Mpox diagnostics: a swift and integrated approach to outbreak control
Chuan Kok Lim

A
B
C
![]() Dr Chuan Kok Lim is a medical virologist at the Victorian Infectious Diseases Reference Laboratory (VIDRL) and senior research fellow at the Peter Doherty Institute for Infection and Immunity. His research focuses on advancing pandemic preparedness through novel diagnostic development and genomic characterisation of emerging viral pathogens including orthopoxvirus and flavivirus. |
![]() Dr Shivani Pasricha is a microbiologist and laboratory head in the Department of Infectious Diseases, The University of Melbourne. Using molecular and genomic approaches, her research aims to improve the detection, prevention and surveillance of sexually transmitted infections (STIs). Her current research includes developing cutting-edge CRISPR-diagnostics for the point-of-care detection of STIs and antimicrobial resistance. |
![]() Dr Soo Jen Low is a research officer and bioinformatician at the Peter Doherty Institute for Infection and Immunity. Her research focuses on the application of genomics and development of bioinformatic pipelines to support innovative diagnostic approaches. |
Abstract
The unprecedented global spread of monkeypox (mpox) through physical and sexual transmission revealed significant gaps in diagnostics and sexually transmitted infection (STI) outbreak preparation. An early understanding of viral infectivity and shedding dynamics is critical in informing testing strategies. Capability for viral culture in high-containment laboratories allows rapid dissemination of control materials and evaluation of high-throughput assays, in preparation for testing upscaling. Early genomic characterisation in local clusters informs suitable public health applications and limitations. Monkeypox virus (MPXV) genomics can provide global contextualisation and assist in determining disease importation. As mpox outbreak control strategies evolve towards preventing importation, investment in point-of-care rapid diagnostics such as CRISPR-based lateral flow assays is critical for an agile STI disease-control response.
Keywords: clustered regularly interspaced short palindromic repeats, CRISPR, mpox, monkeypox virus, MPXV, PoCT, point-of-care test, rapid diagnostics, recombinase polymerase amplification, RPA, sexually transmitted infections, STI.
2022 mpox outbreaks reveal diagnostic gaps
Prior to 2022, monkeypox (mpox) was largely considered a zoonotic pathogen. Infections were predominantly described from the African continent with occasional overspill events as a result of contact between humans and small rodents.1 The global transmissions of mpox in 2022, manifested predominately through close or sexual contacts among men who have sex with men was unanticipated.2 Owing to the lack of commercial assays and accessibility to control materials (containment requirements), diagnoses of mpox at the early stage of the outbreak were restricted to specialised laboratories.
As part of Australia’s outbreak response, literature review and mapping of existing diagnostic capabilities were conducted.3 It highlighted several challenges in the control of mpox, for example, paucity of evidence on viral shedding dynamics and infectivity, the extent of serological cross-reactivity, absence of rapid diagnostics and a lack of genomic characterisation to inform transmission linkages.
Diagnostics strategies for mpox control
For early diagnosis and control of mpox, a centralised high-throughput testing in a reference laboratory was considered the preferred approach initially. This was carefully balanced against biosafety considerations, readiness of multi-targeted mpox assays to minimise diagnostic escape, capabilities to conduct viral culture and genomic characterisation and better coordinated responses. This approach was effective in the eradication of mpox from Australia across multiple states. As mpox continues to spread across the globe in 2023–24, diagnostic strategies evolved to focus on early detection of symptomatic returned travellers to prevent local spread. As eradication remains the priority of the disease control strategy in Australia, assays with high sensitivity, specificity and rapid turnaround time are critical.
Evaluation of viral dynamics and shedding
As mpox shedding dynamics were poorly described during the early stage of the outbreak, studies conducted to evaluate the viral shedding patterns were particularly important in informing testing and control strategies.4–8 Multiple body site sampling tests were conducted in the Victorian Infectious Diseases Reference Laboratory (VIDRL) in partnership with Melbourne Sexual Health Centre (MSHC) to evaluate monkeypox virus (MPXV) shedding patterns, viral loads and infectivity of different samples types.9 Virus shedding was observed from multiple body sites; in symptomatic individuals, swabs obtained from involved sites (vesicles, anorectal) have the highest yield whereas oropharyngeal swabs were more useful for pre-symptomatic individuals.9,10 This information was important in guiding public health policy in testing high exposure risk individuals. In parallel infectivity studies demonstrated viability of MPXV in oral and anal sites, indicating that abstinence from close physical and sexual contact for a longer period of time is required to prevent transmission.10
Rapid multi-target assay in vesicular diseases diagnosis
Early confirmation of mpox and exclusion of other causes of vesicular diseases are both important in facilitating clinical assessment of suspicious cases. Evaluation of QIAStat-Dx viral vesicular panel (MPXV clades I & II, HSV1, HSV2, HHV-6, VZV, EV), a rapid 1-h multiplexed assay developed by Qiagen, was evaluated in parallel with the reference PCR at VIDRL. The assays demonstrated high level of concordance without cross-reactivity with other viruses from the Poxviridae family (vaccinia, orf, molluscum contagiosum viruses).11 This is one of the earliest studies to inform rapid assay performance and provided an option for rapid diagnosis at primary clinician testing centres. The assessment could serve as an independent review of assay performance to facilitate regulatory approvals.
Evaluation of high-throughput PCR assays
Most of the currently available molecular assays for mpox are either real-time PCR or loop mediated isothermal amplification (LAMP)-based assays, targeting conserved central coding regions (e.g. E9L_NVAR, B6R, F3L).12,13 The National High Security Quarantine Laboratory at VIDRL serves as a reference laboratory for smallpox and orthopoxvirus by performing culture, distributing inactivated control materials and evaluating the analytical performance of high-throughput molecular assay to support diagnostic laboratories. A study comparing published primers and probes from VIDRL, the US Centers for Disease Control and Prevention (CDC) and US Army Medical Research Institute of Infectious Diseases (USAMRIID) with 13 other commercial PCR kits demonstrated good concordance between PCR assays, although significant variability in limits of detection (LODs) was observed for a few assays (LODs between 57 and 14,495 copies mL–1).14 Interestingly, a few MPXV-specific assays non-specifically detected vaccinia virus. These data suggest that variabilities in assay sensitivity and specificity should be taken into consideration in testing strategies, in particular for the detection of MPXV in non-vesicular samples (e.g. urine, semen, blood), where viral loads can be low or variable. The study findings help support the development of WHO mpox laboratory testing policy.15
Application of mpox genomics for surveillance
In recent years, public health authorities have begun relying heavily on genomic surveillance to assist with epidemiological linkages. However, it is often challenging to determine the threshold for genomic linkages in emerging pathogens without prior validation with epidemiological data. Whole-genome sequencing of MPXV was performed from multiple body sites using sample cohorts collected at MSHC. The presence of different subclades of MPXV in some individuals suggested potential complex transmission chains.16 Additionally, the presence of large deletions and elevated number of mutations in the MPXV genome (due to human APOBEC3 enzyme editing activity) provides evidence that MPXV is continuously evolving and diversifying during human-to-human transmissions. The lack of geographical representative genomic data poses a challenge in using genomics for case linkages. Despite this, there are a number of public health applications in mpox genomics, for example (i) global contextualisation to determine origin of case importation,17 (ii) assessment for diagnostic and potentially therapeutic or vaccine escape 18 and (iii) excluding case or cluster linkages. Most importantly, the early genomic characterisation of local clusters continues to guide public health actions based on scientific evidence.
Cutting-edge CRISPR technology for rapid PoCTs
Despite the availability of good commercial mpox PCR assays,14 because of the lack of endemicity in Australia, few of these tests have undergone regulatory approval. For an effective control of sexually transmitted infection (STI) outbreaks, investment in capability to develop in-house diagnostic assays, especially good quality point-of-care tests (PoCTs) is necessary. Advancement in CRISPR-based diagnostics have catalysed the development of DNA- or RNA-targeting assays that display high sensitivity and specificity, high portability, rapid turnaround, and require minimal manipulation (Fig. 1). Owing to the highly programmable nature of the CRISPR system, these assays can be designed in-house, with the flexibility to be rapidly modified in the event of diagnostic escape.19–22 However, complexed computational capability is required for high-throughput primer and guide RNA design, essential for target amplification and the CRISPR assay (Fig. 1).
CRISPR Diagnostic Platforms – SHERLOCK and DETECTR. (a) Key steps in CRISPR diagnostic assay design using the pangenome approach in PathoGD. (b) Two CRISPR diagnostic systems. In the SHERLOCK system, target DNA or RNA are amplified with recombinase polymerase amplification (RPA) and RT-RPA (reverse transcription RPA) respectively. An in vitro transcription (IVT) step with T7 polymerase converts DNA into RNA for detection with Cas13. Binding of the gRNA specifically designed to complement the target sequence triggers the collateral cleavage of quenched fluorescent reporters by the Cas13 endonuclease. The reporter system can be adapted to different readouts including lateral flow assay (LFA), fluorescence, next-generation sequencing (NGS) or electrochemical detection. In the DETECTR system, a similar approach is applied using Cas12a endonuclease for the detection of DNA.
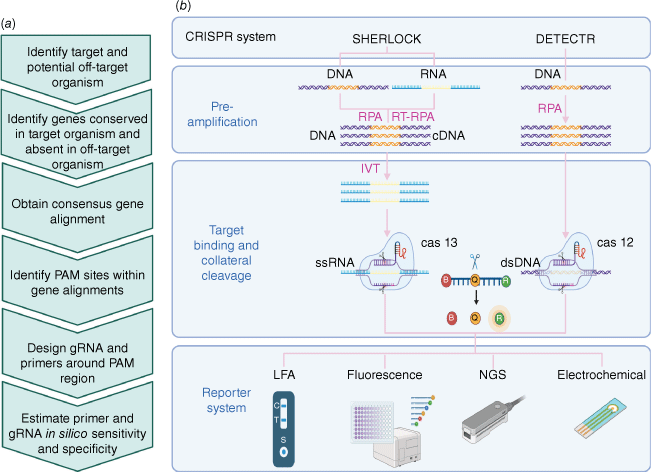
Recently, PathoGD, a bioinformatic pipeline for high-throughput recombinase polymerase amplification (RPA) primer and guide RNA design has been developed, which allows for rapid design of CRISPR–Cas12a-based diagnostic assays.23 This automated, end-to-end computational tool is a major developmental milestone, as it enables the assay to be developed in diagnostic laboratories with minimal bioinformatic capabilities.24
During the 2022 mpox outbreak, several assays incorporating CRISPR technology were developed for the rapid detection of MPXV, including one in Australia.25 This CRISPR–Cas12a based assay was the first Australian assay, designed for applications in the field (lateral flow readout) and laboratory (fluorescent readout). This assay enabled the detection of MPXV within 45 min with 100% specificity and a LOD comparable to real-time PCR (1 copy μL–1). This assay was subsequently streamlined to a portable heating instrument, allowing deployment outside of the laboratory (Fig. 2).
Summary
As mpox continues to spread in 2024, repeated importation of cases is posing a significant challenge to disease control in Australia. Reference laboratories play a significant role in early characterisation of infectivity, viral dynamics, genomics and distribution of control materials. As the outbreaks continue to evolve, in-house capability to develop innovative rapid diagnostic assays becomes important in preventing endemicity and reducing reliance on commercial assays. Investing in computational infrastructure and CRISPR diagnostics not only provides flexibility in responding to outbreaks and tracking diagnostic escape in viruses, but could also serve as a cost-effective public health strategy for controlling STIs.
Data availability
Data sharing is not applicable as no new data were generated or analysed during this study.
References
1 Brown K, Leggat PA (2016) Human monkeypox: current state of knowledge and implications for the future. Trop Med Infect Dis 1, 8.
| Crossref | Google Scholar | PubMed |
2 Liu X et al. (2022) Monkeypox claims new victims: the outbreak in men who have sex with men. Infect Dis Poverty 11, 84.
| Crossref | Google Scholar | PubMed |
3 Lim CK et al. (2023) Mpox diagnostics: review of current and emerging technologies. J Med Virol 95, e28429.
| Crossref | Google Scholar | PubMed |
4 Moschese D et al. (2022) Isolation of viable monkeypox virus from anal and urethral swabs, Italy, May to July 2022. Euro Surveill 27, 2200675.
| Crossref | Google Scholar | PubMed |
5 Antinori A et al. (2022) Epidemiological, clinical and virological characteristics of four cases of monkeypox support transmission through sexual contact, Italy, May 2022. Euro Surveill 27, 2200421.
| Crossref | Google Scholar | PubMed |
6 Adler H et al. (2022) Clinical features and management of human monkeypox: a retrospective observational study in the UK. Lancet Infect Dis 22, 1153-1162.
| Crossref | Google Scholar | PubMed |
7 Peiró-Mestres A et al. (2022) Frequent detection of monkeypox virus DNA in saliva, semen, and other clinical samples from 12 patients, Barcelona, Spain, May to June 2022. Euro Surveill 27, 2200503.
| Crossref | Google Scholar | PubMed |
8 Thornhill JP et al. (2022) Monkeypox virus infection in humans across 16 countries — April–June 2022. N Engl J Med 387, 679-691.
| Crossref | Google Scholar | PubMed |
9 Lim CK et al. (2023) Correlation between monkeypox viral load and infectious virus in clinical specimens. J Clin Virol 161, 105421.
| Crossref | Google Scholar | PubMed |
10 Towns JM et al. (2023) Persistence of monkeypox virus at oral and rectal sites. Lancet Microbe 4, e210.
| Crossref | Google Scholar | PubMed |
11 Batty M et al. (2023) Laboratory assessment of a multi-target assay for the rapid detection of viruses causing vesicular diseases. J Clin Virol 165, 105525.
| Crossref | Google Scholar | PubMed |
12 Iizuka I et al. (2009) Loop-mediated isothermal amplification-based diagnostic assay for monkeypox virus infections. J Med Virol 81, 1102-1108.
| Crossref | Google Scholar | PubMed |
13 Davi SD et al. (2019) Recombinase polymerase amplification assay for rapid detection of monkeypox virus. Diagn Microbiol Infect Dis 95, 41-45.
| Crossref | Google Scholar | PubMed |
14 Papadakis G et al. (2023) Evaluation of 16 molecular assays for the detection of orthopox and mpox viruses. J Clin Virol 161, 105424.
| Crossref | Google Scholar | PubMed |
15 WHO Surveillance Systems (2024) Diagnostic testing for the monkeypox virus (MPXV): interim guidance, 10 May 2024. Technical document. World Health Organization. https://www.who.int/publications/i/item/WHO-MPX-Laboratory-2024.1
16 Taouk ML et al. (2023) Intra‐ and interhost genomic diversity of monkeypox virus. J Med Virol 95, e29029.
| Crossref | Google Scholar | PubMed |
17 Borges V et al. (2023) Viral genetic clustering and transmission dynamics of the 2022 mpox outbreak in Portugal. Nat Med 29, 2509-2517.
| Crossref | Google Scholar | PubMed |
18 Ahmed SF et al. (2022) Vaccinia-virus-based vaccines are expected to elicit highly cross-reactive immunity to the 2022 monkeypox virus. Viruses 14, 1960.
| Crossref | Google Scholar | PubMed |
19 Cunningham CH et al. (2021) A novel CRISPR-based malaria diagnostic capable of Plasmodium detection, species differentiation, and drug-resistance genotyping. EBioMedicine 68, 103415.
| Crossref | Google Scholar | PubMed |
20 Gootenberg JS et al. (2018) Multiplexed and portable nucleic acid detection platform with Cas13, Cas12a, and Csm6. Science 360, 439-444.
| Crossref | Google Scholar | PubMed |
21 Kellner MJ et al. (2019) SHERLOCK: nucleic acid detection with CRISPR nucleases. Nat Protoc 14, 2986-3012.
| Crossref | Google Scholar | PubMed |
22 Mustafa MI, Makhawi AM (2021) SHERLOCK and DETECTR: CRISPR–Cas systems as potential rapid diagnostic tools for emerging infectious diseases. J Clin Microbiol 59(3), e00745-20.
| Crossref | Google Scholar | PubMed |
23 Low SJ et al. (2024) PathoGD: an integrative genomics approach for CRISPR-based target design of rapid pathogen diagnostics. bioRxiv 2024.05.14.593882 [Preprint, posted 15 May 2024].
| Crossref | Google Scholar |
24 Khalil A et al. (2023) The need for better diagnostics to support diagnosis and surveillance in monkeypox endemic countries. Lancet Microbe 4, e67.
| Crossref | Google Scholar | PubMed |
25 Low SJ et al. (2023) Rapid detection of monkeypox virus using a CRISPR–Cas12a mediated assay: a laboratory validation and evaluation study. Lancet Microbe 4, e800-e810.
| Crossref | Google Scholar | PubMed |
![]() Dr Chuan Kok Lim is a medical virologist at the Victorian Infectious Diseases Reference Laboratory (VIDRL) and senior research fellow at the Peter Doherty Institute for Infection and Immunity. His research focuses on advancing pandemic preparedness through novel diagnostic development and genomic characterisation of emerging viral pathogens including orthopoxvirus and flavivirus. |
![]() Dr Shivani Pasricha is a microbiologist and laboratory head in the Department of Infectious Diseases, The University of Melbourne. Using molecular and genomic approaches, her research aims to improve the detection, prevention and surveillance of sexually transmitted infections (STIs). Her current research includes developing cutting-edge CRISPR-diagnostics for the point-of-care detection of STIs and antimicrobial resistance. |
![]() Dr Soo Jen Low is a research officer and bioinformatician at the Peter Doherty Institute for Infection and Immunity. Her research focuses on the application of genomics and development of bioinformatic pipelines to support innovative diagnostic approaches. |