Knowledge of macrophyte requirements and tolerances holds the key to successful shallow lake restoration – a New Zealand perspective
Deborah Hofstra
A
Abstract
Many shallow lakes are degraded to the point where they are permanently turbid and macrophytes no longer grow. Without the macrophytes, wave action resuspends lakebed sediments, and a feedback loop is set up, trapping the lake in a degraded state. Multiple restoration actions are required to reverse this process, including catchment management and in-lake actions to remove barriers to establishing native vegetation. In particular, when macrophytes have been lost for a long time, the native seedbank may be depauperate and limit re-establishment opportunities. Re-establishment of submerged vegetation is critical to the restoration of lakes, but to date there have been few efforts to actively do this. There are significant barriers preventing this goal, relating to poor water quality, low-density sediments and the browsing impacts of fish and waterfowl, that will be further exacerbated by the warmer temperatures and more extreme weather events forecast under climate change. A combination of experimental and field studies determining optimal environmental ranges of different macrophyte species, methods to culture en masse and techniques to promote sufficient water clarity and sediment density to sustain macrophytes are necessary. Once minimum vegetation cover thresholds are exceeded, sustainable restoration to a macrophyte-dominated clear-water state should eventuate.
Keywords: climate change, degraded, habitat matching, light requirements, re-establish aquatic plants, sediment density, seedbank, water quality.
Introduction
Over 90% of shallow (<10 m), lowland (<170 m above sea level) lakes monitored in New Zealand are in poor or very poor health as measured by Trophic Level Index (Land and Water Aotearoa, see https://www.lawa.org.nz/explore-data/lakes/). An increasing number of these water bodies have switched (‘flipped’) from a desirable clear-water state supporting the growth of extensive beds of submerged rooted plants (Ministry for the Environment and Stats NZ 2017), to a degraded and undesirable state dominated by turbid water and planktonic algal blooms. Both the algal and macrophyte-dominated states are recognised as stable states that are rather resilient to change (Scheffer et al. 1993) due to the creation of internal feedback loops that improve conditions for the dominant primary producer (Søndergaard et al. 2008; Hilt 2015). More recently, a potential third but less stable intermediate state was identified where shallow lakes move between being macrophyte dominated in spring and early summer and become algal dominated as summer progresses (Hilt et al. 2013).
The degradation of lakes is generally attributed to catchment land-use change. In New Zealand, this is primarily the enrichment and pollution of lakes resulting from increasingly intensive agriculture, with associated increased loads of nitrogen (N), phosphorus (P) and suspended sediments entering waterways (Howard-Williams et al. 2010; National Institute of Water and Atmospheric Research 2010; Abell et al. 2022). As well as biodiversity loss, lakes in this degraded state lose much of their social amenity value as they are unlikely to be safe for swimming or the gathering of food.
Although there is both a desire to restore degraded freshwaters and a statutory requirement (National Policy Statement for Freshwater Management, Ministry for the Environment 2020) to improve freshwaters, there are significant barriers to restoration, with complex interactions between those barriers (Moss 1990; Hilt et al. 2013). Additionally, there are many examples of failed lake restoration projects globally, which can make managers hesitant to commission restoration work (Abell et al. 2022).
There have been significant published works over the last decade in particular, that examine barriers to restoration, set limits or criteria for likely success based on water quality, as well as restoration approaches (e.g. Bakker et al. 2013; Spears et al. 2016; Verhofstad et al. 2017; Hilt et al. 2018; Sarvala et al. 2020; Blindow et al. 2021; Abell et al. 2022). Often restoration approaches focus on catchment management (which is important, e.g. Abell et al. 2022), but do not address in-water actions, with rather a wait-and-see approach to vegetation recovery (passive restoration). This could, in part, be driven by a desire to preserve the natural population genetic structure of lake vegetation (e.g. Wood et al. 2019) by ‘waiting’ for macrophytes to re-establish on their own from seedbanks, connected watersheds or introduction via waterfowl (Bakker et al. 2013).
Sustainable freshwater improvement requires the re-establishment of in-lake processes that improve and stabilise water quality and ecological diversity (Moss 1990; Scheffer et al. 1993), resulting in healthier lakes and an improved ecological condition (Søndergaard et al. 2007; Gulati et al. 2008). However, active in-lake re-establishment of macrophytes is still relatively infrequently undertaken, with managers citing uncertainty of outcome relative to the costs involved as a key reason for the lack of action (Hofstra et al. 2021). Without active in-lake restoration approaches, submerged vegetation may take long periods to recover (Jeppesen et al. 2007; Hilt et al. 2013) or macrophytes may fail to establish in the window of time where favourable conditions occur (e.g. sufficient water clarity), or may fail to perpetuate. Notably where vegetation begins to re-establish, positive feedbacks can occur that support the continuance of the conditions favourable to further native macrophyte recovery (Hilt et al. 2006).
This perspectives paper examines the barriers to the re-establishment of macrophytes, and what is required to mitigate or remove those barriers and improve the likelihood of restoration success. We propose that in addition to existing restoration approaches, knowledge of macrophyte ecological requirements and tolerances is key to their re-establishment and successful to lake restoration in the long term.
Causes of macrophyte loss in degraded lakes
In considering what is required to successfully re-establish macrophytes, we need first to consider what the causes of macrophyte loss are. These loss processes need to be addressed, as well as subsequent additional barriers to restoration that may have developed.
There are two overarching causes of decline: eutrophication and the impacts of non-native (alien) invasive species. For example, nutrient enrichment leads to increased algal production, which along with increased sediment loads, results in shading and eventual loss of macrophytes. The continued cycles of algal productivity and senescence, fuelled by internal P loads and seasonal P release (in stratified or polymictic systems) (Moss et al. 1996; Cooke et al. 2005; Hilt et al. 2013), also results in increasingly flocculent substrates, where native macrophyte seed banks become buried to depths that reduce future germination opportunities (de Winton and Clayton 1996; de Winton et al. 2000). In addition, flocculent substrates provide poor habitat (e.g. low density, highly organic sediment, Barko et al. 1986) to support macrophyte re-establishment (e.g. poor anchorage, anaerobic root zone) (de Winton and Champion 1993; Hilt et al. 2013). In the absence of established macrophytes, substrates are more readily resuspended by wind and wave action (Özkundakci and Allan 2019) providing a feedback loop of sustained poor water clarity that limits opportunity for native seedling recruitment.
The switch in lake stable state, resulting in the loss of macrophytes, can also be influenced by weed control activities, overgrazing by birds or cyprinid fish, and changes in water level or salinity (Moss et al. 1996).
Alien invasive species (AIS) often act in concert with the above stressors, but can also destabilise and lead to ecosystem degradation (or a lake regime shift) on their own (Schallenberg and Sorrell 2009). Both invasive fish and plants are drivers of native biodiversity loss (Closs et al. 2004). Examples include trophic cascades, when invasive fish species exacerbate eutrophication through ‘top-down’ processes, selectively grazing zooplankton which leads to unchecked phytoplankton growth. For example, perch and gambusia have been associated with reductions in water quality through predatory effects on zooplankton (Meiro et al. 2001; Rowe and Smith 2001; Nagdali and Gupta 2002; Rowe 2007; Smith and Lester 2007). For perch, impacts on zooplankton are most pronounced when the population structure is dominated by juvenile fish (<120 mm; Smith and Lester 2007). Adult life stages of invasive fish may either graze on macrophytes (if present, e.g. rudd, Scardinius erythrophthalmus) or disturb sediments (e.g. koi, Cyprinus rubrofuscus) exacerbating poor water clarity (Crivelli 1983; Roberts et al. 1995). Invasive plants (Egeria densa in particular) have similarly been responsible for lake regime shifts, following root die-off in anoxic sediments with subsequent lake-scale vegetation death (e.g. Champion 2002).
Examples of barriers to restoration and in-lake actions to restore degraded lakes
The primary in-lake barriers to the re-establishment of macrophytes are created initially by insufficient water clarity and propagule presence, and lack of suitable substrates, with myriad additional interrelated secondary biotic and abiotic factors that may exert different stressors on shallow lakes, providing resistance to restoration actions. Importantly, the factors involved with maintenance of the devegetated condition need to be considered separately from the causes of macrophyte decline (above) and alongside potential inertia preventing change of the algal-dominated state as a result of hysteresis (Spears et al. 2017; Hilt et al. 2018; Abell et al. 2022).
The complexity of interrelated biotic and abiotic factors requires the holistic integration of multiple approaches to support sustainable restoration. For example, there are well-recognised publications that have demonstrated that nutrient reduction alone is usually not sufficient to promote a shift to a macrophyte-dominated clear-water state (Jeppesen et al. 2007), with an additional ‘shock therapy’ necessary to facilitate this shift (Scheffer et al. 1993). Similarly, manipulation of the foodweb (biomanipulation) to promote top down (consumer) control of phytoplankton cannot be expected to be successful over longer periods in eutrophic shallow lakes (Benndorf et al. 2002), but rather the strongest effect of biomanipulation occurs when both fish and nutrients are reduced (Benndorf et al. 2002 with reference to pelagic foodwebs; McQueen et al. 1986). Even so, other authors recognise a need for fish removal, nutrient reduction and macrophyte re-establishment to reduce the likelihood of a relapse to poor lake conditions. For example, Søndergaard et al. (2007) state that insufficient external load reduction, internal phosphorus load and the absence of a stable submerged macrophyte community to stabilise the clear-water state are the most probable causes for a relapse (of lake condition) to earlier degraded conditions in restoration projects. Likewise, in another review, Gulati et al. (2008) also attributed the greater number of failures than successes in restoration projects, to insufficient nutrient reduction (external and internal P load), insufficient submersed macrophyte abundance, ineffective reduction of planktivorous fish and the presence of cyanobacteria that are inedible to daphniids (zooplankton). In addition, there is an increasing need to manage local stressors (e.g. nutrients, Graham et al. 2020) because there are synergistic effects with global stressors (CO2 enrichment) (Falkenberg et al. 2013) associated with climate change.
Examples of macrophyte restoration approaches in New Zealand include challenging sites in Te Waihora (Lake Ellesmere) where fluctuating water level, low light and wave exposure hamper macrophyte establishment. In this example, a wave barrier was installed to secure a ‘safe site’ for transplanted Ruppia, but the plants did not establish over the longer term (de Winton et al. 2017). Disturbance of another kind hampered the re-establishment of native vegetation in Lake Opouahi. In this case, submerged cages were established to protect germinating charophytes from being consumed by herbivorous fish. However, while protected from large herbivorous fish, the hard surfaces of the cages were favoured habitat for the native bullies (Gobiomorphus cotidianus), and the associated nest cleaning, guarding and fanning behaviour (e.g. Stott and Poulin 1996; Pepper 2015) was later attributed to the disturbance of delicate germling charophytes and their failure to persist beyond a season. The use of cages to provide safe sites, however, has been successfully demonstrated in other examples, such as Lake Rotomanuka with established plants (de Winton and Taumoepeau 2005).
In the examples above, sustainable re-establishment of macrophytes failed quite simply because the barriers to restoration were not adequately addressed. The challenges for macrophyte re-establishment, as evidenced by the examples (and other reviews), is that there is still considerable uncertainty of the outcome and the trajectory that restoration may take (Søndergaard et al. 2007; Matthews et al. 2009; Hilt et al. 2013; Gross and Hagy 2017). However, there are also generally agreed approaches or criteria to provide the best opportunity for success.
Criteria for success
Despite previous failures, we concur with the generally agreed approaches to provide the best opportunity for success, such as having a clear goal or understating of what the outcome is that is being sought from the restoration project and the timeline over which that is likely achievable (e.g. Carpenter and Lathrop 1999). In addition to selecting suitable lakes or waterbodies where (Fig. 1):
Catchment management has already been undertaken, reducing external nutrient and sediment inputs to acceptable levels (e.g. Moss et al. 1996; Jeppesen et al. 2007; Maberly et al. 2020; Abell et al. 2022),
AIS are absent or their impacts have or can be mitigated (National Institute of Water and Atmospheric Research, 2010),
Re-established macrophytes can be protected from browsing or wave damage (Smart and Dick 1999; Evelsizer and Turner 2006),
Internal nutrient loads are managed, for example, with chemical or physical techniques (e.g. Welch et al. 1988; Welch and Cooke 1999; Cooke et al. 2005; Hickey and Gibbs 2009; Pan et al. 2012; Lürling et al. 2016), or potentially with biomanipulation to improve water clarity (e.g. Meijer et al. 1999; Søndergaard et al. 2008).
Conceptual diagram illustrating the connections between lake condition and macrophyte ecological requirements for re-establishment. The black arrows represent the sequence of information required for restoration, whereas the grey arrows indicate in-lake actions to improve the likelihood of successful macrophyte re-establishment.
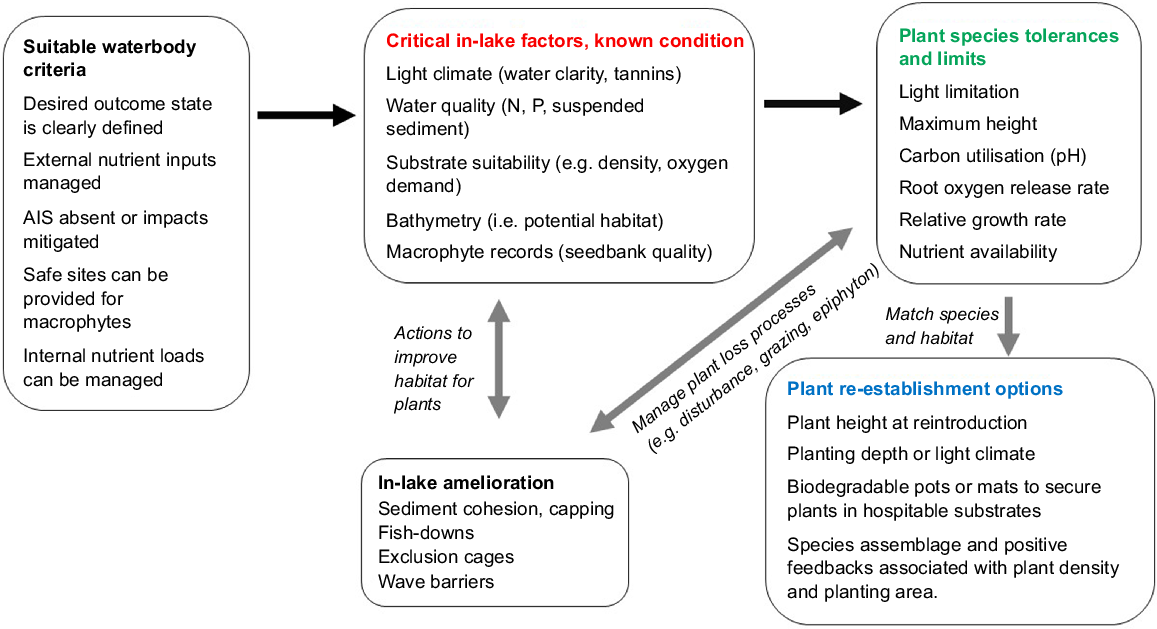
Once the above criteria are met, the guidelines of Hilt et al. (2006) start to address more macrophyte-specific (as opposed to general shallow lake restoration) criteria, including:
The potential area that could be colonised by macrophytes,
Identify the desired species, given the specifics of lake type, which species were historically present,
What the species habitat preferences are.
This necessitates having information on a range of in-lake conditions that can relatively easily be measured or determined. These are the ‘known’ or quantifiable factors that will influence macrophyte restoration actions, such as:
Light climate (water clarity)
Water quality (N and P content, suspended sediment, propensity for algal blooms which influence light climate)
Substrate suitability (e.g. density, cohesion)
Physical characteristics (e.g. size, shape, fetch, bathymetry as it relates to light climate and available habitat)
Macrophyte records and existing seedbank quality (diversity, abundance, viability) or availability of acceptable donor plants
The ‘known factors’ are critical to determining the optimal area of habitat for macrophytes, and whether prior lake restoration actions have been sufficient to create suitable light conditions for macrophytes to re-colonise (Bakker et al. 2013).
Matching the known habitat with the species tolerances and limits
At this point, of primary importance is the selection of plants, both in terms of species, their size (at re-introduction) and their habitat tolerances. To be able to habitat match species for re-establishment and improve the probability of successful restoration, there is a need to better understand the optimal environmental range within which a species can thrive, and the (suboptimal) limits at which it will only persist. To date there have been a limited number of studies that examine environmental requirements for native New Zealand macrophytes. Examples include a few studies with charophytes (Schwarz et al. 2000, 2002), and species of the genera of vascular macrophytes Myriophyllum (Rattray et al. 1991, 1994; Hussner et al. 2015), Potamogeton (Skovsholt et al. 2023), Stuckenia (Hawes et al. 2003; Hu et al. 2019) and Isoëtes (Sorrell 2004; Woodward and Hofstra 2022). In addition to experimental studies, some environmental preferences can be inferred by examining species distribution records (Hawes et al. 2003; Riis and Hawes 2003; Zabarte-Maeztu et al. 2023).
This fundamental ecological information (where available) could be used to inform in-lake interventions required to produce a suitable light climate and sediment density for establishment of the relevant species in the future. Suitable light climate may periodically occur naturally, when wave action is insufficient to resuspend bottom sediments or when these sediments are engineered using flocculating and capping agents to achieve the same effect (e.g. Cooke et al. 2005; Sarvala et al. 2020). Similarly, sediment density may be increased by capping, or using a range of plant species and propagule types in combination with biodegradable matting, which will provide the necessary anchorage in otherwise unsuitable sediments for plant growth (Gettys and Haller 2012; Hofstra 2021).
In addition, previous studies have found target areas for macrophyte establishment of 25% of the lake area coincided with low algal biomass (Norlin et al. 2005), whereas reductions in chlorophyll-a were reported with plant covers as little as 5–10% (Portielje and Van der Molen 1999). Certain species may provide greater environmental benefits than others. For example, Isoëtes kirkii plants were able to oxygenate sediment around their roots and significantly reduce mobilisation of phosphorus (Woodward and Hofstra 2022). This species, however, would not be considered a species for initial revegetation efforts, given its comparatively slow growth. However, establishment of assemblages of species (Hilt 2015) or translocation approaches (Hofstra 2021) that would enable its introduction are likely to confer additional ecosystem benefits (Woodward and Hofstra 2022). Determining the previous aquatic vegetation in the candidate lake can help inform the species targeted for restoration, although lake characteristics may have deteriorated to a state where plant species that were historically present are most unlikely to grow. Experimental determination of environmental limits of native macrophytes (discussed above), coupled with appropriate modelling (e.g. Spears et al. 2017), would inform their suitability for restoration. But experimental studies must be undertaken in the context of predicted climate change (Masson-Delmotte et al. 2021), or any subsequent restoration efforts are unlikely to be sustainable.
Establishing macrophyte species tolerances is currently a significant data gap, but one that could be systematically addressed through combinations of experimental and field studies and modelling. We advocate the use of experimental studies to better determine the tolerance range and limits of desirable native plants for restoration against a suite of relevant environmental conditions. These data could then be used to scenario test when the conditions are right for re-introduction, with greater certainty than is currently achievable, thus supporting the next foundational step enabling successful macrophyte re-establishment in shallow lakes and waterbodies.
Conclusions
The re-establishment of submerged vegetation in degraded, turbid algal-dominated lakes is critical to lake restoration. There are significant barriers preventing this goal, relating to poor water quality, low-density bottom sediments and the browsing impacts of fish and waterfowl, that will be further exacerbated by the warmer temperatures and more extreme weather events forecast under global climate change.
Restoration approaches need to be holistic, addressing all of these barriers, including reduction of nutrient and sediment inputs from the catchment, management of legacy in-lake nutrients and the impacts of invasive species. However, there have been few efforts to actively re-establish aquatic vegetation in degraded lakes in New Zealand. A combination of experimental and field studies determining the optimal environmental ranges of different native macrophytes, methods to culture suitable propagules en masse and techniques to promote sufficient water clarity and sediment density to sustain macrophyte growth are necessary. Data from experimental studies should be used to develop sustainable restoration approaches in the context of climate change predictions using a modelling approach to determine minimum vegetation cover thresholds that will improve the certainty (through scenario testing) of successful re-establishment of a stable macrophyte-dominated clear-water state.
Data availability
Data sharing is not applicable as no new data were generated or analysed during this study.
Declaration of funding
This work was supported by Strategic Science Investment Funding at NIWA (project FWBS2308) from the Ministry of Business, Innovation and Employment (MBIE), and the MBIE Endeavour Smart Idea (C01X2110).
Acknowledgements
We acknowledge and are grateful to our colleagues who have participated in many discussions on this topic in the past, and we appreciate the thoughtful comments of the reviewers.
References
Abell JM, Özkundakci D, Hamilton DP, Reeves P (2022) Restoring shallow lakes impaired by eutrophication: approaches, outcomes, and challenges. Critical Reviews in Environmental Science and Technology 52, 1199-1246.
| Crossref | Google Scholar |
Bakker ES, Sarneel JM, Gulati RD, Liu Z, van Donk E (2013) Restoring macrophyte diversity in shallow temperate lakes: biotic versus abiotic constraints. Hydrobiologia 710, 23-37.
| Crossref | Google Scholar |
Barko JW, Adams MS, Clesceri NL (1986) Environmental factors and their consideration in the management of submersed aquatic vegetation: a review. Journal of Aquatic Plant Management 24, 1-10.
| Google Scholar |
Benndorf J, Böing W, Koop J, Neubauer I (2002) Top-down control of phytoplankton: the role of time scale, lake depth and trophic state. Freshwater Biology 47, 2282-2295.
| Crossref | Google Scholar |
Blindow I, Carlsson M, van de Weyer K (2021) Re-establishment techniques and transplantations of charophytes to support threatened species. Plants 10, 1830.
| Crossref | Google Scholar | PubMed |
Carpenter SR, Lathrop R (1999) Lake restoration: capabilities and needs. Hydrobiologia 395, 19-28.
| Crossref | Google Scholar |
Champion PD (2002) Egeria densa – an alien invasive plant responsible for the loss of submersed vegetation from New Zealand shallow lakes. In ‘13th Australian weeds conference papers and proceedings’, 8–13 September 2002, Perth, WA, Australia. (Eds JH Spafford, J Dodd, JH Moore) pp. 126–129. (RG and FJ Richardson: Perth, WA, Australia) Available at https://caws.org.nz/old-site/awc/2002/awc200211261.pdf
Crivelli AJ (1983) The destruction of aquatic vegetation by carp. Hydrobiologia 106, 37-41.
| Crossref | Google Scholar |
de Winton MD, Champion PD (1993) The vegetation of the Lower Waikato lakes. Vol. 1. Factors affecting the vegetation of lakes in the Lower Waikato. NIWA Ecosystems Publication number 7. (National Institute of Water and Atmospheric Research: Hamilton, New Zealand) Available at https://docs.niwa.co.nz/library/public/NIWAEcospub7.pdf
de Winton MD, Clayton JS (1996) The impact of invasive submerged weed species on seed banks in lake sediments. Aquatic Botany 53, 31-45.
| Crossref | Google Scholar |
de Winton MD, Clayton JS, Champion PD (2000) Seedling emergence from seed banks of 15 New Zealand lakes with contrasting vegetation histories. Aquatic Botany 66, 181-194.
| Crossref | Google Scholar |
Evelsizer VD, Turner AM (2006) Species-specific responses of aquatic macrophytes to fish exclusion in a prairie marsh: a manipulative experiment. Wetlands 26(2), 430-437.
| Crossref | Google Scholar |
Falkenberg LJ, Connell SD, Russell BD (2013) Disrupting the effects of synergies between stressors: improved water quality dampens the effects of future CO2 on a marine habitat. Journal of Applied Ecology 50, 51-58.
| Crossref | Google Scholar |
Gettys LA, Haller WT (2012) Greenhouse culture of submersed aquatic vegetation “sod”. Proceedings Florida State Horticultural Science 125, 386-389.
| Google Scholar |
Graham E, Woodward B, Dudley B, Stevens L, Verburg P, Zeldis J, Hofstra D, Matheson F, Elliot S (2020) Consequences of inaction. Potential ramifications of delaying proposed nutrient source reductions for New Zealand rivers, lakes and estuaries. NIWA Client report 2020046HN, Project MFE20203, National Institute of Water and Atmospheric Research: Hamilton, New Zealand.
Gross C, Hagy JD, III (2017) Attributes of successful actions to restore lakes and estuaries degraded by nutrient pollution. Journal of Environmental Management 187, 122-136.
| Crossref | Google Scholar | PubMed |
Gulati RD, Dionisio Pires LM, Van Donk E (2008) Lake restoration studies: failures, bottlenecks and prospects of new ecotechnological measures. Limnologica 38, 233-247.
| Crossref | Google Scholar |
Hawes I, Riis T, Sutherland D, Flanagan M (2003) Physical constraints to aquatic plant growth in New Zealand lakes. Journal of Aquatic Plant Management 41, 44-52.
| Google Scholar |
Hickey CW, Gibbs MM (2009) Lake sediment phosphorus release management – decision support and risk assessment framework. New Zealand Journal of Marine and Freshwater Research 43(3), 819-856.
| Crossref | Google Scholar |
Hilt S (2015) Regime shifts between macrophytes and phytoplankton – concepts beyond shallow lakes, unravelling stabilizing mechanisms and practical consequences. Limnetica 34(2), 467-480.
| Crossref | Google Scholar |
Hilt S, Gross EM, Hupfer M, Morscheid H, Mählmann J, Melzer A, Poltz J, Sandrock S, Scharf E-M, Schneider S, van de Weyer K (2006) Restoration of submerged vegetation in shallow eutrophic lakes – a guideline and state of the art in Germany. Limnologica 36, 155-171.
| Crossref | Google Scholar |
Hilt S, Köhler J, Adrian R, Monaghan MT, Sayer CD (2013) Clear, crashing, turbid and back – long-term changes in macrophyte assemblages in a shallow lake. Freshwater Biology 58, 2027-2036.
| Crossref | Google Scholar |
Hilt S, Alirangues Nuñez MM, Bakker ES, Blindow I, Davidson TA, Gillefalk M, Hansson L-A, Janse JH, Janssen ABG, Jeppesen E, Kabus T, Kelly A, Köhler J, Lauridsen TL, Mooij WM, Noordhuis R, Phillips G, Rücker J, Schuster H-H, Søndergaard M, Teurlincx S, van de Weyer K, van Donk E, Waterstraat A, Willby N, Sayer CD (2018) Response of submerged macrophyte communities to external and internal restoration measures in north temperate shallow lakes. Frontiers in Plant Science 9, 194.
| Crossref | Google Scholar |
Hofstra D (2021) RotoTurf – seeding freshwater restoration. In ‘2021 Endeavour Round Successful Projects’. MBIE Endeavour Project. (Ministry of Business, Innovation and Employment) Available at https://www.mbie.govt.nz/assets/2021-endeavour-fund-successful-projects.pdf [Verified 18 March 2024]
Howard-Williams C, Davies-Colley R, Rutherford K, Wilcock R (2010) Diffuse pollution and freshwater degradation: New Zealand approaches to solutions. In ‘Issues and solutions to diffuse pollution’. (Eds E van Bochove, PA Vanrolleghem, PA Chambers, G Theriault, B Novotna, MR Burkart) pp. 126–140. (Organisation for Economic Co-operation and Development: Paris, France)
Hu Q, Turnbull M, Hawes I (2019) Estimated light compensation depth explains growth of Stuckenia pectinata in Te Waihora. Aquatic Botany 156, 57-64.
| Crossref | Google Scholar |
Hussner A, Hofstra D, Jahns P, Clayton J (2015) Response capacity to CO2 depletion rather than temperature and light effects explain the growth success of three alien Hydrocharitaceae compared with native Myriophyllum triphyllum in New Zealand. Aquatic Botany 120, 205-211.
| Crossref | Google Scholar |
Jeppesen E, Meerhoff M, Jacobsen BA, Hansen RS, Søndergaard M, Jensen JP, Lauridsen TL, Mazzeo N, Branco CWC (2007) Restoration of shallow lakes by nutrient control and biomanipulation – the successful strategy varies with lake size and climate. Hydrobiologia 581, 269-285.
| Crossref | Google Scholar |
Lürling M, Mackay E, Reitzel K, Spears BM (2016) Editorial – a critical perspective on geo-engineering for eutrophication management in lakes. Water Research 97, 1-10.
| Crossref | Google Scholar | PubMed |
Maberly SC, Pitt J-A, Davies PS, Carvalho L (2020) Nitrogen and phosphorus limitation and the management of small productive lakes. Inland Waters 10(2), 159-172.
| Crossref | Google Scholar |
McQueen DJ, Post JR, Mills EL (1986) Trophic relationships in freshwater pelagic ecosystems. Canadian Journal of Fisheries and Aquatic Sciences 43, 1571-1581.
| Crossref | Google Scholar |
Masson-Delmotte V, Zhai P, Pirani A, Connors SL, Péan C, Berger S, Caud N, Chen Y, Goldfarb L, Gomis MI, Huang M, Leitzell K, Lonnoy E, Matthews JBR, Maycock TK, Waterfield T, Yelekçi O, Yu R, Zhou B (Eds) (2021) Summary for policymakers. In ‘Climate Change 2021: the Physical Science Basis. Contribution of Working Group I to the Sixth Assessment Report of the Intergovernmental Panel on Climate Change’. pp. 3−32. (Cambridge University Press: Cambridge, UK, and New York, NY, USA) doi:10.1017/9781009157896.001
Matthews J, Spyreas G, Endress A (2009) Trajectories of vegetation-based indicators used to assess wetland restoration progress. Ecological Applications 19(8), 2093-2107.
| Crossref | Google Scholar |
Meijer M-L, de Boois I, Scheffer M, Portielje R, Hosper H (1999) Biomanipulation in shallow lakes in The Netherlands: an evaluation of 18 case studies. Hydrobiologia 408, 13-30.
| Crossref | Google Scholar |
Meiro CL, Cabral JA, Marques JC (2001) Predation pressure of introduced mosquitofish (Gambusia holbrooki Girard), on the native zooplankton community. A case-study from representative habitats in the lower Mondego River Valley (Portugal). Limnetica 20, 279-292.
| Google Scholar |
Ministry for the Environment, Stats NZ (2017) New Zealand’s Environmental Reporting Series: our fresh water 2017: data to 2016. (Ministry for the Environment and Stats NZ) Available at https://environment.govt.nz/assets/Publications/Files/our-fresh-water-2017_1.pdf
Ministry for the Environment (2020) National Policy Statement for Freshwater Management 2020. January 2024. (New Zealand Government) Available at https://environment.govt.nz/assets/publications/National-Policy-Statement-for-Freshwater-Management-2020.pdf
Moss B (1990) Engineering and biological approaches to the restoration from eutrophication of shallow lakes in which aquatic plant communities are important components. Hydrobiologia 200/201, 367-377.
| Google Scholar |
Nagdali SS, Gupta PK (2002) Impact of mass mortality of a mosquito fish (Gambusia affinis) on the ecology of a freshwater eutrophic lake (Lake Naini Tal, India). Hydrobiologia 468, 45-51.
| Crossref | Google Scholar |
National Institute of Water and Atmospheric Research (2010) Waikato River Independent Scoping Study. NIWA Client report HAM2010-032 prepared for the Ministry for the Environment, Project MFE10201. (NIWA, Hamilton, New Zealand) Available at https://environment.govt.nz/assets/Publications/Files/wriss-final-report.pdf
Norlin JI, Bayley SE, Ross LCM (2005) Submerged macrophytes, zooplankton and the predominance of low- over high-chlorophyll states in western boreal, shallow-water wetlands. Freshwater Biology 50, 868-881.
| Crossref | Google Scholar |
Özkundakci D, Allan MG (2019) Patterns and drivers of spatio-temporal variability of suspended sediment in the Waikato lakes, New Zealand. New Zealand Journal of Marine and Freshwater Research 53(4), 536-554.
| Crossref | Google Scholar |
Pan G, Dai L, Li L, He L, Li H, Bi L, Gulati RD (2012) Reducing the recruitment of sedimented algae and nutrient release into the overlying water using modified soil/sand flocculation-capping in eutrophic lakes. Environmental Science & Technology 46(9), 5077-5084.
| Crossref | Google Scholar | PubMed |
Portielje R, Van der Molen DT (1999) Relationships between eutrophication variables: from nutrient loading to transparency. Hydrobiologia 408, 375-387.
| Crossref | Google Scholar |
Rattray MR, Howard-Williams C, Brown JMA (1991) Sediment and water as sources of nitrogen and phosphorus for submerged rooted aquatic macrophytes. Aquatic Botany 40, 225-237.
| Crossref | Google Scholar |
Rattray MR, Howard-Williams C, Brown JMA (1994) Rates of early growth of propagules of Lagarosiphon major and Myriophyllum triphyllum in lakes of differing trophic status. New Zealand Journal of Marine and Freshwater Research 28(3), 235-241.
| Crossref | Google Scholar |
Riis T, Hawes I (2003) Effect of wave exposure on vegetation abundance, richness and depth distribution of shallow water plants in a New Zealand lake. Freshwater Biology 48, 75-87.
| Crossref | Google Scholar |
Roberts J, Chick A, Oswald L, Thompson P (1995) Effect of carp, Cyprinus carpio L., an exotic benthivorous fish, on aquatic plants and water quality in experimental ponds. Marine and Freshwater Research 46, 1171-1180.
| Crossref | Google Scholar |
Rowe DK (2007) Exotic fish introductions and the decline of water clarity in small North Island, New Zealand lakes: a multi-species problem. Hydrobiologia 583, 345-358.
| Crossref | Google Scholar |
Sarvala J, Helminen H, Heikkilä J (2020) Invasive submerged macrophytes complicate management of a shallow boreal lake: a 42-year history of monitoring and restoration attempts in Littoistenjärvi, SW Finland. Hydrobiologia 847, 4575-4599.
| Crossref | Google Scholar |
Schallenberg M, Sorrell B (2009) Regime shifts between clear and turbid water in New Zealand lakes: environmental correlates and implications for management and restoration. New Zealand Journal of Marine and Freshwater Research 43, 701-712.
| Crossref | Google Scholar |
Scheffer M, Hosper SH, Meijer M-L, Moss B, Jeppesen E (1993) Alternative equilibria in shallow lakes. Trends in Ecology & Evolution 8(8), 275-279.
| Crossref | Google Scholar | PubMed |
Schwarz A-M, Howard-Williams C, Clayton J (2000) Analysis of relationships between maximum depth limits of aquatic plants and underwater light in 63 New Zealand lakes. New Zealand Journal of Marine and Freshwater Research 34(1), 157-174.
| Crossref | Google Scholar |
Schwarz A-M, de Winton M, Hawes I (2002) Species-specific depth zonation in New Zealand charophytes as a function of light availability. Aquatic Botany 72, 209-217.
| Crossref | Google Scholar |
Skovsholt LJ, Riis T, Matheson F, Hawes I (2023) Growth response to nitrate enrichment helps facilitate success of an alien Potamogeton in New Zealand streams. Heliyon 9, e15528.
| Crossref | Google Scholar |
Smith KF, Lester PJ (2007) Trophic interactions promote dominance by cyanobacteria (Anabaena spp.) in the pelagic zone of lower Karori reservoir, Wellington, New Zealand. New Zealand Journal of Marine and Freshwater Research 41, 143-155.
| Crossref | Google Scholar |
Sorrell BK (2004) Regulation of root anaerobiosis and carbon translocation by light and root aeration in Isoetes alpinus. Plant, Cell & Environments 27, 1102-1111.
| Crossref | Google Scholar |
Spears BM, Mackay EB, Yasseri S, Gunn IDM, Waters KE, Andrews C, Cole S, de Ville M, Kelly A, Meis S, Moore AL, Nurnberg GK, van Oosterhout F, Pitt J-A, Madgwick G, Woods HJ, Lürling M (2016) A meta-analysis of water quality and aquatic macrophyte responses in 18 lakes treated with lanthanum modified bentonite (Phoslock). Water Research 97, 111-121.
| Crossref | Google Scholar | PubMed |
Spears BM, Futter MN, Jeppesen E, Huser BJ, Ives S, Davidson TA, Adrian R, Angeler DG, Burthe SJ, Carvalho L, Daunt F, Gsell AS, Hessen DO, Janssen ABG, Mackay EB, May L, Moorhouse H, Olsen S, Søndergaard M, Woods H, Thackeray SJ (2017) Ecological resilience in lakes and the conjunction fallacy. Nature Ecology & Evolution 1, 1616-1624.
| Crossref | Google Scholar | PubMed |
Stott MK, Poulin R (1996) Parasites and parental care in male upland bullies (Eleotridae). Journal of Fish Biology 48, 283-291.
| Crossref | Google Scholar |
Søndergaard M, Jeppesen E, Lauridson TL, Skov C, Van Nes EH, Roijackers R, Lammens E, Portielje R (2007) Lake restoration: successes, failures and long-term effects. Journal of Applied Ecology 44, 1095-1105.
| Crossref | Google Scholar |
Søndergaard M, Liboriussen L, Pedersen AR., Jeppesen E (2008) Lake restoration by fish removal: short- and long-term effects in 36 Danish lakes. Ecosystems 11(8), 1291-1305.
| Crossref | Google Scholar |
Verhofstad MJJM, Alirangues Núñez MM, Reichman EP, van Donk E, Lamers LPM, Bakker ES (2017) Mass development of monospecific submerged macrophyte vegetation after the restoration of shallow lakes: roles of light, sediment nutrient levels, and propagule density. Aquatic Botany 141, 29-38.
| Crossref | Google Scholar |
Welch EB, Cooke GD (1999) Effectiveness and longevity of phosphorus inactivation with alum. Lake and Reservoir Management 15(1), 5-27.
| Crossref | Google Scholar |
Welch EB, DeGasperi CL, Spyridakis DE, Belnick TJ (1988) Internal phosphorus loading and alum effectiveness in shallow lakes. Lake and Reservoir Management 4(2), 27-33.
| Crossref | Google Scholar |
Wood G, Marzinelli EM, Coleman MA, Campbell AH, Santini NS, Kajlich L, Verdura J, Wodak J, Steinberg PD, Vergés A (2019) Restoring subtidal marine macrophytes in the Anthropocene: trajectories and future-proofing. Marine and Freshwater Research 70, 936-951.
| Crossref | Google Scholar |
Woodward KB, Hofstra D (2022) Rhizosphere metabolism and its effect on phosphorus pools in the root zone of a submerged macrophyte, Isoëtes kirkii. Science of The Total Environment 809, 151087.
| Crossref | Google Scholar | PubMed |
Zabarte-Maeztu I, de Winton M, Hofstra D (2023) Understanding environmental drivers of New Zealand freshwater macrophyte traits. In ‘Freshwater Sciences 2023’, 3–7 June 2023, Brisbane, Qld, Australia. Oral presentation. Abstract available at https://sfs-afss-nzfss-2023.p.asnevents.com.au/days/2023-06-04/abstract/91074