Reproductive phenology and the influence of temperature in two sympatric New Zealand freshwater mussel species
Michele Melchior


A
B
C
Abstract
Phenology plays a key role in shaping population dynamics, community structure and evolutionary adaptations. For freshwater mussels that rely on a parasitic larval (glochidia) phase on fish, shifts in reproductive phenology driven by environmental conditions may result in mismatches between glochidia release and host fish availability.
We investigated intra- and interspecific reproductive timing variations in sympatric Echyridella aucklandica and E. menziesii, and identified thermal cues (accumulated degree days, ADD) associated with brooding and glochidia maturation.
Brooding activity and glochidia maturation were assessed fortnightly–monthly over 1 year within four New Zealand streams.
The previously unknown phenology of E. aucklandica showed earlier brooding (May–July) and longer gravidity (9–11 months) than for E. menziesii (August; 6–7 months). Both species exhibited peak brooding in late austral spring–summer (November–December). ADD played a key role in regulating the timing of brooding onset in both species, as evidenced by the narrow ADD range observed across sites, and the relationship between ADD and brooding onset in both species.
The demonstrated link between ADD and reproductive phenology has broad implications in the context of climate change. Specifically, it raises concerns about potential timing mismatches in glochidia release and host-fish availability, which could affect the survival and reproductive success of freshwater mussels.
Keywords: Echyridella aucklandica, Echyridella menziesii, host fish, New Zealand, phenology, reproductive timing, temperature, Unionida.
Introduction
The timing, duration, and synchrony of biological activities in organisms, such as reproduction and development in response to environmental conditions (reproductive phenology), play a key role in shaping population dynamics, community structure and evolutionary adaptations (Schwartz 2003; Visser et al. 2010). Variations in reproductive phenology of coexisting species are common, and have, at times, been reported in congeneric sympatric species (e.g. Watters and O’Dee 2000; Pakanen et al. 2016). However, these patterns are often not fully understood, likely owing to being driven by a complex interplay between abiotic and biotic variables (Thackeray et al. 2010; Pekkonen et al. 2013).
Abiotic factors, such as water temperature, are important in regulating phenology in temperate aquatic environments where they may serve as predictable cues for timing of life-cycle stages (such as reproduction onset) to match with resource availability (Stachowicz et al. 2002; Goldman et al. 2004; Westerman et al. 2009), and thereby optimise offspring growth and survival (McNamara et al. 2011). By contrast, biotic variables reflect the evolutionary processes behind the timing of events and can influence reproductive phenological variations in species through interactions with other organisms (Post 2019).
Freshwater mussels of the order Unionida are characterised by a life cycle that includes a parasitic larval (glochidia) stage dependent on suitable host fish (Strayer et al. 2004; Haag 2012). As thermoconformers (i.e. species whose body temperatures fluctuate according to external temperature; Sanborn 2008), unionids will directly experience any changes in the environmental thermal regime to which they are exposed (Spooner and Vaughn 2008; Pandolfo et al. 2012). Patterns in the timing of brooding and larval release can vary among unionid species, owing, in part, to differences in prevailing water temperature regimes (Young and Williams 1984; Watters and O’Dee 2000; Hastie and Young 2003; Österling 2015; Melchior 2017), as well as photoperiod, velocity, and food availability (Hastie and Young 2003; Barnhart et al. 2008; Galbraith and Vaughn 2009). In particular, measures of accrued degree days (Davenport and Warmuth 1965; Galbraith and Vaughn 2009; Schneider et al. 2018; Dudding et al. 2019) and thermal thresholds (Watters and O’Dee 2000; Schneider et al. 2018) have been reported to have species-specific effects on the timing of unionid recruitment in laboratory and field studies.
The New Zealand Hyriidae Echyridella menziesii and the congeneric E. aucklandica co-occur in waterways throughout the northern regions of New Zealand (Marshall et al. 2014). In this study, we investigated intra- and interspecific variation in reproductive timing among sympatric populations of E. aucklandica and E. menziesii in four Waikato streams, and characterised reproductive phenology in both species in relation to water temperature regimes for the duration of their annual reproductive season, so as to test the hypothesis that (i) water temperature is a key driver regulating the timing and duration of brooding in both species (Young and Williams 1984; Watters and O’Dee 2000; Hastie and Young, 2003; Österling 2015).
Materials and methods
Sampling was conducted in four streams in Waikato, North Island, New Zealand (Strahler order 3–5), known to support large populations of the two study species, E. aucklandica and E. menziesii (Table 1). Three of the four study sites are situated on short coastal waterways draining into the Tasman Sea (Ohautira Stream, 37°45′43.0″S, 174°58′49.0″E; Kahururu Stream, 37°41′11.2″S, 174°58′45.0″E; Pakoka River, 37°55′42.0″S, 174°52′13.0″E), and the fourth site, Mangapiko Stream (37°58′.54.2″S, 175°28′26.3E), is an inland Waipa River tributary draining a predominantly agricultural landscape (Fig. 1, Table 1). Upstream native forest cover ranged from 9% of the catchment area (Kahururu) to 61% (Ohautira), with the remaining land use comprising mainly sheep or beef farming (Pakoka; Ohautira), dairy farming (Mangapiko) and Pinus radiata forestry (Kahururu) (FENZ database, Leathwick et al. 2010; Table 1). The climate in the region is typically warm, with humid summers and mild winters; western areas are exposed to extreme weather conditions brought about by prevailing westerly winds (Chappell 2013).
Item | Ohautira | Kahururu | Pakoka | Mangapiko | |
---|---|---|---|---|---|
E. aucklandica density (individuals m−2) | 0.81 (51) | 0.34 (32) | 0.43 (20) | 0.15 (23) | |
E. menziesii density (individuals m−2) | 0.49 (31) | 0.43 (40) | 1.01 (50) | 0.32 (50) | |
Catchment area (km2) | 38.8 | 42.6 | 24.7 | 40.1 | |
Upstream native forested catchment area (%) | 61.2 | 9.1 | 20.1 | 12.0 | |
Sample reach length (m) | 15 | 20 | 15 | 40 | |
Distance to sea (km) | 2.7 | 13.6 | 2.2 | 195.0 | |
Reach-scale canopy cover (%) | 69 | 44 | 7 | 14 | |
Wetted width (m) | 4.2 ± 0.19 | 4.7 ± 1.10 | 5.6 ± 0.20 | 3.9 ± 0.20 | |
Water temperature (°C) | 13.5 ± 3.6 | 14.5 ± 3.9 | 14.3 ± 4.1 | 14.7 ± 3.3 | |
Dissolved oxygen (mg L−1) | 11.1 ± 1.0 | 10.5 ± 2.5 | 11.3 ± 0.9 | 10.4 ± 0.8 | |
Dissolved oxygen (%) | 105 ± 6.4 | 88.7 ± 23.3 | 108.3 ± 6.0 | 102.3 ± 7.9 | |
Conductivity (μS cm−1) | 137.9 ± 17.7 | 141.6 ± 40.5 | 143.7 ± 24.7 | 110.7 ± 9.7 |
Physicochemical data are means of point measurements (±s.d.) taken during the year-long sampling period. Total number of unionid individuals per species collected at the start of the sampling period is listed in parentheses. Catchment area and Upstream native forested catchment area data were obtained from FENZ (Freshwater Ecosystems of New Zealand, Leathwick et al. 2010). Distance to sea data obtained from NZ Freshwater Fish Database (see http://nzffdms.niwa.co.nz, accessed May 2020). Reach scale canopy cover data were measured using a densiometer at the thalweg midway within each sample reach. Wetted width data are ±s.d. of transects evenly-spaced every 5 m along sampling reach. Dissolved oxygen and Conductivity data are ±s.d. measured fortnightly–monthly at thalweg (YSI 2030 Pro meter; Yellow Springs Instruments, Yellow Springs, OH, USA) (see Table S1 of the Supplementary material for details).
Locations of sampling sites (closed circles) showing (a) coastal sites Kahururu Stream, Ohautira Stream and Pakoka River, and (b) the inland site Mangapiko Stream.
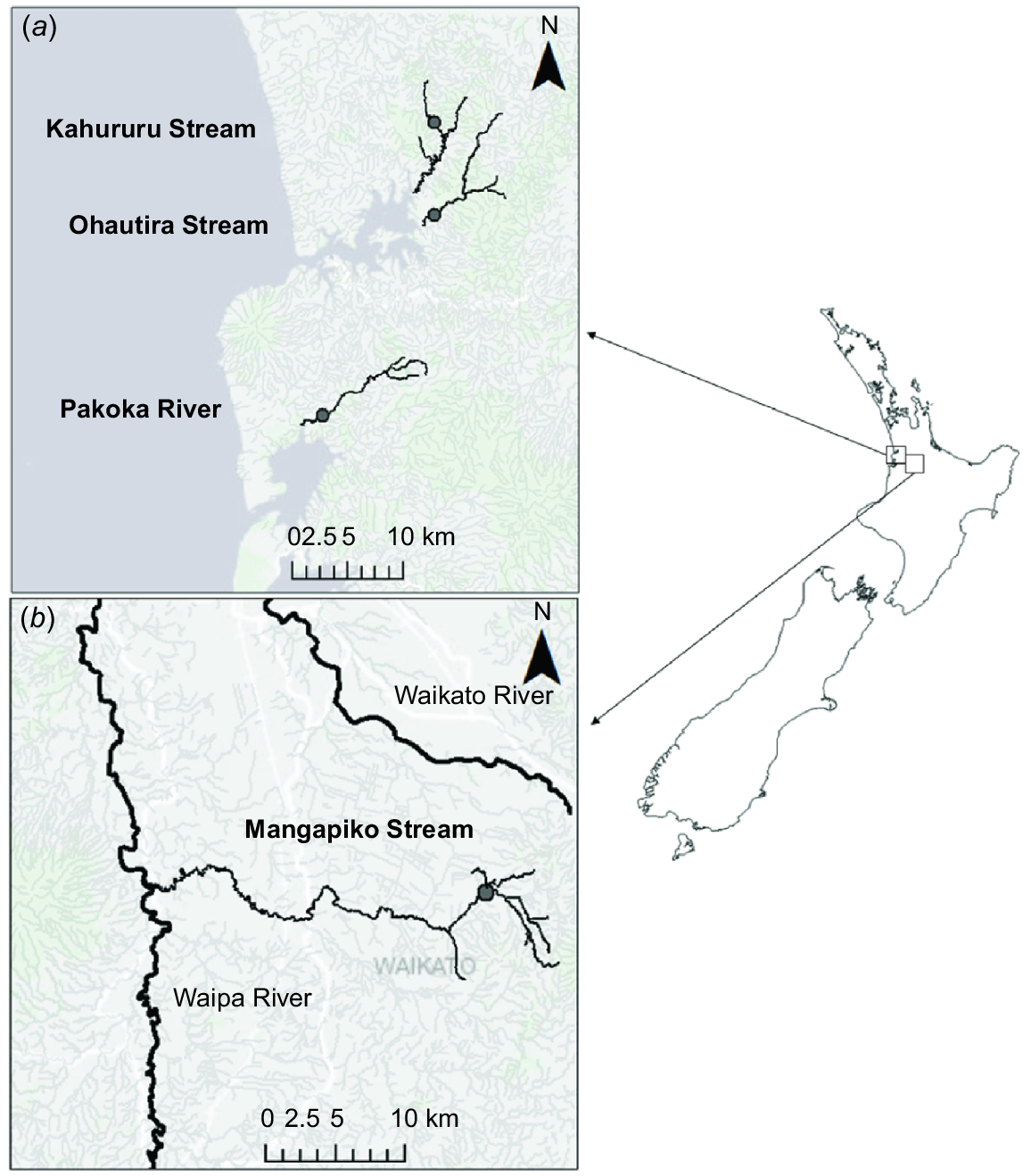
The streambeds for three of the study reaches are characterised by unconsolidated bottom substrates dominated by silt at Kahururu, small gravel at Mangapiko and large gravel at Ohautira. The channel bottom at Pakoka is dominated by cobbles with pockets of fine sediments that mussels inhabit. During sampling, mean wetted channel widths ranged from 3.9 to 5.6 m, and overhead shade was between 7% at Pakoka and 69% at Ohautira (Table 1). Mean monthly temperatures ranged from 13.5 to 14.7°C, dissolved oxygen was between 10.4 and 11.3 mg L−1 and specific conductivity at 25°C 110.7–143.7 μS cm−1 (Table 1).
Brooding status assessments
At all sites, E. menziesii and E. aucklandica sampling was undertaken fortnightly during the late austral spring–summer (excluding December because of high flow events) when female larval brooding activity is known to increase, and monthly at other times over a continuous period of 13 months. Tactile and visual (bathyscope) searches were conducted along the same reaches (15–40 m) on each occasion until 20–40 individuals of each species were collected. To determine their length, all individuals were measured to the nearest millimetre by using calipers. Additionally, the sex of each individual was determined by observing the gill morphology, specifically looking for marsupiumabsence (male) or presence (female) by using a speculum to prise apart valves. In sexually mature female hyriids, the marsupium is clearly visible throughout the year on the inner demibranchs as opaque thickened columns perpendicular to the hinge of the shell (Jones et al. 1986; Jupiter and Byrne 1997; Walker et al. 2001; see Fig. 2). Female brooding status was assessed using the procedure outlined by Melchior et al. (2021; see also Fig. 3), on the basis of classification of gill volume and pigmentation. During this process, individuals were held at ambient water temperatures in 2.5-L buckets or in mesh nets within the stream. Individual mussels were returned to their original location at the end of each sampling event.
(a) Non-brooding Echyridella aucklandica female with empty marsupium clearly visible on ventral inner demibranch (insert), and (b) male E. aucklandica with no marsupium present. (c) Mussels shown are from Kahururu Stream, with E. aucklandica (left) and E. menziesii (right) showing differences in size.
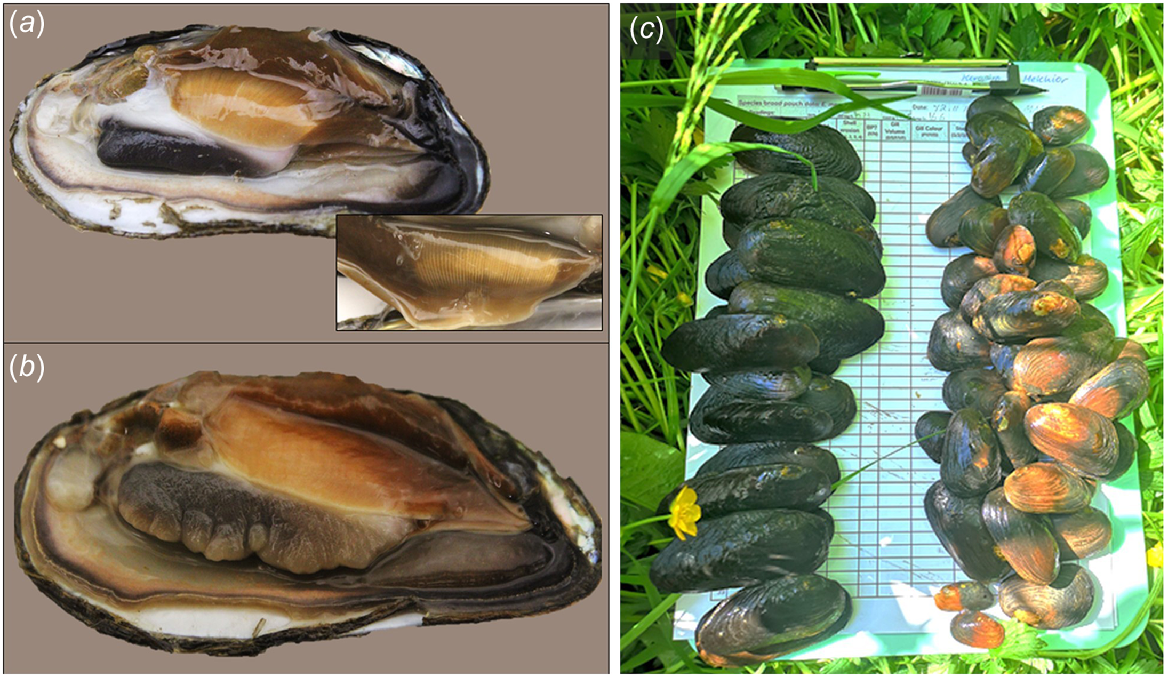
Larval stage microphotography, gill pigmentation gradient and description for staging validation in Echyridella aucklandica and E. menziesii (adapted from Melchior et al. 2021).
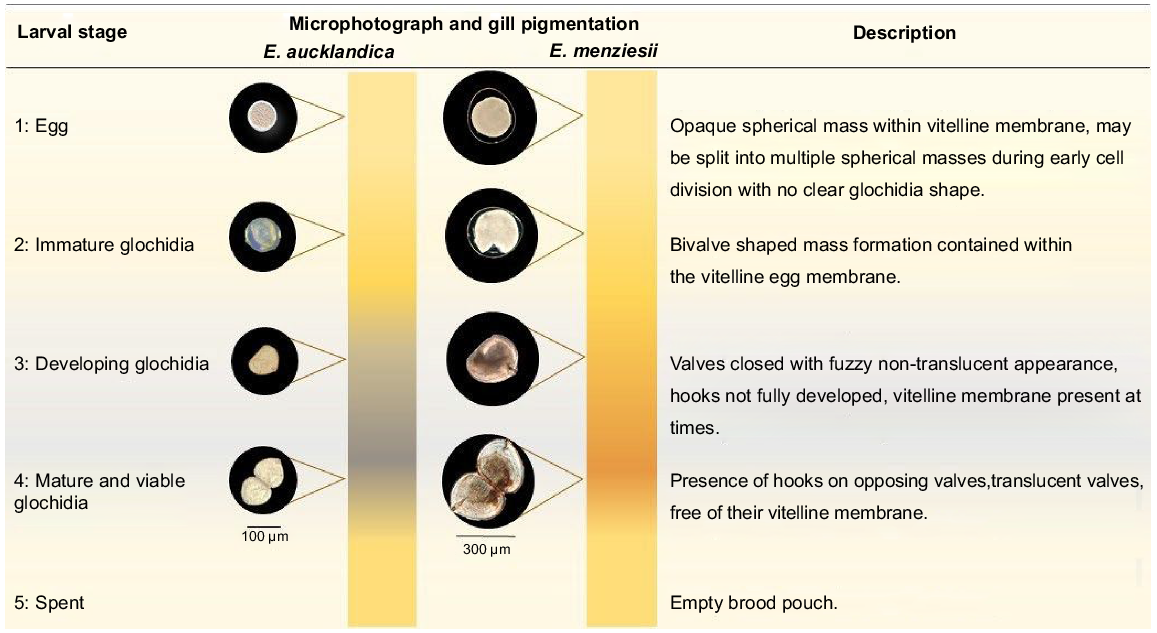
Six additional individuals per species collected upstream of each sampling reach during each sampling event (except March 2018) were used for validation of in vivo brooding stage by extraction of eggs or glochidia from gill marsupia (Fig. 3). The extracted gill contents were examined in the laboratory on a Petri dish under an Olympus SZ-6045 dissecting microscope (20–40×) and characterised into one of the five developmental stages (Fig. 3).
Environmental parameters
Water temperature (°C) was recorded at 15-min intervals at all sites for the duration of the study by using temperature loggers (Hobo Tidbit Data Logger, Onset, Bourne, MA, USA) submerged close to the streambed. Accumulated degree-days (ADD hereafter) were calculated with the University of California’s State-wide Integrated Pest Management Program online degree-day calculator (see https://ipm.ucanr.edu/WEATHER/index.html#DEGREEDAYS; Baskerville and Emin 1969), which uses maximum and minimum daily temperatures to calculate the number of ADD from set dates on the basis of the single sine method (Higley et al. 1986). Start dates were set from (i) the end of the previous brooding period for E. aucklandica and E. menziesii (31 March 2018) to determine ADD required to reach the next season’s brooding onset, and (ii) the sample date at which brooding mussels were first observed for each species within each stream (see Results) to determine the ADD required to reach peak brooding proportion as well as the duration of the brooding period.
The minimum thermal limit used for calculating ADD for each species was defined as 10°C, on the basis of laboratory trials (see the ‘Context’, ‘Methods’ and ‘Results’ sections of the Supplementary material) that tested for low thermal tolerance in brooding E. aucklandica and E. menziesii females. Here, the endpoint was defined as the temperature at which reproduction was likely to be halted and larvae prematurely aborted. The minimum threshold temperature used in this study corresponds to that found in similar international studies of unionids of the genera Quadrula, Cyclonaias and Fucsonia for ADD calculations based on metabolic-rate data (Spooner and Vaughn 2008; Galbraith and Vaughn 2009; Dudding et al. 2019).
Statistical analyses
All analyses were performed using the software R (ver. 4.0.0, R Foundation for Statistical Computing, Vienna, Austria, see https://www.r-project.org/). Data were assessed for normality (Shapiro–Wilk’s test and Q–Q plots) and homogeneity of variances (Levene’s test), and because parametric assumptions could not be confirmed for univariate statistics, non-parametric tests were used, with statistical significance defined at P ≤ 0.05. Following on from this, two-way Kolmogorov–Smirnov two-sample goodness-of-fit tests were used to detect differences in cumulative distribution of brooding proportions over time between each species within each site. Kruskall–Wallis tests (followed by Dunn’s post hoc multiple comparisons tests with Bonferroni correction) were performed to test for differences among sites in ADD required to reach brooding onset, peak brooding, and duration of brooding for each species.
To determine the strength of relationships between water temperature, specifically thermal summation (i.e. ADD), and brooding onset and brooding peak in E. menziesii and E. aucklandica, beta regressions with logit link function were implemented using the betareg package (ver. 3.1-3, A. Zeileis, F. Cribari-Neto and B. Gruen, see https://cran.r-project.org/package=betareg/; Cribari-Neto and Zeileis 2010), with ADD as the single predictor variable and the following response variables analysed separately for E. menziesii and E. aucklandica on the basis of pooled data from multiple sites: (i) proportion of mussels with glochidia development at Stage 1 (i.e. brooding onset), and (ii) proportion of mussels with glochidia development at Stage 4 (peak brooding). The coefficients of determination (r2) calculated using beta regression with logit function are given for each model. Beta regression was used to analyse these data, instead of a Gaussian generalised linear model, because the response variables (brooding onset and brooding peak) were expressed as proportions with a range from 0 to 1 and had variances that are usually not constant across the range of the predictor (Douma and Weedon 2019). Beta regressions can account for the heteroskedasticity and skewness that are common with proportional data (Cribari-Neto and Zeileis 2010). To help interpret the magnitude of the effects of the beta regressions, average marginal effects (which measure the change in a response given a change in a covariate) were calculated for each model by using the margins package (ver. 0.3.26, T. Leeper, J. Arnold, V. Arel-Bundock and J. A. Long, see https://cran.r-project.org/package=margins/; Williams 2012). Model assumptions, including normality and heteroskedasticity of residuals, were verified and assessed visually by diagnostic plots.
Results
Stream temperature regimes
Ohautira had the lowest average temperature every month throughout the entire sampling period (Table S1 of the Supplementary material), reaching a minimum from May to June of 6.4°C (Ohautira), compared with 7.1°C at Mangapiko. Warmest temperatures were experienced in February when averages varied between 18.9°C (Ohautira) and 20.2°C (Kahururu), with maxima at Kahururu of 24.2°C, followed closely by Pakoka (24.1°C), and then Mangapiko (22.9°C) and Ohautira (22.1°C) (Table S1). Lowest average February temperatures ranged from 9.8°C (Ohautira) to 10.9°C (Mangapiko) (Table S1).
Mussel population characteristics
At all sites, valve lengths were skewed towards larger mussels, particularly for E. aucklandica, with few mussels below 40 mm (Table S2). Gravid females were identified across a range of size classes within each species. The smallest brooding female found in this study was 23 mm long for E. menziesii (Pakoka) and 40 mm for E. aucklandica (Mangapiko), whereas the largest brooding E. aucklandica collected was 110 mm (Ohautira), compared with 91 mm for E. menziesii (Pakoka) (Table S2).
In total, 1813 assessments of female brooding status were conducted across all sites, species and dates combined. Brooding season varied among species, with brooding across the four sites starting 1–3 months earlier in E. aucklandica (austral autumn) than E. menziesii, which initiated brooding in late winter–early spring. In E. aucklandica, the timing of brooding onset varied among sites, ranging from late May to mid-July, unlike in E. menziesii, which consistently began brooding larvae at all sites in August when water temperatures started to show steady increases (Fig. 4, 5).
(a–d; upper panels) Brooding proportions in female Echyridella aucklandica and E. menziesii over 1 year (March 2018–March 2019) at the four sampling sites (see Fig. 5 for detail). (a–d; lower panels) Monthly temperature plots are shown for comparison; points indicate means and vertical lines indicate 10th and 90th percentiles (see Table S1 for detail).
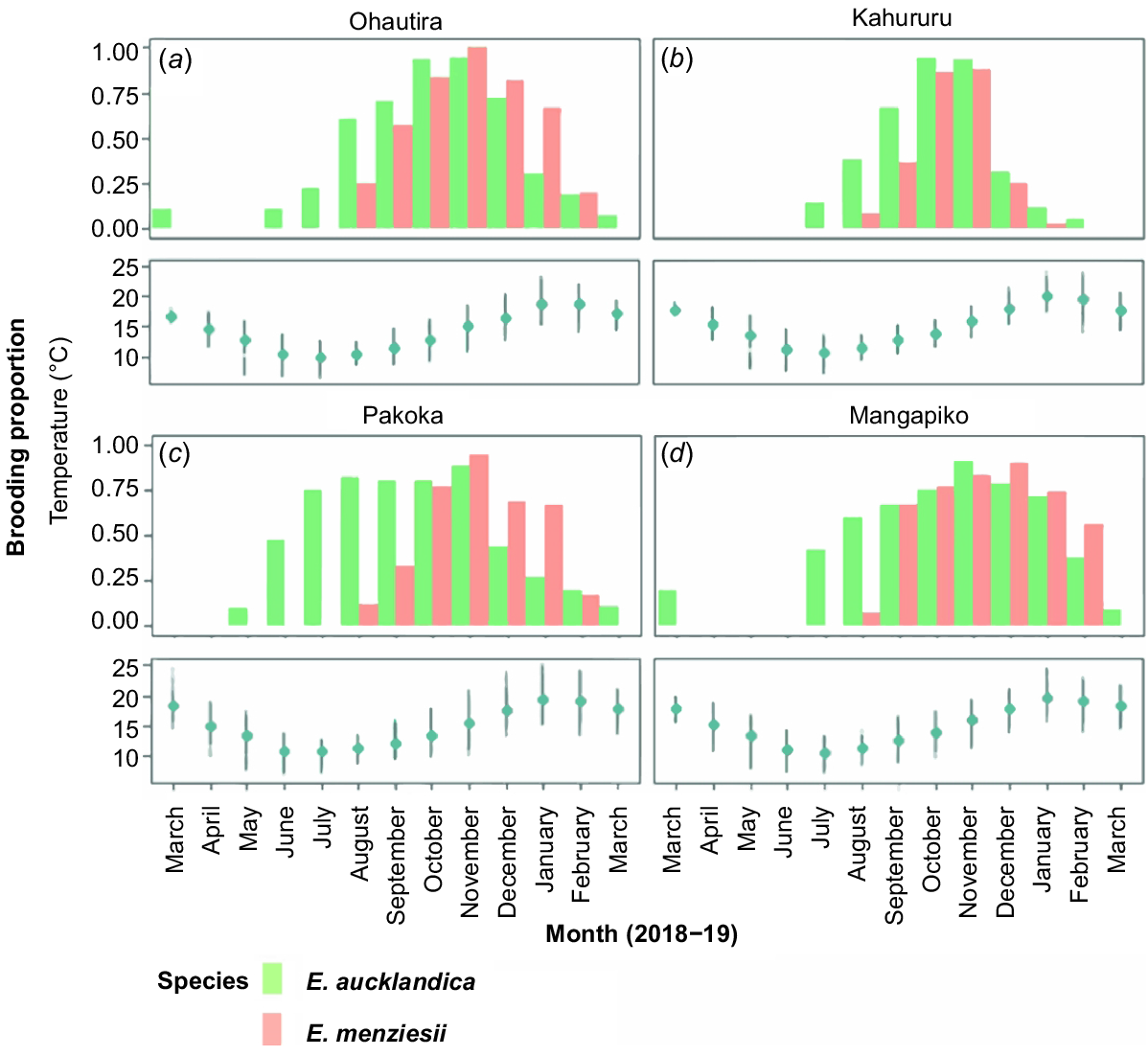
Glochidia brooding stages (1–5; see Fig. 3) over time (March 2018 to March 2019) in female Echyridella aucklandica (left) and E. menziesii (right) at four sites.
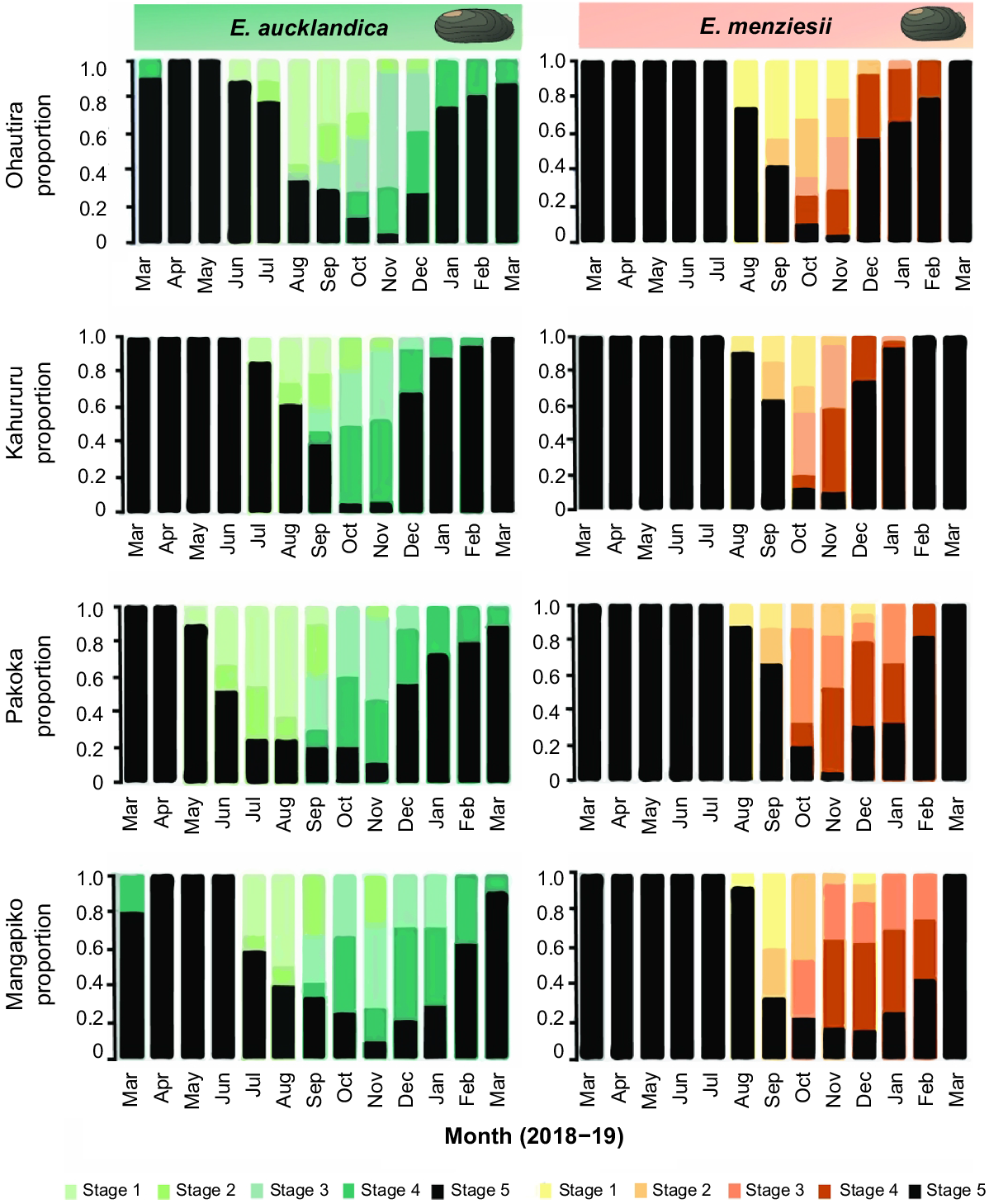
Brooding proportions at all sites except Mangapiko increased gradually over time, with females of E. aucklandica and E. menziesii reaching ~90% gravidity at peak brooding (Stage 4) within each site (Fig. 5). Females of E. menziesii and E. aucklandica were observed to have unimodal brooding peaks, with only one peak reproductive event at each site. Peak brooding (Stage 4) occurred slightly earlier in October–November (spring) and remained high for longer in E. aucklandica than in E. menziesii, which reached maximum brooding proportions in November and December (late spring to early summer) (Fig. 4). Gradual declines in brooding proportions following the peak at each site suggest the start of glochidia release in both species, with minimum brooding proportions in February to March (mid-summer to autumn) for E. aucklandica (coinciding with decreasing water temperatures) and January to February (summer) for E. menziesii, coinciding with maximum stream temperatures (Fig. 4).
Within all sites, the overall brooding season for female E. menziesii was shorter (6–7 months) than that for female E. aucklandica, which remained gravid for 9–11 months (Fig. 4). Although brooding for E. aucklandica occurred earlier and continued for longer than that for E. menziesii, no statistically significant variations were detected in the overall brooding distributions between the species at each site (Ohautira: D = 0.3, P = 0.7; Kahururu: D = 0.2; P = 0.99; Pakoka: D = 0.3 P = 0.6, Mangapiko: D = 0.3, P = 0.6), in part owing to temporal overlaps among sites in peak and non-brooding periods.
Brooding stages determined in vivo (verified by in vitro gill examinations) found that glochidia development within both species across all sites was initially synchronous. Early embryos made up the highest proportions of developing glochidia for several months following brooding onset. These were progressively replaced with later developmental stages (Fig. 5). Throughout mid-brooding, various developmental stages were present within individuals of both species throughout each sample month, until the period preceding the final stage of glochidia maturation (Stage 4). Mature glochidia (Stage 4) within E. aucklandica marsupia first occurred in September or October at all sites, whereas mature glochidia in E. menziesii marsupia were observed 1 month later in October and November across sites (Fig. 5).
Effects of temperature on brooding phenology
Analysis of ADD showed variable thermal regimes experienced by E. aucklandica and E. menziesii over time among sites (Fig. 6). The general observed upward trend in ADD steadied throughout the cooler months (May–July) and increased from August and September onward when water temperatures began to increase (Fig. 6). Despite being widely separated geographically, Kahururu (coastal) and Mangapiko (inland) had synchronous ADD regimes throughout almost the entire sample year and experienced similar increasing rates in ADD from October to November onward. Pakoka, too, followed patterns similar to those of Kahururu and Mangapiko, but experienced a slowing in ADD from November, whereas Ohautira’s forested headwaters and riparian shade meant that the ADD curve was lower throughout the entire study period than for the other sites (Fig. 6).
Cumulative stream temperature curves as degree days for each stream, showing phenologically sensitive time periods: EA onset, first observation of brooding in Echyridella aucklandica (solid vertical line) at each site; EM onset, first observation of brooding in E. menziesii (dotted vertical line); peak brooding, month at which brooding proportions were highest for each species.
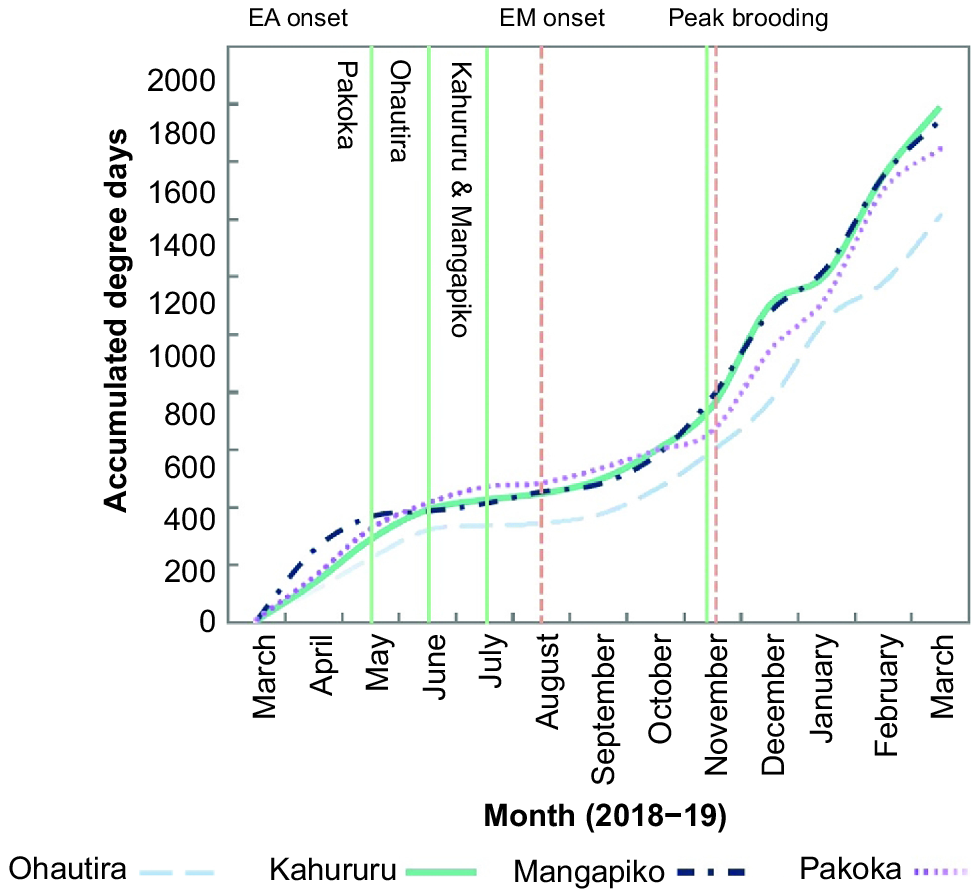
Significant differences between E. aucklandica and E. menziesii in median ADD required to reach brooding onset were found across all sites (Ohautira: P = 0.018, Kahururu: P = 0.01, Pakoka: P < 0.001, Mangapiko: P < 0.001; Table S3 of the Supplementary material). First observations of brooding in E. aucklandica varied substantially in terms of ADD (321, 326, 412 and 426 ADD at Ohautira, Pakoka, Mangapiko and Kahururu respectively; Table 2). Median ADD required to reach brooding Stage 1 for each E. aucklandica varied significantly among sites (K–W: n = 81, H = 424.2, P = 0.04), with differences occurring between Ohautira ( = 431 ADD) and Pakoka ( = 471 ADD adj P = 0.03), and between Ohautira and Kahururu ( = 494 ADD, adj P = 0.05), but not between the other sites. Echyridella menziesii showed a similar wide range of ADD among sites for brooding onset (345, 448, 454, and 484 ADD at Ohautira, Kahururu, Pakoka and Mangapiko respectively) (Table 2). Median ADD required to reach Stage 1 for each E. menziesii varied significantly among sites (K–W: n = 70, H = 30.9, P < 0.001), with Ohautira ( = 431 ADD) requiring lower ADD to reach brooding onset than all of the other sites (Kahururu: = 494 ADD, adj P < 0.001; Pakoka: = 533 ADD, adj P < 0.001; Mangapiko: = 480 ADD, adj P = 0.04; Table 2).
Ohautira | Kahururu | Pakoka | Mangapiko | Mean (±s.d.) | ||
---|---|---|---|---|---|---|
E. aucklandica | ||||||
Onset | 431 (321–484) | 494 (426–494) | 471 (326–471) | 454 (412–480) | 440 ± 44 | |
Peak | 481 (431–1411) | 715 (494–1208) | 665 (533–1500) | 783 (489–1548) | 763 ± 301 | |
Brooding duration | 1411 | 1208 | 1500 | 1548 | 1424 ± 159 | |
E. menziesii | ||||||
Onset | 431 (345–484) | 494 (448–597) | 533 (484–597) | 480 (454–577) | 502 ± 68 | |
Peak | 242 (118–931) | 288 (96–851) | 523 (168–1066) | 549 (190–1107) | 478 ± 289 | |
Brooding duration | 931 | 851 | 1066 | 1107 | 988 ± 118 |
Minimum–maximum ADD range across the four sample sites is shown in parentheses. Brooding duration was the first brood observation to last brood observation during the brooding season 2018–19.
Significant differences in median ADD for E. aucklandica and E. menziesii to reach peak brooding (Stage 4) were found across all sites (Ohautira: P = 0.018, Kahururu: P = 0.01, Pakoka: P < 0.001, Mangapiko: P = 0.002). In E. aucklandica, median ADD for peak brooding varied significantly among sites (K–W: n = 162, H = 26.60, P < 0.001), with significant differences between Ohautira (lower ADD at peak brooding) and all other sites (Kahururu: P = 0.002, Pakoka: P < 0.001, Mangapiko: P < 0.001) (Table S3). Median ADD for peak brooding in E. menziesii also varied significantly among sites (K–W: n = 184, H = 31.95, P < 0.001), with significant differences occurring only between Mangapiko (higher ADD at peak brooding) and each of the other sites (Ohautira: adj P < 0.001; Kahururu: adj P < 0.001; Pakoka: adj P = 0.01; Table 2). The ADD required to complete the brooding period ranged from 1411 (Ohautira) to 1548 (Mangapiko) for E. aucklandica, and from 851 (Kahururu) to 1107 (Mangapiko) for E. menziesii (Table 2).
Beta regressions used to determine the strength of relationships between water temperature expressed as ADD and brooding onset and brooding peak in E. menziesii and E. aucklandica showed variable results. Significant relationships for brooding onset (Stage 1) were stronger in E. aucklandica (β = 0.010, s.e. = 0.004, P = 0.001) than E. menziesii (β = 0.005, s.e. = 0.002, P = 0.047), with marginal effect sizes averaging 0.2 percentage points in E. aucklandica and 0.1 percentage point in E. menziesii for every increase in ADD (Table S4 of the Supplementary material). Peak maturity (Stage 4) was negatively related to ADD in E. aucklandica (β = −0.001, s.e. = 0.001, P = 0.003), but there was no significant relationship for E. menziesii (β =−0.001, s.e. = 0.001, P = 0.2; Fig. 7). Here, average marginal effect sizes were −0.03 percentage points for every increase in ADD for both species (Table S4). Percentage variance explained by ADD for significant relationships ranged from 28 to 47% (Fig. 7).
Relationship (a, b) between ‘brooding onset’ proportion (glochidia Stage 1) and (c, d) between ‘peak brooding’ proportion (glochidia Stage 4) and number of accumulated degree-days in Echyridella aucklandica and E. menziesii. Solid lines show beta regression relationships that are statistically significant at P < 0.01, whereas the dashed line indicates P < 0.05. Pseudo r2 values calculated using beta regression are given for each model.
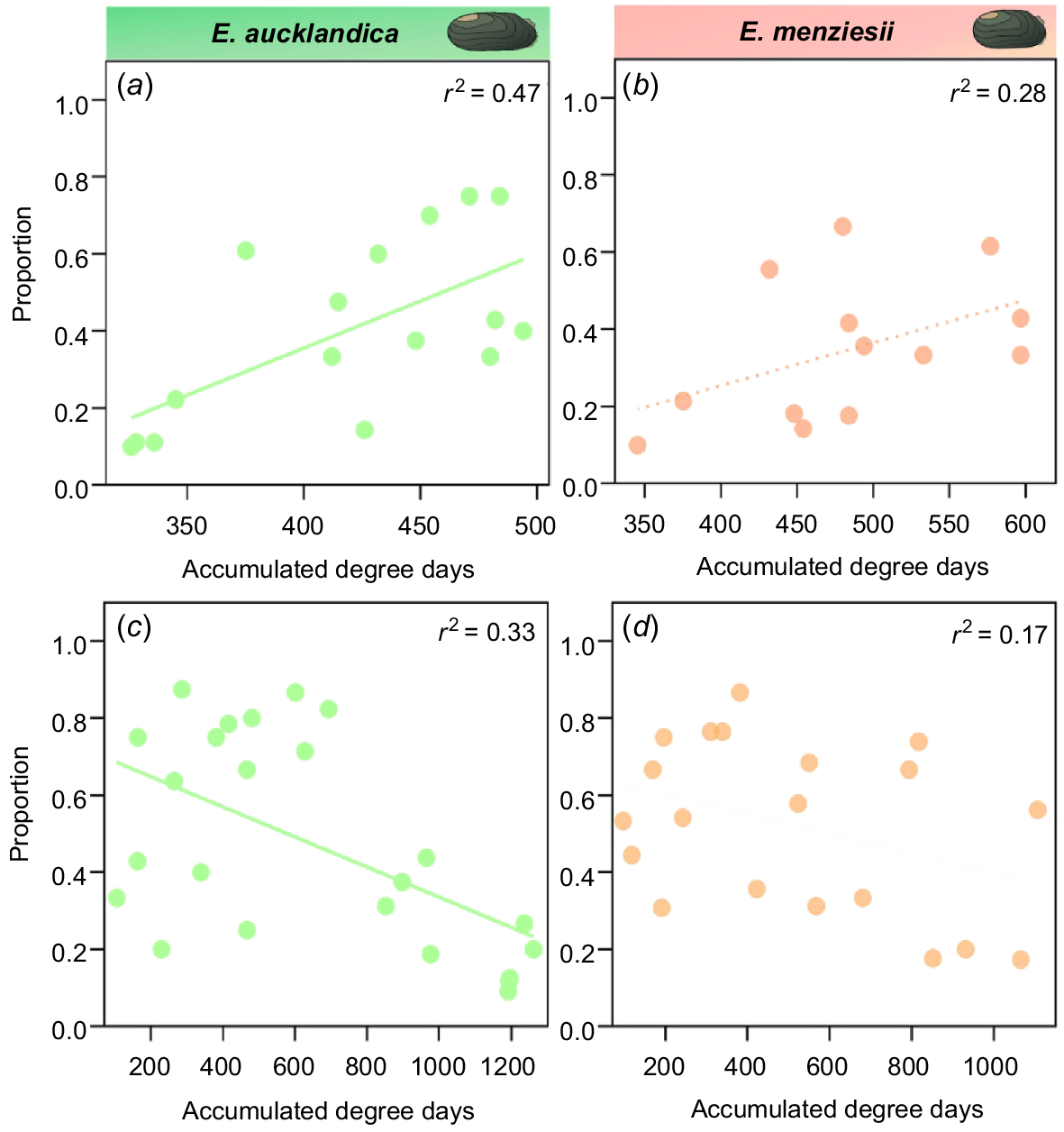
Discussion
We investigated the brooding phenology in relation to temperature in the two sympatric southern unionid species, E. menziesii and E. aucklandica. The previously unknown phenology of E. aucklandica showed earlier brooding (May–July) and longer gravidity (9–11 months) than for E. menziesii (August; 6–7 months). However, both species exhibited peak brooding in late austral spring–summer (November–December).
Our findings demonstrated that ADD played a role in regulating the timing of brooding onset in both species. This was evident from the narrow range of ADD observed across sites, and the significant relationship between ADD and brooding onset in both species. It is worth noting that ADD appeared less critical for timing of the peak brooding phase (which may be influenced by endogenous or exogenous factors other than thermal regime within the streams studied), than for timing of brooding onset in both species. These results provide partial support for the hypothesis that water temperature serves as a key driver in regulating the timing and duration of brooding in unionid mussels.
Unionid demographics
All sites were dominated by older individuals, with no small mussels found below 40 mm for E. aucklandica or below 23 mm for E. menziesii. The presence of geriatric, senescing populations dominated by large individuals, as found in all study streams, has been observed within waterways throughout New Zealand (e.g. James 1985; Roper and Hickey 1994; Catlin et al. 2018; Melchior et al. 2023a) and abroad (Vaughn and Spooner 2004; Geist et al. 2006; Hastie 2011; Stöckl et al. 2014; Österling 2015), and provides evidence of recruitment constraints that could lead to ‘extinction debt’ (i.e. delayed extinction of species owing to past events or disturbances; Tilman et al. 1994; Vaughn 2012). Accordingly, the longevity of mussels (e.g. >50 years for E. menziesii, see Grimmond 1968; unknown for E. aucklandica), coupled with the scarcity of smaller mussels in these Waikato streams, could indicate that both species may not be recruiting sufficient numbers of juveniles to sustain viable populations over the long term. However, the mechanisms that affect unionid recruitment are complex and further studies are required to understand recruitment dynamics in both species, including host fish specificity (Melchior et al. 2021, 2023b).
In this study, the smallest female mussels collected in both species were found to be gravid. Thus, the minimum sizes for brooding maturity (23 mm in E. menziesii and 40 mm in E. aucklandica) inferred here are considered conservative estimates because the lack of juveniles found in the field meant that smaller females were not assessed. Nevertheless, the minimum sizes reported in this study fall within the normal range for age at maturity of unionids elsewhere in New Zealand and globally. For example, histological analyses of E. menziesii in Lake Taupo found the smallest sexually mature female to be 37 mm long, estimated to be ~4 years old (S. J. Clearwater, D. S. Roper, C. W. Hickey, M. L. Martin and E. K. Williams, unpubl. data). Small size at sexual maturity has also been reported in females of an Australian Hyriidae species Velesunio angasi, which attained maturity at 1.5 years of age (40 mm; cf. in 1 year or 30 mm length in males), whereas Hyridella species have been reported to mature in 2–3 years (40 mm) and Cucumerunio novaehollandiae at 3–4 years (60 mm) (Jones et al. 1986; Byrne 1998).
Interspecific variations in brooding phenology
Echyridella aucklandica began brooding earlier and remained gravid for longer than did E. menziesii in this study, reaching peak brooding (and thus glochidia release) in late austral spring to summer. Despite differing brooding onsets between species, both E. aucklandica and E. menziesii displayed synchrony in the peak brooding period, such that mature glochidia of both species were ready for release across all sites throughout the warmer part of the year in November and December, although timing appears to vary between regions, at least for E. menziesii (Percival 1931; Hine 1978), and waterbody types (e.g. stream v. lake; S. J. Clearwater, D. S. Roper, C. W. Hickey, M. L. Martin and E. K. Williams, unpubl. data). This general brooding seasonality is consistent with other hyriids found in temperate coastal Australia (New South Wales), including Hyridella australis, H. depressa and H. drapeta, which coexist in the Macleay River, and in the sympatric Velesunio ambiguous and A. jacksoni found in the Murray River (Jones et al. 1986; Walker 2017). As with E. menziesii and E. aucklandica, brooding in the above-mentioned hyriids was initiated in winter and glochidia were released in spring to summer (extending into autumn in the case of H. australis; Jones et al. 1986).
The extended brooding durations of both mussel species in the present study (although shorter for E. menziesii than E. aucklandica) are generally consistent with ‘bradyticty’ or a long-term brooding strategy, rather than ‘tachyticty’ or a short-term brooding strategy as recognised and described in many North American freshwater mussels (Sterki 1895, 1898; Ortmann 1911; Graf and Foighil 2000; Price and Eads 2011). In tachytictic mussels, fertilisation occurs in spring, with larvae brooded until they are fully developed and released within weeks to a few months over summer (Graf and Foighil 2000). Contrastingly, in bradityctic mussels, fertilisation usually starts in autumn, with brooding continuing over the winter, followed by the release of mature glochidia in spring (Graf and Foighil 2000). The important distinction between these patterns is that bradytictic mussels complete fertilisation and ontogenesis earlier and continue to brood long after glochidia are mature and viable (Kat 1984). Bradyticty, known only from temperate lineages of freshwater mussels, is hypothesised to be advantageous because earlier ontogeny permits earlier infestation and metamorphosis on hosts in spring, allowing more time for growth in juveniles before temperature reductions in winter (Graf 1997; Graf and Foighil 2000).
Although the bradyticty and tachyticty model is used widely in the literature to broadly classify unionid brooding strategies, some researchers have pointed out that the tachytictic or bradytictic classification is too simplistic and needs to be re-evaluated because brooding periods among unionid species can be highly variable (Kondo 1987; Watters and O’Dee 2000; Haag 2012). In support of this, the brooding durations exhibited in both E. aucklandica and E. menziesii showed interspecific variations across the study sites, a common feature found among coexisting species within the Hyriidae in Australia and South America (Jones et al. 1986; Callil et al. 2012; Walker 2017). Earlier brooding onsets and longer brooding durations in E. aucklandica than in E. menziesii were found to lead to earlier onset of glochidia maturation and longer duration of mature glochidia present in the marsupia of E. aucklandica. Although brooding peaks in both species overlapped, early brooding onset elsewhere is thought to facilitate the release of mature glochidia over a longer period (Haag and Warren 2000), potentially increasing the chance of successful host fish attachment for E. aucklandica
Varying developmental stages were present within the marsupia of both E. menziesii and E. aucklandica throughout their respective brooding seasons. Mature glochidia were present for up to 5 months in E. menziesii and 7 months in E. aucklandica, and the number of empty demibranchs was observed to decrease toward the end of the reproductive season, indicating that glochidia occurred over an extended period. This finding is consistent with other Hyriidae, including the Australian Alathyria jacksoni and V. ambiguous, which release their larvae throughout spring and summer (Jones et al. 1986; Walker 2017). However, it contrasts with findings for the northern hemisphere Margaritifera margaritifera by Hastie and Young (2003) and M. auriculata by Soler et al. (2019), who reported releases to occur as sudden synchronised events triggered by environmental cues such as water temperature or river levels (something we did not evaluate).
Longer glochidia release durations in unionids, as observed in E. aucklandica in Waikato streams, may occur for numerous reasons. First, they may reduce the risk of temporal mismatches with host abundances (Cushing 1990; Hochwald 1997; see Melchior et al. 2023b). Second, asynchronous, brooding (and thus glochidia release), found in both E. aucklandica and E. menziesii, may make both species less vulnerable to environmental disruptions, such as seasonal pulsed flow events.
Thermal cues associated with brooding
Our investigation indicated that thermal cues are important in regulating the timing of brooding onset, brooding duration, and peak brooding in both E. aucklandica and E. menziesii. Water temperature is known to influence the timing of phenological events by effects of thermal summation (i.e. ADD) (Davenport and Warmuth 1965; Young and Williams 1984; Hastie and Young 2003; Galbraith and Vaughn 2009; Dudding et al. 2019); however, minimum threshold temperature (Trübsbach 1998; Watters and O’Dee 2000; Blažek and Gelnar 2006; Schneider et al. 2018), and thermal shock (Jungbluth and Lehmann 1976; Hastie and Young 2003) are also processes that may be involved in different phenological phases (Jungbluth and Lehmann 1976; Hastie and Young 2003) and highlight the complexities of resolving thermal influences on reproductive phenology.
Generally, the ADD required to reach each of the phenological phases varied between species, with E. menziesii requiring broadly more ADD to reach brooding onset but less ADD for peak brooding and brooding duration than did E. aucklandica. Reasonably narrow ranges across sites in ADD required to reach brooding onset, particularly in E. aucklandica, coupled with a strong relationship between ADD and brooding onset in both species (although the strength of the relationship was weaker in E. menziesii), suggest that ADD may have some importance in regulating the timing of brooding onset in both species. Echyridella aucklandica brooding began in early to mid-winter when water temperatures were lowest among sites, whereas E. menziesii brooding onset occurred simultaneously across all sites in early spring when stream temperatures were increasing, suggesting an interaction with temperature thresholds as a secondary process initiating E. menziesii brooding. The negative relationship between peak brooding and ADD in E. aucklandica highlighted a gradual decline in the proportion of brooding unionids carrying mature glochidia with increasing ADD, probably indicating the onset of gradual larval release.
The proportion of brooding females in both species among all streams increased gradually over time, reaching peak brooding in November and December, before gradually declining until February and March. This synchronous pattern suggests that thermal summation (ADD) played a key role in determining brooding duration more so than a minimum threshold, which would have triggered sudden and simultaneous brooding or release events. The wide ADD ranges within species and between sites, particularly for peak brooding and brooding duration, along with large amounts of unexplained variation seen in the relationships of peak brooding and brooding onset with ADD, may be attributed to phenotypic plasticity in response to local environmental conditions (Bauer 1992; Johnson and Brown 2000; Hochwald 2001). For example, fish presence has been suggested to influence the timing of glochidia release, particularly in bradytictic brooding mussels, with some studies suggesting that host recognition mechanisms mediated by physical and chemical interactions may be important for the stimulation of glochidia release (Haag and Warren 2003; Barnhart et al. 2008; Schneider et al. 2018).
Melchior et al. (2023b) showed that E. aucklandica appears to use only Retropinna retropinna as a host fish, whereas E. menziesii is a host generalist, transforming on a range of native and introduced fish species, but not on R. retropinna. Timing glochidia release to coincide with host presence may be particularly important in New Zealand unionid species, where a large proportion of the known host fish species pool is diadromous. However, large flood events, which are usually associated with fish migrations, may override the impact of thermal cues, indicating that extended availability of glochidia may be an adaptive advantage where flooding is unpredictable.
As freshwater mussels are predominantly sedentary, with limited dispersal abilities as adults, they are especially vulnerable to the effects of a warming climate (Archambault et al. 2018). We have shown that some aspects of the reproductive phenology of southern hemisphere freshwater mussels appear sensitive to environmental cues such as water temperature. Specifically, shifts in temperature may potentially disrupt or shift reproductive timing in these species, with the potential for a disconnect between glochidia maturation and release and host fish abundances (Forrest and Miller-Rushing 2010; Schneider et al. 2018). Additionally, sudden fluctuations in temperature from a thermal regime to which species have adapted may impair mussel recruitment through abortions of immature and unviable larvae in early brooding females (Van Vreede et al. 1999; Schneider et al. 2018; see the ‘Context’, ‘Methods’ and ‘Results’ sections of the Supplementary material). It is therefore imperative to understand the potential impacts of climate change on both population- and community-level patterns in the reproductive phenology of unionid mussels and their reproductive synchrony with host fish.
Data availability
The data that support this study will be shared upon request to the corresponding author. This paper forms part of the PhD thesis of Melchior (2021).
Declaration of funding
This research was supported by the New Zealand Ministry of Business Innovation and Employment (MBIE) grant to NIWA for the Cultural Keystone Species: Co-management and restoration of our freshwater taonga species (CKS2020) programme (grant number C01X1616).
Acknowledgements
The authors thank NIWA and University of Waikato staff and students for help in the field. Special thanks go to Karen Thompson, Nicole Squires, Vanessa Barbosa, Tom Moore, Georgina Flowers, Anita Pearson, Thomas Allen and Elmie Senekal and the University of Waikato Volunteer Group for field assistance. Further thanks go to landowners Brent Pini, Nora Palmer (Paepaeruru Trust), and Noel Bamber for site access. The authors also appreciate the anonymous reviewers for constructive comments and valuable suggestions on the paper.
References
Archambault JM, Cope WG, Kwak TJ (2018) Chasing a changing climate: reproductive and dispersal traits predict how sessile species respond to global warming. Diversity and Distributions 24, 880-891.
| Crossref | Google Scholar |
Barnhart MC, Haag WR, Roston WN (2008) Adaptations to host infection and larval parasitism in Unionoida. Journal of the North American Benthological Society 27, 370-394.
| Crossref | Google Scholar |
Baskerville GL, Emin P (1969) Rapid estimation of heat accumulation from maximum and minimum temperatures. Ecology 50, 514-517.
| Crossref | Google Scholar |
Bauer G (1992) Variation in the life span and size of the freshwater pearl mussel. Journal of Animal Ecology 61, 425-436.
| Crossref | Google Scholar |
Blažek R, Gelnar M (2006) Temporal and spatial distribution of glochidial larval stages of European unionid mussels (Mollusca: Unionidae) on host fishes. Folia Parasitologica 53, 98-106.
| Crossref | Google Scholar | PubMed |
Byrne M (1998) Reproduction of river and lake populations of Hyridella depressa (Unionacea: Hyriidae) in New South Wales: implications for their conservation. Hydrobiologia 389, 29-43.
| Crossref | Google Scholar |
Callil CT, Krinski D, Silva FA (2012) Variations on the larval incubation of Anodontites trapesialis (Unionoida, Mycetopodidae): synergetic effect of the environmental factors and host availability. Brazilian Journal of Biology 72, 545-552.
| Crossref | Google Scholar |
Cribari-Neto F, Zeileis A (2010) Beta regression in R. Journal of Statistical Software 34, 1-24.
| Crossref | Google Scholar |
Cushing DH (1990) Plankton production and year-class strength in fish populations: an update of the match/mismatch hypothesis. Advances in Marine Biology 26, 249-293.
| Crossref | Google Scholar |
Davenport D, Warmuth M (1965) Notes on the relationship between the freshwater mussel Anodonta implicata Say and the alewife Pomolobus pseudoharengus (Wilson). Limnology and Oceanography 10, 74-78.
| Crossref | Google Scholar |
Douma JC, Weedon JT (2019) Analysing continuous proportions in ecology and evolution: a practical introduction to beta and Dirichlet regression. Methods in Ecology and Evolution 10, 1412-1430.
| Crossref | Google Scholar |
Dudding J, Hart M, Khan J, Robertson CR, Lopez R, Randklev CR (2019) Host fish associations for two highly imperiled mussel species from the southwestern United States: Cyclonaias necki (Guadalupe orb) and Fusconaia mitchelli (false spike). Freshwater Mollusk Biology and Conservation 22, 12-19.
| Crossref | Google Scholar |
Forrest J, Miller-Rushing AJ (2010) Toward a synthetic understanding of the role of phenology in ecology and evolution. Philosophical Transactions of the Royal Society of London – B. Biological Sciences 365, 3101-3112.
| Crossref | Google Scholar | PubMed |
Galbraith HS, Vaughn CC (2009) Temperature and food interact to influence gamete development in freshwater mussels. Hydrobiologia 636, 35-47.
| Crossref | Google Scholar |
Geist J, Porkka M, Kuehn R (2006) The status of host fish populations and fish species richness in European freshwater pearl mussel (Margaritifera margaritifera) streams. Aquatic Conservation: Marine and Freshwater Ecosystems 16, 251-266.
| Crossref | Google Scholar |
Graf DL, Foighil DÓ (2000) The evolution of brooding characters among the freshwater pearly mussels (Bivalvia: Unionoidea) of North America. Journal of Molluscan Studies 66, 157-170.
| Crossref | Google Scholar |
Haag WR, Warren ML, Jr (2000) Effects of light and presence of fish on lure display and larval release behaviours in two species of freshwater mussels. Animal Behaviour 60, 879-886.
| Crossref | Google Scholar | PubMed |
Haag WR, Warren ML, Jr (2003) Host fishes and infection strategies of freshwater mussels in large Mobile Basin streams, USA. Journal of the North American Benthological Society 22, 78-91.
| Crossref | Google Scholar |
Hastie LC (2011) Are Scottish freshwater pearl mussel populations recruiting normally? Toxicological & Environmental Chemistry 93, 1748-1763.
| Crossref | Google Scholar |
Hastie LC, Young MR (2003) Timing of spawning and glochidial release in Scottish freshwater pearl mussel (Margaritifera margaritifera) populations. Freshwater biology 48, 2107-2117.
| Crossref | Google Scholar |
Higley LG, Pedigo LP, Ostlie KR (1986) DEGDAY: a program for calculating degree-days, and assumptions behind the degree-day approach. Environmental Entomology 15, 999-1016.
| Crossref | Google Scholar |
Hine PM (1978) Distribution of some parasites of freshwater eels in New Zealand. New Zealand Journal of Marine and Freshwater Research 12, 179-187.
| Crossref | Google Scholar |
Hochwald S (1997) Populationsökologie der Bachmuschel (Unio crassus). Bayreuther Forum Ökologie 50, 1-166.
| Google Scholar |
James MR (1985) Distribution, biomass and production of the freshwater mussel, Hyridella menziesii (Gray), in Lake Taupō, New Zealand. Freshwater Biology 15, 307-314.
| Crossref | Google Scholar |
Johnson PD, Brown KM (2000) The importance of microhabitat factors and habitat stability to the threatened Louisiana pearl shell, Margaritifera hembeli (Conrad). Canadian Journal of Zoology 78, 271-277.
| Crossref | Google Scholar |
Jones HA, Simpson RD, Humphrey CL (1986) The reproductive cycles and glochidia of freshwater mussels (Bivalvia: Hyriidae) of the Macleay River, northern New South Wales, Australia. Malacologia 27, 185-202.
| Google Scholar |
Jungbluth JH, Lehmann G (1976) Untersuchungen zur verbreitung, morphologie und okologie der Margaritifera–population an atypischen standorten des jungertiarenbasaltes im Vogelsberg Oberhessen (Mollusca: Bivalvia). Archiv für Hydrobiologie 78, 265-323.
| Google Scholar |
Jupiter SD, Byrne M (1997) Light and scanning electron microscopy of the embryos and glochidia larvae of the Australian freshwater bivalve Hyridella depressa (Hyriidae). Invertebrate Reproduction & Development 32, 177-186.
| Crossref | Google Scholar |
Kat PW (1984) Parasitism and the unionacea (bivalvia). Biological Reviews 59, 189-207.
| Crossref | Google Scholar |
Kondo T (1987) Breeding seasons of seven species of unionid mussels (Bivalvia: Unionidae) in a small creek. Japanese Journal of Malacology 46, 227-236.
| Crossref | Google Scholar |
Marshall BA, Fenwick MC, Ritchie PA (2014) New Zealand Recent Hyriidae (Mollusca: Bivalvia: Unionida). Molluscan Research 34, 181-200.
| Crossref | Google Scholar |
McNamara JM, Barta Z, Klaassen M, Bauer S (2011) Cues and the optimal timing of activities under environmental changes. Ecology Letters 14, 1183-1190.
| Crossref | Google Scholar | PubMed |
Melchior M (2021) Partitioning along reproductive niche dimensions in sympatric New Zealand freshwater bivalve species. PhD Thesis, The University of Waikato, Hamilton, New Zealand. Available at https://hdl.handle.net/10289/14552
Melchior M, Collier KJ, Clearwater SJ (2021) First record of complex release strategies and morphometry of glochidia in sympatric Echyridella species (Bivalvia: Unionida: Hyriidae). Hydrobiologia 848, 3115-3126.
| Crossref | Google Scholar |
Melchior M, Squires NJ, Clearwater SJ, Collier KJ (2023b) Discovery of a host fish species for the threatened New Zealand freshwater mussel Echyridella aucklandica (Bivalvia: Unionida: Hyriidae). New Zealand Journal of Marine and Freshwater Research 57, 152-159.
| Crossref | Google Scholar |
Ortmann AE (1911) The anatomical structure of certain exotic naiades compared with that of the North American forms. Nautilis 24, 103-108.
| Google Scholar |
Österling EM (2015) Timing, growth and proportion of spawners of the threatened unionoid mussel Margaritifera margaritifera: influence of water temperature, turbidity and mussel density. Aquatic Science 77, 1-8.
| Crossref | Google Scholar |
Pakanen VM, Orell M, Vatka E, Rytkönen S, Broggi J (2016) Different ultimate factors define timing of breeding in two related species. PLoS ONE 11, e0162643.
| Crossref | Google Scholar | PubMed |
Pandolfo TJ, Kwak TJ, Cope WG (2012) Thermal tolerances of freshwater mussels and their host fishes: species interactions in a changing climate. Freshwater Mollusk Biology and Conservation 15, 69-82.
| Crossref | Google Scholar |
Pekkonen M, Ketola T, Laakso JT (2013) Resource availability and competition shape the evolution of survival and growth ability in a bacterial community. PLoS ONE 8, e76471.
| Crossref | Google Scholar | PubMed |
Percival E (1931) A note on the life history of Diplodon lutulentus Gould. Transactions and Proceedings of the New Zealand Institute 62, 86-91.
| Google Scholar |
Price JE, Eads CB (2011) Brooding patterns in three freshwater mussels of the genus Elliptio in the Broad River in South Carolina. American Malacological Bulletin 29, 121-126.
| Crossref | Google Scholar |
Roper DS, Hickey CW (1994) Population structure, shell morphology, age and condition of the freshwater mussel Hyridella menziesii (Unionacea: Hyriidae) from seven lake and river sites in the Waikato River system. Hydrobiologia 284, 205-217.
| Crossref | Google Scholar |
Schneider LD, Nilsson PA, Österling EM (2018) Evaluating temperature- and host-dependent reproduction in the parasitic freshwater mussel Unio crassus. Hydrobiologia 810, 283-293.
| Crossref | Google Scholar |
Soler J, Boisneau C, Jugé P, Richard N, Guerez Y, Morisseau L, Wantzen M, Araujo R (2019) An unexpected host for the endangered giant freshwater pearl mussel Margaritifera auricularia (Spengler, 1793) as a conservation tool. Aquatic Conservation: Marine and Freshwater Ecosystems 29, 1758-1770.
| Crossref | Google Scholar |
Spooner DE, Vaughn CC (2008) A trait-based approach to species’ roles in stream ecosystems: climate change, community structure, and material cycling. Oecologia 158, 307-317.
| Crossref | Google Scholar | PubMed |
Stachowicz JJ, Terwin JR, Whitlatch RB, Osman RW (2002) Linking climate change and biological invasions: ocean warming facilitates nonindigenous species invasions. Proceedings of the National Academy of Sciences 99, 15497-15500.
| Crossref | Google Scholar |
Sterki V (1895) Descriptions of new Pisidia. Nautilus 9, 74-76.
| Google Scholar |
Sterki V (1898) New pisidia. Nautilus 11, 112-114.
| Google Scholar |
Strayer DL, Downing JA, Haag WR, King TL, Layzer JB, Newton TJ, Nichols JS (2004) Changing perspectives on pearly mussels, North America’s most imperiled animals. BioScience 54, 429-439.
| Crossref | Google Scholar |
Stöckl K, Taeubert JE, Geist J (2014) Fish species composition and host fish density in streams of the thick-shelled river mussel (Unio crassus): implications for conservation. Aquatic Conservation: Marine and Freshwater Ecosystems 25, 276-287.
| Crossref | Google Scholar |
Thackeray SJ, Sparks TH, Frederiksen M, Burthe S, Bacon PJ, Bell JR, Botham MS, Brereton TM, Bright PW, Carvalho L, Clutton-Brock T, Dawson A, Edwards M, Elliott JM, Harrington R, Johns D, Jones ID, Jones JT, Leech DI, Roy DB, Scott WA, Smith M, Smithers RJ, Winfield IJ, Wanless S (2010) Trophic level asynchrony in rates of phenological change for marine, freshwater and terrestrial environments. Global Change Biology 16, 3304-3313.
| Crossref | Google Scholar |
Tilman D, May RM, Lehman CL, Nowak MA (1994) Habitat destruction and the extinction debt. Nature 371, 65-66.
| Crossref | Google Scholar |
Van Vreede KB, Macintosh DL, Black MC (1999) Estimating time-to-gravid for a freshwater mussel, Utterbackia imbecillis (Unionidae), after temperature conditioning in the laboratory. Environmental Toxicology and Chemistry 18(7), 1469-1473.
| Crossref | Google Scholar |
Vaughn CC (2012) Life history traits and abundance can predict local colonisation and extinction rates of freshwater mussels. Freshwater Biology 57, 982-992.
| Crossref | Google Scholar |
Vaughn CC, Spooner DE (2004) Status of the mussel fauna of the Poteau River and implications for commercial harvest. The American Midland Naturalist 152, 336-346.
| Crossref | Google Scholar |
Visser ME, Caro SP, van Oers K, Schaper SV, Helm B (2010) Phenology, seasonal timing and circannual rhythms: towards a unified framework. Philosophical Transactions of the Royal Society of London – B. Biological Sciences 365, 3113-3127.
| Crossref | Google Scholar |
Walker KF (2017) Reproductive phenology of river and lake populations of freshwater mussels (Unionida: Hyriidae) in the River Murray. Molluscan Research 37, 31-44.
| Crossref | Google Scholar |
Watters GT, O’Dee SH (2000) Glochidial release as a function of water temperature: beyond bradyticty and tachyticty. In ‘Proceedings of the Conservation, Captive Care, and Propagation of Freshwater Mussels Symposium’, January 1998, Columbus, OH, USA. (Eds RA Tankersley, DI Warmolts, GT Watters, BJ Armitage, PD Johnson, RS Butler) pp. 135–140. (Ohio Biological Survey: Columbus, OH, USA)
Westerman EL, Whitlatch R, Dijkstra JA, Harris L (2009) Variation in brooding period masks similarities in response to changing temperatures. Marine Ecology Progress Series 391, 13-19.
| Crossref | Google Scholar |
Williams R (2012) Using the margins command to estimate and interpret adjusted predictions and marginal effects. Stata Journal 12, 308-331.
| Crossref | Google Scholar |
Young M, Williams J (1984) The reproductive biology of the freshwater pearl mussel Margaritifera margaritifera (Linn.) in Scotland. I: field studies. Archiv für Hydrobiologie 99, 405-422.
| Google Scholar |