Conditioning to predators improves survival of stocked Murray cod (Maccullochella peelii) fingerlings
Michael Hutchison

A Department of Primary Industries and Fisheries, Bribie Island Research Centre, PO Box 2066, Woorim, Qld 4507, Australia.
B Present address: The Gap, Qld, Australia.
Marine and Freshwater Research 74(12) 1039-1049 https://doi.org/10.1071/MF22242
Submitted: 11 November 2022 Accepted: 20 June 2023 Published: 14 July 2023
© 2023 The Author(s) (or their employer(s)). Published by CSIRO Publishing. This is an open access article distributed under the Creative Commons Attribution-NonCommercial-NoDerivatives 4.0 International License (CC BY-NC-ND)
Abstract
Context: Following recent major fish kill events, there is increasing interest in stocking Murray cod fingerlings to help fish stocks recover. Strategies that can increase post-release survival of stocked fingerlings are important for conservation stocking of Murray cod and may also have application to other species.
Aims: The aim of this work was to evaluate whether pre-release predator recognition and avoidance training conferred a survival advantage on stocked Murray cod (Maccullochella peelii) fingerlings. Predator-free release enclosures were also evaluated as a method to improve survival.
Methods: Fingerlings were trained by exposure to predators and skin extract. Fingerlings were marked with visual implant elastomer (VIE) tags to denote training status and release strategy (trained, untrained, soft release, standard release). Fingerlings were released at three sites in the northern Murray–Darling Basin. Marked fish were recaptured by electrofishing 24 h post-release and quarterly up to 15 months post-release.
Key results: Trained Murray cod had higher relative survival than did untrained fish. The mean recapture rate of trained fish was twice that of untrained fish. Fingerlings released directly into the waterbody had significantly higher recapture rates than did fingerlings acclimated for 90 min in predator-free enclosures.
Conclusions: Predator conditioning training provides a survival advantage to stocked Murray cod fingerlings. However, predator-free release enclosures conferred a disadvantage.
Implications: Pre-release training of Murray cod fingerlings could be beneficial to conservation and recreational-fish stocking programs. We recommend stocking trained cod fingerlings directly into the receiving environment, rather than into soft-release enclosures, so as to maximise their chance of survival.
Keywords: acclimation, hatchery-reared fish, Maccullochella, post-release survival, predation, predator conditioning, stocking, threatened species.
Introduction
Murray cod (Maccullochella peelii) is Australia’s largest freshwater fish species (Lintermans 2007). It is a member of the family Percichthyidae and is endemic to the Murray–Darling Basin (MDB), where it is the basis of a popular recreational fishery (Lintermans 2007). Murray cod is listed as vulnerable under the Australian Environmental Protection and Biodiversity Conservation Act 1999 (Commonwealth of Australia 2016) and is locally extinct in the Paroo River system (Sarac et al. 2011). Following major fish kills in the summer of 2018–2019, caused by a catastrophic hypoxia event in the lower Darling River (Baldwin 2019; Ellis et al. 2022), restocking is one tool being used to try to assist recovery of Murray cod (Ellis et al. 2022). In recent decades, stocking of fingerlings has been extensively used to create angling opportunities for this species in impoundments or to restore and enhance populations in rivers (Hollaway and Hamlyn 2001; Rowland 2013), but the results of stocking have been mixed (Forbes et al. 2016).
Hatchery-reared native fishes are extensively used for conservation and recreational-fishing enhancement programs. However, stocking of hatchery-reared fish does not always lead to the expected improvements in fish stocks (Larscheid et al. 1995; Blaxter 2000; Hutchison et al. 2006). Poor post-release survival rates of hatchery-reared fishes have been observed by fisheries scientists for over a century (Brown and Day 2002). Svåsand et al. (2000) concluded that more than 100 years of stocking cod (Gadus morhua) in the Atlantic did not lead to any significant increases in cod production or catches. Brown and Laland (2001) provided evidence that hatchery-reared fish have reduced post-release survival rates compared with wild fish. Rearing practices, and the artificial environments used to raise fish in hatcheries, can lead to domestication effects. These can include reduced capacity to recognise and avoid predators and foraging behavioural deficits that reduce survivorship of fish stocked into natural environments (Olla et al. 1994; Stickney 1994). This provides lower returns from stocking to anglers than might be expected and poor outcomes for conservation restocking efforts. Brown and Laland (2001) noted in their review paper that mortality rates were highest in hatchery-reared fish within the first few days after stocking.
Life-skills such as foraging, predator avoidance and reproductive behaviour, once thought inherited or instinctive, are now believed to have a significant learned component. This includes social learning from other fish (Jonsson 1997; Brown and Laland 2001, 2003). The absence of natural conditions and experienced conspecifics in a hatchery environment can reduce natural behaviours and social learning in hatchery-reared fishes (Brown and Day 2002). A key deficit in hatchery-reared fish is their failure to recognise and respond appropriately to predators. This has been confirmed in a variety of marine and freshwater fish species (Stunz and Minello 2001; Álvarez and Nicieza 2003; Malavasi et al. 2004; Ebner et al. 2006; Tang et al. 2017). This behavioural deficit is likely to arise because under most hatchery-rearing conditions, fish are reared without exposure to predators, and are therefore naïve to predation when stocked.
The naïveté of hatchery-reared fish, combined with transport-related stress, has the potential to make stocked fingerlings more susceptible to predation when stocked into the wild. Acclimation to the release site in a predator-free enclosure, where fish can overcome transport stress, has been demonstrated to increase post-release survival in some fish species (Brown and Day 2002; Brennan et al. 2006; Schlechte and Buckmeier 2006).
Suboski and Templeton (1989) suggested that large-scale training for predator recognition may be feasible, effective and economical before hatchery-reared fish are released into the wild. In this paper, we examine whether large-scale pre-release predator conditioning of Murray cod fingerlings in tanks can lead to improved survival after release into the wild. We also investigate whether a soft-release technique, where fingerlings are temporarily protected (for 90 min) from predators inside an enclosure, to overcome handling and transport stress, improves post-release survival of Murray cod fingerlings.
Actions that can improve post-release survival of stocked Murray cod will benefit both recreational fishing enhancement programs and conservation restocking programs that aim to recover Murray cod populations in catchments where their populations are threatened or have been extirpated.
Materials and methods
All experimental procedures were approved under Animal Ethics Permit CA 2007/08/207. Stocking of fish into the wild for the experiments was approved under a Fisheries Queensland General Fisheries Permit.
Field release sites
Three sites were selected in the northern MDB, Queensland, Australia, for experimental releases of hatchery-reared Murray cod fingerlings (Fig. 1). The sites were Cotswold Dam, a 250-ha dam on a small tributary stream, 800 m upstream of its junction with the Condamine River; Caliguel Lagoon, an 18-ha permanent waterhole backed by a low weir on an anabranch of the Condamine River, near Condamine township; and Storm King Dam, a 70-ha dam on Quart Pot Creek in the upper Dumaresq catchment near the town of Stanthorpe.
Care and tagging of fingerlings
Approximately 18 000 Murray cod fingerlings were purchased from a commercial hatchery (Granite Belt Fish Hatchery). Transport from the hatchery took approximately 3 h. Fingerlings were held in four equal-sized groups in 5000-L tanks with trickled-through (10 L min−1) UV-treated, activated-carbon-filtered freshwater to maintain water quality. Fingerlings were fed to satiation daily on a mixture of commercial pellet feeds and frozen blood worms. After a 3-day settling-in period, fingerlings were tagged with fluorescent visible implant elastomer tags (VIE, supplied by Northwest Marine Technology Inc.) at the base of the anal fin. VIE tags are reported to have no significant effect on predation rates of fingerlings (Roberts and Kilpatrick 2004; Reeves and Buckmeier 2009; Bouska and Paukert 2010).
Each treatment group was tagged with a different VIE tag colour (Table 1) to indicate whether fish were trained to recognise predators or untrained controls, and to denote whether the trained or untrained fish were to be released into predator-free habituation enclosures (soft release) or directly into the waterbody (standard release).
Item | Predator-free cage prior to release (soft release) | Standard release |
---|---|---|
Predator trained | Green tag 1350 fingerlings per site | Red tag 1350 fingerlings per site |
Predator naïve | Orange tag 1350 fingerlings per site | Pink tag 1350 fingerlings per site |
Numbers tagged exceeded 1400 per site for each treatment to allow for potential losses during training, holding and transport.
Predator-awareness training
Fingerlings were given at least 48 h to recover from tagging before being conditioned to predatory fish. Fish predator-awareness training for the non-control treatment groups was conducted in a 5000-L fibreglass tank provided with cover areas and a mesh screen permeable to fingerlings, but not to predators (Fig. 2). The permeable screen was fitted with a solid removable PVC sheet. The solid sheet could be inserted at any time to reduce predation rates or hide predators from view. Predatory fish (one subadult Murray cod, one adult golden perch and one large spangled perch per tank) were collected from the wild and kept together on one side of the screen for at least 2 weeks prior to introduction of fingerlings to ensure that they were behaving and feeding normally. These wild fish were fed a diet of frozen prawns and small fish purchased from commercial suppliers. Prawns and fish were thawed prior to feeding.
Tank system used for training fingerlings. Note the solid removable PVC screen (partially removed) used to block the permeable screen. Fingerlings could pass through the permeable screen, but predators could not.
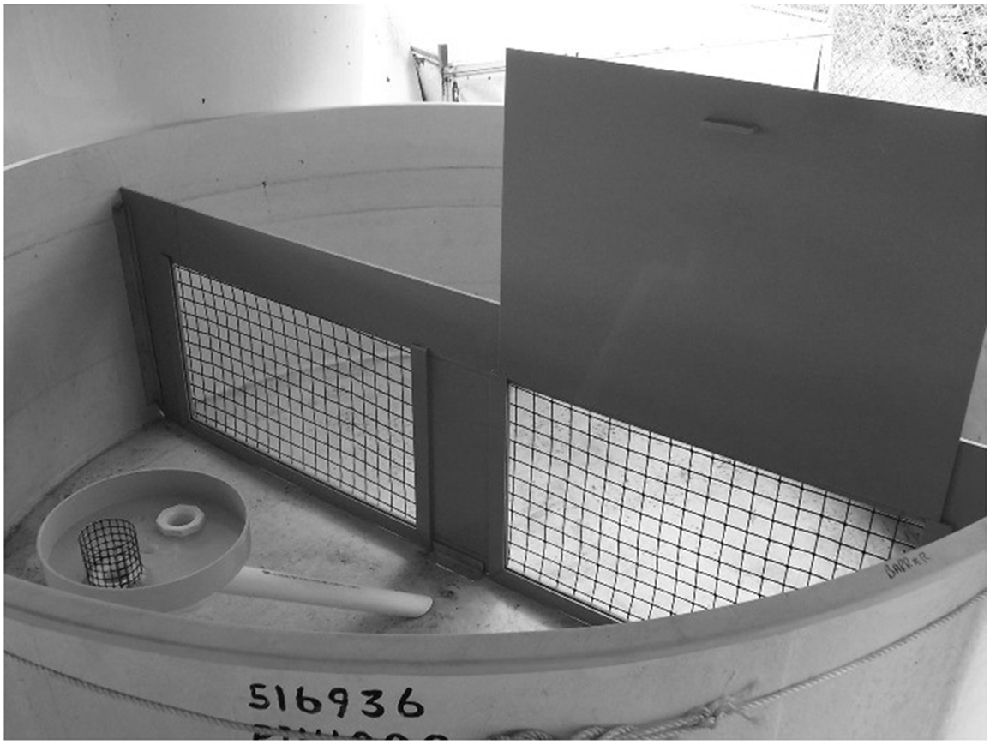
Up to 1400 fingerlings (enough fish for a single treatment at a site) were introduced to the predator-free side of the training tank at 08:00 hours local time. After a 5-min settling in period, the solid sheet was removed, exposing the permeable screen. Fingerlings were then free to swim through the permeable screen to the predator side of the tank and predators could chase and prey on fingerlings that strayed into their side of the tank. Fingerlings were provided with anchored strips of shade cloth (to mimic macrophytes) as cover on the predator-free side of the training tank. Fingerlings remained in the training tank for 3 days. Shortly after introduction of fingerlings to the tank, 40 mL of skin extract from Murray cod containing alarm pheromone (Ferrari and Chivers 2006) was added to the water on the predator side of the tank. This was repeated at 12:00 and 15:00 hours on Day 1, and at 09:00, 12:00 and 15:00 hours over the following 2 days. Two training tanks were used simultaneously. One tank was used to train fish bound for soft release, and the second identical tank was used to train fish bound for standard release. Untrained control fish were maintained in two separate predator-free 5000-L tanks at the same densities as the trained fish. One group was destined for standard release and the other for soft release. Between 2 and 3% of fingerlings were lost to predators in the training tanks over the training period.
Transport to field sites
After 3 days of training, fish were removed from the tank, and counted into labelled (by treatment) oxygenated double bags for transport to the field sites. Bags were 60 L in capacity and filled with 20 L of water. The two untrained groups were also counted into labelled oxygenated bags. Densities were ~140 fish per bag. Fish were transported to the release sites in an airconditioned vehicle. The time to travel to each of the sites ranged from 3 to 4 h.
The transport method was modified after the delivery to Cotswold Dam by moving fingerlings in four labelled, insulated 400-L fibreglass-tank compartments, supplied with bubbled oxygen and air. One compartment containing 1300–1400 fish was used per treatment.
Release
On arrival at the release site, two predator-proof enclosures (Fig. 3) were installed in each of the trained-fish and untrained-fish release zones. Enclosures were 1.8 m in diameter, with a 1.6-m drop to an open-ended lead-lined skirt. A pool-noodle float was cable-tied to a 1.8-m diameter polypipe ring at the top of the enclosure to keep the top of the enclosure at the surface. A second polypipe ring was set approximately three quarters of the way down the enclosure, to keep the interior of the enclosure open. Enclosures were set at a depth of 1.2 m. The lead skirt followed the bottom contours, which prevented fingerling escape while excluding predators. A floating aerator pump was installed in each enclosure, and a floating plastic cover was added to provide protection from bird predation. Enclosures were set at least 50 m apart to reduce the risk of all fish being released next to a high concentration of predators, or into an area devoid of predators. Trained fish were released at least 1 km apart from untrained fish (Fig. 4) to minimise the behaviour of trained fish influencing untrained fish. Standard (hard)-release fish were stocked directly into the waterbody, at least 200 m from fish released into enclosures. Each training group (trained or untrained) was released in two batches, 50 m apart, to minimise predator feeding interactions among release sites.
Release enclosure. Note the pool-noodle float, zip-tied to a poly-pipe ring at the top of the enclosure, a second poly-pipe ring near the bottom of the enclosure to keep the enclosure open, and an open lead-lined skirt at the base of the enclosure.
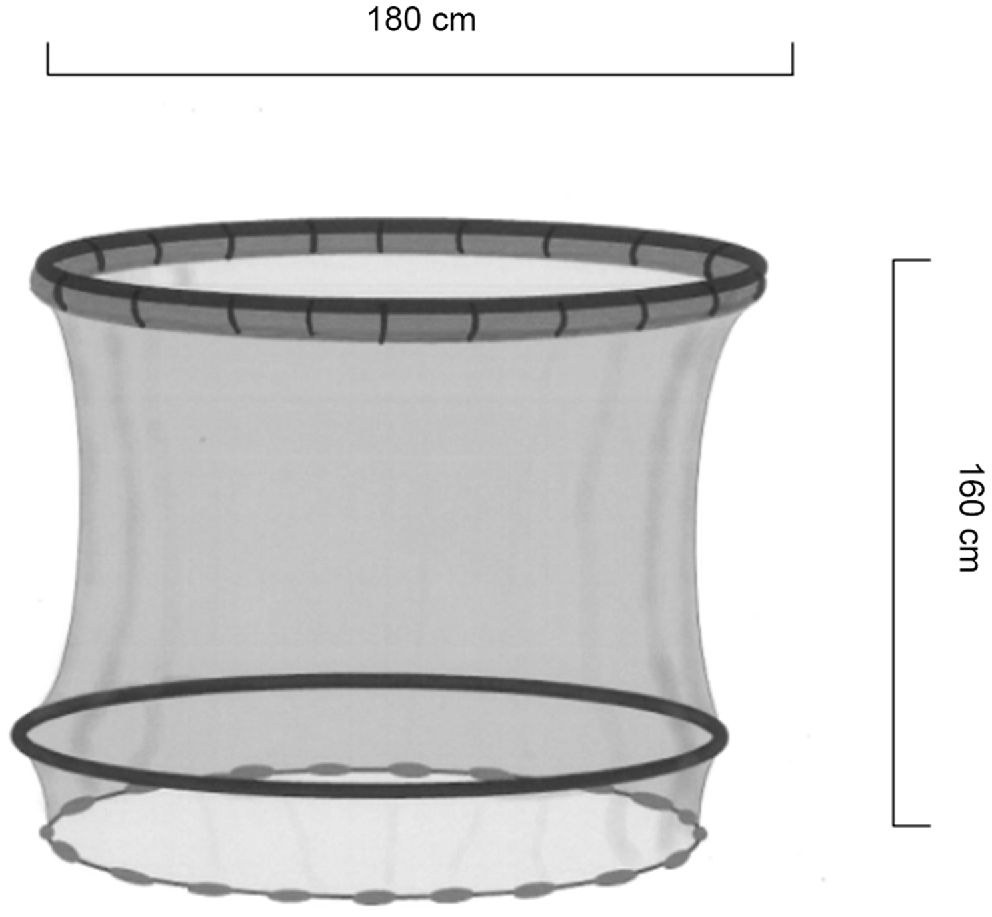
Schematic diagram showing spacing of the different release strategies for Murray cod fingerlings. Soft-release and standard-release sites were separated by at least 200 m. Trained and untrained fingerlings were released at least 1 km apart and fingerlings in the same treatment group were released at two points at least 50 m apart. Not to scale.
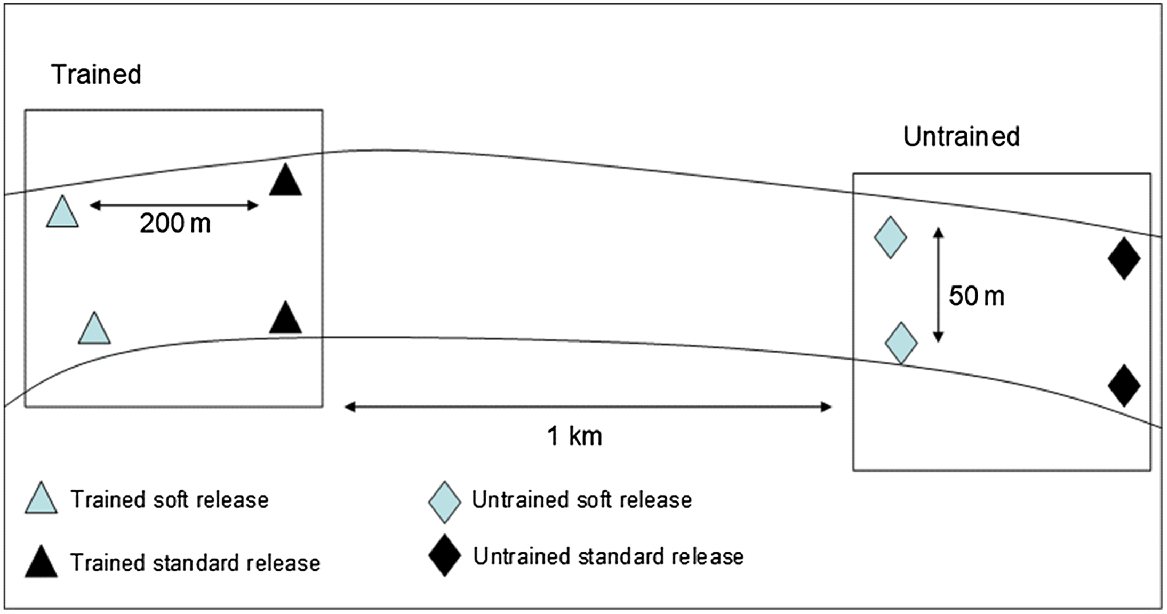
Prior to stocking, each batch of fish was loaded into plastic mesh-sided cages in the live well of a 5.4-m electrofishing vessel. Ambient water was pumped through the live well to allow fish to adjust to the local water chemistry, while the boat motored to the predefined release points. The acclimation period was ~10 min.
Fish stocked into release enclosures were left in the enclosures for 90 min. This period was based on the findings of Olla and Davis (1989) that coho salmon (Oncorhynchus kisutch) was able to overcome transport stress in predator-free enclosures after 90 min. After 90 min, enclosures were lifted vertically onto a boat, enabling fish to swim away from the enclosure site. The mean total length (TL) ± s.e.m. of Murray cod stocked into Caliguel Lagoon and Cotswold Dam was 66.1 mm (± 12.5 mm) and 63.8 mm (± 3.2 mm) for Murray cod stocked into Storm King Dam. At the time of release, any transport mortalities were tallied, and the total number released alive in each batch was recorded. Transport mortality rates among the different treatment groups were analysed by one-way ANOVA, using Genstat (ver. 22, VSN International).
Post-release surveys
Post-release surveys commenced 24 h after each stocking event. The first post-release survey at each site was by a 5.4-m electrofishing boat, with a 7.5-kVA generator, with two dip netters operating from the bow. Surveys commenced at dusk and continued until after midnight. Experience has shown that fingerlings tend to move into the shallow margins at night where they are more susceptible to electrofishing. Surveys commenced within 50 m of each release point. Both potential predators of fingerlings and recaptured fingerlings were recorded in the vicinity of each release point. Any recaptured fingerlings were held temporarily in an on-board 300-L live-well with flow-through water. At the end of each sampling shot, fingerlings were sedated with AquiS™ (Melling, Lower Hutt, New Zealand) at a rate of 0.025 mL L−1, measured to TL, then checked for a VIE tag with a blue light torch. Tag colour was confirmed by a minimum of two observers and compared with reference tags on a plastic fish provided by Northwest Marine Technology, Inc. (Ancortes, WA, USA). The tally of tag colours was recorded onto a data sheet. After recovery from sedation, recaptured fingerlings were released.
Predator numbers at release points were not assessed at the time of stocking to avoid confounding post-release predatory behaviour. A review of the published literature infers that the bulk of predation would occur on the first day after release (e.g. Buckmeier et al. 2005; Hutchison et al. 2006; Sparrevohn and Støttrup 2007). Predator index scoring was confined to 24 h after stocking, on the basis of the assumption that there was some degree of short-term site fidelity by the predators. Such behaviour has been reported in radiotelemetry studies for spangled perch (Hutchison et al. 2008), golden perch (Crook 2004), common carp (Crook 2004; Jones and Stuart 2007) and Murray cod (Jones and Stuart 2007). The numbers and species of predators within 50 m of each release point were used to calculate a predator index for the release points of each release strategy.
Only species large enough to potentially prey on the fingerlings were scored for the predator index. Most predators had to be at least 150 mm fork length (FL), but carp (Cyprinus carpio) and goldfish (Carassius auratus) had to be at least 200 mm FL. Common carp and goldfish were given a weighting of one, because they are only occasional piscivores (Morgan and Beatty 2007; Vejřík et al. 2016), whereas spangled perch, golden perch, Murray cod and freshwater catfish (Tandanus tandanus) were given a weighting of three. The number of each potential predatory fish species was multiplied by its weighting. Thus, the predator index was calculated as follows:
where PI is the predator index, N is the number of a particular species of predator recorded at a site, the subscript numbers represent the different predator species and W is the weighting score given to each species of predator. The scores for each species were summed to give a total score for the release point. One was added to the total score for each release site to prevent any zero scores. The predator index score was used as a parameter in the statistical analyses of recapture results.
Predator numbers more than 50 m from release points were not assessed because the assumption was that predators near release points would have a greater effect on survival than would predators away from the release points, because those closest to release points are more likely to encounter fish that are still recovering from transport stress.
Following the 24-h post-stocking survey, further post-stocking surveys were conducted quarterly by boat electrofishing to investigate longer-term survival rates. Follow-up surveys generally consisted of sampling over two nights per site, covering as much ground as possible to maximise recaptures of tagged fish.
Total sampling effort varied slightly across sites, owing to access difficulties post-flooding at some sites. Effort was recorded as total sampling nights and entered as a covariate in the analyses of the data (see below). Recapture ratios of the different treatment groups were assumed to represent relative survival rates. As fingerlings were batch tagged to represent treatments, and not individually tagged, it is possible that some fish could have been recaptured more than once. We believe that the total catch rates will still reflect the relative survival rates of the different treatments because any effect would have been consistent across treatments.
After processing, recaptured fish were released near to the areas where they were captured. The electrofishing boat would then move on to sample new ground, to minimise the chance of recapturing the same batch-marked individuals. Some supplementary sampling was conducted by backpack electrofishing and fyke netting, to sample areas less accessible by electrofishing boat, but these methods were less efficient, accounting for less than 1% of the total catch.
Statistical analyses of recapture data
Data on the relative recapture rates of the different batches of fish were analysed with Genstat (ver. 9.2, VSN International), by using a generalised linear model (GLM) (McCullagh and Nelder 1989) with binomial distribution and a logit link function. The model used actual recaptures as a proportion of the number of fish stocked in each category at each site. The maximal model was set with site, predator index, training status, release method and sampling effort as parameters. All factors in the GLM were fixed effects and of specific interest. Sampling effort, based on the number of sampling nights (4–6), was included as a standardising covariate. The significance of the design terms in the model (release method, training status, site and predator-index), along with their respective interaction effects, were assessed by a forward stepwise procedure. Non-significant terms were dropped for the final model, unless they were the specific treatments of interest to this study (i.e. release method and training status). Adjusted mean recapture rates were calculated for each stocking treatment by using the predict function in Genstat (ver. 9.2), adjusted for the effects of other covariates and factors in the model. The dispersion parameter was fixed at 1 (McCullagh and Nelder 1989).
Following sustained rainfall events after release of fingerlings, the backwaters of Cotswold Dam were flooded and remained backed up into the riparian zone for the duration of the sampling period. The extensively flooded and heavily timbered vegetated flats contained ideal cover for juvenile Murray cod, but were difficult to access and sample efficiently with an electrofishing boat, leading to lower than anticipated catch rates at that site. Data analyses were run for all three sites (Storm King Dam, Caliguel Lagoon and Cotswold Dam) and then repeated using data only from Caliguel Lagoon and Storm King Dam. The reason for the second analysis was to determine whether the low catch rates at Cotswold Dam (probably related to prevailing habitat availability) were masking any trends otherwise discernible at the sites with higher recapture rates. Recapture rates were used as indicators of relative survival and were not intended to be used as estimates of absolute survival.
Results
Mean transport mortality rates were less than 1% across all treatment groups and one-way ANOVA showed no significant difference in transport mortality rates among treatments (P = 0.543). In total, 203 VIE-marked Murray cod individuals were recaptured in this study. Very few unmarked fish in the appropriate size range were recaptured, except at Storm King Dam, where a local stocking group had released unmarked fingerlings in isolation of this research stocking. At Caliguel Lagoon where there was no supplementary stocking, 97.8% of cod captured had visible VIE tags.
Recaptures of Murray cod at Cotswold Dam were low, with just 16 fish recaptured. Recapture rates for the different treatments at Cotswold Dam ranged from 0 to 0.15%. In total, 136 tagged Murray cod individuals were recaptured at Caliguel Lagoon and 51 tagged Murray cod individuals at Storm King Dam, generating recapture rates of the different treatment groups ranging from 0.16 to 4.26%.
Predator indices ranged from 4 to 14 in Cotswold Dam, from 3 to 12 in Caliguel Lagoon and from 39 to 90 in Storm King Dam. High and low values were distributed across all treatment groups.
No significant interaction effects were found between release strategy and training status in any of the GLM models. The final GLM of binomial proportions for recaptures of Murray cod across all three sites showed several significant effects on recapture rates, including sampling effort, training status, release strategy and predator index. Adjusted mean values for recapture rates of trained fish (1.17%) were significantly (P = 0.012) higher than those for untrained fish (0.80%). Standard-released fish had higher adjusted mean recapture rates (1.16%) than did soft-released fish (0.82%). Site could not be included in the model because of aliasing with sampling effort. However, one or both accounted for the lower recapture rates at Cotswold Dam. The model is summarised in Table 2 and significance levels of the different parameters in the model are shown in Table 3.
Item | d.f. | Deviance | Mean deviance | Deviance ratio | χ2-probability |
---|---|---|---|---|---|
Regression | 4 | 174.53 | 46.632 | 43.63 | <0.001 |
Residual | 7 | 20.97 | 2.995 | ||
Total | 11 | 195.49 | 17.772 |
Dispersion parameter is fixed at 1.
Parameter | t-probability |
---|---|
Constant | <0.001 |
Sampling effort | <0.001 |
Status untrained | 0.012 |
Release standard | 0.015 |
Predator index | 0.047 |
Parameters for factors are compared with reference levels ‘Status trained’ and ‘Release soft’.
The final GLM of binomial proportions for Murray cod recaptures at Storm King Dam and Caliguel Lagoon showed that sampling effort was not a significant influence on total recaptures. In this model, release strategy, predator index and training status remained as significant effects. Adjusted mean values for recapture rates of trained fish were significantly higher than for untrained fish, and fish released by standard-release methods had higher recapture rates than did soft-released fish. The model is summarised in Table 4 and significance levels for the different parameters in the model are shown in Table 5. Adjusted mean recapture rates for Murray cod across the two sites by training status and release strategy are shown in Figs 5 and 6 respectively. Recapture rates for trained fish are almost double those of untrained fish.
Item | d.f. | Deviance | Mean deviance | Deviance ratio | χ2-probability |
---|---|---|---|---|---|
Regression | 3 | 104.103 | 34.701 | 34.7 | <0.001 |
Residual | 4 | 4.061 | 1.015 | ||
Total | 7 | 108.164 | 15.452 |
Dispersion parameter is fixed at 1.
Parameter | t-probability |
---|---|
Constant | <0.001 |
Predator index | <0.001 |
Predator index.Status untrained | 0.008 |
Release standard | 0.021 |
The parameter ‘Release standard’ is compared with the reference level ‘Release soft’.
Adjusted mean recapture rates by training status for Murray cod from Storm King Dam and Caliguel Lagoon. Means have been adjusted for fixed values of predator index (34.34) and marginal weights have been held constant over levels of other factors. Error bars show one standard error of the mean.
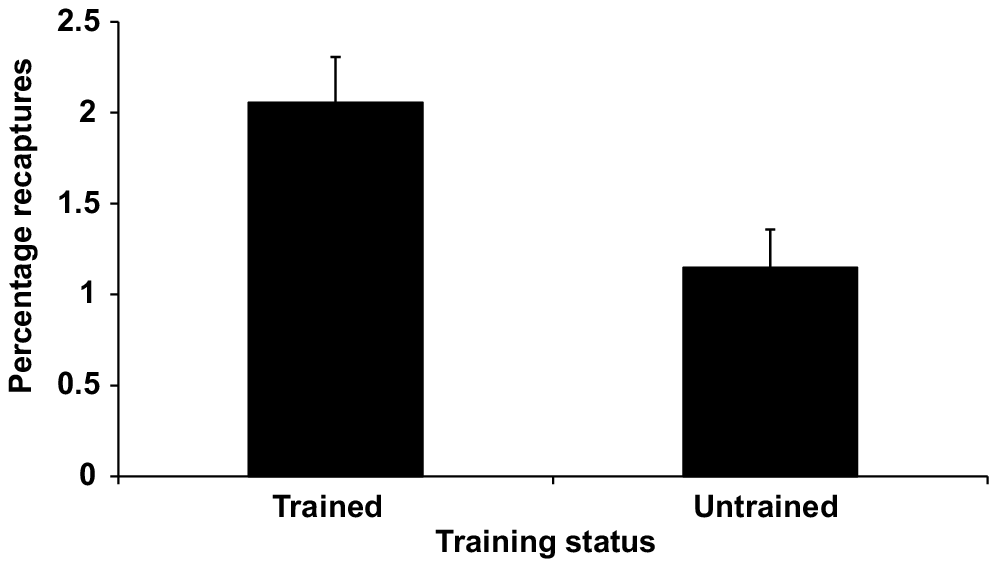
Adjusted mean recapture rates by release strategy for Murray cod from Storm King Dam, and Caliguel Lagoon. Means have been adjusted for fixed values of predator index (34.34) and marginal weights have been held constant over levels of other factors. Error bars show one standard error of the mean.
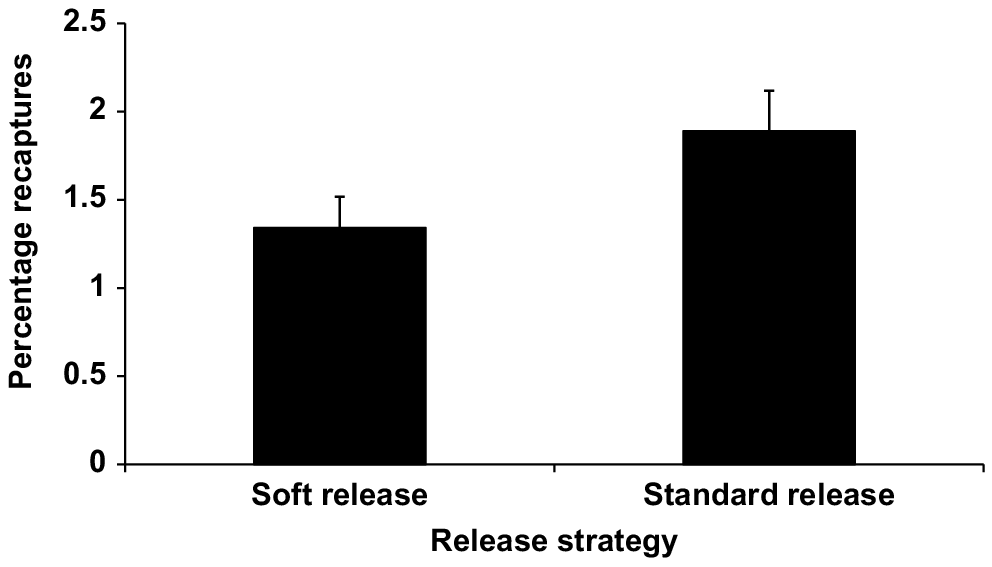
There was also a significant interaction between training status and predator index. This interaction suggests that at low levels of predator abundance, trained and untrained fish survived at similar rates, but as predator density increased, survival of untrained fish declined at a faster rate than for trained fish. The interaction between predator index and training status on recapture rates is shown in Fig. 7.
Interaction between predator index and training status of Murray cod stocked into Storm King Dam and Caliguel Lagoon. Recapture rates are adjusted mean values. Solid bars represent trained fish and open bars untrained fish. Marginal weights have been held constant over levels of other factors. Error bars show one standard error of the mean.
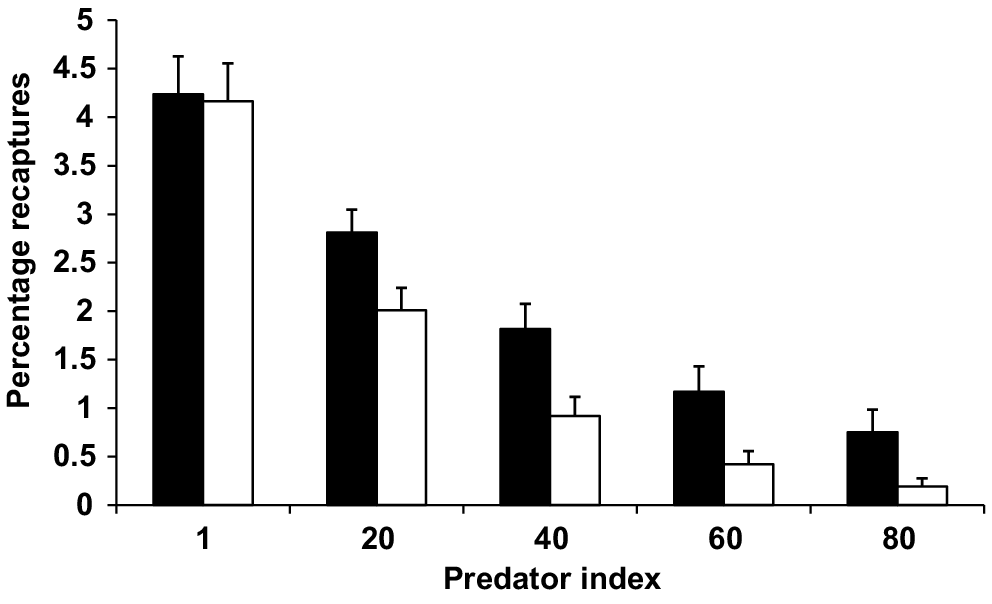
Discussion
Recapture rates of micro-tagged Murray cod at the non-flood-affected sites of Storm King Dam and Caliguel Lagoon were comparable to those reported from other studies of stocked fingerlings. For example, recapture rates have been reported to be between 0.4 and 6% for barramundi (Hutchison et al. 2006) and 3.23% for striped mullet (Leber et al. 1996). It was clear from our results that trained Murray cod had higher adjusted mean recapture rates (2.06%) than did untrained control fish (1.15%), with mean relative survival of trained fish being almost twice that of naïve control fish. Therefore, it can be concluded that pre-release predator training has conferred a survival advantage to the trained fingerlings. Where predator densities were higher, overall survival was reduced in both trained and untrained fish. However, trained fish fared up to four times better at high predator densities than did the untrained control fish. The benefits of training significantly outweighed the small amount of loss of fingerlings, which occurred during the training period. For logistical reasons, we did not use replicate training tanks, but given that all tanks were identical and held adjacent to each other under identical lighting conditions, it is unlikely that tank effects had any influence on the results.
Although recapture rates were low and may reflect low survival rates, a mean doubling in survival rates of trained cod fingerlings is important. Millions of Murray cod individuals are stocked in the Murray–Darling Basin every year. For example, in 2020, 3 647 980 Murray cod individuals were stocked in the state of Victoria alone (Victorian Fisheries Authority 2023). Therefore, even at a low overall post-release survival rate of 1%, a doubling of mean post-release survival to 2% as a result of pre-release predator conditioning could potentially mean tens of thousands more fish recruiting each year in conservation stocking and recreational-fishery enhancement programs, provided the habitat was there to support them.
The predator free release enclosures had an effect opposite to that expected, conferring a survival disadvantage to the stocked Murray cod fingerlings. The reason for this is not known; however, one possible explanation is that the aggregation of fingerlings within the release enclosures for 90 min may have drawn the attention of predators. Predatory green crabs (Carcinus maenas) were found to be attracted to acclimation cages containing winter flounder (Pseudopleuronectes americanus) juveniles being conditioned for release in the Hampton–Seabrook Estuary, New Hampshire (Fairchild et al. 2008). Thus, it is possible that predators may have been attracted to conditioning enclosures containing Murray cod fingerlings. If predators were attracted to, and aggregated around the Murray cod release enclosures, they may have been easily able to predate on the fingerlings when the enclosure was withdrawn from the water, despite the increased acclimation time the fingerlings gained within the enclosure.
Schlechte et al. (2005) found that habituation periods as short as 15 min in predator-free enclosures improved post-release survival in tank-based experiments, where Florida largemouth bass fingerlings (Micropterus salmoides floridanus) were exposed to predators (Schlechte et al. 2005). Given that a 90 min acclimation time in predator-free enclosures led to a decrease in survival relative to standard-released fish (which had no acclimation time in a predator-free enclosure), it is doubtful that extending the acclimation period beyond 90 min would have led to any improvements in Murray cod survival. However, there may be a benefit in undertaking further research on the effect of acclimation period duration in predator-free enclosures on post-release survival of Murray cod, to confirm whether or not shorter or longer acclimation times can lead to improvements in survival.
Alternative soft-release strategies may need to be developed to further enhance survival of predator-conditioned Murray cod. One possibility could be to target releases of fingerlings into constructed rocky reefs or into natural complex habitats. Habitat-preference experiments by Hutchison et al. (2020) showed that hatchery-reared Murray cod fingerlings have strong preference for rocky cover. Complex spaces in rocky reefs can provide protection for juvenile fish from piscivores (Miranda 2017). Whether releasing Murray cod fingerlings into complex habitats can improve post-release survival is worthy of future research. Russell et al. (2004) suggested that releasing barramundi (Lates calcarifer) fingerlings into complex habitat improved survival. By contrast, Stunz and Minello (2001) found that although wild juvenile red drum (Sciaenop ocellatus) had improved survival in structurally complex habitats such as sea grass, this was not the case for stocked hatchery-reared red drum.
Many studies have been able to demonstrate changed behaviours and improved predator response by predator-conditioned fish, compared with predator-naïve fish in laboratory-based or pond-based studies (e.g. Järvi and Uglem 1993; Brown et al. 1997; Arai et al. 2007; Walsh et al. 2014). However, this current study is one of the few (e.g. D’Anna et al. 2012) to demonstrate the benefits of pre-release predator conditioning for fish released in the wild. Archer and Crowl (2014) suggested that this lack of demonstrated benefit for trained fish stocked into the wild may be because trained fish do not retain association of cues for novel predators for extended periods, without ongoing reinforcement. For example, June sucker (Chasmistes liorus liorus) retained learning for at least 2 days but lost evidence of it by 10 days after initial exposure. In the case of the Murray cod stocking experiment, fish were transported and stocked immediately after the 3-day training period, and this may have negated any potential memory-deficit effects.
Berejikian et al. (2000) suggested that one of the problems with past attempts to assess the effects of training fingerlings on the success of field releases is that both trained and untrained fish have been released together. This enables the control fish to rapidly acquire anti-predator behaviour from the trained fish through social learning processes. The improved survivorship of the control fish offsets the apparent effect of the training procedure by reducing differences in mortality between test and control fish. We tried to avoid the effects of social learning by stocking trained and untrained Murray cod at release points at least 1 km apart. Furthermore, Murray cod is not a schooling species. They are territorial (Moffatt and Voller 2002) and cannibalistic (Ryan et al. 2007). During the 24 h post-stocking survey, Murray cod fingerlings were captured only within 500 m of their initial release points. The combination of this sedentary and territorial behaviour suggests that trained and untrained cod individuals were unlikely to intermingle in the first 24 h after stocking. Lack of intermingling would prevent social learning by untrained fish from trained fish.
We have been able to demonstrate that training Murray cod fingerlings en masse to avoid predators is feasible and has demonstrated benefits to post-release survival in the wild. We recommend using pre-release predator conditioning as a tool to enhance success of conservation stockings of Murray cod. It may also be a useful tool for recreational-fisheries enhancement stocking of Murray cod in impoundments. This pre-release conditioning method may also be appropriate for conservation stockings of fingerlings of the other three Maccullochella species (Mary River cod, Maccullochella mariensis; trout cod, Maccullochella macquariensis; and eastern freshwater cod, Maccullochella ikei), all of which are listed as threatened species under the under the Australian Environmental Protection and Biodiversity Conservation Act 1999 (Office of Parliamentary Counsel 2014).
We also recommend using three or four release points when stocking large batches of Murray cod. Predator index was a significant parameter explaining recapture rates of Murray cod. Although it is generally not possible to predict what predator densities are going to be at any given release point, this research highlighted their spatial variability throughout a waterbody. Predators of fingerlings may include piscivorous fish, birds, turtles and macro-crustaceans. We, therefore, recommend that for conservation stockings, more than one release point should be used to minimise the risk of predation. Developing an understanding of where predators might be distributed, or peak predation times may assist with improving future fingerling releases and could be another area of future research. For example, future fish stocking research could involve pre-release surveys of predator distributions by using non-intrusive techniques, such as high-end sonar, to help improve fingerling release strategies.
Stocking in large batches may have the advantage of helping swamp predators, such that reasonable numbers of fingerlings escape predation while acclimating to the receiving waters. The results clearly demonstrated that use of predator-free enclosures should be avoided when releasing Murray cod fingerlings. Future research should examine the benefits of releasing Murray cod into complex habitats such as rocky reefs, which may lead to further improvements in release strategies for this species.
Acknowledgements
We thank Danielle Stewart for assisting with care of fish during the experimental procedures prior to stocking and for assistance with field work. Mark McLennan, John Kirkwood and Angela Henderson all helped with transport and release of fish and with aspects of the fieldwork. David Mayer (DAF biometrician) provided advice on the statistical analyses. Special thanks go to the late Keith Chilcott who assisted with fish care and fish stocking and who drove the electrofishing boat during post-release surveys. Thanks go to Sian Breen, Jenny Shiau and David Nixon for useful comments on an early draft of this document.
References
Álvarez D, Nicieza AG (2003) Predator avoidance behaviour in wild and hatchery-reared brown trout: the role of experience and domestication. Journal of Fish Biology 63, 1565-1577.
| Crossref | Google Scholar |
Arai T, Tominaga O, Seikai T, Masuda R (2007) Observational learning improves predator avoidance in hatchery-reared Japanese flounder Paralichthys olivaceus juveniles. Journal of Sea Research 58, 59-64.
| Crossref | Google Scholar |
Archer SK, Crowl TA (2014) Retention of learned predator recognition in an endangered sucker Chasmistes liorus liorus. Aquatic Biology 20, 195-202.
| Crossref | Google Scholar |
Berejikian BA, Tezak EP, Flagg TA, LaRae AL, Kummerow E, Mahnken CVW (2000) Social dominance, growth, and habitat use of age-0 steelhead (Oncorhynchus mykiss) grown in enriched and conventional hatchery rearing environments. Canadian Journal of Fisheries and Aquatic Sciences 57, 628-636.
| Crossref | Google Scholar |
Blaxter JHS (2000) Enhancement of marine fish stocks. Advances in Marine Biology 38, 1-54.
| Crossref | Google Scholar |
Bouska WW, Paukert CP (2010) Effects of visible implant elastomer mark colour on the predation of red shiners by largemouth bass. Fisheries Management and Ecology 17, 294-296.
| Crossref | Google Scholar |
Brennan NP, Darcy MC, Leber KM (2006) Predator-free enclosures improve post-release survival of stocked common snook. Journal of Experimental Marine Biology and Ecology 335, 302-311.
| Crossref | Google Scholar |
Brown C, Day RL (2002) The future of stock enhancements: lessons for hatchery practice from conservation biology. Fish and Fisheries 3, 79-94.
| Crossref | Google Scholar |
Brown C, Laland K (2001) Social learning and life skills training for hatchery reared fish. Journal of Fish Biology 59, 471-493.
| Crossref | Google Scholar |
Brown C, Laland KN (2003) Social learning in fishes: a review. Fish and Fisheries 4, 280-288.
| Crossref | Google Scholar |
Brown GE, Chivers DP, Smith RJF (1997) Differential learning rates of chemical versus visual cues of a northern pike by fathead minnows in a natural habitat. Environmental Biology of Fishes 49, 89-96.
| Crossref | Google Scholar |
Buckmeier DL, Betsill RK, Schlechte JW (2005) Initial predation of stocked fingerling largemouth bass in a Texas reservoir and implications for improving stocking efficiency. North American Journal of Fisheries Management 25, 652-659.
| Crossref | Google Scholar |
Crook DA (2004) Movements associated with home-range establishment by two species of lowland river fish. Canadian Journal of Fisheries and Aquatic Sciences 61, 2183-2193.
| Crossref | Google Scholar |
D’Anna G, Giacalone VM, Vega Fernández T, Vaccaro AM, Pipitone C, Mirto S, Mazzola S, Badalamenti F (2012) Effects of predator and shelter conditioning on hatchery-reared white seabream Diplodus sargus (L., 1758) released at sea. Aquaculture 356-357, 91-97.
| Crossref | Google Scholar |
Ebner B, Thiem J, Lintermans M (2006) Fate of two-year old, hatchery-reared Maccullochella macquariensis, stocked into two upland rivers. In ‘An ecological approach to re-establishing Australian freshwater cod populations: an application to Trout cod in the Murrumbidgee catchment’. (Eds B Ebner, J Thiem, M Lintermans, D Gilligan) pp. 79–94. Final report to Fisheries Research and Development Corporation Project No. 2003/034. Parks, Conservation and Lands, Canberra, ACT, Australia.
Ellis I, Bates W(Badger), Martin S, McCrabb G, Koehn J, Heath P, Hardman D (2022) How fish kills affected traditional (Baakandji) and non-traditional communities on the Lower Darling–Baaka River. Marine and Freshwater Research 73, 259-268.
| Crossref | Google Scholar |
Fairchild EA, Rennels N, Howell WH (2008) Predators are attracted to acclimation cages used for winter flounder stock enhancement. Reviews in Fisheries Science 16, 262-268.
| Crossref | Google Scholar |
Ferrari MCO, Chivers DP (2006) Learning threat-sensitive predator avoidance: how do fathead minnows incorporate conflicting information? Animal Behaviour 71, 19-26.
| Crossref | Google Scholar |
Forbes J, Watts RJ, Robinson WA, Baumgartner LJ, McGuffie P, Cameron LM, Crook DA (2016) Assessment of stocking effectiveness for Murray cod (Maccullochella peelii) and golden perch (Macquaria ambigua) in rivers and impoundments of south-eastern Australia. Marine and Freshwater Research 67, 1410-1419.
| Crossref | Google Scholar |
Hutchison MJ, Gallagher T, Chilcott K, Simpson R, Aland G, Sellin M (2006) Impoundment stocking strategies for Australian native fishes in eastern and northern Australia: with an assessment of the value of scales as tags for stocked barramundi. Final report to Fisheries Research and Development Corporation (Project No. 98/221). Department of Primary Industries and Fisheries, Brisbane, Qld, Australia.
Hutchison M, Norris A, Nixon D (2020) Habitat preferences and habitat restoration options for small-bodied and juvenile fish species in the northern Murray–Darling Basin. Ecological Management & Restoration 21, 51-57.
| Crossref | Google Scholar |
Jones MJ, Stuart IG (2007) Movements and habitat use of common carp (Cyprinus carpio) and Murray cod (Maccullochella peelii peelii) juveniles in a large lowland Australian river. Ecology of Freshwater Fish 16, 210-220.
| Crossref | Google Scholar |
Jonsson B (1997) A review of ecological and behavioural interactions between cultured and wild Atlantic salmon. ICES Journal of Marine Science 54, 1031-1039.
| Crossref | Google Scholar |
Järvi T, Uglem I (1993) Predator training improves the anti-predator behaviour of hatchery reared Atlantic salmon (Salmo salar) smolt. Nordic Journal of Freshwater Research 68, 63-71.
| Google Scholar |
Leber KM, Arce SM, Sterritt DA, Brennan NP (1996) Marine stock enhancement potential in nursery habitats of striped mullet, Mugil cephalus in Hawaii. Fishery Bulletin 94, 452-471.
| Google Scholar |
Malavasi S, Georgalas V, Lugli M, Torricelli P, Mainardi D (2004) Differences in the pattern of antipredator behaviour between hatchery-reared and wild European sea bass juveniles. Journal of Fish Biology 65(s1), 143-155.
| Crossref | Google Scholar |
Morgan DL, Beatty SJ (2007) Feral goldfish (Carassius auratus) in Western Australia: a case study from the Vasse River. Journal of the Royal Society of Western Australia 90, 151-156.
| Google Scholar |
Olla BL, Davis MW (1989) The role of learning and stress in predator avoidance of hatchery-reared coho salmon (Oncorhynchus kisutch) juveniles. Aquaculture 76, 209-214.
| Crossref | Google Scholar |
Olla BL, Davis MW, Ryer CH (1994) Behavioural deficits in hatchery-reared fish: potential effects on survival following release. Aquaculture and Fisheries Management 25(Supplement 1), 19-34.
| Google Scholar |
Reeves KS, Buckmeier DL (2009) Mortality, predation, and tag visibility of fish marked with visible implant elastomer tags. North American Journal of Fisheries Management 29, 323-329.
| Crossref | Google Scholar |
Roberts JH, Kilpatrick JM (2004) Predator feeding preferences for a benthic stream fish: effects of visible injected marks. Journal of Freshwater Ecology 19, 531-538.
| Crossref | Google Scholar |
Russell DJ, Rimmer MA, McDougal AJ, Kistle SE, Johnston WL (2004) Stock enhancement of barramundi, Lates calcarifer (Bloch), in a coastal river system in northern Australia: stocking strategies, survival and benefit cost. In ‘Stock enhancement and sea ranching: developments, pitfalls and opportunities’. 2nd edn. (Eds KM Leber, S Kitada, HL Blankenship, T Svåsand) pp. 490–500. (Blackwell Publishing: Oxford, UK)
Ryan SG, Smith BK, Collins RO, Turchini GM (2007) Evaluation of weaning strategies for intensively reared Australian freshwater fish, Murray cod, Maccullochella peelii peelii. Journal of the World Aquaculture Society 38, 527-535.
| Crossref | Google Scholar |
Schlechte JW, Buckmeier DL (2006) A pond evaluation of habituation as a means to reduce initial mortality associated with poststocking predation of hatchery-reared largemouth bass. North American Journal of Fisheries Management 26, 119-123.
| Crossref | Google Scholar |
Schlechte JW, Betsill RK, Buckmeier DL (2005) A laboratory evaluation of poststocking predatory losses for cultured largemouth bass. Transactions of the American Fisheries Society 134, 141-148.
| Crossref | Google Scholar |
Sparrevohn CR, Støttrup JG (2007) Post-release survival and feeding in reared turbot. Journal of Sea Research 57, 151-161.
| Crossref | Google Scholar |
Stickney RR (1994) Use of hatchery fish in enhancement programs. Fisheries 19(5), 6-13.
| Crossref | Google Scholar |
Stunz GW, Minello TJ (2001) Habitat-related predation on juvenile wild-caught and hatchery-reared red drum Sciaenops ocellatus (Linnaeus). Journal of Experimental Marine Biology and Ecology 260, 13-25.
| Crossref | Google Scholar |
Suboski MD, Templeton JJ (1989) Life skills training for hatchery fish: social learning and survival. Fisheries Research 7, 343-352.
| Crossref | Google Scholar |
Svåsand T, Kristiansen TS, Pedersen T, Salvanes AGV, Engelsen R, Nævdal G, Nødtvedt M (2000) The enhancement of cod stocks. Fish and Fisheries 1, 173-205.
| Crossref | Google Scholar |
Tang L, Jacquin L, Lek S, Liu H, Li Z, Liu J, Zhang T (2017) Differences in anti-predator behavior and survival rate between hatchery-reared and wild grass carp (Ctenopharyngodon idellus). Annales de Limnologie-International Journal of Limnology 53, 361-367.
| Crossref | Google Scholar |
Vejřík L, Matĕjíčková I, Sed’a J, Blabolil P, Jůza T, Vašek M, Ricard D, Matĕna J, Frouzová J, Kubečka J, Říha M, Čech M (2016) Who is who: an anomalous predator-prey role exchange between cyprinids and perch. PLoS ONE 11(6), e0156430.
| Crossref | Google Scholar |
Victorian Fisheries Authority (2023) Fish stocking database. Available at https://vfa.vic.gov.au/recreational-fishing/fish-stocking/db [Accessed 23 January 2023]
Walsh ML, Masuda R, Yamashita Y (2014) The influence of cage conditioning on the performance and behavior of Japanese flounder reared for stock enhancement: burying, feeding, and threat response. Journal of Sea Research 85, 447-455.
| Crossref | Google Scholar |