyses of Z assume constant vulnerability, constant recruitment and an unbiased sample; however, these cannot be assumed for this population, particularly when recruitment is thought to be variable (Leigh et al. 2017; Lovett et al. 2020). Although the current study combined multiple fishery sectors, it had only five age classes (Ages 3–7) with which to estimate Z. This means that the estimate of Z in this study should be interpreted cautiously because its heavy reliance on fishery-dependent samples means that it may not be representative of the population overall.
All global populations of P. saltatrix show similar spawning migration patterns, and are also subject to various management strategies that attempt to limit fishing pressure (Maggs et al. 2012). The Mediterranean population appears to be the only population (except the eastern Atlantic) that is not subject to strict management. Although the Mediterranean population does not have the highest estimate of Z, it is assessed to be overexploited, with most of the harvest consisting of small fish because of the lack of a minimum size limit (Ceyhan et al. 2007; Cengiz et al. 2013).
Differences in the life-history parameters of growth and mortality are potentially driving differences in other life-history parameters, including reproductive age. In the populations of P. saltatrix that have sustained fast growth and greatest longevity, length at 50% female maturity (L50) is 45.1 cm FL in the north-western Atlantic population (Robillard et al. 2008) and 38.0 cm FL in the eastern Atlantic population (Champagnat 1983). In the other populations, L50 ranges from 25 to 31.5 cm FL (van der Elst 1976; Bade 1977; Smith et al. 2013; Schilling et al. 2019) and is reflected in the slowing of growth rates by Age 2. This could be a strong evolutionary response signal and may reflect the lower Z in the north-western Atlantic population (and potentially the eastern Atlantic; Swain et al. 2007). With higher survival, these populations may delay the start of their reproductive development to increase investment in somatic growth and, therefore, be larger at first spawning, with potentially higher fecundity in the first spawning season (Schilling et al. 2019).
Conclusions
P. saltatrix in the south-western Pacific (eastern Australia) shows fast growth and high mortality, which is consistent with the global pattern of growth and mortality for most P. saltatrix populations. This species demonstrates a persistent life-history strategy as a successful globally distributed mesopredator, across multiple isolated genetic populations, with differences potentially driven by variation in mortality.
Supplementary material
Supplementary material is available online.
Data availability
The data and code used in these analyses are available at https://github.com/HaydenSchilling/TailorGrowthMortality.
Conflicts of interest
The authors declare that they have no conflicts of interest.
Declaration of funding
This work was supported by an Australian Research Council Linkage Project (LP150100923) and the NSW Recreational Fishing Trust. The funders had no involvement in the design, data collection or analysis of this project.
Acknowledgements
This paper forms part of the PhD thesis of Schilling (2019). Francis Juanes and John Morrongiello commented on the thesis chapter that this paper is based on. Recreational fishers donated fish to the project through the NSW Research Angler Program; special thanks go to Australian Surfcaster and Ben van der Woude for donating large numbers of fish. Queensland recreational fishers, commercial fishers and seafood processors participated in the Fisheries Queensland Monitoring program. The UNSW Stats Central helped with the growth models and Jim Craig (NSW DPI) converted the Qld length frequencies from total length to fork length. Internal reviewers at Fisheries Queensland improved the paper. We thank two reviewers for constructive comments on this paper. This is manuscript number #304 from the Sydney Institute of Marine Science.
References
Antoine, D, André, J-M, and Morel, A (1996). Oceanic primary production: 2. Estimation at global scale from satellite (Coastal Zone Color Scanner) chlorophyll. Global Biogeochemical Cycles 10, 57–69.| Oceanic primary production: 2. Estimation at global scale from satellite (Coastal Zone Color Scanner) chlorophyll.Crossref | GoogleScholarGoogle Scholar |
Bade TM (1977) The biology of tailor (Pomatomus saltatrix Linn.) from the east coast of Australia. MSc thesis, University of Queensland, Brisbane, Qld, Australia.
Beamish, RJ, and Fournier, DA (1981). A method for comparing the precision of a set of age determinations. Canadian Journal of Fisheries and Aquatic Sciences 38, 982–983.
| A method for comparing the precision of a set of age determinations.Crossref | GoogleScholarGoogle Scholar |
Behrenfeld, MJ, O’Malley, RT, Siegel, DA, McClain, CR, Sarmiento, JL, Feldman, GC, Milligan, AJ, Falkowski, PG, Letelier, RM, and Boss, ES (2006). Climate-driven trends in contemporary ocean productivity. Nature 444, 752–755.
| Climate-driven trends in contemporary ocean productivity.Crossref | GoogleScholarGoogle Scholar |
Booth, AJ, and Isted, ED (1997). Comparison of methods used to convert length-frequency data. Fisheries Research 29, 271–276.
| Comparison of methods used to convert length-frequency data.Crossref | GoogleScholarGoogle Scholar |
Bowker, AH (1948). A test for symmetry in contingency tables. Journal of the American Statistical Association 43, 572–574.
| A test for symmetry in contingency tables.Crossref | GoogleScholarGoogle Scholar |
Brodie, S, Litherland, L, Stewart, J, Schilling, HT, Pepperell, JG, and Suthers, IM (2018). Citizen science records describe the distribution and migratory behaviour of a piscivorous predator, Pomatomus saltatrix. ICES Journal of Marine Science 75, 1573–1582.
| Citizen science records describe the distribution and migratory behaviour of a piscivorous predator, Pomatomus saltatrix.Crossref | GoogleScholarGoogle Scholar |
Brown IW, Butcher A, McLennan M, Mayer D (2003) Age validation in tailor (Pomatomus saltatrix). FRDC Report 1999/123, Queensland Department of Primary Industries, Brisbane, Qld, Australia.
Bürkner, P-C (2017). brms: an R package for Bayesian multilevel models using Stan. Journal of Statistical Software 80, 1–28.
| brms: an R package for Bayesian multilevel models using Stan.Crossref | GoogleScholarGoogle Scholar |
Campana, SE, and Thorrold, SR (2001). Otoliths, increments, and elements: keys to a comprehensive understanding of fish populations? Canadian Journal of Fisheries and Aquatic Sciences 58, 30–38.
| Otoliths, increments, and elements: keys to a comprehensive understanding of fish populations?Crossref | GoogleScholarGoogle Scholar |
Cengiz, Ö, Özekinci, U, Öztekin, A, and Kumaova, CA (2013). Growth parameters and mortality of bluefish (Pomatomus saltatrix Linnaeus, 1766) from Gallipoli peninsula and Dardanelles (northeastern Mediterranean, Turkey). Marine Science and Technology Bulletin 2, 1–7.
Ceyhan, T, Akyol, O, Ayaz, A, and Juanes, F (2007). Age, growth, and reproductive season of bluefish (Pomatomus saltatrix) in the Marmara region, Turkey. ICES Journal of Marine Science 64, 531–536.
| Age, growth, and reproductive season of bluefish (Pomatomus saltatrix) in the Marmara region, Turkey.Crossref | GoogleScholarGoogle Scholar |
Champagnat C (1983) ‘Pêche, biologie et dynamique du tassergal (Pomatomus saltator, Linnaeus, 1766) sur les côtes sénégalo-mauritaniennes.’ (IRD Editions)
Chapman, DG, and Robson, DS (1960). The analysis of a catch curve. Biometrics 16, 354–368.
| The analysis of a catch curve.Crossref | GoogleScholarGoogle Scholar |
Evans, GT, and Hoenig, JM (1998). Testing and viewing symmetry in contingency tables, with application to readers of fish ages. Biometrics , 620–629.
| Testing and viewing symmetry in contingency tables, with application to readers of fish ages.Crossref | GoogleScholarGoogle Scholar |
Fisheries Queensland (2013) Fisheries long term monitoring program sampling protocol – Tailor (2007 onwards) Section 1 (V2). Report. Fisheries Queensland, Brisbane, Qld, Australia.
Fisheries Queensland (2017) Fisheries monitoring program sampling protocol – fish ageing: Section 2: Tailor. Report. Fisheries Queensland, Brisbane, Qld, Australia.
Fisheries Queensland (2018) Fisheries monitoring program sampling protocol – fish ageing. Report. Fisheries Queensland, Brisbane, Qld, Australia.
Food and Agriculture Organization of the United Nations (2017) ‘Global capture production.’ (Fisheries and Aquaculture Information and Statistics Service)
Goodbred, CO, and Graves, JE (1996). Genetic relationships among geographically isolated populations of bluefish (Pomatomus saltatrix). Marine and Freshwater Research 47, 347–355.
| Genetic relationships among geographically isolated populations of bluefish (Pomatomus saltatrix).Crossref | GoogleScholarGoogle Scholar |
Govender, A (1999). A statistical procedure for validating age estimates from otolith bands using mark–recapture data, with an illustration for Pomatomus saltatrix. South African Journal of Marine Science 21, 1–8.
| A statistical procedure for validating age estimates from otolith bands using mark–recapture data, with an illustration for Pomatomus saltatrix.Crossref | GoogleScholarGoogle Scholar |
Haimovici, M, and Krug, LC (1996). Life history and fishery of the enchova, Pomatomus saltatrix, in southern Brazil. Marine and Freshwater Research 47, 357–363.
| Life history and fishery of the enchova, Pomatomus saltatrix, in southern Brazil.Crossref | GoogleScholarGoogle Scholar |
Hoenig, JM, Lawing, WD, and Hoenig, NA (1983). Using mean age, mean length and median length data to estimate the total mortality rate. ICES CM 500, 23.
Hoyle S, Brown I, Dichmont C, Sellin M, Cosgrove M, McLennan M (2000) Integrated fish stock assessment and monitoring program. FRDC report 94/161, Queensland Department of Primary Industries and Fisheries Research and Development Corporation, Brisbane, Qld, Australia.
Isermann, DA, and Knight, CT (2005). A computer program for age–length keys incorporating age assignment to individual fish. North American Journal of Fisheries Management 25, 1153–1160.
| A computer program for age–length keys incorporating age assignment to individual fish.Crossref | GoogleScholarGoogle Scholar |
Juanes, F, Hare, JA, and Miskiewicz, AG (1996). Comparing early life history strategies of Pomatomus saltatrix: a global approach. Marine and Freshwater Research 47, 365–379.
| Comparing early life history strategies of Pomatomus saltatrix: a global approach.Crossref | GoogleScholarGoogle Scholar |
Kuparinen, A, and Merilä, J (2007). Detecting and managing fisheries-induced evolution. Trends in Ecology & Evolution 22, 652–659.
| Detecting and managing fisheries-induced evolution.Crossref | GoogleScholarGoogle Scholar |
Leigh GM, O’Neill MF, Stewart J (2017) Stock assessment of the Australian east coast tailor (Pomatomus saltatrix) fishery. April 2017. (Queensland Department of Agriculture and Fisheries: Brisbane, Qld, Australia) Available at https://era.daf.qld.gov.au/id/eprint/5689/7/TailorStockAssessmentReport_2017%20-%20Final%2018052017.pdf
Litherland L, Andrews J, Stewart J, Lewis P (2016) Tailor Pomatomus saltatrix. In ‘Status of Australian fish stocks reports 2016’. (Eds C Stewardson, J Andrews, C Ashby, M Haddon, K Hartmann, P Hone, P Horvat, S Mayfield, A Roelofs, K Sainsbury, T Saunders, J Stewart, I Stobutzki, B Wise) pp. 1–11. (Fisheries Research and Development Corporation: Canberra, ACT, Australia)
Longhurst, A, Sathyendranath, S, Platt, T, and Caverhill, C (1995). An estimate of global primary production in the ocean from satellite radiometer data. Journal of Plankton Research 17, 1245–1271.
| An estimate of global primary production in the ocean from satellite radiometer data.Crossref | GoogleScholarGoogle Scholar |
Lovett R, Leigh GM, Litherland L, Stewart J (2020) Stock assessment of the Australian east coast tailor (Pomatomus saltatrix) fishery. 2020. (Queensland Government: Brisbane, Qld, Australia) Available at https://era.daf.qld.gov.au/id/eprint/7870/1/Tailor%20stock%20assessment%20report%202020.pdf
Lucena, FM, O’Brien, CM, and Reis, EG (2002). Effects of exploitation by two co-existing fleets on the bluefish, Pomatomus saltatrix, in southern Brazil: an application of a seasonal catch-at-age model. Marine and Freshwater Research 53, 835–847.
| Effects of exploitation by two co-existing fleets on the bluefish, Pomatomus saltatrix, in southern Brazil: an application of a seasonal catch-at-age model.Crossref | GoogleScholarGoogle Scholar |
Maggs, JQ, Mann, BQ, and van der Elst, RP (2012). Long-term trends in the recreational shore-fishery for elf Pomatomus saltatrix (Pomatomidae) along the KwaZulu–Natal coast, South Africa. African Journal of Marine Science 34, 401–410.
| Long-term trends in the recreational shore-fishery for elf Pomatomus saltatrix (Pomatomidae) along the KwaZulu–Natal coast, South Africa.Crossref | GoogleScholarGoogle Scholar |
Mann BQ (2000) ‘Southern African marine linefish status reports.’ (Oceanographic Research Institute: Durban, South Africa)
McBride, RS (2015). Diagnosis of paired age agreement: a simulation of accuracy and precision effects. ICES Journal of Marine Science 72, 2149–2167.
| Diagnosis of paired age agreement: a simulation of accuracy and precision effects.Crossref | GoogleScholarGoogle Scholar |
McNemar, Q (1947). Note on the sampling error of the difference between correlated proportions or percentages. Psychometrika 12, 153–157.
| Note on the sampling error of the difference between correlated proportions or percentages.Crossref | GoogleScholarGoogle Scholar |
Miralles, L, Juanes, F, Pardiñas, AF, and Garcia-Vazquez, E (2014). Paleoclimate shaped bluefish structure in the northern hemisphere. Fisheries 39, 578–586.
| Paleoclimate shaped bluefish structure in the northern hemisphere.Crossref | GoogleScholarGoogle Scholar |
Morrongiello, JR, and Thresher, RE (2015). A statistical framework to explore ontogenetic growth variation among individuals and populations: a marine fish example. Ecological Monographs 85, 93–115.
| A statistical framework to explore ontogenetic growth variation among individuals and populations: a marine fish example.Crossref | GoogleScholarGoogle Scholar |
Murphy JJ, Ochwada-Doyle FA, West LD, Stark KE, Hughes JM (2020) Survey of recreational fishing in NSW, 2017/18. Fisheries Final Report Series Number 158, NSW Department of Primary Industries Fisheries, Port Stephens, NSW, Australia.
Nicolle, P, Hughes, J, Fowler, A, and Schilling, HT (2022). Long-term increase in growth of an estuarine predator, mulloway Argyrosomus japonicus, predicted to continue under future warming scenarios. Marine Ecology Progress Series 688, 1–17.
| Long-term increase in growth of an estuarine predator, mulloway Argyrosomus japonicus, predicted to continue under future warming scenarios.Crossref | GoogleScholarGoogle Scholar |
Nieto A, Ralph GM, Comeros-Raynal MT, Kemp J, García Criado M, Allen DJ, Dulvy NK, Walls RHL, Russell B, Pollard D, García S, Craig M, Collette BB, Pollom R, Biscoito M, Labbish Chao N, Abella A, Afonso P, Álvarez H, Carpenter KE, Clò S, Cook R, Costa MJ, Delgado J, Dureuil M, Ellis JR, Farrell ED, Fernandes P, Florin A-B, Fordham S, Fowler S, Gil de Sola L, Gil Herrera J, Goodpaster A, Harvey M, Heessen H, Herler J, Jung A, Karmovskaya E, Keskin C, Knudsen SW, Kobyliansky S, Kovačić M, Lawson JM, Lorance P, McCully Phillips S, Munroe T, Nedreaas K, Nielsen J, Papaconstantinou C, Polidoro B, Pollock CM, Rijnsdorp AD, Sayer C, Scott J, Serena F, Smith-Vaniz WF, Soldo A, Stump E, Williams JT (2015) ‘European red list of marine fishes.’ (Publications Office of the European Union: Luxembourg)
Northeast Fisheries Science Center (1997) A report of the 23rd Northeast Regional Stock Assessment Workshop. 23rd northeast regional stock assessment workshop (23rdSAW): public review workshop. (Northeast Fisheries Science Center: Woods Hole, MA, USA) Available at https://repository.library.noaa.gov/view/noaa/5187
Northeast Fisheries Science Center (2015) 60th northeast regional stock assessment workshop (60th SAW) assessment report. (Northeast Fisheries Science Center: Woods Hole, MA, USA) Available at https://repository.library.noaa.gov/view/noaa/4975
NSW SPCC (1981) ‘The ecology of fish in Botany Bay: biology of commercially and recreationally valuable species.’ (New South Wales State Pollution Control Commission)
Nurthen, RK, Cameron, R, and Briscoe, DA (1992). Population genetics of tailor, Pomatomus saltatrix (Linnaeus) (Pisces: Pomatomidae) in Australia. Marine and Freshwater Research 43, 1481–1486.
| Population genetics of tailor, Pomatomus saltatrix (Linnaeus) (Pisces: Pomatomidae) in Australia.Crossref | GoogleScholarGoogle Scholar |
Nyman, RM, and Conover, DO (1988). The relation between spawning season and the recruitment of young-of-the-year bluefish, Pomatomus saltatrix, to New York. Fishery Bulletin 86, 237–250.
Pollock, BR (1984). The tailor (Pomatomus saltatrix) fishery at Fraser Island and its relation to the life-history of the fish. Proceedings of the Royal Society of Queensland 95, 23–28.
Potts, WM, Bealey, RSJ, and Childs, A-R (2016). Assessing trophic adaptability is critical for understanding the response of predatory fishes to climate change: a case study of Pomatomus saltatrix in a global hotspot. African Journal of Marine Science 38, 539–547.
| Assessing trophic adaptability is critical for understanding the response of predatory fishes to climate change: a case study of Pomatomus saltatrix in a global hotspot.Crossref | GoogleScholarGoogle Scholar |
Quince, C, Abrams, PA, Shuter, BJ, and Lester, NP (2008a). Biphasic growth in fish I: theoretical foundations. Journal of Theoretical Biology 254, 197–206.
| Biphasic growth in fish I: theoretical foundations.Crossref | GoogleScholarGoogle Scholar |
Quince, C, Shuter, BJ, Abrams, PA, and Lester, NP (2008b). Biphasic growth in fish II: empirical assessment. Journal of Theoretical Biology 254, 207–214.
| Biphasic growth in fish II: empirical assessment.Crossref | GoogleScholarGoogle Scholar |
Reygondeau, G, Longhurst, A, Martinez, E, Beaugrand, G, Antoine, D, and Maury, O (2013). Dynamic biogeochemical provinces in the global ocean. Global Biogeochemical Cycles 27, 1046–1058.
| Dynamic biogeochemical provinces in the global ocean.Crossref | GoogleScholarGoogle Scholar |
Robillard, E, Reiss, CS, and Jones, CM (2008). Reproductive biology of bluefish (Pomatomus saltatrix) along the East Coast of the United States. Fisheries Research 90, 198–208.
| Reproductive biology of bluefish (Pomatomus saltatrix) along the East Coast of the United States.Crossref | GoogleScholarGoogle Scholar |
Robillard, E, Reiss, CS, and Jones, CM (2009). Age-validation and growth of bluefish (Pomatomus saltatrix) along the East Coast of the United States. Fisheries Research 95, 65–75.
| Age-validation and growth of bluefish (Pomatomus saltatrix) along the East Coast of the United States.Crossref | GoogleScholarGoogle Scholar |
Sabatés, A, and Martin, P (1993). Spawning and distribution of bluefish Pomatomus saltatrix (L.) in the northwestern Mediterranean. Journal of Fish Biology 42, 109–118.
| Spawning and distribution of bluefish Pomatomus saltatrix (L.) in the northwestern Mediterranean.Crossref | GoogleScholarGoogle Scholar |
Sabatés, A, Martín, P, and Raya, V (2012). Changes in life-history traits in relation to climate change: bluefish (Pomatomus saltatrix) in the northwestern Mediterranean. ICES Journal of Marine Science 69, 1000–1009.
| Changes in life-history traits in relation to climate change: bluefish (Pomatomus saltatrix) in the northwestern Mediterranean.Crossref | GoogleScholarGoogle Scholar |
Schilling HT (2019) Ecology of tailor, Pomatomus saltatrix, in eastern Australia. PhD Thesis, University of New South Wales, Sydney, NSW, Australia.
Schilling, HT, Hughes, JM, Smith, JA, Everett, JD, Stewart, J, and Suthers, IM (2017). Latitudinal and ontogenetic variation in the diet of a pelagic mesopredator (Pomatomus saltatrix), assessed with a classification tree analysis. Marine Biology 164, 75.
| Latitudinal and ontogenetic variation in the diet of a pelagic mesopredator (Pomatomus saltatrix), assessed with a classification tree analysis.Crossref | GoogleScholarGoogle Scholar |
Schilling, HT, Reis-Santos, P, Hughes, JM, Smith, JA, Everett, JD, Stewart, J, Gillanders, BM, and Suthers, IM (2018). Evaluating estuarine nursery use and life history patterns of Pomatomus saltatrix in eastern Australia. Marine Ecology Progress Series 598, 187–199.
| Evaluating estuarine nursery use and life history patterns of Pomatomus saltatrix in eastern Australia.Crossref | GoogleScholarGoogle Scholar |
Schilling, HT, Smith, JA, Stewart, J, Everett, JD, Hughes, JM, and Suthers, IM (2019). Reduced exploitation is associated with an altered sex ratio and larger length at maturity in southwest Pacific (east Australian) Pomatomus saltatrix. Marine Environmental Research 147, 72–79.
| Reduced exploitation is associated with an altered sex ratio and larger length at maturity in southwest Pacific (east Australian) Pomatomus saltatrix.Crossref | GoogleScholarGoogle Scholar |
Schilling, HT, Everett, JD, Smith, JA, Stewart, J, Hughes, JM, Roughan, M, Kerry, C, and Suthers, IM (2020). Multiple spawning events promote increased larval dispersal of a predatory fish in a western boundary current. Fisheries Oceanography 29, 309–323.
| Multiple spawning events promote increased larval dispersal of a predatory fish in a western boundary current.Crossref | GoogleScholarGoogle Scholar |
Shepherd, GR, Moser, J, Deuel, D, and Carlsen, P (2006). The migration patterns of bluefish (Pomatomus saltatrix) along the Atlantic coast determined from tag recoveries. Fishery Bulletin 104, 559–571.
Silvano, RAM, and Begossi, A (2005). Local knowledge on a cosmopolitan fish: ethnoecology of Pomatomus saltatrix (Pomatomidae) in Brazil and Australia. Fisheries Research 71, 43–59.
| Local knowledge on a cosmopolitan fish: ethnoecology of Pomatomus saltatrix (Pomatomidae) in Brazil and Australia.Crossref | GoogleScholarGoogle Scholar |
Sipe, AM, and Chittenden, ME (2002). A comparison of calcified structures for aging bluefish in the Chesapeake bay region. Transactions of the American Fisheries Society 131, 783–790.
| A comparison of calcified structures for aging bluefish in the Chesapeake bay region.Crossref | GoogleScholarGoogle Scholar |
Smith, MW, Then, AY, Wor, C, Ralph, G, Pollock, KH, and Hoenig, JM (2012). Recommendations for catch-curve analysis. North American Journal of Fisheries Management 32, 956–967.
| Recommendations for catch-curve analysis.Crossref | GoogleScholarGoogle Scholar |
Smith K, Lewis P, Brown J, Dowling C, Howard A, Lenanton R, Molony B (2013) Status of nearshore finfish stocks in south-western Western Australia Part 2: Tailor. Fisheries Research Report Number 247, Department of Fisheries, Fisheries Research Division, WA, Australia.
Stewart, J, Ferrell, DJ, and van der Walt, B (2004). Sizes and ages in commercial landings with estimates of growth, mortality and yield per recruit of yellowtail kingfish (Seriola lalandi) from New South Wales, Australia. Marine and Freshwater Research 55, 489–497.
| Sizes and ages in commercial landings with estimates of growth, mortality and yield per recruit of yellowtail kingfish (Seriola lalandi) from New South Wales, Australia.Crossref | GoogleScholarGoogle Scholar |
Stewart, J, Hegarty, A-M, Young, C, and Fowler, AM (2018). Sex-specific differences in growth, mortality and migration support population resilience in the heavily exploited migratory marine teleost Mugil cephalus (Linnaeus 1758). Marine and Freshwater Research 69, 385–394.
| Sex-specific differences in growth, mortality and migration support population resilience in the heavily exploited migratory marine teleost Mugil cephalus (Linnaeus 1758).Crossref | GoogleScholarGoogle Scholar |
Stock, CA, John, JG, Rykaczewski, RR, Asch, RG, Cheung, WWL, Dunne, JP, Friedland, KD, Lam, VWY, Sarmiento, JL, and Watson, RA (2017). Reconciling fisheries catch and ocean productivity. Proceedings of the National Academy of Sciences 114, E1441–E1449.
| Reconciling fisheries catch and ocean productivity.Crossref | GoogleScholarGoogle Scholar |
Swain, DP, Sinclair, AF, and Mark Hanson, J (2007). Evolutionary response to size-selective mortality in an exploited fish population. Proceedings of the Royal Society of London – B. Biological Sciences 274, 1015–1022.
| Evolutionary response to size-selective mortality in an exploited fish population.Crossref | GoogleScholarGoogle Scholar |
Teixeira D, Janes R, Webley J (2021) 2019/20 statewide recreational fishing survey: key results. (Queensland Department of Agriculture and Fisheries: Brisbane, Qld, Australia) Available at https://era.daf.qld.gov.au/id/eprint/7879/1/SRFS2019_Key_highlights_report.pdf
van der Elst R (1976) Game fish of the east coast of southern Africa 1. The biology of the elf, Pomatomus saltatrix (Linnaeus), in the coastal waters of Natal. Investigational Report Number 44, Oceanographic Research Institute.
Webley J, McInnes K, Teixeira D, Lawson A, Quinn R (2015) Statewide recreational fishing survey 2013–14. Report number CS4725 10/15. (Department of Agriculture and Fisheries: Brisbane, Qld, Australia) Available at https://fish.gov.au/Archived-Reports/Documents/Crimson%20Snapper%20-%20Qld%20rec%20fishing%20survey%202013-14.pdf
Young, GC, Wise, BS, and Ayvazian, SG (1999). A tagging study on tailor (Pomatomus saltatrix) in Western Australian waters: their movement, exploitation, growth and mortality. Marine and Freshwater Research 50, 633–642.
| A tagging study on tailor (Pomatomus saltatrix) in Western Australian waters: their movement, exploitation, growth and mortality.Crossref | GoogleScholarGoogle Scholar |
Zeller, BM, Pollock, BR, and Williams, LE (1996). Aspects of life history and management of tailor (Pomatomus saltatrix) in Queensland. Marine and Freshwater Research 47, 323–329.
| Aspects of life history and management of tailor (Pomatomus saltatrix) in Queensland.Crossref | GoogleScholarGoogle Scholar |