No effects of abiotic and anthropogenic factors on reef-associated neonate shark abundance within a shark nursery-area system
I. A. Bouyoucos
A
B
C
D
E
F
G
H
Abstract
Coastal habitats function as shark nursery areas; however, coastal habitats can experience extreme variation in abiotic conditions and are susceptible to human disturbances.
Drivers of abundance were tested within a shark nursery-area system in two populations of reef-associated neonate sharks, namely, blacktip reef sharks (Carcharhinus melanopterus) and sicklefin lemon sharks (Negaprion acutidens).
Catch data from a fisheries-independent gill-net survey (n = 90 sets from October 2018 to March 2019) at 10 sites around Moorea, French Polynesia, were used to test for associations between shark abundance and abiotic conditions (temperature, oxygen, pH, salinity, lunar phase and depth). Historical levels of fin-fish fishing effort, trampling (i.e. human movement through habitat), and coastal artificialisation (i.e. walls and embankments) estimated for each site were used to test for anthropogenic effects on shark abundance.
There were no effects of any abiotic or anthropogenic factor on abundance of either species.
Previous work corroborates our findings by demonstrating neonate sharks’ physiological tolerance to extreme abiotic conditions and high survival in response to anthropogenic stressors. Alternatively, populations are already degraded from decades of coastal development.
These data can aid in predicting the use of coastal habitats as shark nursery areas.
Keywords: blacktip reef shark, catch-per-unit-effort, coastal development, fishing pressure, human disturbance, sicklefin lemon shark, temperature, young-of-the-year.
Introduction
Coastal habitats can play important roles for sharks. Some species spend their entire life among coastal habitats, whereas others use them repeatedly over varying temporal scales (e.g. for foraging, reproduction, or refuge from seasonally extreme abiotic conditions), and some just rely on them during early ontogeny (Knip et al. 2010). For example, neonates and juveniles may use coastal habitats as nursery areas (Heupel et al. 2019), which are thought to improve the recruitment of reproducing adults (Beck et al. 2001; Fodrie et al. 2009). Because of the potential significance of shark nursery areas for fitness in shark populations (Heupel et al. 2007; Kinney and Simpfendorfer 2009), there is general interest in identifying such habitats (e.g. Simpfendorfer and Milward 1993; DeAngelis et al. 2008; Froeschke et al. 2010a) and defining drivers of habitat use within those potential nursery areas as population management or conservation tools (e.g. Carrier and Pratt 1998; Heupel and Simpfendorfer 2005; Oh et al. 2017a). Known drivers of shark abundance include local abiotic conditions and anthropogenic influences (Schlaff et al. 2014; MacNeil et al. 2020), and these may also explain why sharks use, or do not use, habitats as nursery areas.
Within coastal areas, changes in abiotic conditions affect the distribution, abundance and patterns of habitat use in young sharks. Associations among various abiotic conditions (e.g. temperature, salinity, turbidity, oxygen, pH, tide, photoperiod) have been observed in sharks across life-history stages and have been discussed in a comprehensive review by Schlaff et al. (2014). Temperature can affect the abundance of neonate and juvenile reef sharks (Yates et al. 2015; Oh et al. 2017a; Vierus et al. 2018). For example, juvenile bull sharks (Carcharhinus leucas) were documented to shift their nursery area poleward in response to climate-driven ocean warming (Bangley et al. 2018a). Oxygen also has variable effects on young shark abundance (Froeschke et al. 2010b; Oh et al. 2017a; Bangley et al. 2018b), which is thought to reflect the inter-specific differences in oxygen-supply capacity (Dubuc et al. 2021). Salinity is another important driver of the abundance of young sharks (Froeschke et al. 2010b; Yates et al. 2015), including euryhaline species (Dwyer et al. 2020). There is interest in identifying abiotic drivers of abundance in young sharks because of the importance of predicting important habitats for shark management and conservation (Oh et al. 2017a), and for adaptive management of shark populations in the face of climate change (Bouyoucos and Rummer 2021).
Globally, reef shark populations are experiencing declines owing to environmental change and anthropogenic pressures (Sherman et al. 2023). Anthropogenic influences also demonstrate potent effects on the distribution, abundance and habitat-use patterns in sharks. Fishing pressure (i.e. general fishing activity that may interact with predators via capture as bycatch, loss of prey species, etc.) and the accessibility of marine habitats by humans has been shown to negatively affect the abundance of predators, including sharks (Nadon et al. 2012; Cinner et al. 2018; Frisch and Rizzari 2019; Clementi et al. 2021; Lester et al. 2022). However, this can be reversed by implementing science-based fisheries management (e.g. reducing catch limits, fishing bans, or designating marine protected areas, MPAs); indeed, these strategies have been found to support high shark abundance (Ward-Paige and Worm 2017; MacNeil et al. 2020). Anthropogenic-driven habitat loss or degradation can also affect the survival and abundance of sharks. For example, the abundance of juvenile lemon sharks (Negaprion brevirostris) within a Bahamian nursery area decreased in response to coastal development, likely because of the associated habitat loss (Jennings et al. 2008). Similarly, studies using environmental DNA also suggested that shark species richness declines with increasing levels of anthropogenic disturbances (Bakker et al. 2017). As such, sources of anthropogenic disturbance related to fishing pressure, direct human impacts on shark nursery areas, and coastal development should be considered when assessing drivers of shark abundance.
The purpose of this study was to test the effects of abiotic conditions and the level of anthropogenic impact on the abundance of two populations of reef-associated neonate sharks within a shark nursery-area system. Neonate blacktip reef (Carcharhinus melanopterus) and sicklefin lemon (Negaprion acutidens) sharks were studied at 10 sites around the island of Moorea, French Polynesia. This is a system that is known to contain shark nursery areas for both species (Bouyoucos et al. 2022), where shark fishing has been prohibited since 2012. The first study objective was to test for differences in neonate C. melanopterus and N. acutidens abundance and abiotic conditions (i.e. temperature, oxygen, pH, salinity and depth) among sites around Moorea. Then, we tested for associations between neonate abundance and abiotic conditions (i.e. temperature, oxygen, pH, salinity, tide, depth and lunar phase) across all sites. The second study objective was to determine the historical levels of anthropogenic impact (i.e. non-targeted fishing effort, direct human impact and coastal artificialisation by construction of walls and embankments) for each site around the island and then test for associations between neonate abundance and the level of anthropogenic impact across all sites. Together, these data are important for understanding the effects of environmental change and anthropogenic effects on the abundance of reef-associated neonate sharks. More broadly, our results can aid in predicting the use of habitats as shark nursery areas, information that is critical for spatiotemporal planning and management of important shark habitats.
Materials and methods
Study site and species
This study was conducted on the island of Moorea in French Polynesia (17°30′S, 149°50′W). French Polynesia was designated a ‘shark sanctuary’ when the commercial harvest of all shark species was banned in 2012 (Ward-Paige 2017). The lagoon surrounding the island of Moorea has resident populations of neonatal C. melanopterus and N. acutidens that have been studied extensively by the Centre de Recherches Insulaires et Observatoire de l’Environnement (CRIOBE) in collaboration with the Physioshark Project on Moorea since 2007 (Mourier et al. 2013a; Debaere et al. 2023). Ten sites (i.e. Apaura, Haapiti, Maharepa, Paorea, Papetoai, Pihaena, Tiki, Vaiane, Vaiare and Valorie) that encompass the island’s ~60-km coastline are surveyed annually during the wet season (October–March) with gill-nets to sample shark neonates (Fig. 1). The areas of these sites that are being surveyed are very shallow (i.e. <2 m deep), relatively small (i.e. <1 km2), and are delineated by deep channels that occur naturally or have been dredged. Channels are typically within 50 m of shore and are thought to limit the distribution of shark neonates (Bouyoucos et al. 2020a). Apaura and Vaiane are nursery areas for N. acutidens (Bouyoucos et al. 2022), meaning that these sites exhibit high shark abundance relative to other sites and persistent use of these habitats by individuals over time (Heupel et al. 2007). Abundance of neonatal C. melanopterus appears to fluctuate between Maharepa and Paorea on an annual basis, suggesting that these sites may serve as nursery areas over longer time scales (Bouyoucos et al. 2022). Yet, there are some sites around Moorea where both neonates of both species can co-occur (Matich et al. 2017).
Locations of 10 reef-associated neonate shark sampling sites all around the island of Moorea, French Polynesia (17°30‘S, 149°50′W).
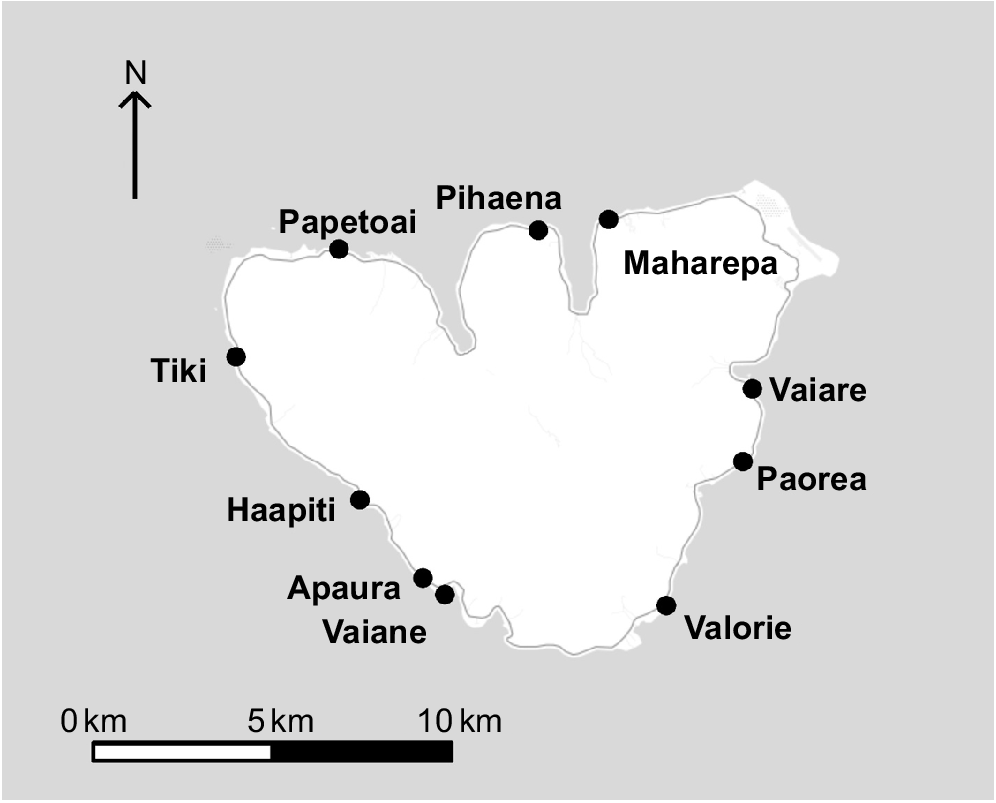
The abundance of neonates of both species around Moorea increases annually around October. The parturition season for C. melanopterus is thought to occur annually from September through February, with the most births occurring in November (Debaere et al. 2023), whereas the parturition season for N. acutidens occurs biennially from July through November (Porcher 2005; Mourier et al. 2013b). Then, the abundance of neonates decreases around January, as parturition ends and neonates emigrate from the sampling sites or experience mortality (Mourier and Planes 2013; Mourier et al. 2013b). During the parturition season, monthly temperatures at the above-mentioned sites range between 27 and 31°C (Eustache et al. 2024), with daily temperatures ranging from 26 to 36°C and most sites experiencing oxygen supersaturation (Bouyoucos et al. 2021). Neonates of both species are reported to exhibit site fidelity around Moorea, as evidenced by recaptures of individuals at a single site and not multiple sites during the months following parturition (Bouyoucos et al. 2022); indeed, this reflects what has been documented for these species elsewhere (Lea et al. 2016; Oh et al. 2017b).
Gill-net survey
Sampling occurred between 1 October 2018 and 12 February 2019. One site was fished per night, and all 10 sites were fished at least once per fortnight. Sampling effort was standardised to control for possible confounding effects of time of day by fishing for a 3-h period, usually from 17:00 to 20:00 hours. Neonatal C. melanopterus and N. acutidens were captured using monofilament gill-net (50 m long by 1.5 m tall with 5-cm mesh; 10-cm stretch) set perpendicularly from shore. In some sites (e.g. Apaura, Maharepa, Pihaena, Valorie), the gill-net reached from shore all the way to the nearest deep channel delineating the site. Sharks were removed from gill-nets within minutes of capture to minimise the possibility of capture-induced mortality (Dapp et al. 2017; Bouyoucos et al. 2018). Once removed, sharks were photographed and tagged for identification with passive integrated transponder (PIT) tags, measured (fork and total length, mm; weight, kg; and girth, mm), sexed and then released. The duration of each gill-net set and the number of C. melanopterus and N. acutidens individuals were recorded. Set number was coded (i.e. 1, 2… n) for inclusion in models to test for temporal effects. Catch-per-unit effort (CPUE, sharks h−1) was calculated for each species for each set as the total number of sharks caught per hour of fishing.
Measuring abiotic conditions
To test whether shark abundance was correlated with abiotic conditions, environmental conditions were measured and recorded at the beginning of each gill-net set. Single temperature (°C), dissolved oxygen (mg L−1), pH (National Bureau of Standards scale) and salinity readings were taken at the mid-point of the net at the suface of the water. Temperature and oxygen were measured with a YSI Pro 20 (YSI Inc., Yellow Springs, OH, USA), pH was measured with a Seven2Go Pro (Mettler-Toledo GmbH, Greifensee, Switzerland), and salinity was measured with a hand-held refractometer. Depth (cm) was measured at the mid-point of the net. Tidal phase (i.e. ebbing, flooding) and lunar phase (percentage of full moon) were recorded from local online tables.
Defining anthropogenic impact
The following three types of anthropogenic impacts were investigated: fishing effort, direct human impact (i.e. trampling) and coastal artificialisation. Fishing effort was obtained from survey and indirect mapping from Thiault et al. (2017), by combining fishing capacity on the basis of the density of households and their dependency on fishing for livelihood, with fishing suitability, based on fishers’ preference for fishing grounds (Thiault et al. 2017). These data, therefore, provide an indication of relative fin-fish fishing effort for a given area. Indeed, fishing effort does not reflect targeted shark fishing, which was banned in French Polynesia (c. 2012); rather, fishing effort describes the potential for shark bycatch, alongside catch of target, non-shark species that may be predators or prey of shark neonates. Indeed, fin-fish fishing, predominantly by hook-and-line or spear, is not banned at any of the study sites; however, several of the sites (e.g. Paorea, Pihaena) occur on the border of MPAs (Thiault et al. 2019). Direct human impact, which represents the volume of human movement through an area, was assessed for areas <1.8 m deep by applying a kernel-density function with a 200-m radius around all households (weighted by the number of people per household) and high-density tourism sites (weighted by daily visitation rates), as described in Loiseau et al. (2021). These data were collected in 2012 (household size) and 2017 (tourism visitation) and were originally presented in Loiseau et al. (2021). Finally, coastal artificialisation was modelled on the basis of a high-resolution shoreline categorisation undertaken around the entire island in 2018, by using previously published data (Madi Moussa et al. 2019). A coastal artificialisation index was obtained by extrapolating the ‘walls and embankments’ category onto the lagoon by using a 100-m radius (Loiseau et al. 2021). Each metric was mapped throughout Moorea’s lagoon at a 5- × 5-m resolution, and values were extracted at each of the 10 study sites by taking the average value within a 100-m radius of the centre of the net.
Statistical analysis
Catch-per-unit effort was compared among sites by using Kruskal–Wallis rank sum tests, by using the R package Stats (ver. 4.4.0, see https://stat.ethz.ch/R-manual/R-devel/library/stats/html/00Index.html; R Foundation for Statistical Computing, Vienna, Austria, see https://www.r-project.org/). Post hoc multiple comparisons were examined using the R package pgirmess (ver. 2.0.3, P. Giraudoux, see https://CRAN.R-project.org/package=pgirmess). Similarly, differences in temperature, oxygen, pH, salinity and depth among sites were tested by using Kruskal–Wallis rank sum tests.
Correlation and collinearity between continuous explanatory variables (i.e. temperature, oxygen, pH, salinity, depth, log ratio transformed lunar phase and chronological set number) was first assessed with Pearson’s correlation coefficients (r) and variance inflation factors (VIF) respectively. Pearson’s r and VIF were calculated using the R packages Stats and car packages (ver. 3.1-2, see https://CRAN.R-project.org/package=car; Fox and Weisberg 2019). Criteria for correlation and collinearity were r ≥ 0.80 and VIF ≥ 3 respectively (Zuur et al. 2009). Parameters that did not meet these criteria were excluded from the final models.
Effects of abiotic conditions on shark abundance were assessed using generalised linear mixed models (GLMM). Shark abundance was assumed to follow a negative binomial distribution (Yates et al. 2015), and visual inspection of histograms of C. melanopterus and N. acutidens abundance suggested that shark abundance data were zero-inflated for both species. To account for variation in sampling effort among sets, the logarithm of sampling effort in minutes was included as an offset variable (Yates et al. 2015; Vierus et al. 2018). Site was included as a random effect to account for repeated sampling within sites and to investigate trends across C. melanopterus and N. acutidens neonate subpopulations around Moorea. Within models for each species, sites were excluded if no sharks were caught during the entire study. Zero-inflated negative binomial GLMMs were implemented for each species by using the R package glmmTMB (ver. 1.1.9, see https://cran.r-project.org/package=glmmTMB; Brooks et al. 2017). Model validation included visual inspection of scatterplots of model residuals against fitted values to assess homogeneity, and model residuals against explanatory variables to assess independence (Zuur et al. 2009). Statistical significance was determined using 95% confidence intervals (CIs) of fixed-effect term estimates generated from 1000 posterior distributions (Hasler et al. 2016).
Because of differences in the temporal scale of abiotic and anthropogenic variables, these were modelled separately. Effects of anthropogenic impacts on CPUE were tested using linear models. Associations were investigated within each species between the natural logarithm of anthropogenic impact score (fishing effort, trampling and artificialisation) and mean CPUE at each site. Owing to differences in variation in CPUE among sites, CPUE was weighted by its standard error for each site. Model validation was conducted as described previously. Analyses were conducted using the R package Stats.
Ethical approval
All methods described herein were approved by the James Cook University Animal Ethics Committee (A2394). Permission to conduct research on sharks was granted by the French Polynesian Ministère de la Promotion des Langues, de la Culture, de la Communication, et de l’Environnement (Arrêté Number 5129).
Results
Site-specific differences
Over the course of the 2018–2019 parturition season, 90 gill-net sets resulted in the capture of 110 C. melanopterus and 116 N. acutidens individuals (Table 1). Accounting for recaptures, 94 C. melanopterus individuals and 80 N. acutidens individuals were caught in total. Shark abundance, measured as catch-per-unit effort (CPUE; sharks h−1), differed among sites for C. melanopterus (Kurskal–Wallis rank sum test, χ2 = 42.65, d.f. = 9, P < 0.001; Fig. 2) and N. acutidens (χ2 = 37.74, d.f. = 6, P < 0.001; Fig. 2). At Haapiti, CPUE of C. melanopterus was higher than that at Apaura, Maharepa, Pihaena, Tiki, and Vaiane, whereas CPUE of N. acutidens at Vaiane and Tiki was higher than that at Maharepa, Papetoai and Valorie. All C. melanopterus and most N. acutidens individuals were neonates. At least four N. acutidens individuals were 1- or 2-year-old juveniles; three of these sharks were recaptured at Vaiane and Apaura from the previous survey year (i.e. 2017–2018), and one individual that was captured at Valorie was 1100 mm in total length. C. melanopterus was present at all sites, whereas N. acutidens was absent from Haapiti, Paorea and Vaiare.
Site | Gill-net sets (n) | Sampling effort (h) | Carcharhinus melanopterus (n) | Negaprion acutidens (n) | |
---|---|---|---|---|---|
Apaura | 8 | 21.0 | 2 | 13 | |
Haapiti | 12 | 25.7 | 33 | 0 | |
Maharepa | 10 | 25.6 | 3 | 2 | |
Paorea | 9 | 22.1 | 13 | 0 | |
Papetoai | 10 | 25.7 | 24 | 1 | |
Pihaena | 9 | 19.25 | 1 | 9 | |
Tiki | 9 | 21.9 | 4 | 24 | |
Vaiane | 7 | 15.8 | 4 | 62 | |
Vaiare | 9 | 23.4 | 12 | 0 | |
Valorie | 7 | 16.3 | 14 | 5 |
Catch-per-unit effort (CPUE) of reef-associated neonate sharks from 10 sites around Moorea, French Polynesia. Data are presented as means with standard errors of the mean. Individual observations (n = 7–12) represent CPUE for C. melanopterus and N. acutidens calculated for individual sampling events at each site. Different letters represent significant differences in CPUE between sites within each species (Kruskal–Wallis test, P < 0.05). Note that N. acutidens was not encountered at the Haapiti, Paorea and Vaiare sites.
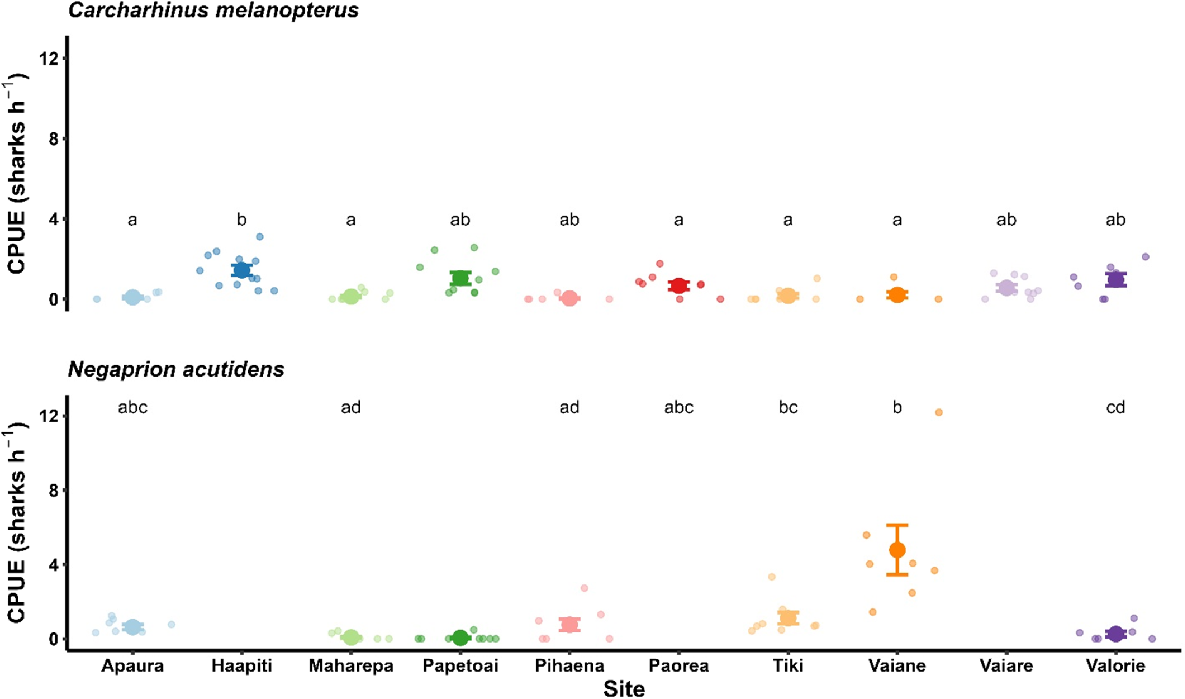
There were significant differences in dissolved oxygen (Kurskal–Wallis rank sum test, χ2 = 41.72, d.f. = 9, P < 0.001), pH (χ2 = 48.19, d.f. = 9, P < 0.001) and depth among sites (χ2 = 40.76, d.f. = 9, P < 0.001). Temperature and salinity did not differ significantly among sites (Fig. 3). No variable, including depth, differed between tide states (i.e. ebbing or flooding; data not shown).
Abiotic conditions of 10 reef-associated neonate shark sampling sites around Moorea, French Polynesia. Data are presented as means with standard errors of the mean. Individual observations (n = 7–12) represent values for abiotic conditions recorded at individual sampling events within each site. Different letters represent significant differences in CPUE between sites within each species (Kruskal–Wallis test, P < 0.05).
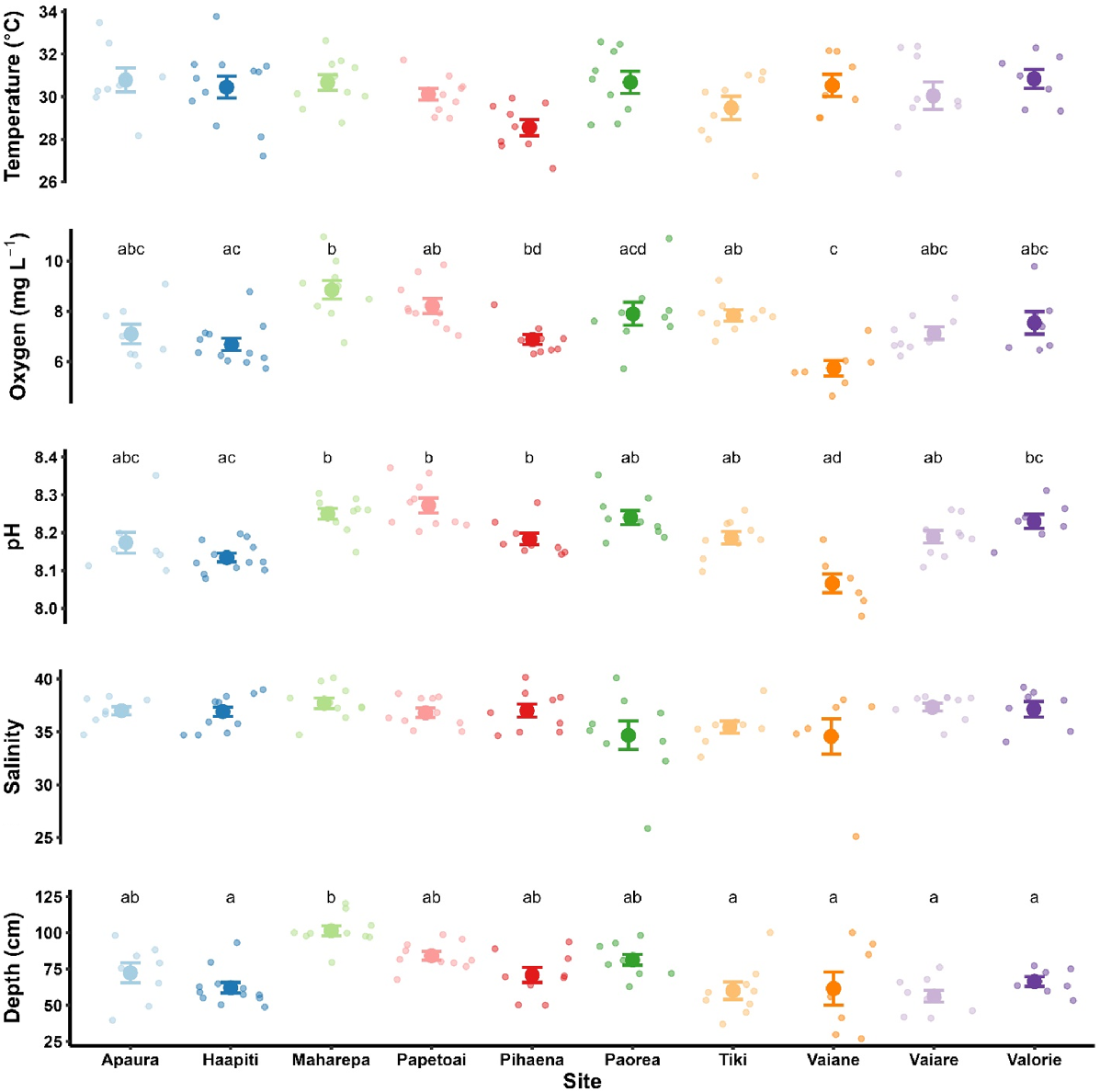
Abiotic conditions
Oxygen was found to be correlated with pH (r = 0.801) and exhibited collinearity (VIF ≥ 3) with temperature, salinity, depth, lunar phase. pH exhibited collinearity with set number and lunar phase. Therefore, statistically informed selection criteria (i.e. Pearson’s r and VIF) justified the a priori decision to exclude oxygen and pH as explanatory variables of shark abundance in further models. All other explanatory variables (i.e. temperature, salinity, depth, lunar phase and set number) were not correlated (r = −0.433–0.441), did not exhibit collinearity (VIF < 2), and were, therefore, included in final models. Although abiotic conditions did differ among sites (Fig. 3), there were no significant effects of any variable (i.e. temperature, salinity, tide, depth, lunar phase and set number) on the abundance of C. melanopterus or N. acutidens (Table 2).
Response | Parameter | Effect size | 95% CI | |
---|---|---|---|---|
Abundance of C. melanopterus | Intercept | 1.459 | −4.88 to 7.79 | |
Temperature | −0.084 | −0.244 to 0.075 | ||
Salinity | −0.019 | −0.122 to 0.082 | ||
Depth | −0.006 | −0.022 to 0.009 | ||
Lunar phase | 0.011 | −0.053 to 0.075 | ||
Tide | 0.428 | −0.031 to 0.89 | ||
Set number | −0.004 | −0.014 to 0.004 | ||
Abundance of N. acutidens | Intercept | −7.781 | −15.471 to −0.092 | |
Temperature | 0.188 | −0.029 to 0.406 | ||
Salinity | 0.007 | −0.081 to 0.095 | ||
Depth | 0.002 | −0.011 to 0.015 | ||
Lunar phase | 0.029 | −0.064 to 0.122 | ||
Tide | −0.245 | −0.742 to 0.253 | ||
Set number | −0.008 | −0.018 to 0.002 |
Shark abundance data were zero-inflated and analysed assuming a negative binomial distribution. Outputs are presented as mean effect sizes and 95% confidence intervals (CI) of fixed-effect estimates generated from 1000 posterior simulations.
Anthropogenic impact
Anthropogenic-impact scores varied considerably among sites, as demonstrated by coefficients of variation for fishing effort (32%), artificialisation index (82%) and direct human impact (58%). However, linear regressions did not show statistically significant relationships between CPUE and any anthropogenic impact score (Fig. 4, Table 3).
Associations between various anthropogenic impacts and reef-associated neonate shark abundance at different sites around Moorea, French Polynesia. Individual observations (Carcharhinus melanopterus n = 10; Negaprion acutidens n = 7) represent the mean impact value estimated for individual sites and mean catch-per-unit effort (CPUE) for (a–c) C. melanopterus and (d–f) N. acutidens at each site. Error bars represent standard errors of the mean of CPUE at each site. Shading around regression lines represents 95% confidence intervals. Regression parameters are presented in Table 3.
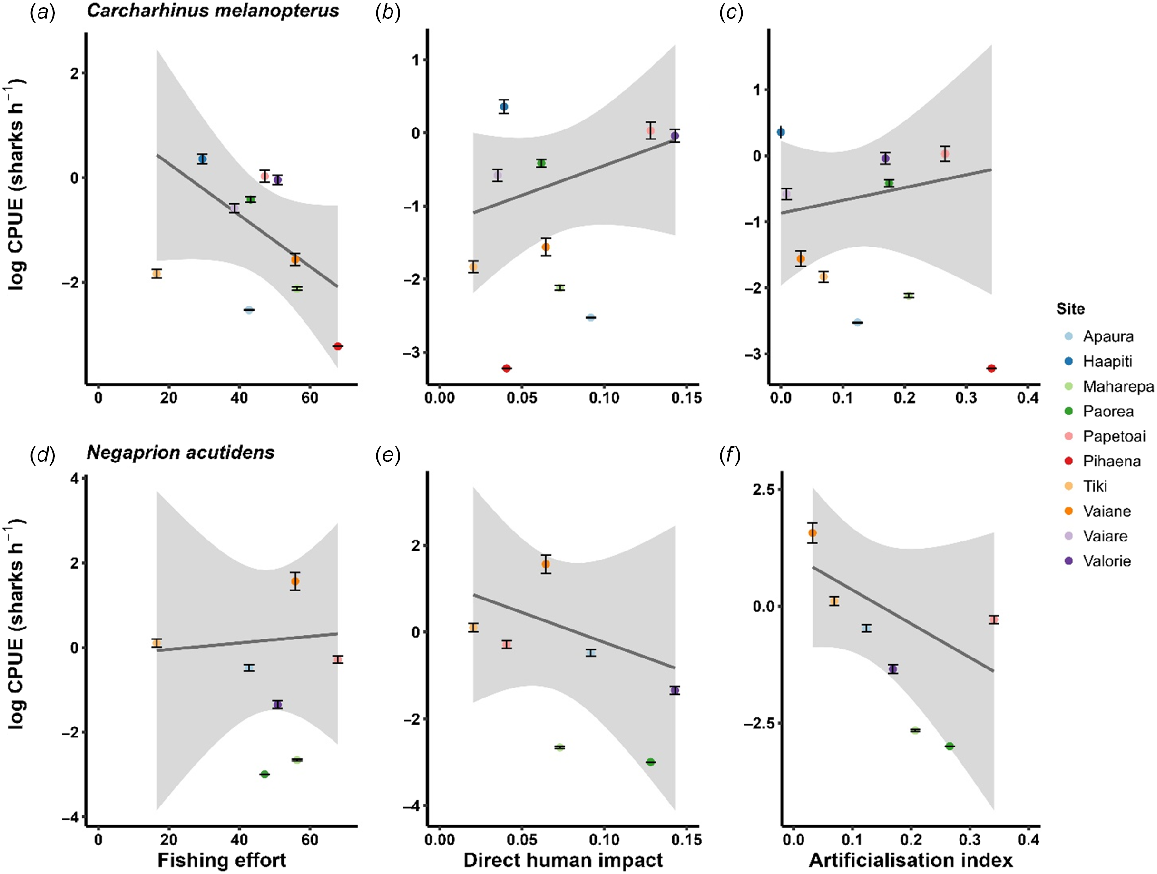
Response | Parameter | Estimate | Error | t-value | P-value | |
---|---|---|---|---|---|---|
CPUE of C. melanopterus | Intercept | 1.248 | 1.282 | 0.973 | 0.358 | |
Fishing effort | −0.049 | 0.026 | −1.871 | 0.098 | ||
Intercept | −1.261 | 0.576 | −2.188 | 0.065 | ||
Direct human impact | 8.139 | 6.747 | 1.206 | 0.267 | ||
Intercept | −0.870 | 0.462 | −1.881 | 0.102 | ||
Artificialisation index | 1.934 | 3.160 | 0.612 | 0.560 | ||
CPUE of N. acutidens | Intercept | −0.199 | 1.944 | −0.103 | 0.923 | |
Fishing effort | 0.008 | 0.038 | 0.203 | 0.849 | ||
Intercept | 1.140 | 1.143 | 0.998 | 0.375 | ||
Direct human impact | −13.785 | 14.393 | −0.958 | 0.392 | ||
Intercept | 1.068 | 0.718 | 1.488 | 0.211 | ||
Artificialisation index | −7.227 | 4.427 | −1.632 | 0.178 |
Discussion
The purpose of this study was to identify potential abiotic and anthropogenic drivers of abundance in neonatal reef sharks. Data collected at dusk during the 2018–2019 parturition season around the island of Moorea, French Polynesia, demonstrated that abiotic conditions were not associated with abundance or catch-per-unit effort (CPUE) of C. melanopterus and N. acutidens neonates. Our findings contradicted other studies on the same species that demonstrated that abiotic conditions, some of the same measured here, do affect abundance and habitat use (Oh et al. 2017b; Schlaff et al. 2017). Level of anthropogenic impact also did not explain among-site differences in CPUE of either species. As our data suggested, site-attached neonates must have some capacity to tolerate environmental and anthropogenic stressors within the parturition season and within their first months of life (Bouyoucos et al. 2018, 2022). It will be necessary to evaluate long-term trends to determine whether inter-annual variability in abiotic conditions or continued anthropogenic disturbances affect populations (Eustache et al. 2024). This type of information will be key to understanding whether additional management or protections (i.e. beyond shark-sanctuary status), especially on temporal (e.g. parturition season) and spatial (e.g. potential nurseries) scales, would affect the abundance of either population.
Abundance of neonates was not influenced by temperature. Although temperature acts directly on the physiology of aquatic ectotherms (e.g. Little et al. 2020) and exhibits variable effects on the abundance of reef-associated neonate sharks (Yates et al. 2015; Oh et al. 2017a; Vierus et al. 2018), we did not find that to be the case for C. melanopterus and N. acutidens around Moorea. Temperature also had only weak effects on habitat use (i.e. residence and kernel area) in other populations of juvenile C. melanopterus (Schlaff et al. 2017) and N. acutidens (Oh et al. 2017b) in Australia. Although we sampled only at dusk, the range of water temperatures at which sharks were caught in this study (26.3–33.8°C) matches the range of body temperatures (26.1–34.1°C) reported for free-ranging C. melanopterus neonates around Moorea (Bouyoucos et al. 2020b). Moreover, thermal tolerance estimates (i.e. critical thermal maximum; CTMax) for C. melanopterus (CTMax = 37°C; Bouyoucos et al. 2020b) and N. acutidens (CTMax = 36°C; Bouyoucos et al. 2021) exceed the maximum recorded temperatures (i.e. 33.8°C) in this study. Importantly, the abundance of C. melanopterus neonates around Moorea was not shown to be affected by seasonal temperatures over 7 years of monitoring (Eustache et al. 2024). Together, species-specific CTMax and body-temperature data corroborate the finding of this study of no effect of temperature on shark abundance.
Neonate shark abundance was also not associated with salinity. Rainfall and drought can affect the salinity of seawater in shallow, coastal environments, such that changes in salinity may affect the abundance of young sharks and rays (Chin et al. 2010; Knip et al. 2010). Indeed, decreases in salinity have been associated with decreases in abundance of reef-associated neonate sharks (Yates et al. 2015; Oh et al. 2017a; Vierus et al. 2018), including changes in patterns of habitat use in juvenile C. melanopterus (Schlaff et al. 2017). Rainfall, which is associated with reductions in salinity in shallow, coastal environments, also affected the habitat use (i.e. kernel area) of N. acutidens (Oh et al. 2017b). Conversely, C. melanopterus exhibits no changes in habitat use during tropical storms (Udyawer et al. 2013). During this study, sampling was conducted during all weather conditions to achieve a range of salinity (25–40); however, salinity only marginally dropped below that of full-strength seawater (~34) at two sites. However, of note is that the single greatest catch of N. acutidens in this study (12.2 sharks h−1) occurred at the Vaiane site immediately after rainfall, which resulted in a salinity of 25. Increases in shark neonate abundance with a decreasing salinity have been previously documented, where Vierus and colleagues observed an increase in the abundance of juvenile blacktip sharks (Carcharhinus limbatus) with a decreasing salinity (Vierus et al. 2018). Indeed, it has been suggested for young (euryhaline) sharks that salinity preference may differ from that of adults (Simpfendorfer et al. 2005; Heupel and Simpfendorfer 2008), and this may be associated with sufficient salinity tolerance to occupy habitats the variable salinities of which may exclude predators, such as estuaries (Morash et al. 2016). Although salinity tolerances of neonatal C. melanopterus and N. acutidens are unknown, salinity did not appear to consistently affect the abundance of neonatal C. melanopterus and N. acutidens around Moorea.
Dissolved oxygen and pH were both correlated with depth, which was also not a significant predictor of shark abundance despite past studies having found that shark abundance decreases with a decreasing dissolved oxygen (Schlaff et al. 2014). However, around Moorea, oxygen concentrations rarely fell below 100% saturation (i.e. environmental hyperoxia), and dissolved oxygen fell only below hypoxia tolerance thresholds (i.e. critical oxygen partial pressures; pcrit) in two sampling sets (Bouyoucos et al. 2021). Therefore, more severe hypoxia than what is typical of this system may have been necessary to elicit declines in abundance. We have previously demonstrated that neither C. melanopterus nor N. acutidens are hypoxia tolerant (pcrit = 85 and 78% saturation respectively; Bouyoucos et al. 2021); however, these species may undergo various physiological and behavioural responses to declining dissolved oxygen that could allow them to remain within their habitat, as has been documented for other shark and ray species (Parsons and Carlson 1998; Carlson and Parsons 2003; Dabruzzi and Bennett 2013). Thus, environmental hyperoxia is likely to have masked any potential effects of dissolved oxygen on neonate abundance here, which could also be the case for other species that are characteristically hypoxia intolerant.
The abundance of neonate sharks was also not affected by environmental cues, such as tidal state and lunar phase. Although these variables are generally associated with abundance and habitat use in sharks (Schlaff et al. 2014), effects can be quite variable, even within habitats (Hansell et al. 2018). Sharks in this study were fished on both ebbing and flooding tides and from new to full moon; yet, tidal state was not associated with water depth, which is unsurprising given Moorea’s geographic location in the South Pacific where tidal change is quite minimal (Andréfouët and Adjeroud 2019). Elsewhere, neonatal and juvenile C. melanopterus and N. acutidens individuals follow tidal cues to make use of mangroves for shelter (Oh et al. 2017b; George et al. 2019). Around Moorea, mangrove cover for sharks to exploit is unsubstantial (Madi Moussa et al. 2019), and minimal variation in tide height does not affect the availability of other refugia. Thus, a lack of tidal effects may relate to minimal tidal variability; however, an explanation for the lack of lunar-phase effects remains unclear. Shark abundance and lunar phase are sometimes associated through foraging (Lowry et al. 2007) and prey behaviour (Mourier et al. 2016); however, such trends may not be evident in shallow, coastal environments (Hansell et al. 2018), or be applicable to neonates (Vierus et al. 2018).
Although fishing effort, human impact and coastal artificialisation varied considerably among sites, these parameters did not predict neonate shark abundance around Moorea during the 2018–2019 parturition season. Reef shark populations in French Polynesia appear to be among the most abundant relative to reef shark populations elsewhere (MacNeil et al. 2020); however, benefits of local MPAs around Moorea have not been evaluated for sharks (Thiault et al. 2019). In the event that sharks are caught as bycatch, delayed mortality is low if C. melanopterus and N. acutidens are quickly released (Dapp et al. 2017; Bouyoucos et al. 2018). Neonates with retained fishing hooks are observed around Moorea (~4 sharks per parturition season; J. Rummer, pers. comm.), which suggests that some fishers may cut the line if a shark is hooked; indeed, this approach has been shown to improve survival in catch-and-release angling scenarios (Fobert et al. 2009). Furthermore, low post-release mortality rates suggest that fishing effort might have little effect on neonatal C. melanopterus and N. acutidens populations (Bouyoucos et al. 2018). Importantly, fishing effort, as defined in this study, includes other methods than hook-and-line or net techniques that can catch sharks as bycatch. In adult C. melanopterus, there is evidence of learned avoidance of fishing gear (Mourier et al. 2017). No studies have directly tested for an effect of trampling as a metric of human disturbance on shark abundance; however, trampling can lead to the destruction of mangrove habitat and coral reefs (Hardiman and Burgin 2010), which reef-associated neonate sharks depend on. Coastal artificialisation also did not directly affect shark abundance in this study; although, coastal artificialisation has been occurring for decades around Moorea (Madi Moussa et al. 2019). It may be that coastal artificialisation is not severe enough to affect the ecosystem to affect shark abundance. Conversely, at the time of the present study, shark populations around Moorea may have already been in a degraded state resulting from coastal artificialisation that has been increasing since the 1970s (Madi Moussa et al. 2019).
Beyond abiotic and anthropogenic stressors, an important driver of neonate abundance is fidelity to the location where parturition occurs. We have demonstrated through mark–recapture that C. melanopterus and N. acutidens neonates are not caught at more than one site (Bouyoucos et al. 2022; Debaere et al. 2023). Further, we have demonstrated that C. melanopterus neonates exhibit remarkably small home ranges (Bouyoucos et al. 2020a). Thus, site fidelity among reef-associated neonate sharks around Moorea may limit individuals from moving among sites despite variable abiotic conditions and levels of anthropogenic disturbance. Site fidelity in neonates is then potentially driven by reproductive philopatry by mothers, or the tendency of females to give birth at the same location over multiple years, which has been demonstrated for adult female C. melanopterus around Moorea (Mourier and Planes 2013; Eustache et al. 2023). Drivers of reproductive philopatry in C. melanopterus females are not understood; therefore, the extent to which variable abiotic conditions and levels of anthropogenic disturbance influence reproductive philopatry or explain why some female C. melanopterus individuals have been shown to reproduce at single or multiple sites is not clear (Eustache et al. 2023). Beyond reproductive philopatry, it is also pertinent to consider the effects of survival and dispersal from parturition sites over the course of each parturition season. Abundance of C. melanopterus generally declines over time during parturition seasons around Moorea (Eustache et al. 2024), which is suggestive of mortality within this population. Regarding dispersal, there is a notable absence of 1+ year-old reef sharks from abundance surveys of Moorea (Mourier et al. 2013a), which suggests that neonates will eventually leave their parturition site and is further suggestive of high mortality among neonates. Indeed, characteristics of the reef shark populations around Moorea (e.g. site fidelity, reproductive philopatry, natural mortality, etc.) are among myriad possible drivers of the abundance of neonates.
In conclusion, the abundance of neonate subpopulations of two reef shark species during the 2018–2019 parturition season was unaffected by abiotic conditions and historical levels of anthropogenic impact. Regarding anthropogenic impact, it is not clear from the present study whether shark neonate populations are resilient to the stressors that were investigated, or whether the populations are already in a degraded state owing to decades of impact (e.g. coastal artificialisation). Thus, a key area for future research will be establishing paired time series of anthropogenic impacts and shark abundance to examine temporal trends over larger scales. Ongoing monitoring of anthropogenic impacts on shark populations within shark nursery areas will be particularly important because the restricted distribution of the populations requires that they are tolerant of the sometimes-extreme abiotic conditions of their habitat. Indeed, animals cannot adapt to habitat loss, which is a strong driver of declines in reef shark and ray populations (Sherman et al. 2023), and a mechanism through which climate change is threatening sharks and rays (Dulvy et al. 2021). Because coastal and reef-associated shark species are thought to be among the most vulnerable to environmental change (Chin et al. 2010), efforts to mitigate compounding anthropogenic stressors in these environments will be important. As such, the present study has potential broader impacts on shark management within French Polynesia, which accounts for 25% of the area of all MPAs for sharks (i.e. ‘shark sanctuaries’), and similar systems through the implementation of quantitative techniques to assess nursery-area use in two widely distributed Indo-Pacific reef shark species.
Data availability
Data presented in this paper are available at the Research Data Repository (Tropical Data Hub) at James Cook University: https://doi.org/10.25903/2SFB-QX79.
Conflicts of interest
C. A. Simpfendorfer is an Associate Editor for Marine and Freshwater Research. Despite this relationship, he did not at any stage have editor-level access to this manuscript while in peer review, as is the standard practice when handling manuscripts submitted by an editor to this journal. Marine and Freshwater Research encourages its editors to publish in the journal and they are kept totally separate from the decision-making process for their manuscripts. The authors have no further conflicts of interest to declare.
Declaration of funding
I. A. Bouyoucos was supported by a JCU Postgraduate Research Scholarship. G. D. Schwieterman was supported by the National Science Foundation Graduate Research Program (DGE-1444317) and the Graduate Research Opportunities Worldwide Supplemental Funding Program. This project was supported by the Australian Research Council (ARC) Centre of Excellence for Coral Reef Studies, an ARC Discovery Early Career Researcher Award (PDE150101266 to J. L. Rummer), the Laboratoire d’Excellence ‘CORAIL’, the Station d’Écologie Expérimentale of the CRIOBE, and the French Ministère de l’Environnement.
References
Andréfouët S, Adjeroud M (2019) French Polynesia. In ‘World seas: an environmental evaluation. Vol. 2: the Indian Ocean to the Pacific’, 2nd edn. (Ed. C Sheppard) pp. 827–854. (Academic Press: London, UK) doi:10.1353/cp.2019.0013
Bakker J, Wangensteen OS, Chapman DD, Boussarie G, Buddo D, Guttridge TL, Hertler H, Mouillot D, Vigliola L, Mariani S (2017) Environmental DNA reveals tropical shark diversity in contrasting levels of anthropogenic impact. Scientific Reports 7, 16886.
| Crossref | Google Scholar | PubMed |
Bangley CW, Paramore L, Shiffman DS, Rulifson RA (2018a) Increased abundance and nursery habitat use of the bull shark (Carcharhinus leucas) in response to a changing environment in a warm-temperate estuary. Scientific Reports 8, 6018.
| Crossref | Google Scholar | PubMed |
Bangley CW, Paramore L, Dedman S, Rulifson RA (2018b) Delineation and mapping of coastal shark habitat within a shallow lagoonal estuary. PLoS ONE 13, e0195221.
| Crossref | Google Scholar | PubMed |
Beck MW, Heck KL, Able KW, Childers DL, Eggleston DB, Gillanders BM, Halpern B, Hays CG, Hoshino K, Minello TJ, Orth RJ, Sheridan PF, Weinstein MP (2001) The identification, conservation, and management of estuarine and marine nurseries for fish and invertebrates: a better understanding of the habitats that serve as nurseries for marine species and the factors that create site-specific variability in nursery quality will improve conservation and management of these areas. BioScience 51, 633-641.
| Crossref | Google Scholar |
Bouyoucos IA, Rummer JL (2021) Improving ‘shark park’ protections under threat from climate change using the conservation physiology toolbox. In ‘Conservation physiology: applications for wildlife conservation and management’. (Eds CL Madliger, CE Franklin, OP Love, SJ Cooke) pp. 185–204. (Oxford University Press) doi:10.1093/oso/9780198843610.003.0011
Bouyoucos IA, Weideli OC, Planes S, Simpfendorfer CA, Rummer JL (2018) Dead tired: evaluating the physiological status and survival of neonatal reef sharks under stress. Conservation Physiology 6, coy053.
| Crossref | Google Scholar |
Bouyoucos IA, Romain M, Azoulai L, Eustache K, Mourier J, Rummer JL, Planes S (2020a) Home range of newborn blacktip reef sharks (Carcharhinus melanopterus), as estimated using mark–recapture and acoustic telemetry. Coral Reefs 39, 1209-1214.
| Crossref | Google Scholar |
Bouyoucos IA, Morrison PR, Weideli OC, Jacquesson E, Planes S, Simpfendorfer CA, Brauner CJ, Rummer JL (2020b) Thermal tolerance and hypoxia tolerance are associated in blacktip reef shark (Carcharhinus melanopterus) neonates. Journal of Experimental Biology 223, jeb221937.
| Crossref | Google Scholar |
Bouyoucos IA, Trujillo JE, Weideli OC, Nakamura N, Mourier J, Planes S, Simpfendorfer CA, Rummer JL (2021) Investigating links between thermal tolerance and oxygen supply capacity in shark neonates from a hyperoxic tropical environment. Science of The Total Environment 782, 146854.
| Crossref | Google Scholar | PubMed |
Bouyoucos IA, Simpfendorfer CA, Planes S, Schwieterman GD, Weideli OC, Rummer JL (2022) Thermally insensitive physiological performance allows neonatal sharks to use coastal habitats as nursery areas. Marine Ecology Progress Series 682, 137-152.
| Crossref | Google Scholar |
Brooks ME, Kristensen K, van Benthem KJ, Magnusson A, Berg CW, Nielsen A, Skaug HJ, Mächler M, Bolker BM (2017) glmmTMB balances speed and flexibility among packages for zero-inflated generalized linear mixed modeling. The R Journal 9, 378-400.
| Crossref | Google Scholar |
Carlson JK, Parsons GR (2003) Respiratory and hematological responses of the bonnethead shark, Sphyrna tiburo, to acute changes in dissolved oxygen. Journal of Experimental Marine Biology and Ecology 294, 15-26.
| Crossref | Google Scholar |
Carrier JC, Pratt HL (1998) Habitat management and closure of a nurse shark breeding and nursery ground. Fisheries Research 39, 209-213.
| Crossref | Google Scholar |
Chin A, Kyne PM, Walker TI, McAuley RB (2010) An integrated risk assessment for climate change: analysing the vulnerability of sharks and rays on Australia’s Great Barrier Reef. Global Change Biology 16, 1936-1953.
| Crossref | Google Scholar |
Cinner JE, Maire E, Huchery C, MacNeil MA, Graham NAJ, Mora C, McClanahan TR, Barnes ML, Kittinger JN, Hicks CC, D’Agata S, Hoey AS, Gurney GG, Feary DA, Williams ID, Kulbicki M, Vigliola L, Wantiez L, Edgar GJ, Stuart-Smith RD, Sandin SA, Green A, Hardt MJ, Beger M, Friedlander AM, Wilson SK, Brokovich E, Brooks AJ, Cruz-Motta JJ, Booth DJ, Chabanet P, Gough C, Tupper M, Ferse SCA, Sumaila UR, Pardede S, Mouillot D (2018) Gravity of human impacts mediates coral reef conservation gains. Proceedings of the National Academy of Sciences 115, E6116-E6125.
| Crossref | Google Scholar |
Clementi GM, Babcock EA, Valentin-Albanese J, Bond ME, Flowers KI, Heithaus MR, Whitman ER, Van Zinnicq Bergmann MPM, Guttridge TL, O’Shea OR, Shipley ON, Brooks EJ, Kessel ST, Chapman DD (2021) Anthropogenic pressures on reef-associated sharks in jurisdictions with and without directed shark fishing. Marine Ecology Progress Series 661, 175-186.
| Crossref | Google Scholar |
Dabruzzi TF, Bennett WA (2013) Hypoxia effects on gill surface area and blood oxygen-carrying capacity of the Atlantic stingray, Dasyatis sabina. Fish Physiology and Biochemistry 40, 1011-1020.
| Crossref | Google Scholar | PubMed |
Dapp DR, Huveneers C, Walker TI, Reina RD (2017) Physiological response and immediate mortality of gill-net-caught blacktip reef sharks (Carcharhinus melanopterus). Marine and Freshwater Research 68, 1734-1740.
| Crossref | Google Scholar |
DeAngelis BM, McCandless CT, Kohler NE, Recksiek CW, Skomal GB (2008) First characterization of shark nursery habitat in the United States Virgin Islands: evidence of habitat partitioning by two shark species. Marine Ecology Progress Series 358, 257-271.
| Crossref | Google Scholar |
Debaere SF, Weideli OC, Bouyoucos IA, Eustache KB, Trujillo JE, De Boeck G, Planes S, Rummer JL (2023) Quantifying changes in umbilicus size to estimate the relative age of neonatal blacktip reef sharks (Carcharhinus melanopterus). Conservation Physiology 11, coad028.
| Crossref | Google Scholar |
Dubuc A, Collins GM, Coleman L, Waltham NJ, Rummer JL, Sheaves M (2021) Association between physiological performance and short temporal changes in habitat utilisation modulated by environmental factors. Marine Environmental Research 170, 105448.
| Crossref | Google Scholar | PubMed |
Dulvy NK, Pacoureau N, Rigby CL, Pollom RA, Jabado RW, Ebert DA, Finucci B, Pollock CM, Cheok J, Derrick DH, Herman KB, Sherman CS, VanderWright WJ, Lawson JM, Walls RHL, Carlson JK, Charvet P, Bineesh KK, Fernando D, Ralph GM, Matsushiba JH, Hilton-Taylor C, Fordham SV, Simpfendorfer CA (2021) Overfishing drives over one-third of all sharks and rays toward a global extinction crisis. Current Biology 31, 4773-4787.e8.
| Crossref | Google Scholar |
Dwyer RG, Campbell HA, Cramp RL, Burke CL, Micheli-Campbell MA, Pillans RD, Lyon BJ, Franklin CE (2020) Niche partitioning between river shark species is driven by seasonal fluctuations in environmental salinity. Functional Ecology 34, 2170-2185.
| Crossref | Google Scholar |
Eustache KB, Boissin É, Tardy C, Bouyoucos IA, Rummer JL, Planes S (2023) Genetic evidence for plastic reproductive philopatry and matrotrophy in blacktip reef sharks (Carcharhinus melanopterus) of the Moorea Island (French Polynesia). Scientific Reports 13, 14913.
| Crossref | Google Scholar | PubMed |
Eustache KB, van Loon E, Rummer JL, Planes S, Smallegange I (2024) Spatial and temporal analysis of juvenile blacktip reef shark (Carcharhinus melanopterus) demographies identifies critical habitats. Journal of Fish Biology 104, 92-103.
| Crossref | Google Scholar | PubMed |
Fobert E, Meining P, Colotelo A, O’Connor C, Cooke SJ (2009) Cut the line or remove the hook? An evaluation of sublethal and lethal endpoints for deeply hooked bluegill. Fisheries Research 99, 38-46.
| Crossref | Google Scholar |
Fodrie FJ, Levin LA, Lucas AJ (2009) Use of population fitness to evaluate the nursery function of juvenile habitats. Marine Ecology Progress Series 385, 39-49.
| Crossref | Google Scholar |
Frisch AJ, Rizzari JR (2019) Parks for sharks: human exclusion areas outperform no-take marine reserves. Frontiers in Ecology and the Environment 17, 145-150.
| Crossref | Google Scholar |
Froeschke JT, Stunz GW, Sterba-Boatwright B, Wildhaber ML (2010a) An empirical test of the ‘shark nursery area concept’ in Texas bays using a long-term fisheries-independent data set. Aquatic Biology 11, 65-76.
| Crossref | Google Scholar |
Froeschke J, Stunz GW, Wildhaber ML (2010b) Environmental influences on the occurrence of coastal sharks in estuarine waters. Marine Ecology Progress Series 407, 279-292.
| Crossref | Google Scholar |
George LW, Martins APB, Heupel MR, Simpfendorfer CA (2019) Fine-scale movements of juvenile blacktip reef sharks Carcharhinus melanopterus in a shallow nearshore nursery. Marine Ecology Progress Series 623, 85-97.
| Crossref | Google Scholar |
Hansell AC, Kessel ST, Brewster LR, Cadrin SX, Gruber SH, Skomal GB, Guttridge TL (2018) Local indicators of abundance and demographics for the coastal shark assemblage of Bimini, Bahamas. Fisheries Research 197, 34-44.
| Crossref | Google Scholar |
Hardiman N, Burgin S (2010) Recreational impacts on the fauna of Australian coastal marine ecosystems. Journal of Environmental Management 91, 2096-2108.
| Crossref | Google Scholar | PubMed |
Hasler CT, Midway SR, Jeffrey JD, Tix JA, Sullivan C, Suski CD (2016) Exposure to elevated pCO2 alters post-treatment diel movement patterns of largemouth bass over short time scales. Freshwater Biology 61, 1590-1600.
| Crossref | Google Scholar |
Heupel MR, Simpfendorfer CA (2005) Using acoustic monitoring to evaluate MPAs for shark nursery areas: the importance of long-term data. Marine Technology Society Journal 39, 10-18.
| Crossref | Google Scholar |
Heupel MR, Simpfendorfer CA (2008) Movement and distribution of young bull sharks Carcharhinus leucas in a variable estuarine environment. Aquatic Biology 1, 277-289.
| Crossref | Google Scholar |
Heupel MR, Carlson JK, Simpfendorfer CA (2007) Shark nursery areas: concepts, definition, characterization and assumptions. Marine Ecology Progress Series 337, 287-297.
| Crossref | Google Scholar |
Heupel MR, Kanno S, Martins APB, Simpfendorfer CA (2019) Advances in understanding the roles and benefits of nursery areas for elasmobranch populations. Marine and Freshwater Research 70, 897-907.
| Crossref | Google Scholar |
Jennings DE, Gruber SH, Franks BR, Kessel ST, Robertson AL (2008) Effects of large-scale anthropogenic development on juvenile lemon shark (Negaprion brevirostris) populations of Bimini, Bahamas. Environmental Biology of Fishes 83, 369-377.
| Crossref | Google Scholar |
Kinney MJ, Simpfendorfer CA (2009) Reassessing the value of nursery areas to shark conservation and management. Conservation Letters 2, 53-60.
| Crossref | Google Scholar |
Knip DM, Heupel MR, Simpfendorfer CA (2010) Sharks in nearshore environments: models, importance, and consequences. Marine Ecology Progress Series 402, 1-11.
| Crossref | Google Scholar |
Lea JSE, Humphries NE, von Brandis RG, Clarke CR, Sims DW (2016) Acoustic telemetry and network analysis reveal the space use of multiple reef predators and enhance marine protected area design. Proceedings of the Royal Society B: Biological Sciences 283, 20160717.
| Crossref | Google Scholar |
Lester E, Langlois T, Lindgren I, Birt M, Bond T, McLean D, Vaughan B, Holmes TH, Meekan M (2022) Drivers of variation in occurrence, abundance, and behaviour of sharks on coral reefs. Scientific Reports 12, 728.
| Crossref | Google Scholar | PubMed |
Little AG, Loughland I, Seebacher F (2020) What do warming waters mean for fish physiology and fisheries? Journal of Fish Biology 97, 328-340.
| Crossref | Google Scholar | PubMed |
Loiseau C, Thiault L, Devillers R, Claudet J (2021) Cumulative impact assessments highlight the benefits of integrating land-based management with marine spatial planning. Science of The Total Environment 787, 147339.
| Crossref | Google Scholar | PubMed |
Lowry M, Williams D, Metti Y (2007) Lunar landings – relationship between lunar phase and catch rates for an Australian gamefish-tournament fishery. Fisheries Research 88, 15-23.
| Crossref | Google Scholar |
MacNeil MA, Chapman DD, Heupel M, Simpfendorfer CA, Heithaus M, Meekan M, Harvey E, Goetze J, Kiszka J, Bond ME, Currey-Randall LM, Speed CW, Sherman CS, Rees MJ, Udyawer V, Flowers KI, Clementi G, Valentin-Albanese J, Gorham T, Adam MS, Ali K, Pina-Amargós F, Angulo-Valdés JA, Asher J, Barcia LG, Beaufort O, Benjamin C, Bernard ATF, Berumen ML, Bierwagen S, Bonnema E, Bown RMK, Bradley D, Brooks E, Brown JJ, Buddo D, Burke P, Cáceres C, Cardeñosa D, Carrier JC, Caselle JE, Charloo V, Claverie T, Clua E, Cochran JEM, Cook N, Cramp J, D’Alberto B, de Graaf M, Dornhege M, Estep A, Fanovich L, Farabaugh NF, Fernando D, Flam AL, Floros C, Fourqurean V, Garla R, Gastrich K, George L, Graham R, Guttridge T, Hardenstine RS, Heck S, Henderson AC, Hertler H, Hueter R, Johnson M, Jupiter S, Kasana D, Kessel ST, Kiilu B, Kirata T, Kuguru B, Kyne F, Langlois T, Lédée EJI, Lindfield S, Luna-Acosta A, Maggs J, Manjaji-Matsumoto BM, Marshall A, Matich P, McCombs E, McLean D, Meggs L, Moore S, Mukherji S, Murray R, Kaimuddin M, Newman SJ, Nogués J, Obota C, O’Shea O, Osuka K, Papastamatiou YP, Perera N, Peterson B, Ponzo A, Prasetyo A, Quamar LMS, Quinlan J, Ruiz-Abierno A, Sala E, Samoilys M, Schärer-Umpierre M, Schlaff A, Simpson N, Smith ANH, Sparks L, Tanna A, Torres R, Travers MJ, van Zinnicq Bergmann M, Vigliola L, Ward J, Watts AM, Wen C, Whitman E, Wirsing AJ, Wothke A, Zarza-Gonzâlez E, Cinner JE (2020) Global status and conservation potential of reef sharks. Nature 583, 801-806.
| Crossref | Google Scholar | PubMed |
Madi Moussa R, Fogg L, Bertucci F, Calandra M, Collin A, Aubanel A, Polti S, Benet A, Salvat B, Galzin R, Planes S, Lecchini D (2019) Long-term coastline monitoring on a coral reef island (Moorea, French Polynesia). Ocean & Coastal Management 180, 104928.
| Crossref | Google Scholar |
Matich P, Kiszka JJ, Mourier J, Planes S, Heithaus MR (2017) Species co-occurrence affects the trophic interactions of two juvenile reef shark species in tropical lagoon nurseries in Moorea (French Polynesia). Marine Environmental Research 127, 84-91.
| Crossref | Google Scholar | PubMed |
Morash AJ, Mackellar SRC, Tunnah L, Barnett DA, Stehfest KM, Semmens JM, Currie S (2016) Pass the salt: physiological consequences of ecologically relevant hyposmotic exposure in juvenile gummy sharks (Mustelus antarcticus) and school sharks (Galeorhinus galeus). Conservation Physiology 4, cow036.
| Crossref | Google Scholar |
Mourier J, Planes S (2013) Direct genetic evidence for reproductive philopatry and associated fine-scale migrations in female blacktip reef sharks (Carcharhinus melanopterus) in French Polynesia. Molecular Ecology 22, 201-214.
| Crossref | Google Scholar | PubMed |
Mourier J, Mills SC, Planes S (2013a) Population structure, spatial distribution and life-history traits of blacktip reef sharks Carcharhinus melanopterus. Journal of Fish Biology 82, 979-993.
| Crossref | Google Scholar | PubMed |
Mourier J, Buray N, Schultz JK, Clua E, Planes S (2013b) Genetic network and breeding patterns of a sicklefin lemon shark (Negaprion acutidens) population in the Society Islands, French Polynesia. PLoS ONE 8, e73899.
| Crossref | Google Scholar |
Mourier J, Maynard J, Parravicini V, Ballesta L, Clua E, Domeier ML, Planes S (2016) Extreme inverted trophic pyramid of reef sharks supported by spawning groupers. Current Biology 26, 2011-2016.
| Crossref | Google Scholar | PubMed |
Mourier J, Brown C, Planes S (2017) Learning and robustness to catch-and-release fishing in a shark social network. Biology Letters 13, 20160824.
| Crossref | Google Scholar | PubMed |
Nadon MO, Baum JK, Williams ID, McPherson JM, Zgliczynski BJ, Richards BL, Schroeder RE, Brainard RE (2012) Re-creating missing population baselines for pacific reef sharks. Conservation Biology 26, 493-503.
| Crossref | Google Scholar | PubMed |
Oh BZL, Sequeira AMM, Meekan MG, Ruppert JLW, Meeuwig JJ (2017a) Predicting occurrence of juvenile shark habitat to improve conservation planning. Conservation Biology 31, 635-645.
| Crossref | Google Scholar | PubMed |
Oh BZL, Thums M, Babcock RC, Meeuwig JJ, Pillans RD, Speed C, Meekan MG (2017b) Contrasting patterns of residency and space use of coastal sharks within a communal shark nursery. Marine and Freshwater Research 68, 1501-1517.
| Crossref | Google Scholar |
Parsons GR, Carlson JK (1998) Physiological and behavioral responses to hypoxia in the bonnethead shark, Sphyrna tiburo: routine swimming and respiratory regulation. Fish Physiology and Biochemistry 19, 189-196.
| Crossref | Google Scholar |
Porcher IF (2005) On the gestation period of the blackfin reef shark, Carcharhinus melanopterus, in waters off Moorea, French Polynesia. Marine Biology 146, 1207-1211.
| Crossref | Google Scholar |
Schlaff AM, Heupel MR, Simpfendorfer CA (2014) Influence of environmental factors on shark and ray movement, behaviour and habitat use: a review. Reviews in Fish Biology and Fisheries 24, 1089-1103.
| Crossref | Google Scholar |
Schlaff AM, Heupel MR, Udyawer V, Simpfendorfer CA (2017) Biological and environmental effects on activity space of a common reef shark on an inshore reef. Marine Ecology Progress Series 571, 169-181.
| Crossref | Google Scholar |
Sherman CS, Simpfendorfer CA, Pacoureau N, Matsushiba JH, Yan HF, Walls RHL, Rigby CL, VanderWright WJ, Jabado RW, Pollom RA, Carlson JK, Charvet P, Bin Ali A, Fahmi , Cheok J, Derrick DH, Herman KB, Finucci B, Eddy TD, Palomares MLD, Avalos-Castillo CG, Kinattumkara B, Blanco-Parra MP, Dharmadi , Espinoza M, Fernando D, Haque AB, Mejía-Falla PA, Navia AF, Pérez-Jiménez JC, Utzurrum J, Yuneni RR, Dulvy NK (2023) Half a century of rising extinction risk of coral reef sharks and rays. Nature Communications 14, 15.
| Crossref | Google Scholar | PubMed |
Simpfendorfer CA, Milward NE (1993) Utilisation of a tropical bay as a nursery area by sharks of the families Carcharhinidae and Sphyrnidae. Environmental Biology of Fishes 37, 337-345.
| Crossref | Google Scholar |
Simpfendorfer CA, Freitas GG, Wiley TR, Heupel MR (2005) Distribution and habitat partitioning of immature bull sharks (Carcharhinus leucas) in a southwest Florida estuary. Estuaries 28, 78-85.
| Crossref | Google Scholar |
Thiault L, Collin A, Chlous F, Gelcich S, Claudet J (2017) Combining participatory and socioeconomic approaches to map fishing effort in small-scale fisheries. PLoS ONE 12, e0176862.
| Crossref | Google Scholar |
Thiault L, Kernaléguen L, Osenberg CW, Lison de Loma T, Chancerelle Y, Siu G, Claudet J (2019) Ecological evaluation of a marine protected area network: a progressive-change BACIPS approach. Ecosphere 10, e02576.
| Crossref | Google Scholar |
Udyawer V, Chin A, Knip DM, Simpfendorfer CA, Heupel MR (2013) Variable response of coastal sharks to severe tropical storms: environmental cues and changes in space use. Marine Ecology Progress Series 480, 171-183.
| Crossref | Google Scholar |
Vierus T, Gehrig S, Brunnschweiler JM, Glaus K, Zimmer M, Marie AD, Rico C (2018) Discovery of a multispecies shark aggregation and parturition area in the Ba Estuary, Fiji Islands. Ecology and Evolution 8, 7079-7093.
| Crossref | Google Scholar | PubMed |
Ward-Paige CA (2017) A global overview of shark sanctuary regulations and their impact on shark fisheries. Marine Policy 82, 87-97.
| Crossref | Google Scholar |
Ward-Paige CA, Worm B (2017) Global evaluation of shark sanctuaries. Global Environmental Change 47, 174-189.
| Crossref | Google Scholar |
Yates PM, Heupel MR, Tobin AJ, Simpfendorfer CA (2015) Ecological drivers of shark distributions along a tropical coastline. PLoS ONE 10, e0121346.
| Crossref | Google Scholar |
Zuur AF, Ieno EN, Walker N, Saveliev AA, Smith GM (2009) ‘Mixed effects models and extensions in ecology with R.’ (Springer: New York, NY, USA) doi:10.1016/B978-0-12-387667-6.00013-0