Residency and movement patterns of juvenile yellowfin bream Acanthopagrus australis, stocked on estuarine artificial reefs
Alistair Becker


A
B
C
D
E
F
Abstract
Successful fish stocking relies upon releasing cohorts directly into habitat containing suitable supporting resources. To facilitate this, an emerging trend has seen fish released onto artificial reefs.
We compared the residency and movement patterns of juvenile sparids (yellowfin bream – Acanthopagrus australis), stocked on artificial reefs and natural habitat, to determine the suitability of incorporating artificial structures as release sites in future stocking programs.
Forty hatchery-reared yellowfin bream were evenly divided and stocked on either artificial reef or natural habitat within an east-Australian estuary, and tracked using acoustic telemetry.
Artificial reefs provided greater habitat complexity than the natural habitat and fish stocked onto these structures showed greater fidelity to their release site and were detected for longer periods of time than those stocked into less complex natural habitat.
The high residency levels of fish around artificial reef sites indicate they contain resources to support stocked juvenile sparids.
Stocked yellowfin bream can tolerate a range of salinity and temperatures, allowing flexibility in stocking programs. Future stocking of bream or other sparids should consider releasing fish onto artificial reefs where available. Additionally, the deployment of reefs could aid existing stocking programs.
Keywords: acoustic telemetry, estuary, fish stocking, habitat use, home range, movement ecology, residency, stock enhancement.
Introduction
Augmenting recruitment-limited fish stocks with hatchery-reared individuals is increasingly being used as part of a management strategy to enhance recreational fisheries within coastal ecosystems (Lorenzen et al. 2010; Loneragan et al. 2013). The success of these programs is mixed, with deficiencies in the availability of suitable habitat during releases identified as a key issue (Bell et al. 2005). Identifying habitats which best support released fish, and targeting these areas with stocking efforts, is essential to maximise survival and associated benefits (Lorenzen 2014).
Identifying habitats that maximise survival of stocked fish can be achieved through pilot stocking programs accompanied with intensive monitoring (Loneragan et al. 2001; Taylor et al. 2006). Recently, acoustic telemetry has been employed in pilot stocking programs to track and identify the habitat preferences of released fish (Yokota et al. 2006; Fairchild et al. 2009; Becker et al. 2021). These studies can measure movements within mosaic habitats and identify putative drivers of these patterns (e.g. salinity and temperature), alongside monitoring of broadscale movements in the wider system (Kawabata et al. 2010; Noda et al. 2019). The resultant models support refinement of release strategies to match environmental conditions within the receiving waterway, but users have not often considered that models may be selecting the ‘best’ habitat from among a range of poor habitats. By monitoring the movement and survival of fishes released in degraded or unaltered habitats compared to fish released in restored habitats, we can assess whether fish stocking programs may be more successful if combined with habitat restoration or enhancement.
An emerging method to increase and enhance habitat for fish is the deployment of artificial reefs (D’Anna et al. 2004). The design of artificial reef modules and their layout can be matched to the requirements of particular species (Becker et al. 2018) making them a useful tool for targeted fisheries enhancements. The combination of fish stocking and artificial reefs is being increasingly considered to maximise the outcomes for target species, with some examples of impressive outcomes emerging. For instance, Liu et al. (2022) documented progress in China where over US$1.2 billion has been invested in sea ranching, which largely involves stocking artificial reefs, and of 108 research projects undertaken on the activity, 93% reported positive effects.
Sparids are among the most commonly stocked species into marine waters, with large-scale programs releasing species such as Black Sea bream (Acanthopagrus schlegelii) and Red Sea bream (Pagrus major) in Japan (Gonzalez et al. 2008; Kitada 2018), gilthead bream (Sparus aurata) in Spain (Sánchez-Lamadrid 2002) and black bream (Acanthopagrus butcheri) in Western Australia (Cottingham et al. 2015). In New South Wales (NSW) on the east coast of Australia, yellowfin bream (Acanthopagrus australis) are the most commonly caught fish by recreational anglers (Murphy et al. 2022) and have been identified as a priority for release into recruitment-limited estuaries (Blount et al. 2017). Previous telemetry studies have shown that wild yellowfin bream make extensive use of reef balls as home territories (Lowry et al. 2017; Taylor et al. 2018), suggesting that incorporating these structures into future stocking programs could increase survival.
To evaluate the potential of stocking sparids in NSW estuaries, we tracked acoustically tagged, hatchery-reared yellowfin bream released on both artificial reefs and other natural habitat and evaluated their movements over a 6-month study period. Specifically, we aimed to determine if residency of released fish on artificial reefs was higher than natural habitat. Secondly, we evaluated the space used by fish, their position along the estuary, and how environmental conditions influenced these patterns. This new information is used to provide recommendations and strategies for future release programs.
Methods
Site description and acoustic array
The Bellinger–Kalang estuarine system is located on the mid-north coast of NSW (30.49°S, 153.03°E), Australia. The estuarine system collectively consists of two riverine branches (Bellinger and Kalang) which converge 500 m from the ocean and share a single mouth (Fig. 1). The Bellinger is the larger of the two, with the river extending 109 km and has a larger catchment, covering 770 km2. The Kalang is shorter, 77 km long and with a catchment of 330 km2.
Map of the Bellinger–Kalang estuarine system. Blue triangle shows the artificial reef group release location and the green triangle the natural habitat group, black dots show the acoustic receiver stations (St.1–St.18), and the black stars are the locations of the water quality loggers. Insert photograph shows an example of the artificial reef type deployed in the Bellinger–Kalang estuary (note: this photograph is from another NSW estuary).
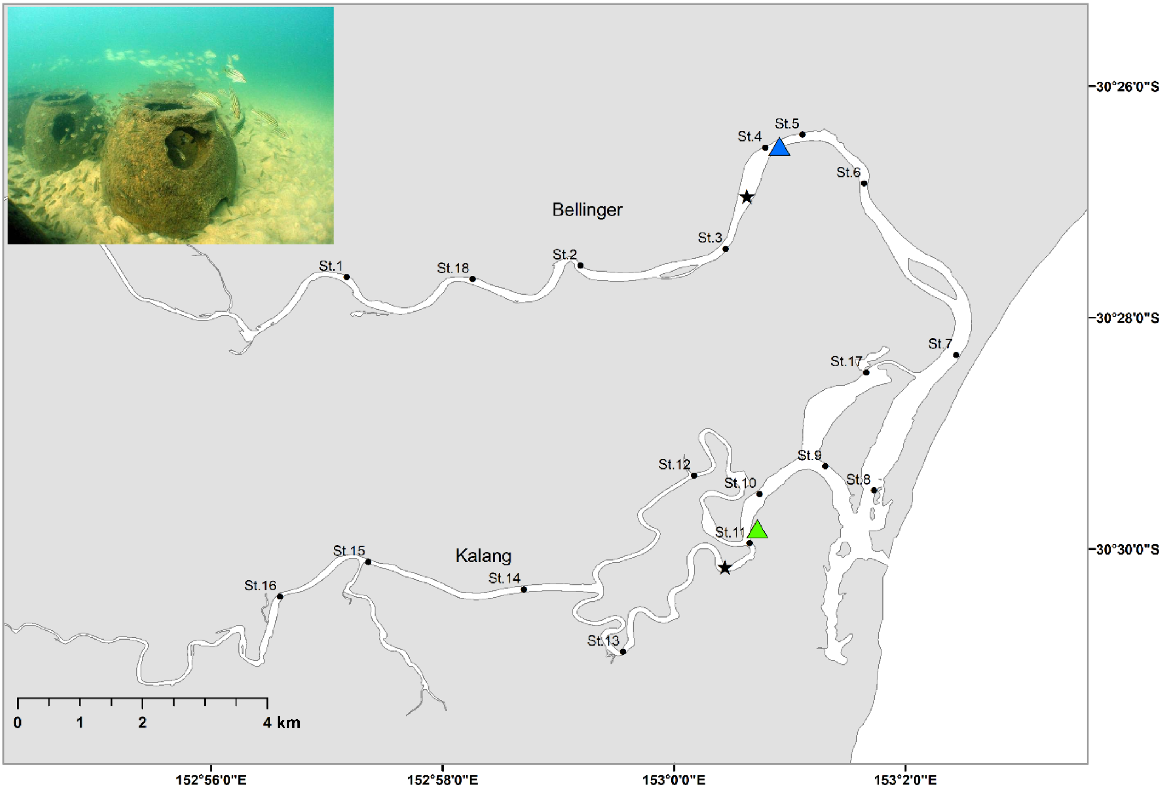
An array of 18 stations was deployed within the system, consisting of 10 acoustic receivers (VR2-AR 69 kHz, Innovasea Pty Ltd) within the Bellinger branch, and eight within the Kalang. Depths of the receivers ranged between 3.5 and 6 m, although most were 4 m deep. Receivers were spread from the lower to upper reaches of each branch of the system, with a minimum of 700 m between any two stations (Fig. 1), meaning their approximate detection range (300–400 m) for our choice of acoustic transmitter and power output did not overlap. The riverine shape of the estuary allowed detections of fish movement up and down the system as they passed each station. A single Hobo U24-002-C (Onset, Cape Cod, MA, USA) conductivity and temperature data logger was placed in the middle reaches of each branch and was set to record data at hourly time intervals. The data loggers were calibrated at the beginning and at the mid-point of the study with spot readings of temperature and salinity using a Horiba U-53G.
Fish production and tagging
The study focused on juvenile yellowfin bream as stocking programs generally release fish at this life-history stage. The juvenile yellowfin bream were produced at the National Marine Science Centre (NMSC) at Coffs Harbour, 25 km north of the Bellinger–Kalang estuary. Twenty broodfish were collected from the population that inhabits the Bellinger–Kalang system and permanently held at the NMSC in 3000-L tanks supplied with flowthrough seawater and maintained on a mix of artificial conditioning diet (Ridley) and fresh squid, pilchards, and prawns. Broodfish were induced to spawn in late winter by the injection of LHRHa. Fish spawned within 36 h of injection in the early evening and continued to spawn for four evenings. Eggs from one of these spawns were collected and hatched in a 100-L hatching tank. Larvae were stocked 24 h after hatching (~10 larvae L−1) in 1000-L larval rearing tanks indoors (8:16 dark:light, cool white fluorescent) containing seawater (23°C, >96% DO) that was tinted with a mixture of the microalgae Nannochloropsis spp. (both live and preserved depending on availability) and Proteomonas sulcata. From day 3 post hatch, larvae were fed rotifers (~5 individuals mL−1). At day 14 post hatch, flowthrough seawater was supplied to the tanks (~3 exchanges per day) and the larvae were offered artemia that had been fed a commercial lipid emulsion (Selco). Fish were weaned onto an artificial diet (Ridley) by week 6 post hatch, at which point they were transferred to outdoor tanks (3000-L) supplied with flowthrough seawater where they were maintained under ambient conditions until they were used in the experiments.
Forty juvenile (166–197-mm total length, TL) fish were tagged with V7-4L acoustic transmitters (Innovasea Pty Ltd), programmed to transmit with a nominal delay (period between transmissions) of 60–120 s, providing 237 days of battery life (Supplementary Table S1). Prior to any surgical procedures, fish were anesthetised using a 60 mg L−1 dose of Aqui-S for >10 min, with fish determined to be suitable for surgery once a complete loss of equilibrium was observed, accompanied by a lack of reaction to external stimuli. Fish were weighed (±0.1 g) and measured (TL ± 1 mm) before a 15–20-mm incision was made along the ventral mid-line into the peritoneal cavity. The transmitter was inserted, and the incision closed using two absorbable sutures tied with a double surgeon’s knot. Post-surgery fish were placed into 3000-L tanks with flowthrough seawater and left to recover for 2 weeks. No fish died during the recovery period, nor were any tags ejected.
Fish were haphazardly divided into two groups of 20 individuals each, with one group released onto artificial reefs, and the other into natural habitat (Fig. 1). The artificial reef field consisted of 100 ‘reef balls’; each structure is dome-shaped and constructed from concrete, with a height of 0.5 m and diameter of 0.7 m (see inset Fig. 1). The units are hollow and include internal voids, creating a complex shape within which fish can swim. The artificial reefs were well established having been deployed in 2007. Acoustic receiver stations 4 and 5 were located within artificial reef fields. The natural habitat included a mix of mangrove, mud banks and natural rocky reef, so did not consist of only one type of structure. The natural rocky reef consisted of a mix of boulders and cobbles, but lacked the vertical profile provided by the artificial reef. Mangrove root systems afforded an additional complex structure, but there were sections of bare mud or sand often separating the mangroves and rocky substrate. The natural release site can be considered typical of the habitat along much of the estuary and were mostly continuous along intertidal and subtidal fringes of the estuary. This natural habitat also flanked either side of the artificial reef sites and could be found <100 m from the edge of the reef field. As such, it was only the addition of artificial reefs which distinguished those sections of the estuary from the rest of the system. Both groups of fish were of a similar size (artificial reef group: mean TL = 179 mm ± 8.4 s.d.; natural habitat group: mean total length = 181 mm ± 8.0 s.d.). Stocking could not logistically be completed in a single day; therefore, the artificial reef group was released on 17 November 2022, while the natural habitat group was released on 24 November 2022. Travel time to both locations was 1 h and no mortalities were recorded during transportation and release. The study concluded on 2 May 2023, when all receivers were removed from the estuary and detection data downloaded.
Ethics
Experimental work was conducted under NSW Department of Primary Industries Animal Ethics approval 14/01; rearing fish was done under Southern Cross University Animal Care and Ethics Committee approvals 21/002, 22/002 and 23/019.
Data analysis
Detection data from the receivers was initially collated using the Vemco User Environment (VUE) software package (ver. 2.7.0, Innovasea Pty Ltd) before being exported to R (ver. 4.3.1, R Foundation for Statistical Computing, Vienna, Austria, see https://www.r-project.org/) for further analysis. High, continual detection rates at single stations may imply mortalities of tagged fish. However, in this study, even fish which were detected almost continually at some stations, were also regularly recorded visiting nearby stations. Considering the detection range of the V7 transmitters, we designed the acoustic array with no overlap in the detection area of any two stations. We therefore believe high detection rates at single stations represent true resident behaviour and fish were deemed alive for the duration of the study.
Addressing the first objective of the study, the residency of fish at each station was calculated using a weighted residency index, which reduces the residency estimation of fish with few but consecutive detections at a station (Kraft et al. 2023). For each fish on each station, the index ranges between 0 and 1, reflecting the amount of time an individual spent at a particular station over the study period, with 0 indicating no residency and 1 full residency. The index is given by:
where Dd is the number of days a fish was detected, Dt was the total number of monitoring days, and Di is the number of days between the first and last detection for each fish.
The final objectives of the study related to space use and position of fish within the estuarine system. To resolve these patterns, raw detection data were used to compile fish tracks, consisting of interpolated positions based on consecutive receiver detections, taking into consideration the morphological constraints imposed by the shape of the water body. To create the tracks, we first screened the detection data using the actel package (ver. 1.3.0, see https://cran.r-project.org/package=actel; Flávio and Baktoft 2021), before using the refined shortest paths (‘RSP’) package (ver. 1.0.5, see https://github.com/YuriNiella/RSP; Niella et al. 2020) to estimate the interpolated positional data. Because of the winding characteristics of the estuary, we specified interpolated distances of 100 m along the tracks for the simulations. Based on the fish tracks, daily utilisation distributions were then calculated for each fish group (artificial reefs and natural habitat) using dynamic Brownian Bridge Movement Models (dBBMM). This provided an estimated core (50%) daily space use (m2) for each group over the monitoring period. We chose to employ core-space use rather than total (95%), as it provides a more precise picture of key habitat and regions. Not all fish were detected each day, with fewer fish detected daily towards the end of the monitoring period. This can bias space use estimates; therefore, we standardised core daily space use by dividing the area by the number of fish detected from each group. We then evaluated the relationship between daily space use (m2) and environmental variables including salinity (PSU) and temperature (°C), as well as the effect of ‘days at liberty’ (days), using generalised additive models (GAMs) with the mgcv package (ver. 1.9.1, S. Wood, see https://cran.r-project.org/package=mgcv/; Wood 2011). We selected the GAM approach as exploration of the data revealed clear non-linear relationships. The model was fit using a Gaussian distribution and identity link, with separate models fitted for fish released onto artificial reefs and natural habitat. The three explanatory variables were modelled using thin-plate regression splines, and Wald tests were used to identify variables with significant effects (P > 0.05) on space use. The deviance explained was used to assess the final model fit (Zuur et al. 2009).
Finally, we evaluated the effect of environmental variables and days at liberty on the location of fish along the length of each estuary. For each day during the study period, we calculated the average distance from the estuary mouth based upon which stations fish were detected, with the two release groups again considered separately. Average daily distance from the mouth (m) was then modelled against salinity, temperature, and days at liberty using GAMs, with the same model structure as the space use models.
Results
Environmental conditions
Both the Bellinger and Kalang branches of the estuary experienced similar temperature regimes which followed a predictable increase during the Austral summer period (Fig. 2). Salinity profiles in both branches also temporally responded to increased flow from their respective catchments, although salinity was generally lower in the Bellinger (Fig. 2).
Detection data and residency
All 40 tagged fish were detected during the study period (November 2022–May 2023), although fish released onto the artificial reef generally had higher detection rates than those released into the natural habitat (Fig. S1 and S2). Most fish released onto artificial reefs were still detected on multiple stations in the final weeks of the study period. Fish released into natural habitat generally had shorter detection periods, and detections were more sporadic. Fish were recorded on all stations except for Station 18 in the upper sections of the Bellinger (Table S1, S2), although fish were regularly detected further upstream at Station 1 (Fig. S1).
The highest residency indices were recorded at the stations at which fish were released (Fig. 3, Tables S1 and S2). However, higher residency was recorded at artificial reefs including Station 4 (release location) and Station 5 compared to all others. Within the Bellinger there were low residency indices at all stations downstream from Station 5. In the Kalang, residency indices for stations upstream of the release location (Station 11) were noticeably lower than in more downstream sections of the estuary.
Mean residency index at each of the stations (±s.e.), stations for fish within the artificial reef, and natural habitat groups. The dotted green line shows the release station for the artificial reef group and the dotted red line shows the station at which fish were released for the natural habitat group.
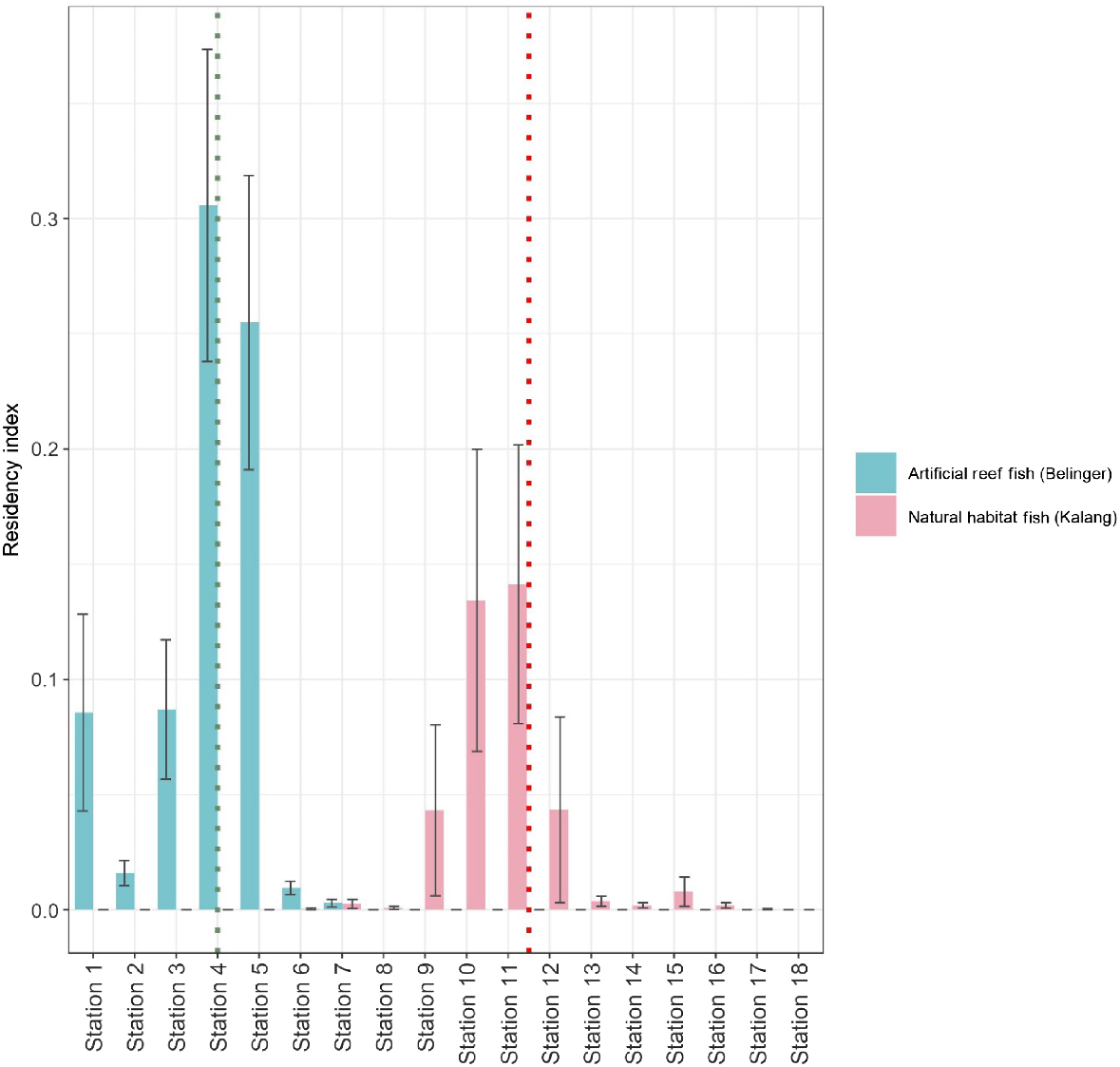
Space use
Generally, space use (standardised by number of detected fish) by fish released into each habitat was similar until late January 2023, when the area utilised by fish released onto artificial reefs became greater (Fig. 4). Towards the end of the study period in April 2023, again the daily space use by both groups was similar. The greatest area recorded was by fish stocked into the natural habitat, soon after fish were released. For both groups, space use changed significantly through time (days at liberty) but this was unrelated to salinity or temperature (Table 1, Fig. 5). Core space use by the artificial reef group increased then fluctuated before declining 100–150 days post release (Fig. 5). There was a reasonable level of variability around the fit for each explanatory variable, and overall, the model explained 25% of the total deviance. For fish released into natural habitat, space use declined until ~100 days at liberty, at which point space use stabilised and perhaps increased marginally at the end of the study (Table 1, Fig. 5). The model of space use for this group had a better overall fit, explaining 35% of the total deviance.
Core (50%) daily space use (m2) for each fish group during the study period. For example, a value of 10,000 m2 means that detections for an average fish (in a given release group) were spread across a core area of 10,000 m2. These can be interpreted as space use by an average fish, because space use was standardised by dividing the area by the daily number of fish detected within the array.
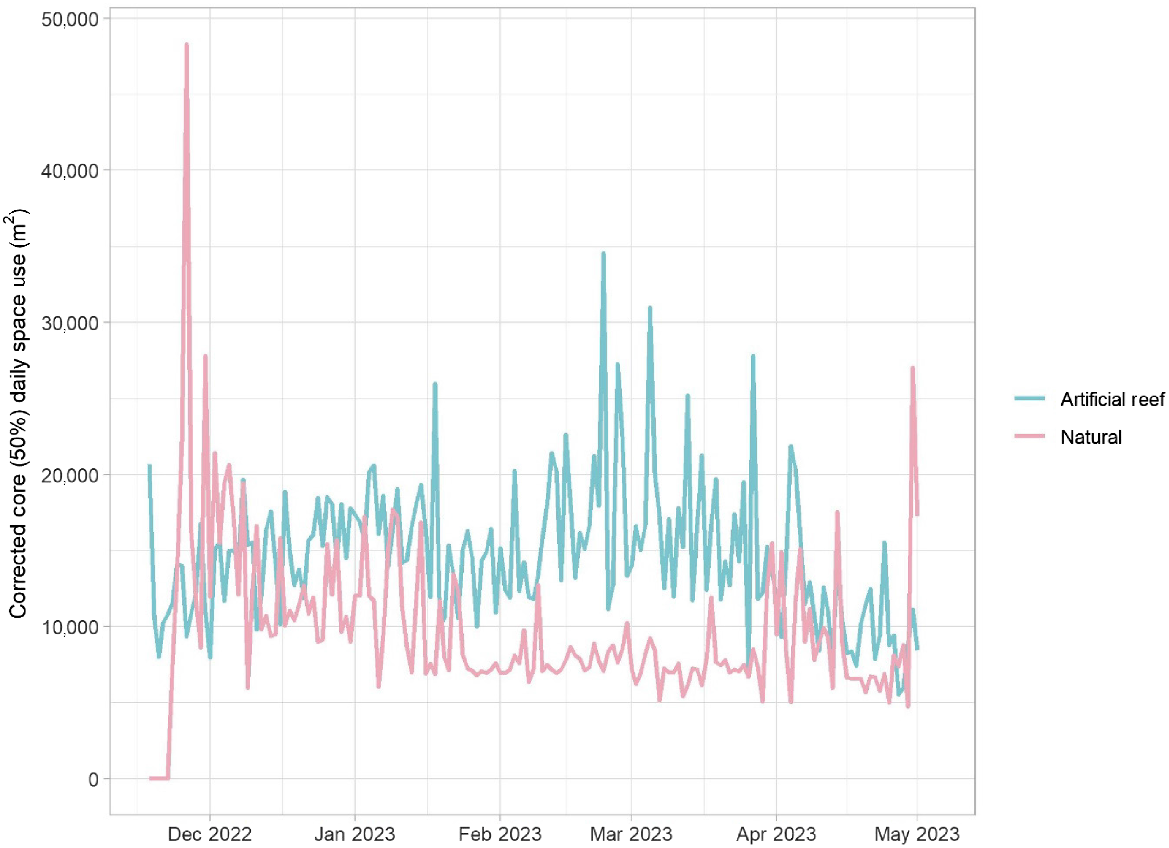
Group | Variable | Estimate | s.e. | d.f.e | d.f.ref | P | |
---|---|---|---|---|---|---|---|
Artificial reef | Intercept | 14,928.2 | 335.3 | ||||
s(salinity) | 1 | 1.002 | 0.642 | ||||
s(temp) | 1 | 1.001 | 0.338 | ||||
s(days liberty) | 4.548 | 5.637 | 0.042 | ||||
Total deviance explained = 24.6% | |||||||
Natural | Intercept | 10,144 | 337 | ||||
s(salinity) | 2.233 | 2.785 | 0.377 | ||||
s(temp) | 1.012 | 1.023 | 0.754 | ||||
s(days liberty) | 3.364 | 4.306 | <0.001 | ||||
Total deviance explained = 34.6% |
Significant effects are shown in bold. d.f.e, effective degrees of freedom; d.f.ref, reference degrees of freedom.
Partial effects of significant smooth terms for the generalised additive model of standardised core space use (50% contour) for fish released within the Bellinger and Kalang estuaries. Model predictions are plotted on the scale of the response variable; shaded areas show 95% confidence region.
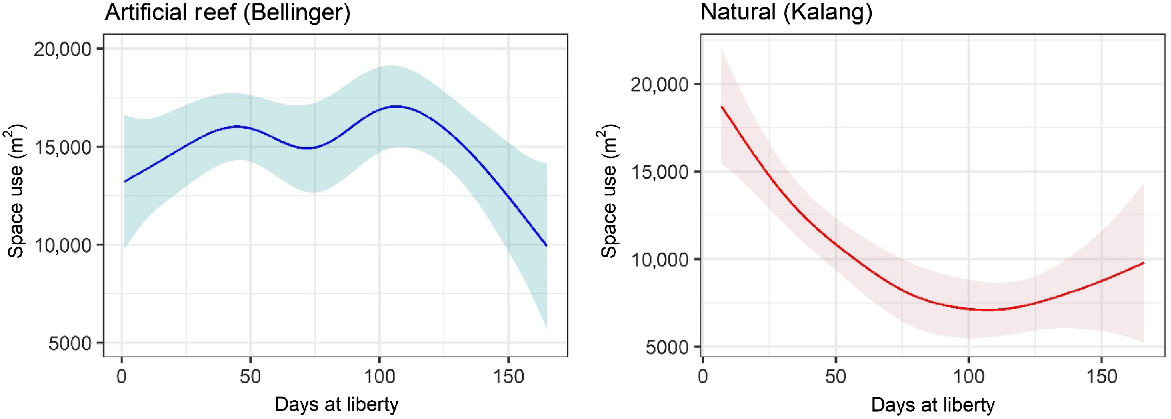
Along-estuary distribution
The average daily distance of fish from the estuary mouth showed differing patterns between the artificial reef and natural habitat groups. Fish on the artificial reef gradually moved further up the system during the study period (Fig. 6, Table 2). However, the upstream movement was modest in overall distance, with an average position 9 km from the mouth at the start of the study (release location) and ~11 km by the end (Fig. 6). There was also an effect of temperature, with increasing temperatures associated with an upstream shift by the tagged fish (Fig. 6, Table 2). For the natural habitat group, only salinity was found to influence the average position of fish, with increasing salinities associated with a downstream shift by tagged fish, although this shift was only ~1 km across the range of salinities recorded (Fig. 6, Table 2).
Partial effects of significant smooth terms for the generalised additive model of average daily distance from the estuary mouth for fish released within the Bellinger and Kalang estuaries. Model predictions are plotted on the scale of the response variable; shaded areas show 95% confidence region.
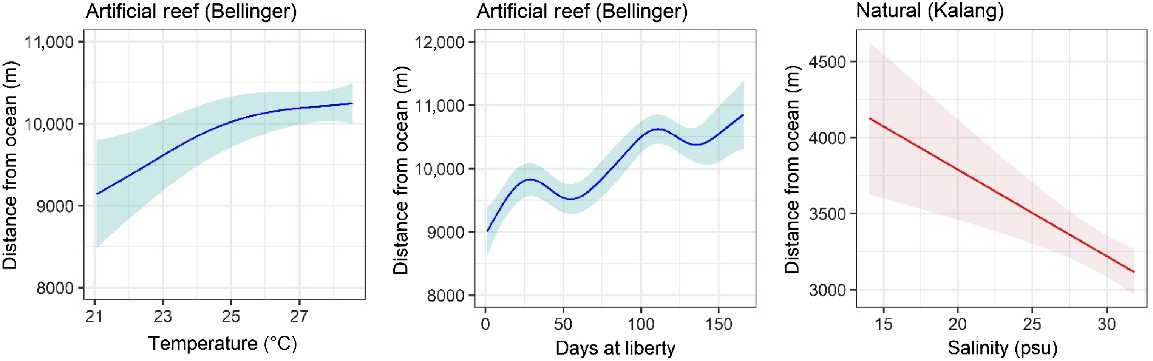
Group | Variable | Estimate | s.e. | d.f.e | d.f.ref | P | |
---|---|---|---|---|---|---|---|
Artificial reef | Intercept | 9903 | 30.89 | ||||
s(salinity) | 1.761 | 2.208 | 0.539 | ||||
s(temp) | 2.367 | 4.531 | 0.004 | ||||
s(days liberty) | 6.750 | 7.839 | <0.001 | ||||
Total deviance explained = 59.1% | |||||||
Natural | Intercept | 3386 | 35.76 | ||||
s(salinity) | 1 | 1 | <0.001 | ||||
s(temp) | 1.004 | 1.007 | 0.255 | ||||
s(days liberty) | 2.028 | 2.707 | 0.074 | ||||
Total deviance explained = 18.5% |
Significant effects are shown in bold. d.f.e, effective degrees of freedom; d.f.ref, reference degrees of freedom.
Discussion
Residency and artificial reef use
Previous studies tracking movements of hatchery-reared fish after release have shown mixed patterns. Whereas some found fish dispersing rapidly from release locations (D’Anna et al. 2004; Eder et al. 2015; Wada et al. 2017), including sparids (Ducos et al. 2022), others recorded fish remaining relatively resident at the release location for weeks to months (Kawabata et al. 2007, 2010; Lee et al. 2015). The yellowfin bream released into the Bellinger–Kalang showed high levels of residency to the release location, particularly those released onto artificial reefs, despite their proximity to nearby natural habitat such as rubble reef and mangroves. Several fish on the artificial reefs were detected at the release location for the duration of the study, making only occasional brief movements to nearby stations. While this pattern was also observed for the natural habitat group, it involved fewer fish. The disparity between the artificial reef and natural habitat group may be due to the quality of habitat at the release site. Sturgeon released into sub-optimal habitat disperse faster than those stocked into habitat typically occupied by juveniles (Eder et al. 2015). Brown meagre (Sciaena umbra) similarly dispersed to habitat typically occupied by wild juveniles (Ducos et al. 2022). Several studies on wild yellowfin bream have found them to show high site fidelity, with small home ranges (Pollock 1982; Gannon et al. 2015; van der Meulen et al. 2023), including on artificial reefs (Lowry et al. 2017). Patterns observed in our study suggest yellowfin bream may disperse when released into sub-optimal habitat, but if released onto complex structure containing shelter, may show high site fidelity as observed in wild conspecifics. The most complex structure in the Bellinger consisted of artificial reefs, which had been in place for over 15 years; indeed, they were installed due to a lack of this type of habitat. However, the yellowfin bream may have shown similar fidelity had there been comparable natural reef available.
The suitability of release habitat is critical in ensuring that released fish have maximal survival and productivity (Lorenzen et al. 2010; Lorenzen 2014). We found the two stations which covered the artificial reef field also had the highest residency indices. Residency indices for the natural habitat group were lower, and detection data suggest fish dispersed to a greater extent. When excluding the first week of the study (when fish were stocked on the artificial reef), all but one fish released onto the artificial reef were recorded at stations containing the reef ball structures. In other studies, yellowfin bream showed higher levels of residency around artificial reef balls in a large NSW estuary, with acoustically tagged fish detected within the reef field for periods spanning over a year (Lowry et al. 2017). A more detailed tracking study at the same location suggested the complex artificial reef structure may provide critical refuge for yellowfin bream as they foraged in adjacent areas (Taylor et al. 2018). Additionally, the deployment of artificial reefs in estuaries is believed to help sustain increased populations of sparids (Folpp et al. 2020), pointing to the resources they provide. Future stocking programs of sparid species would likely benefit if any available artificial reef habitat were incorporated into release plans.
Movement patterns of yellowfin bream
Despite high levels of residency exhibited by the stocked fish, they moved around the system to varying degrees. Days at liberty had the greatest effect on the size of core area used, although the pattern differed between the two release groups. As mentioned above, fish released in natural habitat appeared to disperse to a greater extent, which may explain the high space use during the early portion of the study. It is not unusual for stocked fish to display high levels of movement activity soon after release, as they seek refuge and foraging habitat (Taylor et al. 2013; Klinard et al. 2020). Afterwards, home ranges become established around optimised locations, reducing space use by the group of fish. During the initial parts of the study, space use of the artificial reef group was lower than the natural habitat group, which may be attributed to the resources provided by the artificial reef habitat into which fish were released.
The increased space use over time observed in the artificial reef group coincided with a gradual upstream shift in the average distance of fish from the mouth. This was driven by some fish moving towards the stations in the far upper reaches of the estuary, while those that remained continued to display high residency at artificial reef stations. Partial migration in estuarine fish is not uncommon (Kerr et al. 2009) and has been documented in yellowfin bream (van der Meulen et al. 2023) as well as black bream, a congener of yellowfin bream (Gillanders et al. 2015). Increasing temperature also resulted in the increasing likelihood of fish being detected further upstream, so the movement patterns may be related to seasonal cycles. Unfortunately, the small tags used in this study have limited battery life and precluded monitoring periods spanning the multiple years required to fully consider patterns across seasonal scales.
We found salinity had no effect on the position of the artificial reef group, but a negative relationship was found with increasing salinity and distance from the estuary mouth for the natural habitat group. Differences between the two groups may be partly attributed to some differences in salinity between the Bellinger and Kalang branches. However, movement patterns by both groups in relation to salinity contrast with telemetry studies on both yellowfin bream (van der Meulen et al. 2023) and black bream (Williams et al. 2017), which found fish often moved downstream in response to flow events. Salinity dropped to levels in both branches which elicited responses by wild yellowfin and black bream. The relationship observed by the natural habitat group was largely due to a few data points during an unusually low salinity period, during which fish remained near their release location. Although statistically significant, the overall effect was small, with distance from the mouth varying by only 1 km across the salinity range, while receiver array covered over 13 km of estuary length. In contrast to the hatchery-grown bream in this study, wild fish appear to make larger moves between 5 and 8 km downstream in response to similar reductions in salinity (Williams et al. 2017; van der Meulen et al. 2023). Fish in this study were also juveniles, whereas the tracking study by both van der Meulen et al. (2023) and Williams et al. (2017) included adult fish, and perhaps ontogeny influences the reaction of these fish to flow events. We only deployed a single salinity sensor within each branch of the system, as we were mainly concerned with the response of yellowfin bream to major flow events which would affect the whole system. However, a single station is unlikely to reflect the salinity conditions along the length of the Bellinger–Kalang, limiting the resolution of our dataset which may also explain the lack of any clear relationship between fish positions and salinity.
Conclusion
This was the first time that hatchery-reared yellowfin bream have been evaluated, and the patterns presented thus represent the first investigation of behaviour of released fish. Stocked yellowfin bream showed high levels of site fidelity, particularly when released onto estuarine artificial reefs, suggesting that these habitats provide suitable habitats for hatchery-reared juveniles in much the same way as observed for wild individuals. This study was limited to a single artificial reef and patterns may vary in other systems, particularly on recently installed structures or when other equally complex natural habitat is available, offering an avenue to further pursue this line of research. Although this study focussed on yellowfin bream, it is possible that estuarine artificial reefs represent good release habitat for other sparid species, and this may be an avenue for future research.
Data availability
The data that support this study will be shared upon reasonable request to the corresponding author.
Declaration of funding
Funding for this work was provided to Alistair Becker and Mathew D. Taylor by the NSW Recreational Fishing Trust.
Acknowledgements
We thank Brad Leach for assisting the tagging of fish, deploying acoustic receivers and curating the water quality data, and Clay Hilbert for assisting with releasing fish. Production of fish was assisted by Dan Mathews and SCU undergraduate students. We thank the fisheries managers Michael Gamble and Jim Harnwell who supported the development of this project.
References
Becker A, Taylor MD, Folpp H, Lowry MB (2018) Managing the development of artificial reef systems: the need for quantitative goals. Fish and Fisheries 19(4), 740-752.
| Crossref | Google Scholar |
Becker A, Lowry MB, Fielder DS, Taylor MD (2021) Dispersal of yellowtail kingfish (Seriola lalandi) from a coastal embayment following a recreational fisheries enhancement stocking program: attempts to integrate aquaculture and habitat-based initiatives. Bulletin of Marine Science 97, 615-630.
| Crossref | Google Scholar |
Bell JD, Rothlisberg PC, Munro JL, Loneragan NR, Nash WJ, Ward RD, Andrew NL (2005) Restocking and stock enhancement of marine invertebrate fisheries. Advances in Marine Biology 49, 1-370.
| Google Scholar |
Blount C, O’Donnell P, Reeds K, Taylor MD, Boyd S, Van derWalt B, McPhee DP, Lincoln Smith M (2017) Tools and criteria for ensuring estuarine stock enhancement programs maximise benefits and minimise impacts. Fisheries Research 186, 413-425.
| Crossref | Google Scholar |
Cottingham A, Hall NG, Potter IC (2015) Performance and contribution to commercial catches and egg production by restocked Acanthopagrus butcheri (sparidae) in an estuary. Estuarine, Coastal and Shelf Science 164, 194-203.
| Crossref | Google Scholar |
Ducos S, Garcia J, Mourier J, Aiello A, Durieux EDH (2022) Let them go free: spatial behaviour following the experimental release of hatchery-reared juveniles Sciaena umbra and Dentex dentex. Marine Environmental Research 181, 105712.
| Crossref | Google Scholar | PubMed |
D’Anna G, Giacalone VM, Badalamenti F, Pipitone C (2004) Releasing of hatchery-reared juveniles of the white seabream Diplodus sargus (L., 1758) in the Gulf of Castellammare artificial reef area (NW Sicily). Aquaculture 233(1–4), 251-268.
| Crossref | Google Scholar |
Eder BL, Steffensen KD, Haas JD, Adams JD (2015) Short-term survival and dispersal of hatchery-reared juvenile pallid sturgeon stocked in the channelized missouri river. Journal of Applied Ichthyology 31(6), 991-996.
| Crossref | Google Scholar |
Fairchild EA, Rennels N, Howell H (2009) Using telemetry to monitor movements and habitat use of cultured and wild juvenile winter flounder in a shallow estuary. In ‘Tagging and tracking of marine animals with electronic devices’. (Eds JL Nielsen, H Arrizabalaga, N Fragoso, A Hobday, M Lutcavage, J Sibert) pp. 5–22. (Springer Netherlands: Dordrecht, Netherlands)
Flávio H, Baktoft H (2021) actel: standardised analysis of acoustic telemetry data from animals moving through receiver arrays. Methods in Ecology and Evolution 12, 196-203.
| Crossref | Google Scholar |
Folpp HR, Schilling HT, Clark GF, Lowry MB, Maslen B, Gregson M, Suthers IM (2020) Artificial reefs increase fish abundance in habitat-limited estuaries. Journal of Applied Ecology 57, 1752-1761.
| Crossref | Google Scholar |
Gannon R, Payne NL, Suthers IM, Gray CA, van der Meulen DE, Taylor MD (2015) Fine-scale movements, site fidelity and habitat use of an estuarine dependent sparid. Environmental Biology of Fishes 98(6), 1599-1608.
| Crossref | Google Scholar |
Gillanders BM, Izzo C, Doubleday ZA, Ye Q (2015) Partial migration: growth varies between resident and migratory fish. Biology Letters 11, 20140850.
| Crossref | Google Scholar | PubMed |
Gonzalez EB, Umino T, Nagasawa K (2008) Stock enhancement programme for black sea bream, Acanthopagrus schlegelii (bleeker), in Hiroshima Bay, Japan: a review. Aquaculture Research 39(12), 1307-1315.
| Crossref | Google Scholar |
Kawabata Y, Okuyama J, Mitamura H, Asami K, Yoseda K, Arai N (2007) Post-release movement and diel activity patterns of hatchery-reared and wild black-spot tuskfish Choerodon schoenleinii determined by ultrasonic telemetry. Fisheries Science 73(5), 1147-1154.
| Crossref | Google Scholar |
Kawabata Y, Okuyama J, Asami K, Okuzawa K, Yoseda K, Arai N (2010) Effects of a tropical cyclone on the distribution of hatchery-reared black-spot tuskfish Choerodon schoenleinii determined by acoustic telemetry. Journal of Fish Biology 77(3), 627-642.
| Crossref | Google Scholar | PubMed |
Kerr LA, Secor DH, Piccoli PM (2009) Partial migration of fishes as exemplified by the estuarine-dependent white perch. Fisheries 34, 114-123.
| Crossref | Google Scholar |
Kitada S (2018) Economic, ecological and genetic impacts of marine stock enhancement and sea ranching: a systematic review. Fish and Fisheries 19(3), 511-532.
| Crossref | Google Scholar |
Klinard NV, Matley JK, Halfyard EA, Connerton M, Johnson TB, Fisk AT (2020) Post-stocking movement and survival of hatchery-reared bloater (Coregonus hoyi) reintroduced to Lake Ontario. Freshwater Biology 65, 1073-1085.
| Crossref | Google Scholar |
Kraft S, Gandra M, Lennox RJ, Mourier J, Winkler AC, Abecasis D (2023) Residency and space use estimation methods based on passive acoustic telemetry data. Movement Ecology 11, 12.
| Crossref | Google Scholar | PubMed |
Lee KA, Huveneers C, Peddemors V, Boomer A, Harcourt RG (2015) Born to be free? Assessing the viability of releasing captive-bred wobbegongs to restock depleted populations. Frontiers in Marine Science 2, 18.
| Crossref | Google Scholar |
Liu S, Zhou X, Zeng C, Frankstone T, Cao L (2022) Characterizing the development of sea ranching in China. Reviews in Fish Biology and Fisheries 32(3), 783-803.
| Crossref | Google Scholar |
Loneragan N, Haywood M, Heales D, Kenyon R, Pendrey R, Vance D (2001) Estimating the influence of prawn stocking density and seagrass type on the growth of juvenile tiger prawns (Penaeus semisulcatus): results from field experiments in small enclosures. Marine Biology 139, 343-354.
| Crossref | Google Scholar |
Loneragan NR, Jenkins GI, Taylor MD (2013) Marine stock enhancement, restocking, and sea ranching in australia: future directions and a synthesis of two decades of research and development. Reviews in Fisheries Science 21, 222-236.
| Crossref | Google Scholar |
Lorenzen K (2014) Understanding and managing enhancements: why fisheries scientists should care. Journal of Fish Biology 85, 1807-1829.
| Crossref | Google Scholar | PubMed |
Lorenzen K, Leber KM, Blankenship HL (2010) Responsible approach to marine stock enhancement: an update. Reviews in Fisheries Science 18, 189-210.
| Crossref | Google Scholar |
Lowry M, Becker A, Folpp H, McLeod J, Taylor MD (2017) Residency and movement patterns of yellowfin bream (Acanthopagrus australis) released at natural and artificial reef sites. Marine and Freshwater Research 68, 1479-1488.
| Crossref | Google Scholar |
Niella Y, Flávio H, Smoothey AF, Aarestrup K, Taylor MD, Peddemors VM, Harcourt R (2020) Refined shortest paths (RSP): incorporation of topography in space use estimation from node-based telemetry data. Methods in Ecology and Evolution 11, 1733-1742.
| Crossref | Google Scholar |
Noda T, Wada T, Iwasaki T, Sato T, Narita K, Matsumoto I, Hori T, Mitamura H, Arai N (2019) Post-release behaviors and movements of cultured and wild Japanese eels (Anguilla japonica) in a shallow brackish water lagoon in northeastern Japan. Environmental Biology of Fishes 102(12), 1435-1456.
| Crossref | Google Scholar |
Pollock BR (1982) Movements and migrations of yellowfin bream, Acanthopagrus australis (Günther), in Moreton Bay, Queensland as determined by tag recoveries. Journal of Fish Biology 20(3), 245-252.
| Crossref | Google Scholar |
Sánchez-Lamadrid A (2002) Stock enhancement of gilthead sea bream (Sparus aurata, L.): assessment of season, fish size and place of release in SW Spanish coast. Aquaculture 210(1–4), 187-202.
| Crossref | Google Scholar |
Taylor MW, Laffan SD, Fielder DS, Suthers IM (2006) Key habitat and home range of mulloway Argyrosomus japonicus in a south-east Australian estuary: finding the estuarine niche to optimise stocking. Marine Ecology Progress Series 328, 237-247.
| Crossref | Google Scholar |
Taylor MD, Fairfax AV, Suthers IM (2013) The race for space: using acoustic telemetry to understand density-dependent emigration and habitat selection in a released predatory fish. Reviews in Fisheries Science 21, 276-285.
| Crossref | Google Scholar |
Taylor MD, Becker A, Lowry MB (2018) Investigating the functional role of an artificial reef within an estuarine seascape: a case study of yellowfin bream (Acanthopagrus australis). Estuaries and Coasts 41, 1782-1792.
| Crossref | Google Scholar |
van der Meulen DE, Walsh CT, Reinfelds IV, Payne NL, Ives MC, Roberts DG, Craig JR, Gray CA, Taylor MD (2023) Estuarine movements in a sparid hybrid complex. Marine and Freshwater Research 74(7), 625-640.
| Crossref | Google Scholar |
Wada T, Kamiyama K, Mitamura H, Arai N (2017) Horizontal movement and emigration of juvenile spotted halibut Verasper variegatus released in a shallow brackish lagoon: Matsukawa-ura, northeastern Japan, revealed by acoustic telemetry. Fisheries Science 83(4), 573-585.
| Crossref | Google Scholar |
Williams J, Hindell JS, Jenkins GP, Tracey S, Hartmann K, Swearer SE (2017) The influence of freshwater flows on two estuarine resident fish species show differential sensitivity to the impacts of drought, flood and climate change. Environmental Biology of Fishes 100, 1121-1137.
| Crossref | Google Scholar |
Wood SN (2011) Fast stable restricted maximum likelihood and marginal likelihood estimation of semiparametric generalized linear models. Journal of the Royal Statistical Society – B. Statistical Methodology 73(1), 3-36.
| Crossref | Google Scholar |
Yokota T, Mitamura H, Arai N, Masuda R, Mitsunaga Y, Itani M, Takeuchi H, Tsuzaki T (2006) Comparison of behavioral characteristics of hatchery-reared and wild red tilefish Branchiostegus japonicus released in Maizuru Bay by using acoustic biotelemetry. Fisheries Science 72(3), 520-529.
| Crossref | Google Scholar |