Microplastic presence in dried and fresh fish from seafood markets in Sri Lanka
Nina Wootton

A
# Joint first authorship
Handling Editor: Haseeb Randhawa
Abstract
Microplastics have been found in aquatic ecosystems globally, including in many marine organisms. Despite this, understanding the occurrence of microplastics in seafood products prepared for human consumption has received less attention.
This study aims to determine the abundance and type of microplastic in dried and fresh fish purchased from seafood markets.
Four locally harvested species of anchovies and sardines were sampled from seafood markets across Sri Lanka. These pelagic species are popular seafood items and are commonly consumed whole. We analysed fresh (gastrointestinal tracts and whole body) and dried fish (whole body) under the microscope for microplastic presence (>38 μm, <5 mm), followed by polymer validation.
Across all fish sampled (N = 215), 82.3% had microplastic (3.64 ± 0.26 microplastics per individual), with fibre-shaped pieces making up more than 95% of all microplastic particles. Overall, contamination was higher in fresh fish than in whole dried fish. And, acrylic resin was the most common polymer.
Microplastics were found in nearly all fresh fish and almost half the dry fish from Sri Lanka, confirming the pervasive nature of microplastic contamination.
Differences in contamination levels in popular seafood products demonstrate how preparation and consumption habits can influence microplastic intake.
Keywords: contamination, fish, food source, marine debris, microplastic, plastic pollution, seafood, Sri Lanka.
Introduction
Plastic waste has found its way into every corner of our planet, with plastic recorded from the highest mountains (Napper et al. 2020) to the deepest oceanic trenches (Chiba et al. 2018). In marine systems, small plastics are now ubiquitous, with current estimates of surface-layer concentrations rising to more than 170 trillion particles (Eriksen et al. 2023). Because of their small size (<5 mm), the removal of microplastics from the environment is problematic, and in many cases near impossible (Worm et al. 2017). Microplastics have been identified in several marine biota species, including charismatic mammals (Nelms et al. 2019) and seabirds (Lavers et al. 2014), as well as a suite of fish (Daniel et al. 2020; Wootton et al. 2021a), bivalves (Bom and Sá 2021; Wootton et al. 2022) and crustacean species (Ogunola et al. 2022), many of which are harvested for human consumption.
Microplastic contamination in seafood species can occur via intentional or unintentional ingestion or inhalation. This can be via primary ingestion, where the animal consumes the microplastic directly (Ryan 2019), or secondary ingestion, where microplastic is consumed via a lower trophic-level organism (Nelms et al. 2018; Provencher et al. 2019; Ogunola et al. 2023). Once consumed, microplastic particles can transport persistent organic pollutants (POPs) and other toxic substances, leading to potential bioaccumulation in marine organisms and biomagnification through the food web (Rochman et al. 2013; Ziccardi et al. 2016). Furthermore, microplastics can cause physical harm to marine life, including ingestion blockages, impaired feeding and reduced fitness (Wright et al. 2013). The pervasive nature of microplastics also poses a risk to marine habitats, where they can smother organisms and alter structures, leading to declines in species richness and ecosystem resilience (Hall et al. 2015). Addressing microplastic pollution is thus critical not only for safeguarding seafood safety but also for protecting marine ecosystems and the services they provide.
Plastic particles can also be incorporated into seafood through any one of the many processing steps that occur from capture to consumption (Catarino et al. 2018). For instance, contamination of microplastics can stem from fishing equipment (e.g. trawling nets and lines) or from the facilities and practices used to process or dry seafood (Hasan et al. 2022, 2023; Piyawardhana et al. 2022) or feed seafood in aquaculture (Gomiero et al. 2020; Wootton et al. 2022). Preparation procedures and consumption patterns may also increase or decrease microplastic contamination in seafood, and understanding this risk is critically important to evaluate the potential exposure to microplastics associated with seafood consumption (Catarino et al. 2018; Dawson et al. 2021). Yet, reports of microplastics in gastrointestinal tracts alone generally shed little light on the potential intake of microplastics by humans because these tissues are often discarded before consumption. Thus, there is a need to examine contamination at point of sale, as well as in processed products, and in particular seafood that is consumed whole, to understand the relevance of seafood consumption as a potential source of microplastics (Dawson et al. 2021, 2022; Hasan et al. 2022, 2023).
In this study, we aim to quantify the microplastic content in dried and fresh small pelagics sold for human consumption in Sri Lanka. In the Sri Lankan diet, it is common to consume fish in their entirety, particularly if the product is small and prepared appropriately (e.g. dried, fried). By comparing contamination in fresh (gastrointestinal tracts and whole body) and dried (whole body) fish, we provide information on microplastic ingestion that can be compared with the growing global database on microplastic contamination. In turn, this sheds light on the importance of understanding differences in contamination in popular seafood products, and how preparation and consumption habits may influence human consumption of microplastics.
Methods
Study area
This study was conducted in Sri Lanka, an island nation in southern Asia (Fig. 1), with a population of ~21 million. The widespread consumption of whole fish in the local diet makes Sri Lanka an ideal location to assess human exposure to microplastics through seafood. Sampling sites were selected across different regions to capture a representative overview of microplastic contamination in Sri Lankan seafood.
Sample collection
In total, 215 fish, from four species of clupeiforms (anchovies and sardines), were collected from major fish markets associated with fishing ports in Sri Lanka (Table 1). The species selected are widely consumed and often eaten whole. Three species of fresh fish (Amblygaster sirm, Sardinella gibbosa and Stolephorous commersonnii) were collected from Negombo, Trincomalee and Kudawella (except S. commersonnii, which was unavailable in Kudawella), over a 10-day period in December 2019 (Table 2, Fig. 1). Three species of dried fish (A. sirm, Sardinella albella, S. commersonnii) were purchased from a visit to the Dikwella fish market (near Kudawella) in December 2020 (Fig. 1). Because of logistical constraints and availability, only one location was used to collect the dried fish samples. Two of the species (S. commersonnii and A. sirm) were the same for both dried and fresh fish samples. For Sardinella, we collected S. gibbosa (fresh) and S. albella (dried) because of market availability, but both species have similar feeding strategies and ecological niches, and so, from here on, are discussed as Sardinella spp. In Sri Lanka, fish are commonly dried under the sun in open air, usually on woven matts made of natural materials on or plastic sheeting.
Location | Sample collected | GPS coordinates | Approximate population size | Description of potential pollution sources | |
---|---|---|---|---|---|
Negombo | Fresh | 7.2028°N, 79.8287°E | ~142,000 | Tourism, urban, fishing industry | |
Trincomalee | Fresh | 8.5779°N, 81.2350°E | ~380,000 | Port activities, tourism, military | |
Kudawella | Fresh | 5.9775°N, 80.7344°E | ~10,000 | Fisheries | |
Dikwella | Dried | 5.9651°N, 80.6933°E | ~10,000 | Local agriculture, small-scale fishing |
Species | Preparation | Tissue analysed | N | Fork length (mm) | Total weight (g) | Digested weight (g) | |
---|---|---|---|---|---|---|---|
Amblygaster sim | Dried | Whole | 20 | 92.45 (±2.47) | 7.47 (±0.44) | 7.47 (±0.44) | |
Fresh | GI tract | 55 | 155.42 (±3.28) | 31.36 (±0.07) | 1.87 (±1.99) | ||
Sardinella albella | Dried | Whole | 20 | 113.05 (±2.11) | 9.33 (±0.47) | 9.33 (±0.47) | |
Sardinella gibbosa | Fresh | GI tract | 60 | 152.08 (±16.63) | 27.23 (±0.09) | 1.21 (±2.05) | |
Stolephorous commersonnii | Dried | Whole | 20 | 47.2 (±1.56) | 0.80 (±0.04) | 0.80 (±0.04) | |
Fresh | Whole | 40 | 58.14 (±1.20) | 1.68 (±0.08) | 1.68 (±0.08) |
Data are means (±s.e.). N, total sample size.
The fish used as part of this study were procured dead from commercial seafood markets and as such did not require institutional Animal Ethics Committee Approval.
Following purchase, fresh fish samples were preserved in 10% formalin for air transportation, as per the Australian Government requirements. Dried fish samples were declared and transported in sealed packaging. On arrival in the laboratory at the University of Adelaide, Australia, all fish were washed thoroughly with ultrapure (Milli-Q) water to remove any remaining preservative, and potential external contamination. Fork length and total weight were measured in ~20 individuals per species and site (Table 1).
Microplastic extraction
Fresh A. sirm and S. gibbosa individuals were dissected, and gastrointestinal tracts were removed, placed in glass beakers and weighed. This approach was selected, because individuals from these two species were larger than 120 mm, a size from which they are generally eviscerated and cleaned before being consumed. All dried fish (A. sirm, S. Albella, S. commersonnii) and fresh S. commersonnii were smaller than 120 mm, and were processed whole, because these small, dried fish are not eviscerated and are generally consumed whole. Dried fish were placed in their entirety into separate glass beakers and weighed.
Samples of gastrointestinal tracts and whole fish were covered in 10% potassium hydroxide (KOH) solution with ultrapure water, covered with foil, and left overnight at 40°C for digestion (Gulizia et al. 2022; Bornt et al. 2024; Di Fiore et al. 2024; Wootton et al. 2024). Following digestion, the liquified samples were sieved through two nested metal sieves with mesh sizes of 1 mm and 38 μm, to separate solid materials, including microplastics. The minimum-size threshold for the collection of microplastics was 38 μm. Ultrapure water was used to flush the beakers. Sieves were individually examined under a stereomicroscope (Leica M80). Particles suspected of being microplastic were recorded on a spreadsheet and collected for future plastic confirmation and polymer-composition analysis. Microplastic colour, type (fibres, fragments or film) and sieve size were recorded, following the methods of Hidalgo-Ruz et al. (2012).
Quality assurance and quality control
Quality-assurance and quality-control protocols were implemented to minimise the risk of contamination throughout collection and laboratory processesing. At time of purchase, fresh fish were collected and placed in clean glass containers. Dried fish were purchased in bulk and kept in the original plastic bag in which they were sold. All glass, plastic-ware and dissecting utensils were rinsed three times with ultrapure water and dried in a laminar flow prior to use. Samples were covered with aluminium foil whenever outside the laminar flow (e.g. drying oven). Researchers wore natural-fibre clothing covered by bright pink cotton–polyester laboratory coats. If any pink fibres were identified during analysis, they were excluded because they were categorised as contamination, although no pink fibres were found. Both airborne and procedural control measures were implemented. This involved open vials filled with ultrapure water during dissections and microscope analysis, and glass flasks filled with 10% KOH and treated the same way as the digested samples. All controls were analysed the same way as fish samples, filtering through metal sieves and analysed under the microscope. No contamination was found in any of the controls or blanks, so no correction of the data was required.
Spectroscopic analysis
In total, 80 microplastic particles were analysed to validate visual plastic identification and identify polymer types using micro-Fourier transform infrared spectroscopy (μFTIR) (Bruker Hyperion). Particles were randomly selected from the identified microplastics, prioritising those larger than 300 μm to ensure compatibility with the technical requirements of the μFTIR. This size-based criterion was chosen to maximise the accuracy and reliability of polymer-type identification, as smaller particles may yield less precise spectral data. The selection process was designed to provide a representative subset across samples while adhering to analytical limitations. Specifications were set up following Wootton et al. (2021b), with attenuated total reflection (ATR) applied to the microplastics sampled at 4-cm−1 resolution. A single measurement position was tested, with 64 co-scans and spectrum of 3900–650 cm−1. The region between 2500 and 1900 cm−1 was excluded from spectral analysis because this was defined as the CO2–water region. Spectral outputs were compared with the Bruker ATR Library for Chemicals, Pharma and Forensics, with a match of >70% required.
Data analysis
Plastic load was calculated as the average number of microplastics per individual (MPs individual−1), and per gram of weight for the digested portion of the sample (MPs g−1). For dried fish the dry weight was recorded, and for fresh fish the wet weight was recorded. Average plastic load includes all individuals sampled, whether they had microplastic present or not. The percentage of fish with at least one piece of plastic was quantified for each species, i.e. frequency of occurrence of plastic ingestion (FO). The Shapiro–Wilk test was used to assess the assumption of normality for our data. Because the data exhibited non-normal distributions, we employed non-parametric statistical tests, specifically the Kruskal–Wallis and Dunn’s tests, to evaluate differences among locations. Graphical outputs and statistical analyses were completed in RStudio (ver. 2022.12.0, Posit Software, PBC, Boston, MA, USA, see https://posit.co/products/open-source/rstudio/) using ggplot2 (ver. 3.5.1, see https://CRAN.R-project.org/package=ggplot2; Wickham 2016), dplyr (ver. 1.0.10, see https://CRAN.R-project.org/package=dplyr), glmm (ver. 1.4.4, C. Knudson, see https://cran.r-project.org/package=glmm), MASS (ver. 7.3.58.1, see https://CRAN.R-project.org/package=MASS; Venables and Ripley 2002) and tidyverse (ver. 1.3.2, see https://cran.r-project.org/package=tidyverse; Wickham et al. 2019).
Results
Frequency of microplastic occurrence
In total, 783 microplastic pieces were collected, with 0 to 23 particles found per gastrointestinal tract or whole fish. Of all collected microplastic, 413 pieces were larger than 1 mm in size, whereas 370 were between 38 μm and 1 mm. Overall, 82.8% of all samples had microplastic present, although the frequency of occurrence varied among species and preparation. Overall, fresh fish samples had double the frequency of occurrence of microplastic compared with dried fish (96.1 and 48.3% respectively). Across the three species, fresh fish guts or whole individuals (A. sirm, Sardinella spp. and S. commersonnii respectively) had higher frequencies of occurrence than did dried fish (Fig. 2). In particular, whole fresh S. commersonnii and gut samples of Sardinella spp. had the highest frequency of occurrence, with both having 97.5% of fish with at least one piece of microplastic present. Conversely, dried whole S. commersonnii had the lowest frequency of occurrence, with only 20% of individuals containing plastic particles.
Frequency of occurrence (%) of fish species that had microplastics present, for different locations. Dried fish are represented in the dark blue bars, and fresh fish are shown in the light blue bars. Fish that were sampled whole are represented in solid colours, and those where only the gastrointestinal (GI) tract was sampled are in the striped.
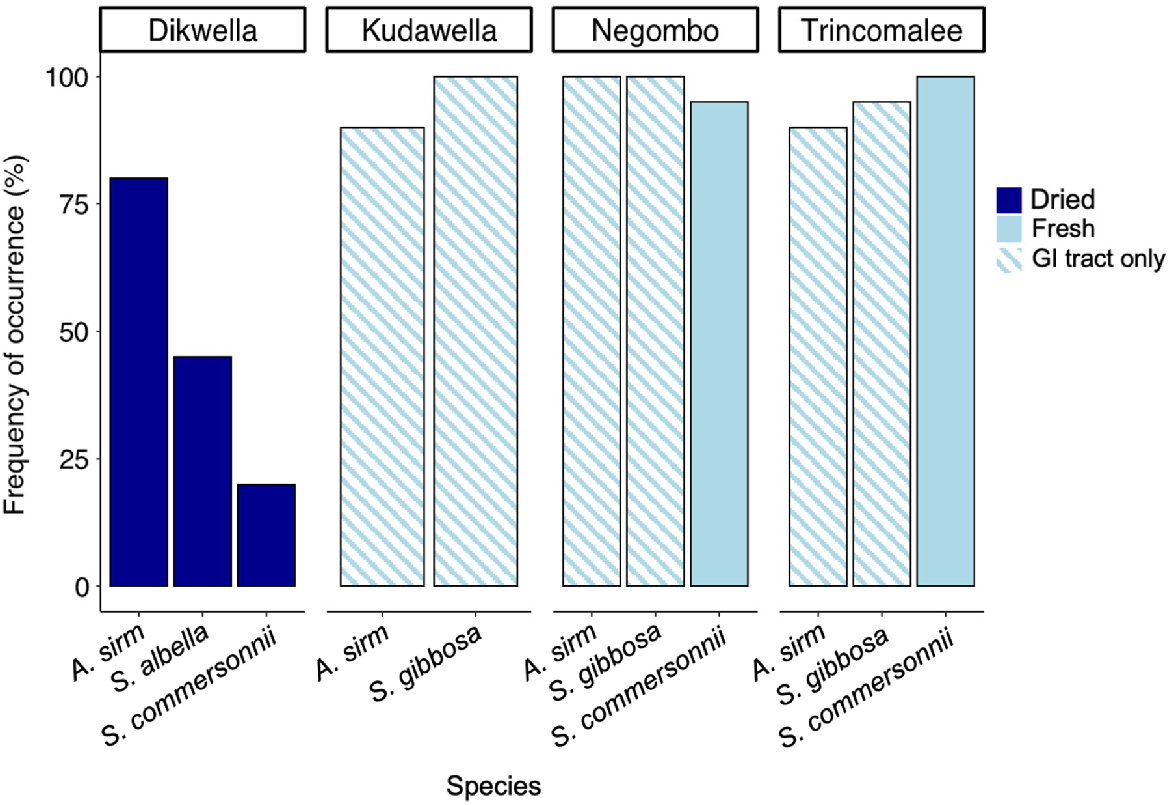
Microplastic load
Overall, plastic load was 3.64 ± 0.26 MPs individual−1 and 2.73 ± 0.22 MPs g−1, although loads varied among species and preparation. The plastic load was significantly (P < 0.001) higher in fresh fish samples than in dried fish, when both microplastics per individual (4.68 ± 0.31 and 0.96 ± 0.17 MPs individual−1 for fresh and dried fish respectively), and microplastics per weight were considered (3.70 ± 0.26 and 0.24 ± 0.07 MPs g−1 for fresh and dried fish respectively) (Fig. 3). The locations where the fish were caught also affected the microplastic load per individual and per weight (P < 0.001), and differences were also seen among species (P < 0.001) (Fig. 3b).
(a) Plastic load of microplastics (MPs) per gram of digested fish and (b) plastic load of microplastics per individual fish. Dried fish are represented in the dark blue bars, and fresh fish are shown in the light blue bars. Fish that were sampled whole are represented in solid colours, whereas those where only the gastrointestinal (GI) tract was sampled are striped. Error bars show the standard error.
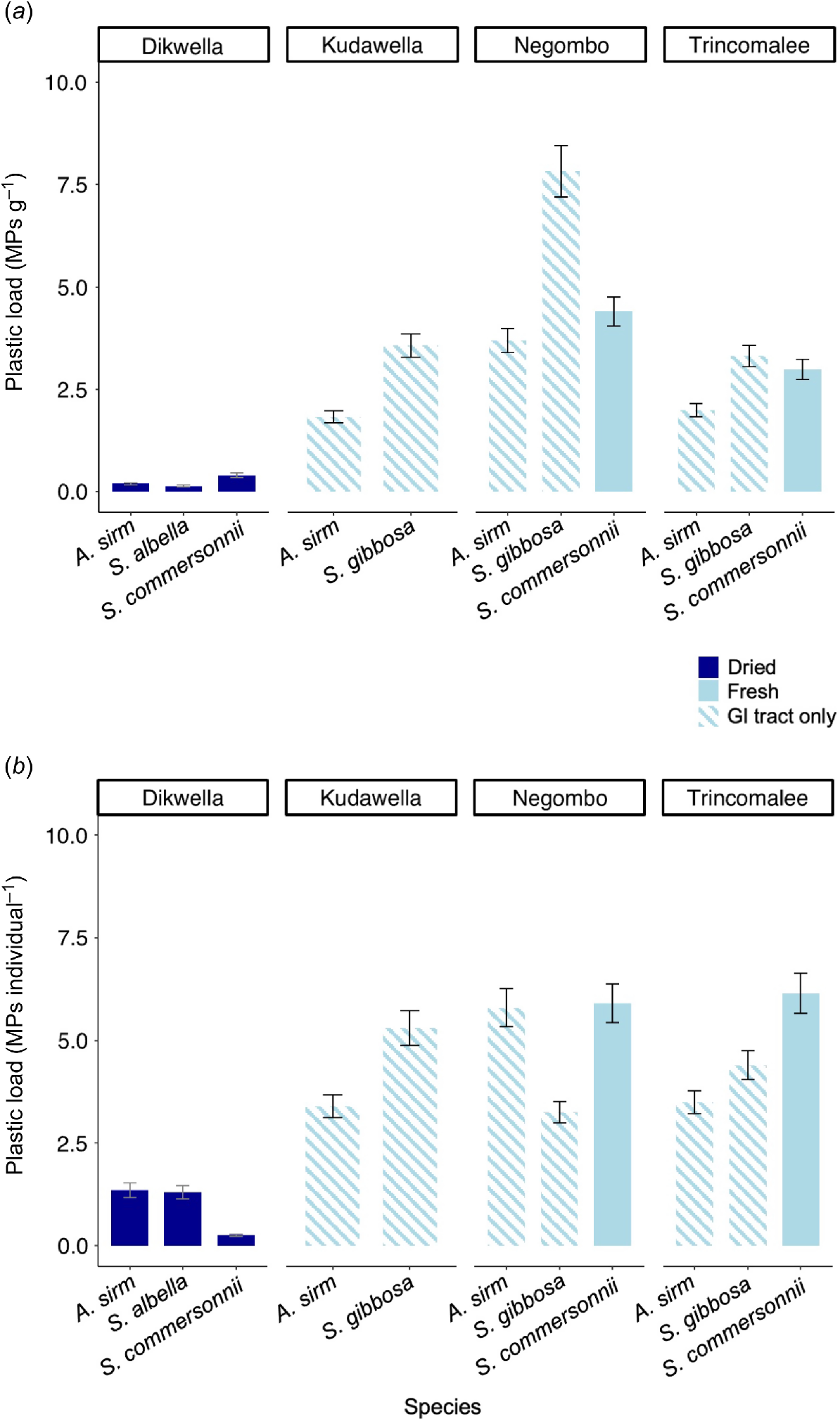
Plastic and polymer type
When investigated under the microscope, plastic type was dominated by fibres in both fresh and dried samples, with 95.9 and 82.8% respectively (Fig. 4). In dried fish, 15.5% of the remaining particles were identified as film, whereas no film particles were found in fresh samples. The μFTIR was used to confirm our visual inspection of plastic pieces, with 96% of the pieces (N = 77) tested being identified as plastic and 100% being confirmed as anthropogenic debris (the three non-plastic pieces were plaster and pigment). Overall, we identified 15 different polymer types (Fig. 5). The dominant types of polymer were acrylic resin, followed by semi-synthetic fibres and polyethylene (Fig. 5).
Polymer types identified in (a) dried and (b) fresh fish samples using the μFTIR. The grey section (other) in the dried fish samples refers to polymers where two or fewer pieces were categorised as a particular type. This includes paint, polyamide, polyaniline, polyester, undefined polymer alloys, polystyrene and polyurethane. Semi-synthetic fibres included several mixed synthetic and naturally occurring fibres (e.g. cotton and lycra, cotton and elastane).
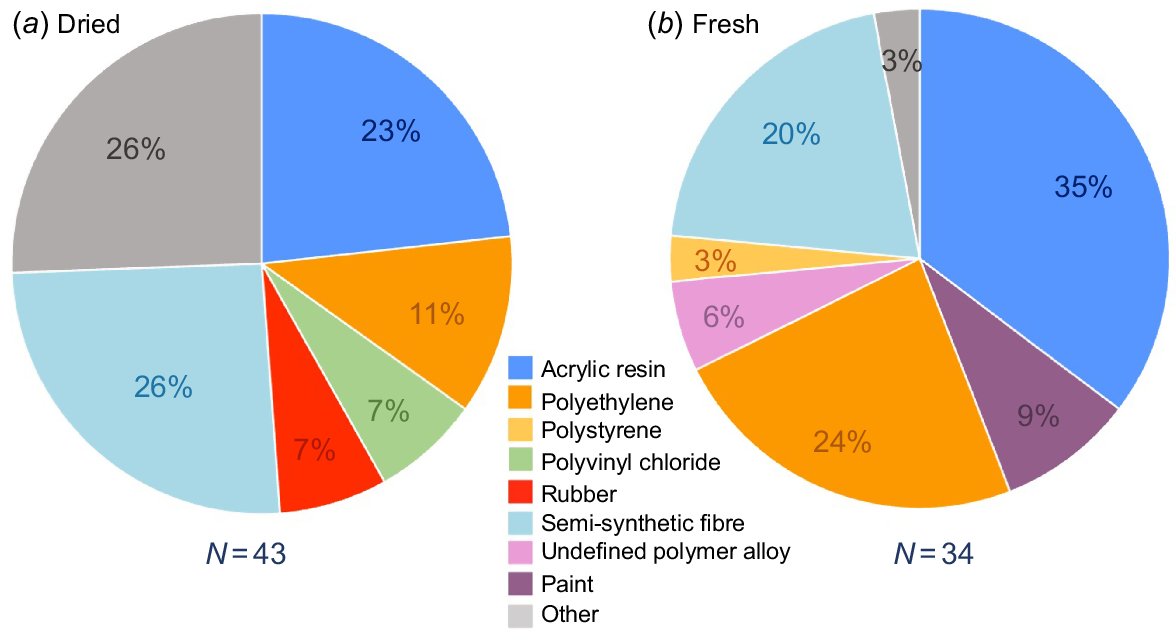
Discussion
Following global trends, this study has shown that small pelagics, anchovies and sardines sold for consumption through seafood markets in Sri Lanka were contaminated with microplastics, with samples from fresh fish showing higher contamination than those from dried fish. There were clear trends confirming that fish were ingesting microplastics, with the gastrointestinal tracts of fresh fish having frequency occurrences of >96%. Although method variability may limit some comparison, these occurrence frequencies were higher than the global average of studies that have followed similar procedures (Wootton et al. 2021a, 2021b). All four species sampled are important species for Sri Lankan fisheries and widely consumed across the island. All samples were collected before the COVID-19 pandemic, but also before the 2021 M/V X-Press Pearl ship nurdle spill disaster, both of which are recognised to have caused significant increases in plastic pollution in the marine environments along the Sri Lankan coast (Peng et al. 2021; de Vos et al. 2022). Overall, these results have provided a valuable baseline dataset on microplastics in pelagic fish in Sri Lanka, that can be used to provide insights on contamination over time, support present and future monitoring thresholds, and how these events may have affected contamination.
The presence of plastic pollution in Sri Lankan coastal environments is well documented, with studies reporting concentrations up to 102–111 MPs m−2 in sediment and 0.28–0.52 MPs m−3 in water (Bimali Koongolla et al. 2018; Sevwandi Dharmadasa et al. 2021; Sewwandi et al. 2022). Our findings further demonstrated that microplastics are not confined to the abiotic environment of Sri Lanka, but have also infiltrated the biota, indicating a broader ecological impact. Interest in microplastic contamination in Sri Lankan coastal environments has been intensifying, particularly following a significant nurdle spill from a shipping incident in 2021, which released substantial quantities of plastic pellets into coastal waters (de Vos et al. 2022). This incident has underscored the urgency of understanding plastic pollution, and the potential vulnerability of marine ecosystems.
Dried fish were smaller than fresh fish and this may explain some of the differences found in microplastic loads between preparation types (e.g. owing to size and number of microplastics ingested below our limit of detection of <38 μm). But recent studies investigating microplastic presence in dried fish species throughout Asia, including a clupeiform from Sri Lanka (Spratelloides delicatulus), have found similar low microplastic loads (Karami et al. 2017; Piyawardhana et al. 2022; Table 3). Others have suggested that dried fish may have higher microplastic contamination levels than do fresh fish (e.g. Hasan et al. 2022, 2023), although these studies analysed pooled minced fish and did not rinse samples beforehand (as was done here, and by others, e.g. Karami et al. 2017; Piyawardhana et al. 2022; Table 3). This difference in methodology may play a key role, because external contamination and microplastics on the body surface are likely to occur. When comparing microplastics in fish tissues, cleaning procedures (e.g. rinsing) are an essential component of the quality assurance and quality control to reduce bias from environmental contamination. For human-ingestion purposes, surface- and skin-attached microplastics are an important aspect to consider, particularly if dried fish are consumed directly from packet or source to plate (e.g. not washed or pre-soaked). This is particularly important, considering microplastics may accumulate during the drying process (commonly, under the sun in open air, on woven matts made of natural materials, or plastic sheet). Ultimately, these differences further underpin the need for added focus on preparation and consumption habits to accurately estimate human ingestion of microplastics (Karami et al. 2017; Catarino et al. 2018; Dawson et al. 2021).
Study | Location(s) | Fish species | Preparation | Microplastic load (MPs g−1) | Main microplastic type observed | |
---|---|---|---|---|---|---|
Karami et al. (2017) | Malaysia | Chelon subvirdis Johnius belangerii Rastrelliger kanagurta Stolephorus waitei | Dried | NR | Fragment | |
Piyawardhana et al. (2022) | China, Japan, South Korea, Sri Lanka, Taiwan, Thailand, Vietnam | Spratelloides spp. Spratelloides gracilis Stolephorus spp. Etrumeus micropus Encrasicholina heteroloba Ammodytes personatus Spratelloides delicatulus | Dried | 0.0–0.56 | Fibres | |
Hasan et al. (2023) | Bangladesh | Harpadon nehereus Trichiurus lepturus | Dried | 2.17–46.00 | Fibres | |
Hasan et al. (2022) | Bangladesh | Harpadon nehereus Trichiurus lepturus | Dried | 28.54–46.00 | Fibres | |
Rukmangada et al. (2023) | India | Rastrelliger kanagurta Arius maculatus Oligoplites saurus Megalapsis cordyla Thunnus obesus Carcharhinus sorrah Chirocentrus dorab Priacanthus hamrur Harpadon neherus Trichiurus lepturus Micropogonias undulates Epinephelus sexfasciatus Cyanoglossus senegalensis Coilia dussumieri Pampus argenteus Lactarius lactarius Anguilla bengalensis Psettodes erumei Leiognathus equulus Thryssa purava Stolephorus indicus | Dried | 44.54 | Fragments | |
This study | Amblygaster sirm Sardinella albella Stolephorous commersonnii | Dried | 0.24 | Fibres |
The range of microplastic load (MPs g−1) is recorded. NR, not recorded.
With respect to plastic type, the high quantities of fibres (82.3% of all plastic types) were similar to those in other studies sampling dried fish across Asia (e.g. Piyawardhana et al. (2022) found ~80% of pieces were fibres), and mirror results globally where fibrous plastic pieces commonly dominate (Ling et al. 2017; Ugwu et al. 2021; Wootton et al. 2021b). Microplastic fibres can originate from a range of sources, including the shedding of synthetic textiles during washing, and derivatives of fishing equipment. Regarding polymer type, a large quantity of acrylic resin was identified, particularly in fresh fish, with over one-third of the microplastics (35%) tested being verified under this category. These acrylic resin fibres and semi-synthetic fibres could be linked to synthetic fishing gear and textiles. Acrylic resin can be used as a standalone material in a range of industrial processes (e.g. paints, adhesives) and synthetic textiles as an additive to improve strength. In the context of a major spill of nurdles to the eastern coast of Sri Lanka from the M/V X-Press Pearl ship disaster, it would be of interest to investigate how the amount and type of plastic in biota may have changed following the spill disaster, as well as to evaluate potential impacts. In particular, in the Negombo region, closest to the nurdle spill, where sediment samples collected following the spill are showing increased contamination levels of nurdles (Sewwandi et al. 2022).
Seafood is a critical source of nutrition and global food security, including throughout the Asia–Pacific region, where fish and other seafood species provide major protein supplies for local populations. In Sri Lanka specifically, an average of 15.3 kg of fish and fish products are consumed annually per person (2010 data) (Needham and Funge-Smith 2015). Globally, it is common for seafood products to undergo treatment processes (e.g. drying, processing, pickling) prior to sale and consumption, to assist with preservation and enhance the longevity of the produce. In Sri Lanka, processed fish are particularly popular, with nearly 30% of all fish undergoing drying or processing prior to consumption, with dried sprats (commonly S. commersonnii) being the most consumed seafood product (Needham and Funge-Smith 2015). Further, distinguishing where in the supply chain (e.g. collection, processing, transport, sales or cooking and preparation) the microplastics could be contaminating the seafood samples is an important next step, to evaluate potential precautionary avenues to mitigate exposure to consumers (Catarino et al. 2018).
Consuming seafood contaminated with microplastics poses potential risks to human health, as microplastics can also carry hazardous chemicals that may transfer to consumers (Smith et al. 2018). Microplastics can adsorb pollutants, including heavy metals, pesticides and persistent organic pollutants, from the surrounding environment, effectively acting as vectors for these toxic substances (Rochman et al. 2014; Tumwesigye et al. 2023). The small size of microplastics enables them to penetrate bodily tissues (e.g. Yang et al. 2023; Hu et al. 2024) or even enter the bloodstream (e.g. Leslie et al. 2022), potentially leading to long-term health effects (Li et al. 2023). Recent studies have also indicated that some microplastic particles may break down further within the digestive system (Li et al. 2024; Prabhu et al. 2024), releasing nanoplastics that could cross cell barriers, although the exact pathways and health impacts require further research. Given the importance of seafood in many diets worldwide, the risk of cumulative exposure to these contaminants through frequent consumption is an emerging area of concern (Smith et al. 2018). This highlights the need for continued research on the potential health effects of microplastic ingestion, and underscores the importance of precautionary approaches and addressing plastic pollution at its source to safeguard both marine ecosystems and human health.
Ultimately, this study has highlighted microplastic contamination in fish from Sri Lanka, a region where microplastic presence in biota is understudied. By analysing how seafood preparation may affect microplastic contamination, it has also provided insight into potential variations in microplastic intake from seafood consumption. Importantly, our data precede a recent shipping accident and nurdle spill and provide a baseline moving forward to analyse how microplastic contamination may potentially change over time. Overall, there are still gaps in our understanding of the impacts of microplastics on fish health (Ory et al. 2018), and, importantly, potential human health impacts (Walkinshaw et al. 2020; Vethaak and Legler 2021). However, considering the ample presence of microplastics in biota, identifying precautionary measures to mitigate environmental contamination and reduce potential presence in seafood are important avenues moving forward.
Acknowledgements
The authors acknowledge the Marine Biological Resources Division of the National Aquatic Resources Research and Development Agency (NARA), Sri Lanka, for supporting the preparation of fresh samples used in this study. We further extend our appreciation to Dr Prabath Jayasinghe of NARA for the guidance given.
References
Bimali Koongolla J, Andrady AL, Terney Pradeep Kumara PB, Gangabadage CS (2018) Evidence of microplastics pollution in coastal beaches and waters in southern Sri Lanka. Marine Pollution Bulletin 137, 277-284.
| Crossref | Google Scholar | PubMed |
Bom FC, Sá F (2021) Concentration of microplastics in bivalves of the environment: a systematic review. Environmental Monitoring and Assessment 193(12), 846.
| Crossref | Google Scholar |
Bornt K, Linge K, How J, de Lestang S, Hovey R, Langlois T (2024) Microplastic extraction from digestive tracts of large decapods. Marine Pollution Bulletin 206, 116709.
| Crossref | Google Scholar |
Catarino AI, Macchia V, Sanderson WG, Thompson RC, Henry TB (2018) Low levels of microplastics (MP) in wild mussels indicate that MP ingestion by humans is minimal compared to exposure via household fibres fallout during a meal. Environmental Pollution 237, 675-684.
| Crossref | Google Scholar | PubMed |
Chiba S, Saito H, Fletcher R, Yogi T, Kayo M, Miyagi S, Ogido M, Fujikura K (2018) Human footprint in the abyss: 30 year records of deep-sea plastic debris. Marine Policy 96, 204-212.
| Crossref | Google Scholar |
Daniel DB, Ashraf PM, Thomas SN (2020) Microplastics in the edible and inedible tissues of pelagic fishes sold for human consumption in Kerala, India. Environmental Pollution 266(Part 2), 115365.
| Crossref | Google Scholar |
Dawson AL, Santana MFM, Miller ME, Kroon FJ (2021) Relevance and reliability of evidence for microplastic contamination in seafood: a critical review using Australian consumption patterns as a case study. Environmental Pollution 276, 116684.
| Crossref | Google Scholar |
Dawson AL, Li JYQ, Kroon FJ (2022) Plastics for dinner: store-bought seafood, but not wild-caught from the Great Barrier Reef, as a source of microplastics to human consumers. Environmental Advances 8, 100249.
| Crossref | Google Scholar |
de Vos A, Aluwihare L, Youngs S, DiBenedetto MH, Ward CP, Michel APM, Colson BC, Mazzotta MG, Walsh AN, Nelson RK, Reddy CM, James BD (2022) The M/VX-Press Pearl nurdle spill: contamination of burnt plastic and unburnt nurdles along Sri Lanka’s beaches. ACS Environmental Au 2(2), 128-135.
| Crossref | Google Scholar | PubMed |
Di Fiore C, Ishikawa Y, Wright SL (2024) A review on methods for extracting and quantifying microplastic in biological tissues. Journal of Hazardous Materials 464, 132991.
| Crossref | Google Scholar |
Eriksen M, Cowger W, Erdle LM, Coffin S, Villarrubia-Gómez P, Moore CJ, Carpenter EJ, Day RH, Thiel M, Wilcox C (2023) A growing plastic smog, now estimated to be over 170 trillion plastic particles afloat in the world’s oceans – urgent solutions required. PLoS ONE 18(3), e0281596.
| Crossref | Google Scholar |
Gulizia AM, Brodie E, Daumuller R, Bloom SB, Corbett T, Santana MMF, Motti CA, Vamvounis G (2022) Evaluating the effect of chemical digestion treatments on polystyrene microplastics: recommended updates to chemical digestion protocols. Macromolecular Chemistry and Physics 223(13), 2100485.
| Crossref | Google Scholar |
Hall NM, Berry KLE, Rintoul L, Hoogenboom MO (2015) Microplastic ingestion by scleractinian corals. Marine Biology 162, 725-732.
| Crossref | Google Scholar |
Hasan J, Islam SMM, Alam MS, Johnson D, Belton B, Hossain MAR, Shahjahan M (2022) Presence of microplastics in two common dried marine fish species from Bangladesh. Marine Pollution Bulletin 176, 113430.
| Crossref | Google Scholar |
Hasan J, Dristy EY, Anjumanara , Mondal P, Hoque MS, Sumon KA, Hossain MAR, Shahjahan M (2023) Dried fish more prone to microplastics contamination over fresh fish – higher potential of trophic transfer to human body. Ecotoxicology and Environmental Safety 250, 114510.
| Crossref | Google Scholar |
Hidalgo-Ruz V, Gutow L, Thompson RC, Thiel M (2012) Microplastics in the marine environment: a review of the methods used for identification and quantification. Environmental Science & Technology 46(6), 3060-3075.
| Crossref | Google Scholar | PubMed |
Hu CJ, Garcia MA, Nihart A, Liu R, Yin L, Adolphi N, Gallego DF, Kang H, Campen MJ, Yu X (2024) Microplastic presence in dog and human testis and its potential association with sperm count and weights of testis and epididymis. Toxicological Sciences 200(2), 235-240.
| Crossref | Google Scholar | PubMed |
Karami A, Golieskardi A, Ho YB, Larat V, Salamatinia B (2017) Microplastics in eviscerated flesh and excised organs of dried fish. Scientific Reports 7(1), 5473.
| Crossref | Google Scholar |
Lavers JL, Bond AL, Hutton I (2014) Plastic ingestion by Flesh-footed Shearwaters (Puffinus carneipes): implications for fledgling body condition and the accumulation of plastic-derived chemicals. Environmental Pollution 187, 124-129.
| Crossref | Google Scholar | PubMed |
Leslie HA, van Velzen MJM, Brandsma SH, Vethaak AD, Garcia-Vallejo JJ, Lamoree MH (2022) Discovery and quantification of plastic particle pollution in human blood. Environment International 163, 107199.
| Crossref | Google Scholar |
Li Y, Tao L, Wang Q, Wang F, Li G, Song M (2023) Potential health impact of microplastics: a review of environmental distribution, human exposure, and toxic effects. Environment & Health 1(4), 249-257.
| Crossref | Google Scholar | PubMed |
Li Y, Chen L, Zhou N, Chen Y, Ling Z, Xiang P (2024) Microplastics in the human body: a comprehensive review of exposure, distribution, migration mechanisms, and toxicity. Science of The Total Environment 946, 174215.
| Crossref | Google Scholar |
Ling SD, Sinclair M, Levi CJ, Reeves SE, Edgar GJ (2017) Ubiquity of microplastics in coastal seafloor sediments. Marine Pollution Bulletin 121(1-2), 104-110.
| Crossref | Google Scholar | PubMed |
Napper IE, Davies BFR, Clifford H, Elvin S, Koldewey HJ, Mayewski PA, Miner KR, Potocki M, Elmore AC, Gajurel AP, Thompson RC (2020) Reaching new heights in plastic pollution – preliminary findings of microplastics on Mount Everest. One Earth 3(5), 621-630.
| Crossref | Google Scholar |
Needham S, Funge-Smith SJ (2015) The consumption of fish and fish products in the Asia–Pacific region based on household surveys. RAP Publication 2015/12. (Food and Agriculture Organization of The United Nations, Regional Office for Asia and the Pacific: Bangkok, Thailand) Available at http://www.fao.org/3/a-i5151e.pdf
Nelms SE, Galloway TS, Godley BJ, Jarvis DS, Lindeque PK (2018) Investigating microplastic trophic transfer in marine top predators. Environmental Pollution 238, 999-1007.
| Crossref | Google Scholar | PubMed |
Nelms SE, Barnett J, Brownlow A, Davison NJ, Deaville R, Galloway TS, Lindeque PK, Santillo D, Godley BJ (2019) Microplastics in marine mammals stranded around the British coast: ubiquitous but transitory? Scientific Reports 9(1), 1075.
| Crossref | Google Scholar |
Ogunola SO, Reis-Santos P, Wootton N, Gillanders BM (2022) Microplastics in decapod crustaceans sourced from Australian seafood markets. Marine Pollution Bulletin 179, 113706.
| Crossref | Google Scholar |
Ogunola SO, Reis-Santos P, Wootton N, Gillanders BM (2023) Investigating microplastic contamination and biomagnification in a remote area of South Australia. Marine and Freshwater Research 74(11), 917-927.
| Crossref | Google Scholar |
Ory NC, Gallardo C, Lenz M, Thiel M (2018) Capture, swallowing, and egestion of microplastics by a planktivorous juvenile fish. Environmental Pollution 240, 566-573.
| Crossref | Google Scholar | PubMed |
Peng Y, Wu P, Schartup AT, Zhang Y (2021) Plastic waste release caused by COVID-19 and its fate in the global ocean. Proceedings of the National Academy of Sciences 118(47), e2111530118.
| Crossref | Google Scholar |
Piyawardhana N, Weerathunga V, Chen H-S, Guo L, Huang P-J, Ranatunga RRMKP, Hung C-C (2022) Occurrence of microplastics in commercial marine dried fish in Asian countries. Journal of Hazardous Materials 423, 127093.
| Crossref | Google Scholar |
Prabhu K, Ghosh S, Sethulekshmi S, Shriwastav A (2024) In vitro digestion of microplastics in human digestive system: insights into particle morphological changes and chemical leaching. Science of The Total Environment 934, 173173.
| Crossref | Google Scholar |
Provencher JF, Ammendolia J, Rochman CM, Mallory ML (2019) Assessing plastic debris in aquatic food webs: what we know and don’t know about uptake and trophic transfer. Environmental Reviews 27(3), 304-317.
| Crossref | Google Scholar |
Rochman CM, Hoh E, Hentschel BT, Kaye S (2013) Long-term field measurement of sorption of organic contaminants to five types of plastic pellets: implications for plastic marine debris. Environmental Science & Technology 47(3), 1646-1654.
| Crossref | Google Scholar | PubMed |
Rochman CM, Hentschel BT, Teh SJ (2014) Long-term sorption of metals is similar among plastic types: implications for plastic debris in aquatic environments. PLoS ONE 9(1), e85433.
| Crossref | Google Scholar |
Rukmangada R, Naidu BC, Nayak BB, Balange A, Chouksey MK, Xavier KAM (2023) Microplastic contamination in salted and sun dried fish and implications for food security – a study on the effect of location, style and constituents of dried fish on microplastics load. Marine Pollution Bulletin 191, 114909.
| Crossref | Google Scholar |
Sevwandi Dharmadasa WLS, Andrady AL, Kumara PBTP, Maes T, Gangabadage CS (2021) Microplastic pollution in marine protected areas of southern Sri Lanka. Marine Pollution Bulletin 168, 112462.
| Crossref | Google Scholar |
Sewwandi M, Amarathunga AAD, Wijesekara H, Mahatantila K, Vithanage M (2022) Contamination and distribution of buried microplastics in Sarakkuwa beach ensuing the MV X-Press Pearl maritime disaster in Sri Lankan sea. Marine Pollution Bulletin 184, 114074.
| Crossref | Google Scholar |
Smith M, Love DC, Rochman CM, Neff RA (2018) Microplastics in seafood and the implications for human health. Current Environmental Health Reports 5(3), 375-386.
| Crossref | Google Scholar | PubMed |
Tumwesigye E, Felicitas Nnadozie C, Akamagwuna FC, Siwe Noundou X, Nyakairu GW, Odume ON (2023) Microplastics as vectors of chemical contaminants and biological agents in freshwater ecosystems: current knowledge status and future perspectives. Environmental Pollution 330, 121829.
| Crossref | Google Scholar |
Ugwu K, Herrera A, Gómez M (2021) Microplastics in marine biota: a review. Marine Pollution Bulletin 169, 112540.
| Crossref | Google Scholar |
Vethaak AD, Legler J (2021) Microplastics and human health. Science 371(6530), 672-674.
| Crossref | Google Scholar | PubMed |
Walkinshaw C, Lindeque PK, Thompson R, Tolhurst T, Cole M (2020) Microplastics and seafood: lower trophic organisms at highest risk of contamination. Ecotoxicology and Environmental Safety 190, 110066.
| Crossref | Google Scholar |
Wickham H, Averick M, Bryan J, Chang W, D’Agostino McGowan L, Francois R, Grolemund G, Hayes A, Henry L, Hester J, Kuhn M, Pedersen TL, Miller E, Milton Bache S, Muller K, Ooms J, Robinson D, Seidel PD, Spinu V, Takahashi K, Vaughan D, Wilke C, Woo K, Yutani H (2019) Welcome to the tidyverse. Journal of Open Source Software 4, 1686.
| Crossref | Google Scholar |
Wootton N, Reis-Santos P, Gillanders BM (2021a) Microplastic in fish – a global synthesis. Reviews in Fish Biology and Fisheries 31(4), 753-771.
| Crossref | Google Scholar |
Wootton N, Reis-Santos P, Dowsett N, Turnbull A, Gillanders BM (2021b) Low abundance of microplastics in commercially caught fish across southern Australia. Environmental Pollution 290, 118030.
| Crossref | Google Scholar |
Wootton N, Sarakinis K, Varea R, Reis-Santos P, Gillanders BM (2022) Microplastic in oysters: a review of global trends and comparison to southern Australia. Chemosphere 307, 136065.
| Crossref | Google Scholar |
Wootton N, Reis-Santos P, Adyel T, Blewitt M, Clarke B, Crutchett T, Hamann M, Hardesty D, Lavers J, Leterme S, Leusch F, Lynch S, Motti C, O’Brien A, Okoffo E, Perera K, Puskic P, Razzell Holls J, Roman L, Santana MFM, Snigirova A, Tuuri EM, Wilson S, Ziajahromi S, Gillanders BM (2024) 13. Microplastics. In ‘Marine Sampling Field Manuals for Monitoring Australia’s Commonwealth Waters’, ver. 3. (Eds R Przeslawski, S Foster) (National Environmental Science Programme) Available at https://microplastics-field-manual.github.io
Worm B, Lotze HK, Jubinville I, Wilcox C, Jambeck J (2017) Plastic as a persistent marine pollutant. Annual Review of Environment and Resources 42, 1-26.
| Crossref | Google Scholar |
Wright SL, Thompson RC, Galloway TS (2013) The physical impacts of microplastics on marine organisms: a review. Environmental Pollution 178, 483-492.
| Crossref | Google Scholar | PubMed |
Yang Y, Xie E, Du Z, Peng Z, Han Z, Li L, Zhao R, Qin Y, Xue M, Li F, Hua K, Yang X (2023) Detection of various microplastics in patients undergoing cardiac surgery. Environmental Science & Technology 57(30), 10911-10918.
| Crossref | Google Scholar | PubMed |
Ziccardi LM, Edgington A, Hentz K, Kulacki KJ, Kane Driscoll S (2016) Microplastics as vectors for bioaccumulation of hydrophobic organic chemicals in the marine environment: a state-of-the-science review. Environmental Toxicology and Chemistry 35(7), 1667-1676.
| Crossref | Google Scholar | PubMed |