Golden perch (Macquaria ambigua) in refuge waterholes in ephemeral rivers: the effect of town proximity on biomass and size structure
David Nixon
A Department of Agriculture and Fisheries, Bribie Island Research Centre, 144 North Street, Woorim, Qld 4507, Australia.
Marine and Freshwater Research 73(12) 1426-1438 https://doi.org/10.1071/MF22074
Submitted: 31 March 2022 Accepted: 31 August 2022 Published: 10 October 2022
© 2022 The Author(s) (or their employer(s)). Published by CSIRO Publishing. This is an open access article distributed under the Creative Commons Attribution 4.0 International License (CC BY)
Abstract
Context: Intermittent rivers make up more than 50% of the world’s rivers. Refuge waterholes in these systems are critical for survival of fish populations.
Aim: This study investigated the effect that angling pressure imparts on native fish within refuge waterholes.
Methods: Golden perch (Macquaria ambigua) size structure was analysed in eight refuge waterholes across three catchments of the northern Murray–Darling Basin. Waterhole characteristics were recorded, with town proximity or remoteness being adopted as a surrogate variable for angling pressure.
Key results: Remoteness was found to be significantly correlated with median length, biomass, and proportion of legal-sized fish, but not with fish density. Sampling occurred after widespread connective flows, and the strength of remoteness as a predictor of median length suggests that redistribution of legal-sized golden perch on these flows was minimal. Legal-sized fish were poorly represented in the length–frequency distributions of near-town waterholes, and smaller size classes were well represented in most waterholes.
Conclusion: This study suggests that populations of golden perch in refuge waterholes near towns face significant angling pressure, with impacts on size structure at a local level.
Implications: These findings may have wider implications for the long-term resilience of the species.
Keywords: angling pressure, biomass, density, drought refuge waterhole, golden perch, intermittent river, Macquaria ambigua, mark–recapture, Murray–Darling Basin.
Introduction
Intermittent rivers constitute more than half of the global river network (Datry et al. 2014). During lengthy dry periods, the only refugia for fish in intermittent rivers are permanent river pools or waterholes (Arthington et al. 2005; Bogan et al. 2019). The importance of river pool or waterhole refugia for fish during periods of drought has been recognised across the world, including Africa (Strauch et al. 2015), North America (Van Horn et al. 2022), Europe (Pires et al. 2004) and Australia (Marshall et al. 2021). Despite the prevalence of intermittent rivers worldwide and the importance of refugia waterholes, there has been only limited work conducted on the effects of angling pressure on such systems (e.g. Close et al. 2014).
The Murray–Darling Basin (MDB) is a large dryland river system, covering >1 × 106 km2 of south-eastern Australia (Crabb 1997). Rainfall varies widely across the MDB, with hydrology in the south driven by predictable winter and spring rainfall, whereas northern hydrology is influenced by unpredictable, semi-monsoonal summer rainfall (Bice et al. 2021). The Darling River in the northern MDB is ranked as one of the most hydrologically variable flow regimes in the world (Puckridge et al. 1998). Connective flows are often short-lived in these northern ephemeral river systems, with permanent waterholes providing crucial drought refugia for most fish species (Perry and Bond 2009). For the purpose of this study, we will refer to these isolated, remnant pools as refuge waterholes.
Golden perch (Macquaria ambigua (Richardson)) is one of the most common and widespread large native percichthyid fish within the MDB (Bond et al. 2015). It can grow to a maximum size of 23 kg, although most fish are less than 5 kg (Cadwallader and Backhouse 1983). Angling is a regular pastime in the regional communities of the MDB, with golden perch forming the basis for a popular recreational fishery (Lintermans 2007). Angled fish are commonly retained for consumption, with a current minimum legal-size of 300 mm in all states except South Australia (330 mm). Targeted fish species in ephemeral rivers are susceptible to the effects of overfishing (Close et al. 2014), therefore it is predicted that angling pressure will alter golden perch size structure in refuge waterholes, where habitat and resources are limited.
In the ephemeral catchments of the northern MDB, suitable fish habitat contracts significantly during periods of no flow, and this is further exacerbated by prolonged droughts. Marshall et al. (2021) reported that refuge waterholes in the northern MDB were small and represent as little as 11% of the total river channel network during extreme drought. However, small populations of maturing and adult fish can act as significant sources for juvenile recruitment during flow periods, when refuge waterholes are reconnected (Balcombe et al. 2006). Sustained angling harvest has the potential to significantly affect golden perch biomass and size structure in these refuge waterholes, and these effects may persist in the long term, despite opportunities for redistribution during connective flows. Golden perch movement patterns are highly variable within populations (Crook 2004; O’Connor et al. 2005; Zampatti et al. 2018), although site fidelity is a common trait. One movement study in the northern MDB demonstrated that 72% of detected individuals had linear ranges less than 50 km (Bice et al. 2021). Movement opportunities are also hampered by instream barriers (Marshall et al. 2021), which may contribute to long-term disparities in size structures among waterholes.
This paper investigates differences between the biomass of golden perch at seven waterholes and the size structure of golden perch in eight refuge waterholes of varying proximity to towns. Recent research has demonstrated that spawning events in the northern MDB contribute significantly to golden perch recruitment downstream in the lower Darling River and Murray River (Stuart and Sharpe 2020). Therefore, the status of golden perch populations in these refuge waterholes may have broader implications for recruitment across the MDB.
Materials and methods
Site selection
The study area encompassed three catchments of the northern MDB within the state of Queensland: the Warrego, Condamine–Balonne and Moonie catchments (Fig. 1). Two waterholes were sampled within each catchment, including one small waterhole (<800 m long), and one large waterhole (>1000 m long). An additional large waterhole was sampled within the Condamine–Balonne catchment at Paradise Downs on the Dogwood Creek. Only waterholes with clearly defined boundaries and good boat access were chosen for sampling.
Sampling commenced in October 2017 at Paradise Downs Waterhole in the Condamine catchment, prior to summer connective flows. Undulla Creek Waterhole was sampled next in November 2017, after a small connective flow. Widespread summer rainfall and multiple connective events in all catchments prevented further sampling for 6 months. The timing and magnitude of flow events in relation to sampling is presented in Fig. 2. Sampling at all other sites (except Saint George) was completed between 22 May and 30 June 2018. At each site, waterhole surface area was calculated by measuring the width at 50-m intervals along the entire length of the waterhole with range-finding binoculars (Bushnell Yardage Pro 400, Overland Park, KS, USA). Each width measurement was multiplied by 50 to obtain the surface area of the preceding 50-m section and summed to obtain the overall surface area. The deepest point at each 50-m interval was also recorded, and the values were averaged to obtain mean thalweg depth for all waterholes.
The distance of each waterhole from the nearest town was calculated using the Google Earth Pro Pathway function (ver. 7.3, see https://earth.google.com/web), where the road distance (km) was calculated on the basis of the most efficient route to the town post office. Towns with fewer than 400 residents were not considered; for example, a village of nine people was closer to both Warrie sites than the nearest town with 400 people or more. This village was assumed to make a negligible contribution to angling pressure and was therefore not used in this study. The nearest town was unequivocal at all other waterholes. All sites were accessible by two-wheel-drive vehicle during dry weather, and it was assumed that travel cost and convenience would be considerations that could influence the effect of distance on fishing pressure.
Remoteness or distance to settlements has been used as a surrogate for fishing pressure in an ephemeral river by Close et al. (2014), and several studies of marine fisheries have also found distance from population centres to be negatively correlated with fishing pressure (e.g. Advani et al. 2015; Campbell et al. 2019).
Sampling methods
Sampling occurred when waterhole connectivity was negligible or absent, ensuring that each waterhole was effectively a closed system. Long-term weather forecasts were consulted prior to sampling to minimise the risk of connective flows occurring between the tag and recapture phases. Waterholes were sampled using either a 5.0- or 7.5-kVa vessel-mounted electrofisher (Smith-Root, Portland, WA, USA), depending on waterhole size. Conductivity averaged 120 μS across all waterholes, with electrofisher output generally set at 500 V, 120 pulses per second and 90% gain. One dip-netter operated from the bow on small waterholes, and two dip-netters operated on large waterholes. Where extensive shallow margins were present at the upstream or downstream ends of a waterhole, blocker nets were deployed prior to sampling at the limit of navigable water to ensure that fish could not evade the electrofishing equipment.
Initial electrofishing occurred over a minimum of two consecutive days, with effort maximised by targeting the complex habitats preferred by golden perch (e.g. woody debris, vegetated margins and rocky reefs). All likely habitats were sampled thoroughly and repeatedly to ensure comprehensive coverage and a large sample size. All golden perch individuals captured during the initial surveys were sedated in a 20 µg g−1 solution of AQUI-S (AQUI-S New Zealand Ltd, Lower Hutt, New Zealand). The weight of each fish was recorded to the nearest gram, and total length (TL) was measured to the nearest millimetre. Fish were then tagged and placed in an aerated live well for full recovery prior to release. Fish longer than 150 mm were tagged with a numbered dart tag inserted into musculature adjacent to the dorsal fin. A mean of 80 fish per waterhole also had a passive integrated transponder (PIT) tag planted in the intraperitoneal cavity to calculate tag loss rates. Fish smaller than 150 mm were deemed too small for dart tags and were instead marked with a small hole in their pelvic fin. Tag numbers, length and date of capture were recorded for all fish. Sampled fish were released beyond the blocker net to avoid early recaptures and to prevent further exposure to electrofishing equipment.
Sample size was increased using fyke nets, where the terrain was suitable, with both small (2-mm mesh, twin 5-m wings and 0.6-m hoops) and large (12-mm mesh, twin 8-m wings and 1.0-m hoops) nets being used. Fyke nets were set overnight, parallel to the bank in back-to-back pairs, with any captured individuals being weighed, measured and tagged as above. A 2-day minimum rest period occurred on the cessation of initial tagging, with the blocker net removed to enable golden perch to adequately recover and mix within the population.
The recapture phase involved intensive electrofishing effort over a 2-day period. All untagged golden perch individuals were weighed and measured as above. The details of recaptured fish were recorded, including tag number and date of recapture. The blocker net was reinstated during this phase, and all captured fish were released beyond this net as above. Efforts were made to capture as many fish as possible during this phase to improve the rigour of size structure and biomass estimates.
This work was conducted in conjunction with fieldwork on FRDC project number 2016-153, with animal ethics approval number CA 2017/09/1106. Additional work at Saint George was completed under MDBA project number MD005162 and animal ethics approval number CA 2020/05/1372. All work was completed under General Fisheries Permit number 186281.
Supplementary sampling at Saint George waterhole
An additional waterhole on the Balonne River was sampled in July 2020. A comprehensive golden perch population survey was undertaken at this site, only 1.3 km from the township of Saint George. This study did not use tag–recapture methods and therefore did not collect population characteristics such as density and biomass. However, the data for the Saint George population survey was collected using a similar intensive application of electrofishing effort, with proven sampling methodology (Hutchison et al. 2020) and a large sample size (n = 373). These data were used for comparative analyses of length frequencies from this location, with length frequencies from the other waterholes being sampled.
Sampling at the Saint George site was sufficiently intense to ensure good representation of the length frequency of the golden perch population. Total effort was similar to that for the other waterholes sampled. The waterhole was divided into four zones, with eight electrofishing shots undertaken in each zone, for a total of 32 shots. A 7.5-kVa vessel-mounted electrofisher (Smith-Root, Portland, WA, USA) was used for this site, with two dip netters operating from the bow. Each shot consisted of 600 s of power-on time, and involved 20 × 24 s of in and out movements and 120 s of movement parallel to the bank (Fig. 3). Each shot targeted representative habitats along a 100-m stretch of riverbank, and a minimum of 50 m was left between each shot to ensure independence. Six small-mesh fyke nets (as per tag–recapture surveys) were set over three nights, in the same manner as previously described, to sample as many golden perch fish as possible. Sampled golden perch fish were initially held in an aerated live well, before being sedated as above and measured to the nearest millimetre (TL). A small hole was placed in the pelvic fin prior to release to identify that the fish had been caught and to prevent duplicate recordings in the dataset.
![]() |
Population density and biomass estimation
The tagged recaptures in each waterhole were used to estimate the total population (N) at that site, by using an unbiased ‘Lincoln–Petersen method’ (Williams et al. 2002), as follows:
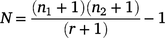
where n1 is the initial number of tagged fish, n2 is the total number of fish caught during recapture phase, and r is the number of tagged recaptures.
The population estimate N was multiplied by the mean weight of all fish sampled at a site (g) to obtain an overall biomass estimate (kg). This was divided by the surface area of the waterhole to obtain a biomass estimate (kg ha−1). Similarly, the population estimate n was divided by the area of the waterhole (ha) to obtain a density estimate (fish ha−1).
Data analysis
The relationships between waterhole characteristics and golden perch population variables were explored through linear regression analysis (Genstat, ver. 18.2), using seven key variables (Table 1). The median length was analysed in preference to the mean, owing to the non-parametric nature of golden perch length–frequencies in this study. Data on golden perch density and biomass were not available for the Saint George waterhole, so median length and percentage of legal-sized fish were the only dependant variables analysed at this site. Relationships among independent variables were also investigated to identify confounding factors.
![]() |
Pairwise comparisons of golden perch length–frequency distributions were made using the non-parametric Kolmogorov–Smirnov statistic (Genstat, ver. 18.2, see https://vsni.co.uk/software/genstat).
Results
Population structure and pairwise comparisons
There was a high level of variability in both the number of fish tagged (min = 90 at Undulla waterhole, max = 266 at Warrie 1, mean = 181), and the number of tagged recaptures (min = 6 at Paradise Downs, max = 47 at Warrie 1, mean = 15.85). The golden perch length–frequencies also showed a high level of variability among waterholes (Fig. 4). Near-town waterholes were heavily represented in the juvenile size classes, with poor representation in size classes above the legal-take limit of 300 mm (Fig. 4e–h). This was especially evident when comparing near-town waterholes with the two remote Warrie sites (Fig. 4a,b), particularly Warrie 2, where fish above 300 mm were abundant and the size structure approached a bell-shaped curve.
![]() |
The disparities between golden perch length–frequencies were confirmed by Kolmorogov–Smirnov pairwise comparisons (Table 2), demonstrating that the length–frequency distributions were significantly different among all waterholes except for Undulla and Warrie 2.
![]() |
Relationships between remoteness and golden perch size, biomass and density
Remoteness was found to be a strong positive predictor of golden perch median length, and a positive predictor of the proportion of legal-sized fish in refuge waterholes (Fig. 5), with both relationships being significant (P < 0.01). The significance values for all relationships are shown in Table 3. Waterhole surface area and mean thalweg depth were not significantly correlated with any other variable, including with remoteness, so these variables were not considered to be confounding factors.
![]() |
There was a significant positive correlation between remoteness and golden perch biomass, but remoteness was a poor predictor of density (P = 0.209). However, the relationship between the density and biomass of golden perch in refuge waterholes was significant (Table 3).
Discussion
Our study demonstrated the effect of the proximity to population centres (angling pressure) on golden perch populations in refuge waterholes of the northern MDB. Although this study did not measure angling activity directly, remoteness has previously been demonstrated as a useful proxy for fishing effort (Close et al. 2014; Advani et al. 2015; Campbell et al. 2019), and angling pressure remains the most logical explanation for the well defined gradient in size and biomass observed in refuge waterholes. These findings are perhaps unsurprising, given the popularity of golden perch among inland angling communities and the paucity of alternate target species in these catchments. However, the strength of waterhole remoteness as a tool for predicting golden perch subpopulation characteristics is noteworthy, with potential wider application for future studies. Similarly, the findings of subpopulation-level effects in refuge waterholes may have broader implications for the resilience and management of golden perch into the future.
The effect of angling pressure on marine finfish populations has been well documented in previous studies, especially for keenly targeted species such as coral trout, Plectropomus leopardus (Mapstone et al. 2004; Russ et al. 2008; Miller et al. 2012). However, studies on the impacts of angling pressure on Australian freshwater fish are uncommon. The majority of MDB studies focus on Murray cod, Maccullochella peeli (Allen et al. 2009; Brown 2010; Douglas et al. 2010), with there being few studies on golden perch (Forbes et al. 2015a). There have been no apparent studies on the impact of recreational fishing within the ephemeral rivers of the northern MDB and very few in ephemeral river systems in other parts of Australia (e.g. Close et al. 2014) or the world. There has been at least one study of the effects of small subsistence and commercial fisheries in the Okavango Delta (Mmopelwa et al. 2009), where it was found that fishing pressure reduced fish biomass proportionally across different trophic levels, but did not reduce diversity.
When compared with the more-connected waters of the southern MDB, golden perch within the northern MDB refuge waterholes is even more susceptible to localised overfishing, given that migration here is both spatially and temporally limited.
The majority of waterholes sampled during this study were small (<4 ha). This is consistent with Marshall et al. (2021), who found that isolated waterholes within the ephemeral rivers of the northern MDB are often small and few in number, comprising as little as 11% of the total river-channel network during severe drought. However, refuge waterholes play a central role in structuring aquatic communities (Magoulick and Kobza 2003), and small populations of maturing and adult fish are regarded as significant sources for juvenile recruitment when flows return and refuge waterholes become connected again (Balcombe et al. 2006). A growing body of evidence suggests that spawning activity in the northern MDB provides a valuable contribution to system-wide golden perch recruitment during large-scale flow events, with eggs and larvae drifting far beyond the catchment of origin. Stuart and Sharpe (2020) found that golden perch recruits in the lower Darling and lower Murray were originally spawned hundreds or even thousands of kilometres away in the Macintyre or Weir Rivers, which are neighbouring catchments to the Moonie. Similarly, the Warrego River has recently been shown to be a fecund nursery habitat, generating valuable recruitment downstream in the Darling River (Warrego–Darling Monitoring Evaluation Research 2020). Therefore, populations of mature golden perch in refuge waterholes of the northern MDB are important for the resilience of this species into the future.
The length–frequency distributions of this study highlight the selective pressure applied to populations in refuge waterholes, with representation declining sharply in sizes above 300 mm, particularly in near-town waterholes. Although these findings may suggest a level of adherence to legal-size limits, further research would be needed to clarify this. Conversely, these findings could be viewed with some concern, as it is possible that mature golden perch are being extracted soon after attaining legal-size. Forbes et al. (2015b) found that the current legal-size limit offered little protection for the spawning population, with less than 16% of the population mature at 300 mm in two catchments of the southern MDB, and the length at which 50% of the fish are mature (LM50) being in the range 350–360 mm. If the LM50 is similar in the northern MDB ephemeral river systems, then increasing the minimum legal length may assist in ensuring that golden perch fish in refuge waterholes are able to spawn at least once. Female golden perch individuals are older and larger than males at maturity, potentially biasing the recreational harvest towards female fish (Mallen-Cooper and Stuart 2003). If a large proportion of adult females within near-town refuge waterholes is being extracted before they have a chance to spawn, this has the potential to reduce the long-term resilience of the species, with flow-on effects to both migration and recruitment.
The truncated size structure at Saint George waterhole is especially pronounced, with very few fish over 225 mm present. This waterhole is immediately below the Jack Taylor weir, an impassable barrier to upstream migration of fish on all but the largest of floods. Therefore, it might be expected that upstream migrating fish would accumulate here, especially considering that long-distance movement of adult golden perch in the northern MDB occurs predominantly in an upstream direction (Bice et al. 2021). As the flow dropped, it would be expected that golden perch might move back downstream into the Saint George waterhole or to other waterholes further downstream. The Saint George waterhole also contains abundant natural habitat, such as complex woody debris and rocky structure; so, it would be expected that larger fish would be retained here in good numbers. However, this study found very few legal-sized fish in this waterhole at the time of sampling. There may be other factors that explain the poor catch of legal-sized fish here, but this waterhole has public boat access, and displays ample evidence of fishing activity, so the assumption of significant angling effort here is not unreasonable.
Previous studies in other catchments have shown that golden perch size structure is generally characterised by dominant year-classes, when conditions have been favourable for widespread recruitment, along with poor or ‘missing’ year-classes, where recruitment has been minimal or unsuccessful (Mallen-Cooper and Stuart 2003; Roberts et al. 2008; Zampatti and Leigh 2013). The present study looked at length–frequency as opposed to age–frequency, and therefore is not directly comparable to such studies. Length of golden perch has been found to be an unreliable indicator of age (Anderson et al. 1992), because growth rates vary substantially among catchments (Roberts et al. 2008), and there is a higher growth variability in the northern catchments of the MDB than in the southern catchments (Wright et al. 2020). This study has drawn data from several catchments of the northern MDB and, although the likelihood exists that the dominant length–frequency classes in this study correlate with dominant year cohorts, this conclusion cannot be validated without ageing the fish.
In a study on golden perch (M. ambigua oriens) in Queensland’s Fitzroy Basin, Roberts et al. (2008) found that recruitment occurred most consistently in unregulated rivers, whereas regulated rivers were more likely to have erratic or failed recruitment events. The current study did not draw on sufficient sample size to lend any support to those findings. Further research is warranted to investigate the relationship between river regulation and golden perch recruitment across the northern MDB, with stocked fingerlings posing an additional factor that requires consideration.
Six of the eight waterholes used in this study were sampled shortly after the cessation of multiple connective flow events, which would have provided ample opportunity for migration and mixing of golden perch populations. Even if the flows did not drown out all weirs, all sampled sites would have had links to waterholes for tens of kilometres or in some cases more than 100 km in at least one direction on the flow events. Therefore, an increased level of population homogeneity might be anticipated, with legal-sized golden perch relocating into heavily fished waterholes where vacant habitat exists and competition for food is lower. However, the significant disparities between golden perch population structure in different waterholes suggests that this did not happen on an appreciable scale in the connective flows preceding this study. There may be temporal or seasonal factors to consider here, although it should be noted that connective flows prior to sampling occurred from February to April. Connective flows during these months have previously been shown to result in golden perch movement. Bice et al. (2021) reported that all large movements in the northern MDB occurred during elevated discharge from February to April, whereas movement in the southern MDB is more common from September to November (O’Connor et al. 2005; Koehn and Nicol 2016). The current study presents a snapshot of golden perch populations at a single juncture, rather than investigating long-term movement behaviour. Although it is likely that golden perch movement and immigration would have occurred in the connective flows preceding this study, this appears not to have occurred at a scale sufficient to overcome the pronounced effects of remoteness on golden perch population characteristics, such as median length and percentage of legal-sized fish. Additional research that investigates long-term golden perch movement behaviour alongside size structure in waterholes of varying remoteness would strengthen predictive population models and would likely lead to improved management options for the species.
Studies on the movement and migration habits of golden perch in the southern MDB have demonstrated a high level of within-population variability of movement behaviours, such as site fidelity, short- and long-distance migration, and homing behaviour (Crook 2004; O’Connor et al. 2005; Zampatti et al. 2018). However, comparison with these studies is difficult given the high spatio-temporal variability in golden perch behaviour (Zampatti et al. 2018), especially when considering that fragmentation of river systems in the northern MDB results in enforced site fidelity for most of the year, with fish movement here being opportunistic in response to flows (Nicholls et al. 2012).
Philopatry has been shown to be a common trait for golden perch in the Moonie River, with individual fish moving during connective flow events only to return to their original waterhole as a flow begins to recede (Marshall et al. 2016). There is strong selective pressure against fish moving at random out of refugia (Whitney et al. 2016), with a high level of philopatry allowing golden perch individuals to avoid the high costs of settling and perishing in a doomed habitat patch (Marshall et al. 2016). Further, the existence of site fidelity in riverine fish species has previously been shown to result in the failure of predictions that individuals will utilise high-quality habitat patches that are located outside the area to which fidelity is exhibited (Railsback et al. 1999). This may help explain the findings of the present study, where legal-sized golden perch were scarce in habitat-rich, near-town waterholes. If legal-sized golden perch residing in heavily fished waterholes exhibit strong site fidelity or philopatry, then these fish will continually be exposed to angler effort, resulting in the gradual localised depletion of large fish over time. Conversely, remote waterholes are more likely to retain their legal-sized fish, with individuals unlikely to permanently relocate elsewhere while also being at reduced risk of extraction by anglers. In this way, the impacts of angling pressure on golden perch populations in near-town refuge waterholes are likely to accumulate over time, despite the repeat occurrence of connective flows.
Immature fish (more than 1 year-old) have previously been shown to comprise the numerically dominant portion of migrating golden perch, moving upstream to compensate for downstream egg and larval drift (Mallen-Cooper 2000). These fish move in stages during connective flows and appear not to move back downstream when flows cease. The exact cues that prompt individual fish to settle in particular waterholes remain unclear, although the present study found that golden perch density did not differ significantly with remoteness, which may suggest a level of uniformity in the colonisation process. However, remoteness did correlate significantly with biomass, with the reduced biomass in near-town refuge waterholes being likely attributable to angler harvest of legal-sized fish and possibly some fish being just under legal size. A movement study in the northern MDB demonstrated that site fidelity was common, with 72% of detected golden perch moving less than 50 km (Bice et al. 2021). Although the migration behaviour of golden perch is well documented (Crook 2004; O’Connor et al. 2005; Zampatti et al. 2018), it is possible that the immigration of adult fish plays a secondary role in bolstering stocks of large golden perch in heavily fished refuge waterholes. Instead, recruitment to the fishery may primarily rely on a much slower process, namely, immature fish colonising waterholes, establishing site fidelity, and subsequently growing out to legal-size.
Angling pressure in many refuge waterholes of the northern MDB is presently limited by remoteness and private land tenure, a situation that is likely to afford some protection to golden perch populations. However, anglers may travel further afield if the stocks of legal-sized fish in their local waterholes become depleted, and this may result in the gradual geographic spread of angling pressure over time. Close et al. (2014) found that angler visitation rates were steadily increasing in the Fitzroy River of Western Australia, with 62% of the catchment already subject to moderate–high levels of fishing pressure. Research by the Department of Environment and Science (2020) has shown that recreational fishing is increasingly popular in Queensland, with an additional 300 000 participants in 2019 when compared with 2013. Resilience in native fish metapopulations in the northern MDB is already severely compromised by the increasing length of drought periods, and by the impacts of numerous instream barriers that limit opportunities for fish movement (Marshall et al. 2021). Angling pressure presents an additional threat to golden perch resilience, as removal of the larger, more-fecund fish from refuge waterholes may reduce the scale of recruitment and dispersal when connective flows occur. Angler education may be one tool worth considering, to promote voluntary catch and release during prolonged droughts. If angling pressure were to increase on a broad scale within the northern MDB, then stronger avenues of protection may need to be considered. These measures may include the adoption of freshwater protected areas similar to those proposed by Close et al. (2014) for the Fitzroy Basin in Western Australia.
Although this study also investigated the effect of waterhole size and depth, these variables were found to have no significant effect on density, biomass, median length, or proportion of legal-sized fish. This aspect of our study was limited by a small sample size (n = 7) and sampling additional waterholes would be beneficial to investigate these relationships in more detail. It should also be noted that angling pressure is likely to be proportional to town population size, and this should be included as a covariate in future studies.
It is likely that angling pressure (or artisanal fisheries) could have impacts on recreational species in refuge pools and waterholes in ephemeral systems in other parts of the world. Although there have been very few studies examining this issue, the presence of recreational fishing species in refugia have been documented. Some examples include largemouth bass, Micropterus salmoides (Dubose et al. 2020), tilapias (Cichlidae; Strauch et al. 2015) and sooty grunter, Hephaestus fuliginosus (Pusey et al. 2018). Understanding global trends on the impacts of fishing pressure on waterhole refugia in ephemeral rivers and how such fisheries can best be managed is an area of research worthy of further investigation.
Additional research over a longer time frame would be beneficial in validating the link between angling and golden perch population dynamics in the current study. It is likely that the cumulative effect of angling pressure presents an additional disturbance for golden perch populations in refuge waterholes, raising concerns for the ongoing resilience of the species if this were to substantially increase. These findings have relevance to the northern catchments of the MDB, and broader implications for golden perch recruitment in the Murray–Darling Basin as a whole. There is potential that other species across the world targeted by anglers in waterhole refugia during droughts could be similarly affected.
Data availability
Data can be made available on reasonable request to the corresponding author.
Conflicts of interest
The authors declare that they have no conflicts of interest.
Declaration of funding
Data collection for most of this work was funded by the Fisheries Research and Development Corporation (FRDC) project number 2016-153. Additional data collection at Saint George was funded by the Murray–Darling Basin Authority (MDBA) project number MD05162.
Acknowledgements
Thanks go to Rod Cheetham and Keith Chilcott for their assistance in sampling. Jenny Shiau also assisted with sampling at the Saint George Waterhole.
References
Advani, S, Rix, LN, Aherne, DM, Alwany, MA, and Bailey, DM (2015). Distance from a fishing community explains fish abundance in a no-take-zone with weak compliance. PLoS ONE 10, e0126098.| Distance from a fishing community explains fish abundance in a no-take-zone with weak compliance.Crossref | GoogleScholarGoogle Scholar |
Allen, MS, Brown, P, Douglas, J, Fulton, W, and Catalano, M (2009). An assessment of recreational fisheries harvest policies for Murray cod in south-east Australia. Fisheries Research 95, 260–267.
| An assessment of recreational fisheries harvest policies for Murray cod in south-east Australia.Crossref | GoogleScholarGoogle Scholar |
Anderson, JR, Morison, AK, and Ray, DJ (1992). Validation of the use of thin-sectioned otoliths for determining the age and growth of golden perch, Macquaria ambigua (Perciformes: Percithyidae), in the lower Murray–Darling Basin, Australia. Australian Journal of Marine and Freshwater Research 43, 1103–1128.
| Validation of the use of thin-sectioned otoliths for determining the age and growth of golden perch, Macquaria ambigua (Perciformes: Percithyidae), in the lower Murray–Darling Basin, Australia.Crossref | GoogleScholarGoogle Scholar |
Arthington, AH, Balcombe, SR, Wilson, GA, Thoms, MC, and Marshall, J (2005). Spatial and temporal variation in fish assemblage structure in isolated waterholes during the 2001 dry season of an arid-zone floodplain river, Cooper Creek, Australia. Marine and Freshwater Research 56, 25–35.
| Spatial and temporal variation in fish assemblage structure in isolated waterholes during the 2001 dry season of an arid-zone floodplain river, Cooper Creek, Australia.Crossref | GoogleScholarGoogle Scholar |
Balcombe, SR, Arthrington, AH, Foster, ND, Thoms, MC, Wilson, GG, and Bunn, SE (2006). Fish assemblages of an Australian dryland river: abundance, assemblage structure and recruitment patterns in the Warrego River, Murray–Darling Basin. Marine and Freshwater Research 57, 619–633.
| Fish assemblages of an Australian dryland river: abundance, assemblage structure and recruitment patterns in the Warrego River, Murray–Darling Basin.Crossref | GoogleScholarGoogle Scholar |
Bice CM, Butler GL, Fanson B, Harding D, Hodges K, Koster W, Thiem JD, Woods R, Zampatti BP (2021) Hydrology and movement of adult golden perch (Macquaria ambigua) in the southern and northern Murray–Darling Basin determined by acoustic telemetry. SARDI Publication Number F2021/000076-1, SARDI Research Report Series Number 1104, South Australian Research and Development Institute (Aquatic Sciences), Adelaide, SA, Australia.
Bogan, MT, Leidy, RA, Neuhaus, L, Hernandez, CJ, and Carlson, SM (2019). Biodiversity value of remnant pools in an intermittent stream during the great California drought. Aquatic Conservation: Marine and Freshwater Ecosystems 29, 976–989.
| Biodiversity value of remnant pools in an intermittent stream during the great California drought.Crossref | GoogleScholarGoogle Scholar |
Bond, NR, Balcombe, SR, Crook, DA, Marshall, JC, Menke, N, and Lobegeiger, JS (2015). Fish population persistence in hydrologically variable landscapes. Ecological Applications 25, 901–913.
| Fish population persistence in hydrologically variable landscapes.Crossref | GoogleScholarGoogle Scholar |
Brown P (2010) Sustainability of recreational fisheries for Murray Cod: creel surveys on the Goulburn, Ovens and Murray Rivers 2006–2008. Fisheries Victoria, Fisheries Research Branch, Alexandra, Vic., Australia.
Cadwallader PL, Backhouse GN (1983) ‘A guide to the freshwater fish of Victoria.’ (Victorian Government Printer: Melbourne, Vic., Australia)
Campbell, SJ, Darling, ES, Pardede, S, Ahmadia, G, Mangubhai, S, Amkieltiala Estradivari Maire, E (2019). Fishing restrictions and remoteness deliver conservation outcomes for Indonesia’s coral reef fisheries. Conservation Letters 13, e12698.
| Fishing restrictions and remoteness deliver conservation outcomes for Indonesia’s coral reef fisheries.Crossref | GoogleScholarGoogle Scholar |
Close, PG, Dobbs, RJ, Tunbridge, DJ, Speldewinde, PC, Warfe, DW, Toussaint, S, and Davies, PM (2014). Customary and recreational fishing pressure: large-bodied fish assemblages in a tropical, intermittent Australian river. Marine and Freshwater Research 65, 466–474.
| Customary and recreational fishing pressure: large-bodied fish assemblages in a tropical, intermittent Australian river.Crossref | GoogleScholarGoogle Scholar |
Crabb P (1997) Impacts of anthropogenic activities, water use and consumption on water resources and flooding. State of the Environment Technical Paper Series (Inland Waters), Department of the Environment, Canberra, ACT, Australia.
Crook, DA (2004). Is the home range concept compatible with the movements of two species of lowland river fish? Journal of Animal Ecology 73, 353–366.
| Is the home range concept compatible with the movements of two species of lowland river fish?Crossref | GoogleScholarGoogle Scholar |
Datry, T, Larned, ST, and Tockner, K (2014). Intermittent rivers a challenge for freshwater ecology. BioScience 64, 229–235.
| Intermittent rivers a challenge for freshwater ecology.Crossref | GoogleScholarGoogle Scholar |
Department of Environment and Science (2020) Recreational fishing catch and participation. State of the Environment Report. (Department of Environment and Science) Available at https://www.stateoftheenvironment.des.qld.gov.au/biodiversity/estuarine-and-marine-ecosystems/recreational-fishing-catch-and-participation
Douglas, J, Brown, J, Hunt, T, Rogers, M, and Allen, M (2010). Evaluating relative impacts of recreational fishing harvest and discard mortality on Murray cod (Maccullochella peelii peelii). Fisheries Research 106, 18–21.
| Evaluating relative impacts of recreational fishing harvest and discard mortality on Murray cod (Maccullochella peelii peelii).Crossref | GoogleScholarGoogle Scholar |
DuBose, TP, Ashford, K, and Vaughn, CC (2020). Freshwater mussels increase survival of largemouth bass (Micropterus salmoides) in drying pools. Ecology of Freshwater Fish 29, 220–229.
| Freshwater mussels increase survival of largemouth bass (Micropterus salmoides) in drying pools.Crossref | GoogleScholarGoogle Scholar |
Forbes, JP, Watts, RK, Robinson, WA, Baumgartner, LJ, Steffe, AS, and Murphy, JJ (2015a). Recreational fishing effort, catch, and harvest for Murray cod and golden perch in the Murrumbidgee River, Australia. North American Journal of Fisheries Management 35, 649–658.
| Recreational fishing effort, catch, and harvest for Murray cod and golden perch in the Murrumbidgee River, Australia.Crossref | GoogleScholarGoogle Scholar |
Forbes, JP, Watts, RK, Robinson, WA, Baumgartner, LJ, Allen, MS, McGuffie, P, Cameron, LM, and Crook, DA (2015b). System specific variability in Murray cod and golden perch maturation and growth influences fisheries management options. North American Journal of Fisheries Management 35, 1226–1238.
| System specific variability in Murray cod and golden perch maturation and growth influences fisheries management options.Crossref | GoogleScholarGoogle Scholar |
Hutchison M, Nixon D, Shiau J, Norris A (2020) Golden perch population structure, Balonne River, St George. Queensland Government, Department of Agriculture and Fisheries, Bribie Island Research Centre, Bribie Island, Qld, Australia.
Koehn, JD, and Nicol, SJ (2016). Comparative movements of four large fish species in a lowland river. Journal of Fish Biology 88, 1350–1368.
| Comparative movements of four large fish species in a lowland river.Crossref | GoogleScholarGoogle Scholar |
Lintermans M (2007) ‘Fishes of the Murray–Darling Basin – an introductory guide.’ (Murray–Darling Basin Authority: Canberra, ACT, Australia)
Magoulick, DD, and Kobza, RM (2003). The role of refugia for fishes during drought: a review and synthesis. Freshwater Biology 48, 1186–1198.
| The role of refugia for fishes during drought: a review and synthesis.Crossref | GoogleScholarGoogle Scholar |
Mallen-Cooper M (2000) Taking the mystery out of migration. In ‘Proceedings of the 1999 Annual Workshop of the Australian Society for Fish Biology’, 28–29 Septermber 1999, Bendigo, Vic., Australia. (Eds DA Hancock, DC Smith, JD Koehn) pp. 101–111. (Australian Society for Fish Biology: Sydney, NSW, Australia)
Mallen-Cooper, M, and Stuart, IG (2003). Age, growth and non-flood recruitment of two potamodromous fishes in a large semi-arid/temperate river system. River Research and Applications 19, 697–719.
| Age, growth and non-flood recruitment of two potamodromous fishes in a large semi-arid/temperate river system.Crossref | GoogleScholarGoogle Scholar |
Mapstone BD, Davies CR, Little LR, Punt AE, Smith ADM, Pantus F, Lou DC, Williams AJ, Jones A, Ayling AM, Russ GR, McDonald AD (2004) The effects of line fishing on the Great Barrier Reef and evaluations of alternative potential management strategies. CRC Reef Research Centre Technical Report 52. CRC Reef Research Centre, Townsville, Qld, Australia.
Marshall, JC, Menke, N, Crook, DA, Lobegeiger, JS, Balcombe, SR, Huey, JA, Fawcett, JH, Bond, NR, Starkey, AH, Sternberg, D, Linke, S, and Arthrington, AH (2016). Go with the flow: the movement behaviour of fish from isolated waterhole refugia during connecting flow events in an intermittent dryland river. Freshwater Biology 61, 1242–1258.
| Go with the flow: the movement behaviour of fish from isolated waterhole refugia during connecting flow events in an intermittent dryland river.Crossref | GoogleScholarGoogle Scholar |
Marshall, JC, Lobegeiger, JS, and Starkey, A (2021). Risks to fish populations in dryland rivers from the combined threats of drought and instream barriers. Frontiers in Environmental Science 9, 671556.
| Risks to fish populations in dryland rivers from the combined threats of drought and instream barriers.Crossref | GoogleScholarGoogle Scholar |
Miller, I, Cheal, AJ, Emslie, MJ, Logan, M, and Sweatman, H (2012). Ongoing effects of no-take marine reserves on commercially exploited coral trout populations on the Great Barrier Reef. Marine Environmental Research 79, 167–170.
| Ongoing effects of no-take marine reserves on commercially exploited coral trout populations on the Great Barrier Reef.Crossref | GoogleScholarGoogle Scholar |
Mmopelwa, G, Mospele, K, Mosepele, B, Moleele, N, and Ngwenya, B (2009). Environmental variability and the fishery dynamics of the Okavango delta, Botswana: the case of subsistence fishing. African Journal of Ecology 47, 119–127.
| Environmental variability and the fishery dynamics of the Okavango delta, Botswana: the case of subsistence fishing.Crossref | GoogleScholarGoogle Scholar |
Nicholls S, Berghuis A, Lay C, Mallen-Cooper M (2012) Fishway options for weirs of the northern Murray Darling Basin. Report to the Murray Darling Basin Authority. Department of Primary Industries, New South Wales, Australia.
O’Connor, JP, O’Mahony, DJ, and O’Mahony, JM (2005). Movements of Macquaria ambigua, in the Murray River, south-eastern Australia. Journal of Fish Biology 66, 392–403.
| Movements of Macquaria ambigua, in the Murray River, south-eastern Australia.Crossref | GoogleScholarGoogle Scholar |
Perry, GLW, and Bond, NR (2009). Spatially explicit modelling of habitat dynamics and fish population persistence in an intermittent lowland stream. Ecological Applications 19, 731–746.
| Spatially explicit modelling of habitat dynamics and fish population persistence in an intermittent lowland stream.Crossref | GoogleScholarGoogle Scholar |
Pires, AM, Da Costa, LM, Alves, MJ, and Coelho, MM (2004). Fish assemblage structure across the Arade Basin (southern Portugal). Cybium 28, 357–365.
| Fish assemblage structure across the Arade Basin (southern Portugal).Crossref | GoogleScholarGoogle Scholar |
Puckridge, JT, Sheldon, F, Walker, KF, and Boulton, AJ (1998). Flow variability and the ecology of large rivers. Marine and Freshwater Research 49, 55–72.
| Flow variability and the ecology of large rivers.Crossref | GoogleScholarGoogle Scholar |
Pusey, BJ, Kennard, MJ, Douglas, M, and Allsop, Q (2018). Fish assemblage dynamics in an intermittent river of the northern Australian wet–dry tropics. Ecology of Freshwater Fish 27, 78–88.
| Fish assemblage dynamics in an intermittent river of the northern Australian wet–dry tropics.Crossref | GoogleScholarGoogle Scholar |
Railsback, SF, Lamberson, RH, Harvey, BC, and Duffy, WE (1999). Movement rules for individual-based models of stream fish. Ecological Modelling 123, 73–89.
| Movement rules for individual-based models of stream fish.Crossref | GoogleScholarGoogle Scholar |
Roberts, DT, Duivenvoorden, LJ, and Stuart, IG (2008). Factors influencing recruitment patterns of golden perch (Macquaria ambigua oriens) within a hydrologically variable and regulated Australian tropical river system. Ecology of Freshwater Fish 17, 577–589.
| Factors influencing recruitment patterns of golden perch (Macquaria ambigua oriens) within a hydrologically variable and regulated Australian tropical river system.Crossref | GoogleScholarGoogle Scholar |
Russ, GR, Cheal, AJ, Dolman, AM, Emslie, MJ, Evans, RD, Miller, I, Sweatman, H, and Williamson, DH (2008). Rapid increase in fish numbers follows creation of world’s largest marine reserve network. Current Biology 18, R514–R515.
| Rapid increase in fish numbers follows creation of world’s largest marine reserve network.Crossref | GoogleScholarGoogle Scholar |
Strauch, AM, Kapust, AR, and Jost, CC (2015). Composition and health of fish in refugia habitat of ephemeral tributaries to the lower Zambezi in southern Africa. Marine and Freshwater Research 66, 343–351.
| Composition and health of fish in refugia habitat of ephemeral tributaries to the lower Zambezi in southern Africa.Crossref | GoogleScholarGoogle Scholar |
Stuart, IG, and Sharpe, CP (2020). Riverine spawning, long distance larval drift, and floodplain recruitment of a pelagophilic fish: a case study of golden perch (Macquaria ambigua) in the arid Darling River, Australia. Aquatic Conservation: Marine and Freshwater Ecosystems 30, 675–690.
| Riverine spawning, long distance larval drift, and floodplain recruitment of a pelagophilic fish: a case study of golden perch (Macquaria ambigua) in the arid Darling River, Australia.Crossref | GoogleScholarGoogle Scholar |
Van Horn, DJ, Reale, JK, and Archdeacon, TP (2022). Water quality in three potential drought refuges in an arid-land river: assessing habitat suitability for at-risk fish species. Knowledge and Management of Aquatic Ecosystems 423, 7.
| Water quality in three potential drought refuges in an arid-land river: assessing habitat suitability for at-risk fish species.Crossref | GoogleScholarGoogle Scholar |
Warrego–Darling Monitoring Evaluation Research (2020) Yellowbelly: the fecund Warrego nursery. University of New England, New South Wales Department of Primary Industries, 2rog consulting. Available at https://2rog.com.au/wp-content/uploads/2020/04/12.05.2020-yellabelly.pdf [Verified 12 March 2021]
Whitney, JE, Gido, KB, Marti, EC, and Hase, KJ (2016). The first to arrive and the last to leave: colonisation and extinction dynamics of common and rare fishes in intermittent prairie streams. Freshwater Biology 61, 1321–1334.
| The first to arrive and the last to leave: colonisation and extinction dynamics of common and rare fishes in intermittent prairie streams.Crossref | GoogleScholarGoogle Scholar |
Williams BK, Nichols JD, Conroy MJ (2002) ‘Analysis and management of animal populations.’ (Academic Press: San Diego, CA, USA)
Wright, DW, Zampatti, BP, Baumgartner, LJ, Brooks, D, Butler, GL, Crook, DA, Fanson, BG, Koster, W, Lyon, J, Strawbridge, A, Tonkin, Z, and Thiem, JD (2020). Size, growth and mortality of riverine golden perch (Macquaria ambigua) across a latitudinal gradient. Marine and Freshwater Research 71, 1651–1661.
| Size, growth and mortality of riverine golden perch (Macquaria ambigua) across a latitudinal gradient.Crossref | GoogleScholarGoogle Scholar |
Zampatti, B, and Leigh, S (2013). Effects of flooding on recruitment and abundance of golden perch (Macquaria ambigua ambigua) in the lower River Murray. Ecological Management & Restoration 14, 135–143.
| Effects of flooding on recruitment and abundance of golden perch (Macquaria ambigua ambigua) in the lower River Murray.Crossref | GoogleScholarGoogle Scholar |
Zampatti, BP, Leigh, SJ, Bice, CM, and Rogers, PJ (2018). Multiscale movements of golden perch (Percichthyidae: Macquaria ambigua) in the River Murray, Australia. Austral Ecology 43, 763–774.
| Multiscale movements of golden perch (Percichthyidae: Macquaria ambigua) in the River Murray, Australia.Crossref | GoogleScholarGoogle Scholar |