Size, growth and mortality of riverine golden perch (Macquaria ambigua) across a latitudinal gradient
Daniel W. Wright

A Department of Primary Industries, Narrandera Fisheries Centre, PO Box 182, Narrandera, NSW 2700, Australia.
B Inland Waters and Catchment Ecology Program, SARDI Aquatic Sciences, PO Box 120, Henley Beach, SA 5022, Australia.
C Institute for Land, Water and Society, Charles Sturt University, PO Box 789, Albury, NSW 2640, Australia.
D Department of Agriculture and Fisheries, GPO Box 46, Brisbane, Qld 4001, Australia.
E Department of Primary Industries, Grafton Fisheries Centre, Private Mail Bag 2, Grafton, NSW 2460, Australia.
F Centre for Freshwater Ecosystems, La Trobe University, PO Box 821, Wodonga, Vic. 3689, Australia.
G Arthur Rylah Institute for Environmental Research, Department of Environment, Land, Water and Planning, PO Box 137, Heidelberg, Vic. 3084, Australia.
H Present address: Commonwealth Scientific and Industrial Research Organisation (CSIRO), Locked Bag 2, Glen Osmond, SA 5064, Australia.
I Corresponding author. Email: daniel.wright@dpi.nsw.gov.au
Marine and Freshwater Research 71(12) 1651-1661 https://doi.org/10.1071/MF20056
Submitted: 19 February 2020 Accepted: 2 June 2020 Published: 21 July 2020
Journal Compilation © CSIRO 2020 Open Access CC BY-NC
Abstract
Effective fisheries management requires fish size, growth and mortality information representative of the population and location of interest. Golden perch Macquaria ambigua is long lived, potamodromous and widespread in the Murray–Darling Basin (MDB), Australia. Using a sample spanning 13 river systems and 10° of latitude, we examined whether the maximum size of golden perch differed by latitude and whether growth and mortality varied between northern and southern MDB regions. The length, weight and age ranges of golden perch sampled (n = 873) were 52–559 mm, 2–3201 g and 0+ to 26+ years respectively, and maximum length and weight were unaffected by latitude. Length and age–length distributions represented by age–length keys varied by region, with greater variability in age-at-length and a larger proportion of smaller individuals in northern MDB rivers, which generally exhibit greater variability in discharge. Growth and mortality rates were similar between regions, and an MDB-wide von Bertalanffy growth model (L∞ = 447, k = 0.32 and t0 = –0.51) and instantaneous mortality rate (Z = 0.20) best described the data. An MDB-wide length–weight equation also provided the best fit (W = 6.76 × 10–6 L3.12). Our data suggest that the MDB can be treated as one management unit in terms of golden perch maximum size, growth and mortality parameters.
Additional keywords: age–length key, Bergmann’s rule, Murray–Darling Basin, otolith aging, von Bertalanffy growth function.
Introduction
Robust age and length information, along with derivative growth and mortality rate estimates, are critical to fisheries management (e.g. size-based harvest regulations). The accuracy of this information is improved by using a sample that is representative of the broad population and collected in a standardised manner (e.g. same sampling method, year and time of year; Coggins et al. 2013). For widespread freshwater fish species, geographic span, particularly between latitudes and their associated climates, is a potential source of variation in age–length relationships within populations (Belk and Houston 2002; Heibo et al. 2005; Rypel 2014). In this respect, freshwater fishes may adhere to Bergmann’s rule, which predicts maximum fish size across a species range will increase with latitude from the equator towards the poles or at lower temperatures (Angilletta and Dunham 2003). However, many freshwater fishes also follow the converse to this rule (i.e. decreasing maximum size at latitudes towards the poles or lower temperatures) or exhibit no temperature–size relationship (Belk and Houston 2002; Heibo et al. 2005; Rypel 2014). Consequently, age–length data should be gathered from a wide geographical and latitudinal area relative to a species range and, where appropriate, sample sizes should be bolstered to explore region-specific growth and mortality rates (e.g. Piddocke et al. 2015).
Golden perch Macquaria ambigua is a common native species in the Murray–Darling Basin (MDB) of eastern Australia that supports extensive recreational and historical and contemporary commercial fisheries (Kailola et al. 1993; Lintermans 2007; Earl 2016). Golden perch are a medium-bodied and long-lived fish, reaching ~550-mm total length (TL) and 27 years of age in river habitats (Mallen-Cooper and Stuart 2003; Forbes et al. 2015). Using the life history classifications of Winemiller and Rose (1992), golden perch are considered a periodic species (King et al. 2013). Although they may be strongly site attached for long periods (Crook 2004; O’Connor et al. 2005), golden perch can also undertake facultative migrations of tens to thousands of river kilometres across a range of river conditions at certain times (Reynolds 1983; Zampatti et al. 2018); they are also highly fecund, with females producing >500 000 eggs (Harris and Rowland 1996), and demonstrate episodic recruitment (Zampatti and Leigh 2013). MDB golden perch are genetically distinct from other members of the golden perch species complex that are found in the Lake Eyre, Bulloo and Fitzroy basins (Beheregaray et al. 2017). Throughout the MDB, minimal genetic structuring in golden perch reflects their ability to migrate and disperse long distances (Faulks et al. 2010; Beheregaray et al. 2017; Attard et al. 2018). Nevertheless, genetically similar MDB golden perch may still grow and survive at different rates depending on climatic conditions, such as rainfall run-off and temperature, which vary over the broad geographical region of the MDB (Attard et al. 2018).
Riverine golden perch age–length relationships have been previously examined, but not from a whole-of-range sample. Anderson et al. (1992) assessed the growth rate of golden perch (n = 796) in six river systems throughout the southern MDB, although that study was somewhat confounded by including typically larger golden perch from impoundments (Mallen-Cooper and Stuart 2003; Forbes et al. 2015). Age–length relationships have also been explored for golden perch in three separate rivers in the northern and southern MDB, although sample sizes were variable (n = 39–216; Mallen-Cooper and Stuart 2003). In a comparison of golden perch growth rates between reservoir and river systems, Forbes et al. (2015) used large sample sizes (n = 540–582) from two rivers in the southern MDB. Nevertheless, suitably robust age–length information, in terms of both sample size and geographical coverage, is lacking. Identifying whether region-specific demographic parameters (e.g. growth and mortality rates) differ is particularly valuable for future monitoring and management efforts because distinct management units may need to be treated differently.
In the present study we used an extensive sample of MDB golden perch collected from 13 river systems throughout their geographic range to examine demographic parameters across a latitudinal gradient. Vital population statistics, including maximum body sizes, growth and mortality rates and length–weight relationships, were developed to provide contemporary whole-of-range information to support and evaluate current and future fisheries management. This was undertaken to: (1) test the hypothesis that, following Bergmann’s rule (Angilletta and Dunham 2003), maximum body size would increase with latitude in a southerly direction and lower temperatures (and potentially other correlated environmental variables, such as discharge volume and variability); and (2) determine whether distinct spatial units, such as northern and southern MDB regions, are required in golden perch management due to differing demographic parameters.
Materials and methods
Between November 2017 and June 2018, 873 golden perch were sampled from 13 river systems throughout the MDB, Australia, spanning 10° of latitude and a range of temperature regimes and hydrological characteristics (Fig. 1, 2). Sampling occurred at multiple sites in eight river systems (including the upper Darling River) in the northern MDB region (latitudinal range from 26.9 to 32.2°S) and in six rivers (including the lower Darling River) in the southern MDB region (latitudinal range from 32.6 to 36.7°S). Southern and northern regions were above and below the Menindee main weir in New South Wales (NSW) on the Darling River (32.4°S, 142.4°E), which was near the latitudinal midpoint of sampling locations and is a semi-impermeable barrier to fish passage separating the two regions. Of the 873 individuals collected, 429 and 444 were from the southern and northern MDB respectively. All individuals were captured using boat-mounted electrofishing (either GPP 5 or 7.5-kW boat-mounted units, Smith-Root, Vancouver, WA, USA) and killed at the point of capture using an anaesthetic overdose (immersion in benzocaine or AQUI-S, AQUI-S New Zealand Ltd, Lower Hutt, New Zealand). Fish were then kept on an ice slurry and were subsequently measured for TL (mm) before sagittal otolith extraction. A subset of individuals (n = 863) was also measured for whole weight (W; g) at the time of sampling. A further subset (n = 704 individuals) was sexed. Methods previously validated for annual age estimation of golden perch (Stuart 2006) were used in this study. Whole otoliths were embedded in clear casting resin and, after setting, were hardened for a minimum of 4 h at 40°C in a drying oven fitted with a Eurotherm 3216 controller (Steridium, Gold Coast, Qld, Australia). Using a Isomet low-speed saw (Buehler, Lake Bluff, IL, USA), multiple 400–500 µm transverse sections were cut through the primordium and the sections were then fixed to a microscope slide with super glue (Morison et al. 1998). Annulus counts for each fish were performed by two experienced readers (A. Strawbridge and D. Fleer.) under a Wild M3C microscope (Leica Biosystems, Wetzlar, Germany) at a magnification of 16×, and age (t) was assessed based on the annulus count and edge type in relation to the sampling date of an individual, with a nominal birthdate of 1 October assigned for all individuals (Stuart 2006).
![]() |
![]() |
This study was conducted in accordance with animal care and ethics committee guidelines (NSW: NSW Animal Care and Ethics permit 14/10 and Scientific Collection Permit P01/0059(A)-3.0; Queensland: General Fisheries Permit 186281 and Animal Care and Ethics CA 2016/01/938; South Australia: under an exemption (No. 9902132) of Section 115 of the Fisheries Management Act 2007 and following the South Australian Animal Welfare Act 1985; and Victoria: Victorian Fisheries Research Permit RP827, Fauna and Flora Guarantee Research Permit 10007273, Department of Environment, Land, Water and Planning Animal Ethics 14/04).
Statistical analysis
Two alternative approaches were used to examine the relationship between age and length of golden perch. First, age–length keys were used to assess age–length distributions; second, von Bertalanffy growth models (VBGMs) were used to investigate growth rates. To predict the proportion of annual ages at each length interval, age–length keys (ALKs) were modelled using multinomial logistic regression model analysis with a maximum of 100 iterations (Ogle 2016). This assigned probabilities to each age–length category, even in the absence of data. Age categories ≥14+ years were combined because there were few contributing individuals in older age classes. Growth rate estimation, allowing predictions of mean length at age (E[L|t]), was examined using the VBGM (von Bertalanffy 1938; Ogle 2016):

where L∞ is the maximum mean length of an individual, k is the growth coefficient and t0 is the theoretical age at length zero.
In addition, we used ages in catch curve linear regression models to predict instantaneous mortality rates (Z; Ogle 2016):
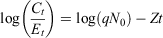
where N0 is starting population size, Ct ÷ Et is catch per unit effort, t is age and q is the constant proportion of the population caught. We only included the age classes at and above the maximum catch, which was approximately at 4 years of age (Smith et al. 2012; Ogle 2016), to ensure that fish were large enough for capture with the sampling method.
Finally, linear regression models were used to predict length–weight relationships between log10(L) and log10(W) to assess condition (Ogle 2016).
Relationships between maximum body size, in terms of length and log-transformed weight, and latitude at sampling sites with ≥10 individuals were examined using linear regression model analysis. Length–frequency distributions were compared between northern and southern MDB golden perch by a two-sample Kolmogorov–Smirnoff test. Differences in multinomial model ALKs between golden perch captured in the northern and southern MDB were assessed by a likelihood ratio test. Non-linear model analysis examined differences in VBGMs between golden perch in the northern and southern MDB (nlstools packages, R Foundation for Statistical Computing, Vienna, Austria, see https://cran.r-project.org/web/packages/nlstools/, accessed October 2019; Baty et al. 2015). In this analysis, we used a model selection approach to compare models from different geographic regions using all combinations of coefficients. Models with all coefficient combinations ({L∞}, {k}, {t0}, {L∞, k}, {L∞, t0}, {k, t0}, {L∞, k, t0} and the null model {Ω}) were ranked using the Akaike information criterion (AIC), and the model that had the lowest AIC was deemed to have the best fit (after Ogle 2016). Models with a ΔAIC of <2 were also identified as having substantial support (Burnham and Anderson 2002). Linear regression model length–weight relationships were compared between northern and southern MDB golden perch using a Type II analysis of variance (ANOVA) to identify whether condition varied between regions. Concomitantly, to test whether mortality rate differed between regions, we examined differences in linear regression model catch curves predicting instantaneous mortality rates (Z) for northern and southern MDB golden perch using a Type II ANOVA. Residual plots and histograms of residuals were used to confirm parametric assumptions were met. All statistical analyses were performed in R (ver. 3.5.2, R Foundation for Statistical Computing, Vienna, Austria, see http://www.R-project.org/, accessed March 2019).
Results
Golden perch lengths and ages were in the range 54–559 mm and 0+ to 26+ years in the northern MDB, and 52–549 mm and 0+ to 24+ years in the southern MDB (Table 1). Weights of sampled golden perch in the northern and southern MDB were in the range 2–3174 and 12–3210 g respectively (Table 1). Sex ratios (males : females) were 1 : 1.8 (n = 385 individuals) and 1 : 2.2 (n = 319 individuals) in the northern and southern regions respectively. Latitude did not affect maximum body size in terms of length (R2 = 0.04, F1,37 = 1.66, P = 0.21) or weight (R2 = 0.07, F1,37 = 2.80, P = 0.10) at sites with ≥10 individuals (see Fig. S1, available as Supplementary material to this paper).
Length–frequency distributions differed between northern and southern regions of the MDB (P < 0.01), with greater proportions of smaller golden perch in the northern MDB (Fig. 3). Differences in age–length composition between regions were identified in ALK comparisons (P < 0.01), with golden perch in the northern MDB demonstrating a more variable age–length distribution. For example, an individual of 300 mm in length in the northern region would have a >0.01 probability of belonging to an age category between 1+ and 13+ years, whereas an individual of the same length in the southern region would have the same probability of being aged between 2+ and 9+ years (Fig. 4; Tables S1, S2).
![]() |
![]() |
Using VBGMs to analyse growth rates, we found the null {Ω} model without regional differences to be the top-ranked VBGM, using overall coefficients of L∞ = 447, k = 0.32 and t0 = –0.51 (Tables 1, 2; Fig. 5, S2). There was also substantial support (ΔAIC < 2) for additional models including regional differences in the coefficients (or combinations of coefficients) {L∞}, {L∞, k}, {L∞, t0} and {k} in VBGMs (Table 2). These mostly included L∞, which was smaller in the northern MDB (439 mm; 95% confidence interval (CI) 415–442) than in the southern MDB (447 mm; 95% CI 445–465; Tables 1, 2; Fig. 5, S3, S4). Prediction intervals (PI) in L∞ also varied between the northern (95% PI 372–506) and southern (95% PI 403–506) MDB (Fig. 5, 6).
![]() |
![]() |
Slopes of length–weight relationships were not significantly different between the northern and southern regions of the MDB (F = 0.68; d.f. = 1, 864; P = 0.41), and the overall model W = 6.76 × 10–6L3.12 explained most of the variation observed (r2 = 0.99; Fig. 6).
Golden perch mortality rates were comparable between the northern and southern MDB (F = 3.53; d.f. = 1, 40; P = 0.07), and an instantaneous mortality rate Z = 0.20, translating to an annual mortality rate (A) of 18%, was determined for the overall population (Fig. 7).
![]() |
Discussion
Rather than following Bergmann’s rule, namely that animals reach larger maximum body sizes at latitudes towards the poles or at cooler temperatures (Angilletta and Dunham 2003), golden perch maximum length and weight was not affected by latitude in the present study. These results suggest that golden perch do not have a pronounced body size relationship with latitude or temperature. There was no conclusive evidence for differences in growth rates, and similar mortality rates and length–weight relationships were also identified for golden perch between the northern MDB (28 to 32°S) and southern MDB (33 to 37°S) regions. Thus, this study indicates that the MDB may be considered as a single management unit for golden perch from the perspective of maximum size, growth and mortality demographic parameters.
Many freshwater fish species exhibit the converse of Bergmann’s rule, particularly warm-water species, which may have reduced growth opportunities and longevity at cooler temperatures (Belk and Houston 2002; Rypel 2014). Golden perch, which could be considered a warm-water or transitional species based on limited knowledge of their thermal preference and tolerance (Lyon et al. 2008), do not appear to have a relationship between body size and latitude or temperature. In other taxa, size–latitude relationships are known to weaken for highly mobile species that can evade environmental extremes by repositioning themselves (Ramirez et al. 2008). In this respect, golden perch may, at times, be highly mobile, such as during broad-scale flooding when riverine connectivity is high (Reynolds 1983; Zampatti et al. 2018). Nevertheless, in the MDB there may be long periods (years) when low discharge and a multitude of instream barriers prevent large-scale fish movement and the ability to escape environmental extremes (Baumgartner et al. 2014). Consequently, it is unlikely that movement is the sole reason that golden perch do not adhere to Bergmann’s rule.
Golden perch growth rate, mortality rate and condition (length–weight relationship) were similar between the northern and southern MDB regions. A factor contributing to these similarities was that we sampled many rivers representative of the variety of environmental conditions across regions. This may have masked differences in parameters between regions for specific river types (e.g. perennial or ephemeral). Anthropogenic effects on river systems also conceivably affected golden perch demographic parameters between regions. For example, recreational fishing pressure (Cooke and Cowx 2004) and human-driven climate and hydrological modifications (Hobday and Lough 2011; Baumgartner et al. 2014) may be reducing growth opportunities and survival throughout the MDB. Global warming also has the potential to increase golden perch growth in cooler southern regions (Morrongiello et al. 2011) and decrease growth in warmer northern regions, conceivably leading to equivocal growth, survival and condition in both regions. Again, large-scale movement by golden perch, enabling access to favourable environments and between regions, is another potential factor explaining equivalent demographic parameters between regions, but these movements are compromised much of the time by barriers to fish.
We generated a VBGM from a representative, whole-of-range sample of riverine MDB golden perch. This model differs substantially from previous models (Fig. 8; Table S3), which can be plausibly explained. First, in contrast to previous studies of individual rivers or regions, we accounted for potential among-river and among-region differences by sampling a range of rivers within the northern and southern MDB. Second, unlike Anderson et al. (1992), we excluded individuals from impoundments, which typically grow faster and larger (Mallen-Cooper and Stuart 2003; Forbes et al. 2015). Large sample sizes of particularly small or large individuals can considerably affect growth models (Lucena and O’Brien 2001). Third, we used a standardised boat electrofishing method to collect all samples, rather than multiple sampling methods (e.g. gill-netting, fyke netting, angler captures and specimen donations), which may have altered the age–length structure of samples used between the present and some previous models (Anderson et al. 1992). However, we acknowledge that there may be some effect of electrofishing capture probability on the lengths of fish captured (Lyon et al. 2014). Fourth, we collected samples within a single year, whereas previous studies have collected samples over multiple years. Despite methodological differences across studies, the lower growth rate in the present study in 2017–18 (k = 0.31) and in the study of Forbes et al. (2015) in 2009–13 (k = 0.14–0.37) compared with those before 2004 (k = 0.45–0.57) warrants further investigation into a possible temporal decline in golden perch growth rate (e.g. from global warming or discharge alteration; Hobday and Lough 2011; Morrongiello et al. 2011; Tonkin et al. 2017; Huss et al. 2019).
![]() |
The oldest golden perch recorded in this study was 26 years, almost equal to the maximum age known for the species of 27 years (Forbes et al. 2015). The overall annual mortality rate of 18% observed here includes both recreational fishing and natural sources of mortality. In regions of the MDB, recreational fishing can remove >30% of fish in some size classes each year (Allen et al. 2009), and this may have overshadowed patterns in natural mortality within the present study. Interestingly, the overall length–weight relationship (W = 6.76 × 10–6L3.12; e.g. 500-mm fish = 1781 g) determined in this study indicates poorer condition than in 1959–70 (W = 7.08 × 10–6L3.12; e.g. 500-mm fish = 1866 g; Llewellyn 2011) and in 1984–91 (W = 3.34 × 10–7L3.66; e.g. 500-mm fish = 2523 g) (Anderson et al. 1992). However, the sample used in Anderson et al. (1992) included a significant number of large fish from impoundments and few fish <100 mm, thus potentially biasing their length–weight relationship. Deteriorating golden perch condition over time could again be linked to discharge alterations or global warming.
Length and age–length distributions (from ALKs) differed between northern and southern MDB regions, with greater variability in age-at-length data in the northern MDB. Regional differences in ALKs, but not VBGMs, likely resulted from ALK age-at-length probability matrices being more sensitive to detecting age variation within length classes than VBGMs forcing a single curve or age-at-length prediction through all data (Goodyear 1997). Greater age-at-length variation in the northern MDB may relate to the hydrological diversity of rivers in this region (Thoms and Sheldon 2000). In the ephemeral northern rivers, rewetting and episodic flooding could contribute more to growth (Sternberg et al. 2008; Balcombe and Arthington 2009), but low discharge over extended periods of drought may lessen growth (Tonkin et al. 2017).
Golden perch appear to have no relationship between maximum size and latitude or temperature. Using a VBGM analysis, an MDB-wide growth model was found to be most appropriate to predict age-at-length for golden perch throughout its range. Nevertheless, ALKs differed between northern and southern regions of the MDB, and in the northern region greater variation in age-at-length data may be associated with increased ephemerality of some rivers. Consequently, to predict age-at-length probabilities for many fish within each length category, region-specific ALKs would be most appropriate, whereas to predict the age of individual fish within a length category an MDB-wide VBGM would suffice. We advocate that researchers considering fish age–length data similarly examine geographic differences and use both VBGM and ALK approaches to provide end users with case-by-case models. Our data were collected within a single year, and the demographic parameters presented here will vary through time as recruitment and cohort strength vary. Thus, further investigation will be essential to: (1) ensure demographic parameters remain relevant for golden perch management; and (2) identify long-term trends in these parameters and the causal effects. Based on our contemporary results, golden perch size, growth, mortality and condition do not differ between northern and southern MDB regions, and the MDB should be considered as a single management unit for these demographic parameters.
Conflicts of interest
Lee J. Baumgartner is an Associate Editor of Marine and Freshwater Research. Despite this relationship, he did not at any stage have Associate Editor-level access to this manuscript while in peer review, as is the standard practice when handling manuscripts submitted by an editor to this journal. Marine and Freshwater Research encourages its editors to publish in the Journal and they are kept totally separate from the decision-making process for their manuscripts. The authors declare that they have no further conflicts of interest.
Declaration of funding
Funding for this study was provided by the Commonwealth Environmental Water Office, Australian Government, under a Murray–Darling Basin Environmental Water Knowledge and Research project, with additional support provided by the Commonwealth Environmental Water Office under its Long-term Intervention Monitoring Project and the Recreational Fishing Freshwater Trust Expenditure Committee. In addition, some sample collections were funded by the Victorian Environmental Flow Monitoring and Assessment Program (Department of Environment, Land, Water and Planning).
Acknowledgements
The authors thank the following staff for their invaluable assistance with field and laboratory work: from NSW, Mark Babbs, Tom Butterfield, Jonathon Doyle, Duncan McLay, Rohan Rehwinkel, Chris Smith and Ian Wooden; from Queensland, Daniel Brooks and Daniel Smith; from South Australia, Chris Bice, David Fleer, George Giatas and Ian Magraith; and from Victoria, David Dawson, Graeme Hackett, Adrian Kitchingman, Jason Lieschke, Andrew Pickworth and Joanne Sharley.
References
Allen, M. S., Brown, P., Douglas, J., Fulton, W., and Catalano, M. (2009). An assessment of recreational fishery harvest policies for Murray cod in southeast Australia. Fisheries Research 95, 260–267.| An assessment of recreational fishery harvest policies for Murray cod in southeast Australia.Crossref | GoogleScholarGoogle Scholar |
Anderson, J., Morison, A., and Ray, D. (1992). Validation of the use of thin-sectioned otoliths for determining the age and growth of golden perch, Macquaria ambigua (Perciformes: Percichthyidae), in the lower Murray–Darling Basin, Australia. Marine and Freshwater Research 43, 1103–1128.
| Validation of the use of thin-sectioned otoliths for determining the age and growth of golden perch, Macquaria ambigua (Perciformes: Percichthyidae), in the lower Murray–Darling Basin, Australia.Crossref | GoogleScholarGoogle Scholar |
Angilletta, M. J., and Dunham, A. E. (2003). The temperature–size rule in ectotherms: simple evolutionary explanations may not be general. American Naturalist 162, 332–342.
| The temperature–size rule in ectotherms: simple evolutionary explanations may not be general.Crossref | GoogleScholarGoogle Scholar | 12970841PubMed |
Attard, C. R. M., Brauer, C. J., Sandoval-Castillo, J., Faulks, L. K., Unmack, P. J., Gilligan, D. M., and Beheregaray, L. B. (2018). Ecological disturbance influences adaptive divergence despite high gene flow in golden perch (Macquaria ambigua): implications for management and resilience to climate change. Molecular Ecology 27, 196–215.
| Ecological disturbance influences adaptive divergence despite high gene flow in golden perch (Macquaria ambigua): implications for management and resilience to climate change.Crossref | GoogleScholarGoogle Scholar |
Balcombe, S. R., and Arthington, A. H. (2009). Temporal changes in fish abundance in response to hydrological variability in a dryland floodplain river. Marine and Freshwater Research 60, 146–159.
| Temporal changes in fish abundance in response to hydrological variability in a dryland floodplain river.Crossref | GoogleScholarGoogle Scholar |
Baty, F., Ritz, C., Charles, S., Brutsche, M., Flandrois, J.-P., and Delignette-Muller, M.-L. (2015). A toolbox for nonlinear regression in R: the package nlstools. Journal of Statistical Software 66, 1–21.
| A toolbox for nonlinear regression in R: the package nlstools.Crossref | GoogleScholarGoogle Scholar |
Baumgartner, L., Zampatti, B., Jones, M., Stuart, I., and Mallen-Cooper, M. (2014). Fish passage in the Murray–Darling Basin, Australia: not just an upstream battle. Ecological Management & Restoration 15, 28–39.
| Fish passage in the Murray–Darling Basin, Australia: not just an upstream battle.Crossref | GoogleScholarGoogle Scholar |
Beheregaray, L. B., Pfeiffer, L. V., Attard, C. R. M., Sandoval-Castillo, J., Domingos, F. M. C. B., Faulks, L. K., Gilligan, D. M., and Unmack, P. J. (2017). Genome-wide data delimits multiple climate-determined species ranges in a widespread Australian fish, the golden perch (Macquaria ambigua). Molecular Phylogenetics and Evolution 111, 65–75.
| Genome-wide data delimits multiple climate-determined species ranges in a widespread Australian fish, the golden perch (Macquaria ambigua).Crossref | GoogleScholarGoogle Scholar | 28347889PubMed |
Belk, M. C., and Houston, D. D. (2002). Bergmann’s rule in ectotherms: a test using freshwater fishes. American Naturalist 160, 803–808.
| Bergmann’s rule in ectotherms: a test using freshwater fishes.Crossref | GoogleScholarGoogle Scholar | 18707466PubMed |
Burnham, K. P., and Anderson, D. R. (2002). ‘Model Selection and Multimodel Inference: A Practical Information–Theoretic Approach’, 2nd edn. (Springer: New York, NY, USA.)
Coggins, L. G., Gwinn, D. C., and Allen, M. S. (2013). Evaluation of age–length key sample sizes required to estimate fish total mortality and growth. Transactions of the American Fisheries Society 142, 832–840.
| Evaluation of age–length key sample sizes required to estimate fish total mortality and growth.Crossref | GoogleScholarGoogle Scholar |
Cooke, S. J., and Cowx, I. G. (2004). The role of recreational fishing in global fish crises. Bioscience 54, 857–859.
| The role of recreational fishing in global fish crises.Crossref | GoogleScholarGoogle Scholar |
Crook, D. A. (2004). Is the home range concept compatible with the movements of two species of lowland river fish? Journal of Animal Ecology 73, 353–366.
| Is the home range concept compatible with the movements of two species of lowland river fish?Crossref | GoogleScholarGoogle Scholar |
Earl, J. (2016). Fishery statistics for the South Australian Lakes and Coorong Fishery 2014/15. South Australian Research and Development Institute (Aquatic Sciences), Adelaide, SA, Australia.
Faulks, L. K., Gilligan, D. M., and Beheregaray, L. B. (2010). Clarifying an ambiguous evolutionary history: range-wide phylogeography of an Australian freshwater fish, the golden perch (Macquaria ambigua). Journal of Biogeography 37, 1329–1340.
| Clarifying an ambiguous evolutionary history: range-wide phylogeography of an Australian freshwater fish, the golden perch (Macquaria ambigua).Crossref | GoogleScholarGoogle Scholar |
Forbes, J. P., Watts, R. J., Robinson, W. A., Baumgartner, L. J., Allen, M. S., McGuffie, P., Cameron, L. M., and Crook, D. A. (2015). System-specific variability in murray cod and golden perch maturation and growth influences fisheries management options. North American Journal of Fisheries Management 35, 1226–1238.
| System-specific variability in murray cod and golden perch maturation and growth influences fisheries management options.Crossref | GoogleScholarGoogle Scholar |
Goodyear, C. P. (1997). Fish age determined from length: an evaluation of three methods using simulated red snapper data. Fisheries Bulletin 44, 39–46.
Harris, J., and Rowland, S. (1996). Family Percichthyidae: Australian freshwater cods and basses. In ‘Freshwater Fishes of South-Eastern Australia’. (Ed. R. McDowall.) pp. 150–163. (Reed Books: Sydney, NSW, Australia.)
Heibo, E., Magnhagen, C., and Vøllestad, L. A. (2005). Latitudinal variation in life history traits in Eurasian perch. Ecology 86, 3377–3386.
| Latitudinal variation in life history traits in Eurasian perch.Crossref | GoogleScholarGoogle Scholar |
Hobday, A. J., and Lough, J. M. (2011). Projected climate change in Australian marine and freshwater environments. Marine and Freshwater Research 62, 1000–1014.
| Projected climate change in Australian marine and freshwater environments.Crossref | GoogleScholarGoogle Scholar |
Huss, M., Lindmark, M., Jacobson, P., van Dorst, R. M., and Gårdmark, A. (2019). Experimental evidence of gradual size-dependent shifts in body size and growth of fish in response to warming. Global Change Biology 25, 2285–2295.
| Experimental evidence of gradual size-dependent shifts in body size and growth of fish in response to warming.Crossref | GoogleScholarGoogle Scholar | 30932292PubMed |
Kailola, P. J., Williams, M. J., Stewart, P. C., Reichelt, R. E., McNee, A., and Grieve, C. (1993). ‘Australian Fisheries Resources.’ (Bureau of Resources Sciences, Department of Primary Industries and Energy: Fisheries Research and Development Corporation: Canberra, ACT, Australia.)
King, A., Humphries, P., and McCasker, N. (2013). Reproduction and early life history. In ‘Ecology of Australian Freshwater Fishes’. (Eds P. Humphries and K. Walker.) pp. 159–194. (CSIRO Publishing: Melbourne, Vic., Australia.)
Lintermans, M. (2007). ‘Fishes of the Murray–Darling Basin: An Introductory Guide’ (Murray–Darling Basin Commission: Canberra, ACT, Australia.)
Llewellyn, L. (2011). Length–weight relationships of freshwater fish from the Murray–Darling River System in inland New South Wales, with particular reference to the Golden Perch Macquaria ambigua. Australian Zoologist 35, 435–457.
| Length–weight relationships of freshwater fish from the Murray–Darling River System in inland New South Wales, with particular reference to the Golden Perch Macquaria ambigua.Crossref | GoogleScholarGoogle Scholar |
Lucena, F. M., and O’Brien, C. M. (2001). Effects of gear selectivity and different calculation methods on estimating growth parameters of bluefish, Pomatomus saltatrix (Pisces: Pomatomidae), from southern Brazil. Fishery Bulletin 99, 432–442.
Lyon, J. P., Ryan, T. J., and Scroggie, M. P. (2008). Effects of temperature on the fast-start swimming performance of an Australian freshwater fish. Ecology Freshwater Fish 17, 184–188.
| Effects of temperature on the fast-start swimming performance of an Australian freshwater fish.Crossref | GoogleScholarGoogle Scholar |
Lyon, J. P., Bird, T., Nicol, S., Kearns, J., O’Mahony, J., Todd, C. R., Cowx, I. G., and Bradshaw, C. J. (2014). Efficiency of electrofishing in turbid lowland rivers: implications for measuring temporal change in fish populations. Canadian Journal of Fisheries and Aquatic Sciences 71, 878–886.
| Efficiency of electrofishing in turbid lowland rivers: implications for measuring temporal change in fish populations.Crossref | GoogleScholarGoogle Scholar |
Mallen-Cooper, M., and Stuart, I. G. (2003). Age, growth and non-flood recruitment of two potamodromous fishes in a large semi-arid/temperate river system. River Research and Applications 19, 697–719.
| Age, growth and non-flood recruitment of two potamodromous fishes in a large semi-arid/temperate river system.Crossref | GoogleScholarGoogle Scholar |
Morison, A. K., Robertson, S. G., and Smith, D. C. (1998). An integrated system for production fish aging: image analysis and quality assurance. North American Journal of Fisheries Management 18, 587–598.
| An integrated system for production fish aging: image analysis and quality assurance.Crossref | GoogleScholarGoogle Scholar |
Morrongiello, J. R., Crook, D. A., King, A. J., Ramsey, D. S., and Brown, P. (2011). Impacts of drought and predicted effects of climate change on fish growth in temperate Australian lakes. Global Change Biology 17, 745–755.
| Impacts of drought and predicted effects of climate change on fish growth in temperate Australian lakes.Crossref | GoogleScholarGoogle Scholar |
O’Connor, J. P., O’Mahony, D. J., and O’Mahony, J. M. (2005). Movements of Macquaria ambigua, in the Murray River, south-eastern Australia. Journal of Fish Biology 66, 392–403.
| Movements of Macquaria ambigua, in the Murray River, south-eastern Australia.Crossref | GoogleScholarGoogle Scholar |
Ogle, D. H. (2016). ‘Introductory Fisheries Analyses with R.’ (Chapman and Hall/CRC: Boca Raton, FL, USA.)
Piddocke, T. P., Butler, G. L., Butcher, P. A., Stewart, J., Bucher, D. J., and Christidis, L. (2015). Age and growth of mangrove red snapper Lutjanus argentimaculatus at its cool-water-range limits. Journal of Fish Biology 86, 1587–1600.
| Age and growth of mangrove red snapper Lutjanus argentimaculatus at its cool-water-range limits.Crossref | GoogleScholarGoogle Scholar | 25943148PubMed |
Ramirez, L., Diniz-Filho, J. A. F., and Hawkins, B. A. (2008). Partitioning phylogenetic and adaptive components of the geographical body-size pattern of New World birds. Global Ecology and Biogeography 17, 100–110.
Reynolds, L. (1983). Migration patterns of five fish species in the Murray–Darling River system. Marine and Freshwater Research 34, 857–871.
| Migration patterns of five fish species in the Murray–Darling River system.Crossref | GoogleScholarGoogle Scholar |
Rypel, A. L. (2014). The cold-water connection: Bergmann’s rule in North American freshwater fishes. American Naturalist 183, 147–156.
| The cold-water connection: Bergmann’s rule in North American freshwater fishes.Crossref | GoogleScholarGoogle Scholar | 24334744PubMed |
Smith, M. W., Then, A. Y., Wor, C., Ralph, G., Pollock, K. H., and Hoenig, J. M. (2012). Recommendations for catch-curve analysis. North American Journal of Fisheries Management 32, 956–967.
| Recommendations for catch-curve analysis.Crossref | GoogleScholarGoogle Scholar |
Sternberg, D., Balcombe, S., Marshall, J., and Lobegeiger, J. (2008). Food resource variability in an Australian dryland river: evidence from the diet of two generalist native fish species. Marine and Freshwater Research 59, 137–144.
| Food resource variability in an Australian dryland river: evidence from the diet of two generalist native fish species.Crossref | GoogleScholarGoogle Scholar |
Stuart, I. G. (2006). Validation of otoliths for determining age of golden perch, a long-lived freshwater fish of Australia. North American Journal of Fisheries Management 26, 52–55.
| Validation of otoliths for determining age of golden perch, a long-lived freshwater fish of Australia.Crossref | GoogleScholarGoogle Scholar |
Thoms, M., and Sheldon, F. (2000). Water resource development and hydrological change in a large dryland river: the Barwon–Darling River, Australia. Journal of Hydrology 228, 10–21.
| Water resource development and hydrological change in a large dryland river: the Barwon–Darling River, Australia.Crossref | GoogleScholarGoogle Scholar |
Tonkin, Z., Kitchingman, A., Lyon, J., Kearns, J., Hackett, G., O’Mahony, J., Moloney, P. D., Krusic-Golub, K., and Bird, T. (2017). Flow magnitude and variability influence growth of two freshwater fish species in a large regulated floodplain river. Hydrobiologia 797, 289–301.
| Flow magnitude and variability influence growth of two freshwater fish species in a large regulated floodplain river.Crossref | GoogleScholarGoogle Scholar |
von Bertalanffy, L. (1938). A quantitative theory of organic growth (inquiries on growth laws. II). Human Biology 10, 181–213.
Winemiller, K. O., and Rose, K. A. (1992). Patterns of life-history diversification in North American fishes: implications for population regulation. Canadian Journal of Fisheries and Aquatic Sciences 49, 2196–2218.
| Patterns of life-history diversification in North American fishes: implications for population regulation.Crossref | GoogleScholarGoogle Scholar |
Zampatti, B. P., and Leigh, S. J. (2013). Within-channel flows promote spawning and recruitment of golden perch, Macquaria ambigua ambigua – implications for environmental flow management in the River Murray, Australia. Marine and Freshwater Research 64, 618–630.
| Within-channel flows promote spawning and recruitment of golden perch, Macquaria ambigua ambigua – implications for environmental flow management in the River Murray, Australia.Crossref | GoogleScholarGoogle Scholar |
Zampatti, B. P., Leigh, S. J., Bice, C. M., and Rogers, P. J. (2018). Multiscale movements of golden perch (Percichthyidae: Macquaria ambigua) in the River Murray, Australia. Austral Ecology 43, 763–774.
| Multiscale movements of golden perch (Percichthyidae: Macquaria ambigua) in the River Murray, Australia.Crossref | GoogleScholarGoogle Scholar |