Emerging viral threats in Australia
Erin Harvey

A
B
C
![]() Dr Erin Harvey is a Postdoctoral Research Associate at the University of Sydney in the research group of Prof. Eddie Holmes (Virus Emergence and Evolution group). Her research focuses on using metatranscriptomic virus discovery to investigate the evolution and ecology of viruses in Australia, with a particular interest in native marsupials, parasitic invertebrates and the effects of changing land use on the viromes of native species. |
![]() Dr Charles Foster is an early career who investigates big questions in evolutionary biology, including the evolutionary timescale of flowering plants, the evolution of live birth in vertebrates, and virus evolution. Since 2020, Charles has worked as a bioinformatician within the Virology Research Laboratory (University of New South Wales). His research involves developing bioinformatics pipelines for genomic epidemiological surveillance of SARS-CoV-2, high-throughput antiviral resistance testing of human cytomegalovirus, and associations of the human virome with disease outcomes. |
Abstract
Pathogenic viruses pose significant threats to human health. Consequently, it is important to consider the mechanisms by which viruses might emerge and spread both within Australia and internationally. Australia is relatively isolated from major global population centres, reachable only by international flight or long boat journey (with the exception of the most southern and eastern parts of Indonesia). This isolation, coupled with the island nature of Australia, allows broadly effective interventions to be put in place to minimise the effect of viruses circulating internationally. However, the threats posed by virus transmission emerging from within Australia, including from novel animal reservoirs as a consequence of anthropogenic activities, warrant investigation. Here we discuss the current emerging viral threats to Australia and the likelihood of a virus emerging from a domestic reservoir. We also discuss the importance of virus discovery methods for understanding the diversity and ecology of viruses in our invasive and native wildlife populations.
Keywords: animal reservoir, infectious disease, virus discovery, virus emergence, zoonosis.
Introduction
Theoretical and technological advancements since the mid-1990s have greatly improved the ability of global public health bodies to respond to emerging pandemic threats.1 Nevertheless, the emergence of SARS-CoV-2 and subsequent global spread of the virus during the COVID-19 pandemic has highlighted how vulnerable we remain to pandemic events in the 21st Century. Our world is rapidly changing, with an unprecedented degree of global interconnectedness, and the looming threat of diseases emerging because of anthropogenic activities and their flow on effects.2 In the past decades, increasingly frequent extreme-weather events associated with climate change have often led to outbreaks of disease in their wake.3 Other hallmarks of human life in the 21st Century such as urbanisation, industrialised farming and changing land use have unmistakable effects on the ecosystems around human populations, and these equally affect the dynamics of virus evolution and emergence.2 However, not all countries are equally at risk. The likelihood of the emergence and sustained transmission of a pathogenic virus within a given region is affected by the interplay between factors such as demographics, geography, infrastructure and biodiversity. In this paper, we consider the intersection of these components to evaluate how viral threats emerge, and what the emerging viral threats to Australia might be.
Risk factors for virus introduction and transmission
Many factors contribute to the emergence of a virus in the human population, with both ecological and sociological elements being equally important.2 The majority of emerging infections identified from the mid-20th Century have been of zoonotic origin, whereby transmission occurs from an animal reservoir into humans followed by sustained human-to-human transmission.4 It is important to identify animals acting as reservoirs for viruses with pathogenic potential. For example, live-animal ‘wet’ markets have previously been identified as areas of strong concern for zoonotic pathogen spillover given that they represent an intersection between ‘exotic’ animals harbouring potentially novel pathogens and (often) a densely populated area with an immunologically naive population.5,6
The likelihood of the global spread of emerging viruses into naïve populations has increased through a high degree of global interconnectedness.2 Australia is in a unique position, being the most isolated inhabited continent in the world and reachable only by international flight or extensive journey by boat from major global population centres beyond Indonesia. These factors allow the risk of the spread of an introduced pathogenic virus to be reduced. For example, during the early stages of the COVID-19 pandemic, Australia’s isolation enabled the rapid closure of international borders. Coupled with Australia’s low-density population, this isolation from the global community enabled effective isolation of positive cases and prevented uncontrolled spread of infections across the country until the eventual relaxation of public health measures. Consequently, the trajectory of SARS-CoV-2 infections in Australia was far different from most other countries that did not have the same geographic isolation and population dynamics.7,8
The increasing human population demands greater resources and puts ecosystems under increasing strain through urbanisation and increased population density, which increases the risk of disease transmission.2 Associated habitat destruction destabilises ecosystems and hastens the rate of species extinctions. We cannot predict fully how these significant ecosystem disruptions will affect virus evolution. Viral lineages could go extinct with their hosts, or, alternatively, they could adapt to increasing evolutionary pressure and become more generalist.9,10 Industrialised farming to feed a growing population creates a dire risk for virus emergence. Genetic diversity among farm animals is often low, and the animals can be in states of poor health and high stress while in extremely dense populations. These factors are ideal for viral evolution during sustained animal-to-animal transmission. Particularly concerning examples include fur farms and farming of non-domestic species, where a number of novel and previously described viruses of concern have been identified across multiple species including outbreaks of SARS-CoV-2 and influenza in European fur farms.5,11–13 The net result of prolonged viral evolution in response to selective pressures or prolonged transmission is an increased risk of disease emergence in the human population by zoonosis.
Anthropogenic climate change has increased the frequency and severity of extreme weather events. The aftermath of these events creates an opportunity for disease emergence as infrastructure is often damaged, and the dynamics of wildlife and disease vectors shift.14,15 Of particular risk are events that lead to a lack of clean water and, in some cases, an increase in stagnant water around urban settlements, which can drive increased mosquito populations.16,17 These conditions have been witnessed in Australia over the 2020–2023 period, with increased severe flooding events coinciding with both an increase in mosquito populations and an increase in arboviral disease, including the emergence of Japanese encephalitis virus (JEV) in south-eastern Australia.18 The outbreak likely stemmed from the introduction of the virus from the Torres Strait Islands, Papua New guinea and Indonesian territories, where the outbreak genotype (IV) had previously been identified.19 A serosurvey within this period indicated that 1 in 11 people sampled across the New South Wales and Victorian border showed evidence of exposure to JEV.20 The JEV outbreak demonstrated the role that anthropogenic climate change can play in the broader chain of arbovirus transmission: the virus was most likely imported into Australia through infected migratory birds opportunistically seeking flooded areas, followed by spread into porcine amplifying hosts by the increased population of mosquito vectors.19
Despite the advantages of being isolated with a generally low population density, it is crucial that Australia is prepared for the next international pandemic event. Some key steps will include monitoring any emerging disease outbreaks globally and being prepared to act accordingly, and heeding warnings surrounding the risks associated with increased urbanisation and industrialisation.21 However, of arguably the most importance is that we understand little about the ecology and epidemiology of zoonotic viruses in Australia and the associated risk of disease emergence.
The threat of animal reservoirs in Australia
Viruses that can jump hosts and sustain transmission in these new hosts are referred to as generalist viruses. Generalists are the most likely viruses to gain the ability to infect humans and sustain transmission within the human population through frequent exposure events, likely occurring at the human–animal interface through activities such as farming and hunting. For example, SARS-CoV-2 has jumped from wildlife into humans and subsequently spilled back into several wild and domestic animal species.9,22 These viruses generally accumulate mutations as they adapt to their hosts that can lead to increased disease severity and transmissibility.23 To realise fully the risk of generalist viruses emerging within Australia, it is important to consider the species richness within the continent.
Australia has a unique assemblage of endemic flora and fauna that have evolved over many millions of years in response to both reproductive isolation and changes in climate over time.24 The incidence of emerging zoonotic diseases has a strong correlation with increased mammalian species richness.25 This is not surprising in an evolutionary context: the phylogenetic distance effect is theoretically and empirically known to affect the success of a pathogenic host switch, with a horizontal host switch more likely to occur across more closely related species.26,27 However, the threat is not restricted to mammals: zoonotic transmission is known to occur from other animal groups such as birds.28 To date, several animal groups have been identified as potential and realised sources of zoonotic disease emergence in Australia. Bats, rodents and birds are established reservoirs of viruses capable of spill over into humans such as influenza, hantaviruses, lyssaviruses and Hendra virus. Sporadic Hendra virus outbreaks have occurred at the interface of domestic species and wildlife in New South Wales and Queensland since 1994, characterised by high levels of morbidity and mortality in horses and humans.29 An outbreak of lymphocytic choriomeningitis virus was detected in New South Wales as a result of the 2021 ‘mouse plague’ experienced in the region, and it is now suspected that the virus may be widespread in the Australian rodent population, although surveillance has not yet been conducted to determine the geographic range of the virus.30 Macropods are suspected to be amplifying hosts for several arbovirus species (the informal grouping for any virus spread by an arthropod vector) in Australia (Fig. 1).31–33 Introduced pigs and chickens are also known reservoirs of influenza virus.34,35 However, there is still much to learn about the diversity and ecology of viruses in Australian wildlife, including their true range and prevalence.
Native reservoirs of zoonotic viruses in Australia, including bats, macropods and water birds, and the zoonotic viruses they are associated with. Viruses written in bold text are arboviruses vectored by mosquitoes.
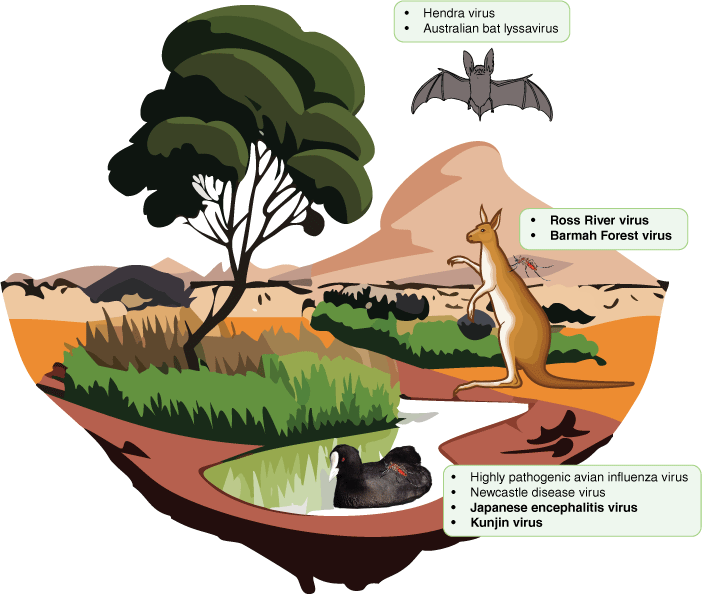
To date, metagenomic virus discovery studies have been conducted for several native and invasive species in Australia including rabbits,36 foxes,37 bats,38 dasyurids (marsupial carnivores),39 koalas40 and shorebirds,41 but expanding the number of species studied and the geographical range of these studies is needed to understand the dynamics of the Australian ‘virome’. It is particularly important to conduct unbiased metagenomic screening of domestic species in frequent contact with humans, wildlife at the human–animal interface, and common invertebrate vectors of disease (e.g. mosquitoes, ticks). The latter are known hosts of arboviruses, which represent a significant threat to Australia with over 75 arboviruses having been identified on the continent.42–44
As climate change shifts weather patterns across Australia, it will be important to better understand the geographic range of endemic and emerging arboviruses such as Dengue virus, Kunjin virus, Ross River virus (RRV) and JEV,44 and the ways in which these ranges will respond to climate change. For example, increased flooding in some regions could create conditions ideal for increased migration of viraemic birds and population expansion of arboviral vectors, but, conversely, increased aridity in other regions might actually decrease the risk of disease emergence. Additionally, viruses that already occur across extremely widespread and climatically variable geographic ranges (e.g. Barmah Forest virus, BFV; RRV) might be less affected by anticipated climate change. The dynamics of these viruses cannot be fully understood without widespread monitoring. Sentinel surveillance has been undertaken in northern Australia for decades, especially after the introduction of the Northern Australia Quarantine Strategy in 1989. For example, this monitoring in northern Australia has aimed to identify cases of Dengue virus and JEV,45,46 including through sentinel surveillance of pigs and mosquitoes.46 In other regions of Australia, surveillance programs focus more on detection of MVEV and KUNV in sentinel populations of chickens or in opportunistic sampling of mosquito vectors.47 Despite the strengths of the existing system, there is a strong need to invest further in capability building and infrastructure to expand the surveillance network as the threat of emerging diseases increases. As the genomic era gains momentum in Australia, an increased use of metagenomics-based methods for surveillance and outbreak monitoring will be of great benefit, although the scale of such a study across a landmass that is largely unpopulated is a great challenge.
Current and emerging threats to Australia
The COVID-19 pandemic most recently brought the risk and consequences of a pandemic into the public consciousness. Subsequent international outbreaks of Mpox and Ebola have also received strong media attention, however, respiratory viruses such as novel strains of influenza, or another novel coronavirus, are far more likely to cause the next global pandemic. Influenza has caused four pandemic events in the past 100 years, and influenza infections are seemingly increasing in frequency.48 The viruses’ wide host range and segmented genome leads to frequent genomic reassortment events, resulting in new strains. At present, the high pathogenicity avian influenza (HPAI) H5 virus is spreading globally through sea birds leading to mass mortality events, as well as causing spillover outbreaks in marine mammals and (occasionally) humans.49 The virus has not yet been detected in Australia and, therefore, is not considered an imminent risk to human health, but is worth monitoring. It is crucial that there is a widespread capacity for the surveillance of viral threats in Australia, with detection not hampered by inter-jurisdictional differences in laboratory or analytical protocols.50
Australia suffers from periodic outbreaks of endemic virus species, many of which are arboviruses. Arboviruses present a significant outbreak threat, particularly in tropical regions and in the aftermath of severe weather events. Of the arboviruses identified in Australia, many are endemic to the tropical northern region of the country, but others are more widespread as host and vector generalist (e.g. RRV, BFV). Outbreak can be common in regions where Australia’s population centres are located (Fig. 2).44 Alphaviruses (e.g. RRV, BFV), orthoflaviviruses (e.g. Murray Valley encephalitis virus, MVEV; Kunjin virus, KUNV)51,52 and orthobunyaviruses (e.g. Gan Gan virus)53 are all examples of arboviruses that have caused sporadic outbreaks of disease across Australia. These outbreaks are usually linked to extreme weather events that result in higher-than-normal levels of precipitation, as well as associated abnormal increases in the viral vector populations. For example, an outbreak of MVEV occurred in 2023 across Australia with cases detected in Victoria, Western Australia, Northern Territory, and the first case reported in New South Wales. This outbreak was suspected to have resulted from a pattern of extreme rainfall during the outbreak period.54 There is also ongoing concern that arboviruses that are not currently endemic in Australia, such as Zika virus, Chikungunya virus and Yellow fever virus, could emerge through widespread outbreaks following introduction by international travellers. These viruses have spread across Asia and South America from their original point of emergence as a consequence of the spread of their vector, the Aedes aegypti mosquito. Local transmission of Dengue virus has occurred several times within northern Queensland because of infected travellers returning to the region. At present, within Australia A. aegypti is only found in Queensland as an invasive species, and A. albopictus (another Dengue virus vector) is established in the Torres Strait but not on the mainland. Previous work has also suggested that other native mosquito species could possibly serve as a vector for viruses of concern (e.g. JEV).18 Continual monitoring to sample the virome of mosquito species is key to preventing the emergence of devastating arbovirus species in Australia.44
Endemic and emerging viruses in Australia. The approximate geographic ranges of these endemic viruses are shown in coloured regions, based on where they are most frequently reported in clinical cases or detected in sentinel surveillance. However, it is important to note that these viruses can, and have been, more rarely detected in other areas of Australia, such as Barmah Forest virus being detected as far south as Tasmania.58 Viruses of predicted emergence risk from neighbouring regions are indicated with a solid arrow. Viruses of predicted risk from broader international regions are indicated with a broken arrow.
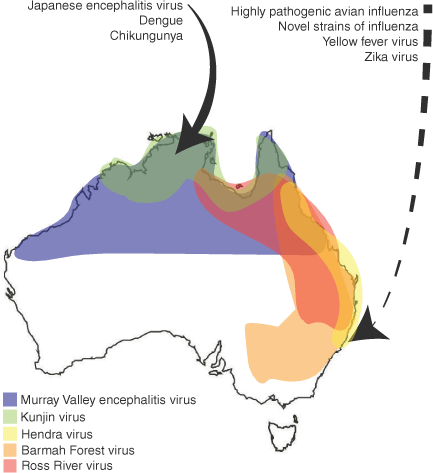
There are also other less common viral threats in Australia, including paramyxoviruses. The Hendra virus is likely endemic in the flying fox populations of eastern Australia, and results in sporadic outbreaks within horses and occasional onwards transmission from horses into humans. Additionally, recently a case of Newcastle disease virus caused fatal disease in a child in eastern Australia.55,56 Likewise, there have also been a small number of spillover events of Australian bat lyssavirus, a relative of the rabies virus, causing disease in humans.57 However, the spillover events associated with these viruses are incidental, with humans representing a ‘dead end host’.
Conclusions
The COVID-19 pandemic exposed Australia’s strengths and weaknesses in responding to a global pandemic virus emergence event. As anthropogenic activities continue to increase the risk of virus emergence, we must be prepared for the emergence of viruses in the future, both within Australia and internationally. For example, increasing extreme weather events are already expanding the range and endemicity of pathogenic arbovirus species (e.g. JEV). The main threats for future pandemics are the emergence and spread of respiratory viruses such as influenza and arboviruses. To prepare for such events, it is crucial that unbiased virus surveillance (e.g. metagenomics) programs be funded across the continent to better understand the diversity of viruses in Australia, the dynamics of virus ecology and the geographic range of viruses with potential to cause disease outbreaks in humans.
References
1 Galaz V (2009) Pandemic 2.0: can information technology help save the planet? Environ Sci Policy Sustain Dev 51, 20-28.
| Crossref | Google Scholar |
2 Weiss RA, McMichael AJ (2004) Social and environmental risk factors in the emergence of infectious diseases. Nat Med 10, S70-S76.
| Crossref | Google Scholar | PubMed |
3 Cheng J et al. (2021) Extreme weather events and dengue outbreaks in Guangzhou, China: a time-series quasi-binomial distributed lag non-linear model. Int J Biometeorol 65, 1033-1042.
| Crossref | Google Scholar | PubMed |
4 Weiss RA, Sankaran N (2022) Emergence of epidemic diseases: zoonoses and other origins. Fac Rev 11, 2.
| Crossref | Google Scholar | PubMed |
5 He W-T et al. (2022) Virome characterization of game animals in China reveals a spectrum of emerging pathogens. Cell 185, 1117-1129.e1118.
| Crossref | Google Scholar | PubMed |
6 Webster RG (2004) Wet markets—a continuing source of severe acute respiratory syndrome and influenza? Lancet 363, 234-236.
| Crossref | Google Scholar | PubMed |
7 Stobart A, Duckett S (2022) Australia’s response to COVID-19. Health Econ Policy Law 17, 95-106.
| Crossref | Google Scholar | PubMed |
8 Foster CSP et al. (2023) Persistence of a frameshifting deletion in SARS-CoV-2 ORF7a for the duration of a major outbreak. Viruses 15, 522.
| Crossref | Google Scholar | PubMed |
9 Petrone ME et al. (2023) Through an ecological lens. EMBO Rep 24, e56578.
| Crossref | Google Scholar | PubMed |
10 Harvey E, Holmes EC (2022) Diversity and evolution of the animal virome. Nat Rev Microbiol 20, 321-334.
| Crossref | Google Scholar | PubMed |
11 Warwick C et al. (2023) One health implications of fur farming. Front Anim Sci 4, 1249901.
| Crossref | Google Scholar |
12 Wasniewski M et al. (2023) Investigations into SARS‐CoV‐2 and other coronaviruses on mink farms in France late in the first year of the COVID‐19 pandemic. PLoS ONE 18, e0290444.
| Crossref | Google Scholar | PubMed |
13 Lindh E et al. (2023) Highly pathogenic avian influenza A (H5N1) virus infection on multiple fur farms in the south and central Ostrobothnia regions of Finland, July 2023. Euro Surveill 28, pii=2300400.
| Crossref | Google Scholar | PubMed |
14 Beşli Y, Sancak B (2023) Gastrointestinal infections after earthquake. Microbiol Aust 44(4), 193-196.
| Crossref | Google Scholar |
15 Yıldız Zeyrek F et al. (2023) Vector-borne parasitic infections after the earthquake. Microbiol Aust 44(4), 197-201.
| Crossref | Google Scholar |
16 Singh SP (2023) Flooding adversely affects fresh produce safety. Microbiol Aust 44(4), 185-189.
| Crossref | Google Scholar |
17 Blaskovich MAT, Harris PNA (2023) Bugs in floods. Microbiol Aust 44(4), 176-180.
| Crossref | Google Scholar |
18 van den Hurk AF et al. (2022) The emergence of Japanese encephalitis virus in Australia in 2022: existing knowledge of mosquito vectors. Viruses 14, 1208.
| Crossref | Google Scholar | PubMed |
19 McGuinness SL et al. (2023) The evolving Japanese encephalitis situation in Australia and implications for travel medicine. J Travel Med 30, taad029.
| Crossref | Google Scholar | PubMed |
20 Furlong M et al. (2023) Japanese encephalitis enzootic and epidemic risks across Australia. Viruses 15, 450.
| Crossref | Google Scholar | PubMed |
21 Holmes EC et al. (2018) Pandemics: spend on surveillance, not prediction. Nature 558, 180-182.
| Crossref | Google Scholar |
22 Feng A et al. (2023) Transmission of SARS-CoV-2 in free-ranging white-tailed deer in the United States. Nat Commun 14, 4078.
| Crossref | Google Scholar | PubMed |
23 Foster CS et al. (2023) Long-term serial passaging of SARS-CoV-2 reveals signatures of convergent evolution. bioRxiv 2023.11.02.565396 [Preprint, published 6 November 2023].
| Crossref | Google Scholar |
24 Renner MAM et al. (2020) Increased diversification rates are coupled with higher rates of climate space exploration in Australian Acacia (Caesalpinioideae). New Phytol 226, 609-622.
| Crossref | Google Scholar | PubMed |
25 Allen T et al. (2017) Global hotspots and correlates of emerging zoonotic diseases. Nat Commun 8, 1124.
| Crossref | Google Scholar | PubMed |
26 Foster CSP (2019) Digest: the phylogenetic distance effect: understanding parasite host switching. Evolution 73, 1494-1495.
| Crossref | Google Scholar | PubMed |
27 Engelstädter J, Fortuna NZ (2019) The dynamics of preferential host switching: host phylogeny as a key predictor of parasite distribution. Evolution 73, 1330-1340.
| Crossref | Google Scholar | PubMed |
28 Reed KD et al. (2003) Birds, migration and emerging zoonoses: West Nile virus, Lyme disease, influenza A and enteropathogens. Clin Med Res 1, 5-12.
| Crossref | Google Scholar | PubMed |
29 Tulsiani SM et al. (2011) Emerging tropical diseases in Australia. Part 5. Hendra virus. Ann Trop Med Parasitol 105, 1-11.
| Crossref | Google Scholar | PubMed |
30 Caly L et al. (2022) Lymphocytic choriomeningitis virus infection, Australia. Emerg Infect Dis 28, 1713-1715.
| Crossref | Google Scholar | PubMed |
31 Tong S (2004) Ross River virus disease in Australia: epidemiology, socioecology and public health response. Intern Med J 34, 58-60.
| Crossref | Google Scholar | PubMed |
32 Moore PR et al. (2010) Emerging tropical diseases in Australia. Part 3. Australian bat lyssavirus. Ann Trop Med Parasitol 104, 613-621.
| Crossref | Google Scholar | PubMed |
33 Holmes EC, Zhang Y-Z (2015) The evolution and emergence of hantaviruses. Curr Opin Virol 10, 27-33.
| Crossref | Google Scholar | PubMed |
34 Deng YM et al. (2020) Locally acquired human infection with swine-origin influenza A (H3N2) variant virus, Australia, 2018. Emerg Infect Dis 26, 143-147.
| Crossref | Google Scholar | PubMed |
35 Scott A et al. (2020) An overview of avian influenza in the context of the Australian commercial poultry industry. One Health 10, 100139.
| Crossref | Google Scholar | PubMed |
36 Mahar JE et al. (2020) Comparative analysis of RNA virome composition in rabbits and associated ectoparasites. J Virol 94, 10.1128/jvi.02119-02119.
| Crossref | Google Scholar |
37 Campbell SJ et al. (2020) Red fox viromes in urban and rural landscapes. Virus Evol 6, veaa065.
| Crossref | Google Scholar | PubMed |
38 Van Brussel K et al. (2022) Faecal virome of the Australian grey-headed flying fox from urban/suburban environments contains novel coronaviruses, retroviruses and sapoviruses. Virology 576, 42-51.
| Crossref | Google Scholar | PubMed |
39 Harvey E et al. (2023) Divergent hepaciviruses, delta-like viruses and a chu-like virus in Australian marsupial carnivores (dasyurids). Virus Evol 9, vead061.
| Crossref | Google Scholar | PubMed |
40 Harvey E et al. (2019) Identification of a novel picorna-like virus, burpengary virus, that is negatively associated with chlamydial disease in the koala. Viruses 11, 211.
| Crossref | Google Scholar | PubMed |
41 Wille M et al. (2019) Virome heterogeneity and connectivity in waterfowl and shorebird communities. ISME J 13, 2603-2616.
| Crossref | Google Scholar | PubMed |
42 Chandra S et al. (2021) Unbiased characterization of the microbiome and virome of questing ticks. Front Microbiol 12, 627327.
| Crossref | Google Scholar | PubMed |
43 Batovska J et al. (2020) Coding-complete genome sequence of Yada Yada virus, a novel alphavirus detected in Australian mosquitoes. Microbiol Resour Announc 9, 10.1128/mra.01476-01419.
| Crossref | Google Scholar |
44 Madzokere ET et al. (2020) Integrating statistical and mechanistic approaches with biotic and environmental variables improves model predictions of the impact of climate and land-use changes on future mosquito-vector abundance, diversity and distributions in Australia. Parasit Vectors 13, 484.
| Crossref | Google Scholar | PubMed |
45 Sohail A et al. (2024) The epidemiology of imported and locally acquired dengue in Australia, 2012–2022. J Travel Med 31, taae014.
| Crossref | Google Scholar | PubMed |
46 van den Hurk AF et al. (2019) Japanese encephalitis virus in Australia: from known known to known unknown. Trop Med Infect Dis 4, 38.
| Crossref | Google Scholar | PubMed |
47 Mackenzie JS et al. (1992) Australian encephalitis: sentinel chicken surveillance programme. Commun Dis Intell 16, 55-57.
| Google Scholar |
48 Harrington WN et al. (2021) The evolution and future of influenza pandemic preparedness. Exp Mol Med 53, 737-749.
| Crossref | Google Scholar | PubMed |
49 Wille M, Klaassen M (2023) No evidence for HPAI H5N1 2.3.4.4b incursion into Australia in 2022. Influenza Other Respir Viruses 17, e13118.
| Crossref | Google Scholar | PubMed |
50 Foster CSP et al. (2022) Assessment of inter-laboratory differences in SARS-CoV-2 consensus genome assemblies between public health laboratories in Australia. Viruses 14, 185.
| Crossref | Google Scholar | PubMed |
51 McGuinness SL et al. (2023) Co-circulation of Murray Valley encephalitis virus and Japanese encephalitis virus in south-eastern Australia. J Travel Med 30, taad059.
| Crossref | Google Scholar | PubMed |
52 Frost MJ et al. (2012) Characterization of virulent West Nile virus Kunjin strain, Australia, 2011. Emerg Infect Dis 18, 792-800.
| Crossref | Google Scholar | PubMed |
53 Briese T et al. (2016) Analysis of arbovirus isolates from Australia identifies novel bunyaviruses including a Mapputta group virus from Western Australia that links Gan Gan and Maprik viruses. PLoS ONE 11, e0164868.
| Crossref | Google Scholar | PubMed |
54 Quigley A, Honeyman D (2023) 2023 Murray Valley encephalitis outbreak in Australia. Global Biosecur 5,.
| Crossref | Google Scholar |
55 Mahalingam S et al. (2012) Hendra virus: an emerging paramyxovirus in Australia. Lancet Infect Dis 12, 799-807.
| Crossref | Google Scholar | PubMed |
56 Hurley S et al. (2023) Fatal human neurologic infection caused by pigeon avian paramyxovirus-1, Australia. Emerg Infect Dis 29, 2482-2487.
| Crossref | Google Scholar | PubMed |
57 Francis JR et al. (2014) Australian bat lyssavirus: implications for public health. Med J Aust 201, 647-649.
| Crossref | Google Scholar | PubMed |
58 Dyke H et al. (2023) The first confirmed outbreak of Barmah Forest virus in Tasmania – 2019. Aust NZ J Public Health 47(2), 100039.
| Crossref | Google Scholar | PubMed |
![]() Dr Erin Harvey is a Postdoctoral Research Associate at the University of Sydney in the research group of Prof. Eddie Holmes (Virus Emergence and Evolution group). Her research focuses on using metatranscriptomic virus discovery to investigate the evolution and ecology of viruses in Australia, with a particular interest in native marsupials, parasitic invertebrates and the effects of changing land use on the viromes of native species. |
![]() Dr Charles Foster is an early career who investigates big questions in evolutionary biology, including the evolutionary timescale of flowering plants, the evolution of live birth in vertebrates, and virus evolution. Since 2020, Charles has worked as a bioinformatician within the Virology Research Laboratory (University of New South Wales). His research involves developing bioinformatics pipelines for genomic epidemiological surveillance of SARS-CoV-2, high-throughput antiviral resistance testing of human cytomegalovirus, and associations of the human virome with disease outcomes. |