Wastewater-based SARS-CoV-2 surveillance and sequencing
Alice Michie A *A
![]() Dr Alice Michie is a postdoctoral scientist at New South Wales Health Pathology. She currently performs whole genome sequencing of SARS-CoV-2 of NSW wastewater samples from sentinel sites. |
Abstract
Though most often associated with poliovirus surveillance, wastewater-based surveillance (WBS) can be employed for viruses shed in human excreta (faeces, urine, skin, sputum, blood) that may enter the wastewater system, including SARS-CoV-2. WBS has been widely adopted during the COVID-19 pandemic, to complement clinical surveillance in monitoring community burden and implementing timely public health interventions. As wastewater is a non-biased, composite sample, it can provide population-level health information in near real-time, in a cost-effective manner compared to similarly scaled clinical surveillance. In many instances, data gathered from wastewater, including viral loads (quantified by RT-qPCR) and variant detections (determined through partial or whole genome sequencing), have been predictive of what is observed eventually in clinical cases. Newly emergent lineages, including the recent BA.2.86 variant, can and have been detected in wastewater samples prior to their detection in clinical specimens. There remain many challenges to wastewater genomic analysis including the presence of RT-qPCR inhibitors, degraded nucleic acid and the lack of consistent or standardised methodology between reporting labs. The wide adoption of WBS practices provides an excellent opportunity to expand this method for surveillance of other pathogens of public health importance. Herein, a broad overview of the WBS field will be provided including discussion on its advantages and applications, challenges, and how it is being utilised to characterise circulating SARS-CoV-2 lineages through sequencing.
Keywords: COVID-19, next generation sequencing, public health, sewage, viral pathogens, virus surveillance, wastewater-based epidemiology.
Analysis of wastewater for the surveillance of human pathogens, such as poliovirus, has been implemented and refined over the past several decades. Wastewater-based surveillance (WBS) can broadly involve the analysis of sewage for pathogen nucleic acid and other biomarkers that are excreted in human waste to provide near-real time population-level health information. Historically, WBS has been used not only for enteric virus surveillance, but also for the monitoring of community illicit drug use,1 alcohol and tobacco use,2,3 and for the monitoring of antimicrobial resistance genes.4
A spectrum of viruses may enter the wastewater system through sputum, skin, blood, faecal and urinary secretions including respiratory viruses such as influenza virus5 and respiratory syncytial virus,6 blood-borne viruses and vector-borne viruses.7 The utility of wastewater as a surveillance tool has been widely and rapidly adopted with the emergence of severe acute respiratory syndrome 2 virus (SARS-CoV-2) in 2020, as a means to mitigate community spread, monitor prevalence and more recently, to characterise circulating lineages. A simple overview of the wastewater surveillance process is presented in Fig. 1.
A broad overview of the wastewater surveillance pipeline for SARS-CoV-2. Virus shed from human secretions such as faeces, urine, sputum and blood may be captured in domestic wastewater. Wastewater from domestic sites is transported to wastewater treatment plants, where it may be collected for analysis. The method and frequency of collection can vary, and may involve a singular grab sample, or a composite sample collected at intervals over a defined period. Collected samples are transported to a lab for SARS-CoV-2 concentration and RNA extraction. RT-qPCR for SARS-CoV-2 RNA quantification may then be performed, as well as whole genome sequencing, either through direct sequencing (e.g. amplicon-based sequencing) or through a metagenomic approach. Trends in viral load and estimated relative lineage abundances observed in wastewater samples can then be reported to the relevant government body to inform public health interventions.
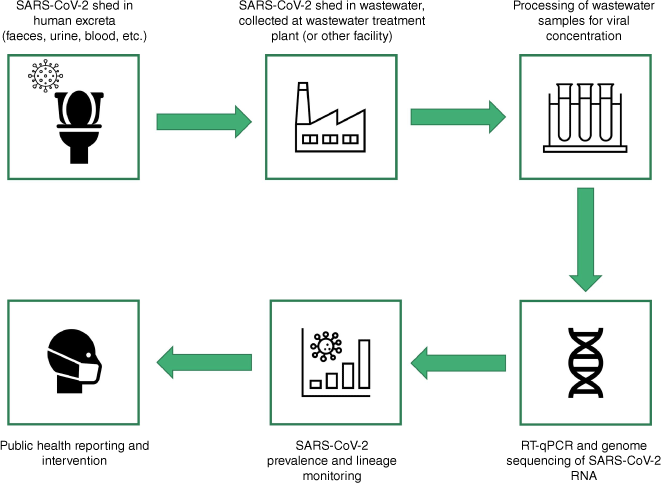
Wastewater-based surveillance for SARS-CoV-2
It has been almost 4 years since the declaration of the Coronavirus disease 2019 (COVID-19; caused by infection with SARS-CoV-2) pandemic in March 2020.
During the early stages of the COVID-19 pandemic, it was established that SARS-CoV-2 RNA was detectable and quantifiable in the faeces8,9 and urine10 of infected individuals. The ability to detect and quantify SARS-CoV-2 RNA in municipal wastewater was also established in multiple countries in the early months of 2020 including Australia,11 USA,12 Netherlands13 and Japan.14 In the Netherlands, for example, RNA targets were detected in the wastewater in the city of Amersfoort 6 days prior to the first clinical case detection.13
SARS-CoV-2 RNA detectable in wastewater may be shed from symptomatic and post-symptomatic individuals as well as the asymptomatic and pre-symptomatic.15 Information from the latter would ordinarily be underreported through traditional clinical case surveillance, making wastewater surveillance an effective complement to clinical detections.
The composite nature of wastewater provides the opportunity to obtain SARS-CoV-2 data at a community level, in a more cost-effective manner compared to similarly scaled clinical testing. The capture of virus shed from the asymptomatic and pre-symptomatic often allows for the foreshadowing of case trends and outbreaks prior to observation by clinical surveillance alone.16 In Montpellier, France, for example, a post-lockdown surge in cases in late June 2020 occurred ~2–3 weeks after an observed increase in viral RNA levels in wastewater.17 Wastewater is a non-biased sample that captures virus shed by anyone in a community connected to a sewage system, independent of healthcare seeking behaviour and access to clinical testing. In resource-poor settings and countries that have limited infrastructure for large-scale clinical testing, WBS is an especially useful strategy for understanding burden and prevalence.18 WBS may be applied at the level of individual, high risk institutions including university campuses, hospitals, cruise ships, aged-care facilities, school, airports, prisons and populations with limited healthcare access.19–23
Throughout the pandemic, WBS has been used in complement to traditional surveillance methods, to address the public health priorities of the time. Early in the pandemic, when many jurisdictions were aiming for zero community transmission, WBS focused on viral RNA detection and RT-qPCR quantification to alert of new cases in a community and to monitor trends, which were often predictive of clinical case data.16 Information from WBS was used to guide public health interventions, usually in the form of social restrictions. As community transmission initially became more widespread, there remained strong community willingness to present for nucleic acid based testing and strong government incentive to monitor case numbers.24 During this phase, WBS remained focused on RT-qPCR quantification to monitor case burden, but also shifted to include lineage characterisation through partial or whole genome SARS-CoV-2 sequencing. This was a complement to the extensively available clinical sequence data and was used to monitor trends and incursions of lineages of concern. As at-home rapid antigen self-testing (RATs) became more widely adopted, there was less clinical lineage data made available. With issues regarding RAT sensitivity and their correct use and interpretation, as well as decreased willingness to perform and report testing, there was a progressive reduction in the understanding of true community disease burden.24 During this latter phase of the pandemic, shifting towards a state of endemicity with high community vaccination rates, there has been greater reliance on wastewater data to supplement missing clinical data, including information on circulating SARS-CoV-2 lineages and their estimated relative abundance. Today, routine SARS-CoV-2 wastewater monitoring has been adopted in many jurisdictions in over 50 countries, including in most states and territories of Australia.24
Challenges of wastewater-based surveillance
Wastewater is an innately complex matrix. Not only does municipal wastewater contain waste from household settings, it also includes waste from industrial, agricultural and retail sources. As such, sewage can contain many substrates inhibitory to RT-qPCR including detergents, metal ions, polysaccharides, RNases and is subject to temperature fluctuations.25 Nucleic acid is often fragmented in wastewater, making sequencing efforts challenging.26 There is great variability between samples collected at a single site (e.g. turbidity and presence of inhibitors), and more variability between samples from different sites.27
Additionally, despite the global expansion of WBS, there is no single, standardised and accredited method for the concentration, quantification and sequencing of viral RNA from treated or untreated wastewater.25 There are many steps involved in wastewater processing, with many deviations at each. Common methods employed for virus concentration include polyethylene glycol (PEG) precipitation, ultrafiltration, ultracentrifugation and adsorption-extraction.7 When designing a wastewater surveillance program, there needs to be consideration of the sampling method, frequency, collection volume and the target catchment to capture a representative population of appropriate size.25 Consideration for the fraction of wastewater (i.e. the solid or aqueous phase) to be tested is also required, based on the partitioning behaviour of the target viruses. Generally, enveloped viruses are sequestered in the solid phase, whereas non-enveloped viruses are poorly adsorbed to solids.28 Although there have been efforts to review and compare wastewater processing strategies, there remains a lack of harmony in the methods used between jurisdictions, which may affect how we interpret data and how it can be shared and utilised.27
SARS-CoV-2 lineage surveillance through wastewater sequencing
Throughout the COVID-19 pandemic, many SARS-CoV-2 variants have emerged, including variants of concern (VOCs) and interest (VOIs), which have been important to identify and monitor. Genomic surveillance through the sequencing of SARS-CoV-2 RNA from wastewater samples has allowed cost-effective elucidation of variant distribution and spread, and early emergent lineage detection.29
Sequencing from wastewater poses several challenges. PCR inhibitors present in wastewater, as well as fragmented template makes it challenging to derive good quality sequence data of satisfactory coverage.25 Perhaps the most challenging aspect of wastewater sequencing is data interpretation, and delineating variant abundance from a sample that would contain various lineages shed from multiple infected individuals.29 Most standard tools and programs for SARS-CoV-2 lineage designation were devised for typing of a single, clinical infection, rather than a mixed environmental sample. The Freyja tool has become one of the most widely adopted methods for delineating the relative abundance of lineages within a wastewater sample.29 It uses a library of lineage-defining mutational barcodes of data from the global phylogenetic tree UShER (ultrafast sample placement on existing trees) to derive relative lineage abundances.29 An example Freyja analysis output is presented in Fig. 2, presenting the relative abundance estimates of major parental lineage groups detected in two wastewater samples collected 2 weeks apart from the same wastewater treatment plant in Sydney, Australia, between October and November 2023.
An example plot that may be generated with the output of a Freyja analysis on wastewater data. The figure shows the estimated relative abundance of two samples (A and B) collected from the same sampling site in Sydney, Australia. Sample A was collected in mid-October 2023, and sample B was collected in early November 2023, ~2 weeks apart. The estimated relative abundance (as a proportion of 1.0) of summarised ‘parental’ lineage groups is presented, as per the colouring in the key. Comparing the results of the two sites, a change in lineage abundance can be observed, particularly for lineage groups XBB.1.9.2* and BA.2.86*. The ‘Other’ lineage group in these examples includes non-XBB* and non-XBC* recombinant lineages.
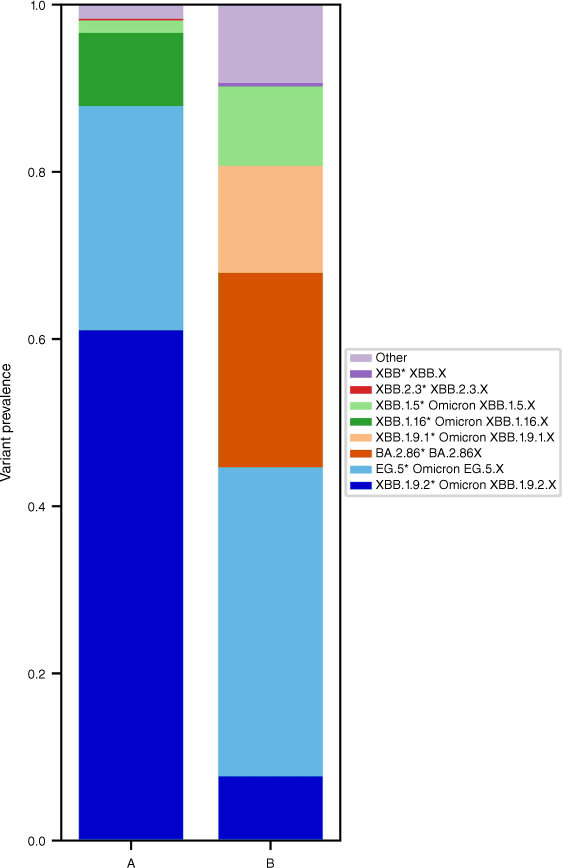
SARS-CoV-2 wastewater sequencing (including partial and whole genome sequencing) has been used to successfully detect and track emergent lineages, occasionally prior to their detection in clinical cases in that community or institution.29,30 The recently emerged BA.2.86 variant, for example, was detected in wastewater 1 week prior to detection in clinical sampling, in the Stockholm region,31 whereas early detections of Omicron (B.1.1.529) in wastewater were made in several American localities.30,32 The application of viral metagenomics to wastewater provides an opportunity for the identification and monitoring of known and unknown pathogens, and, in past studies, has identified a diversity of viral families including Coronaviridae, Flaviviridae, Poxviridae, Adenoviridae, Herpesviridae and Retroviridae.7
Metagenomics is an appealing strategy to derive abundant information from a single test, though is challenged by the high magnitude of background bacterial and fungal sequence data, and challenges in deriving high quality viral sequence data.7
Conclusions
Wastewater surveillance provides a cost-effective, non-biased, non-invasive surveillance tool that can complement traditional clinical surveillance practices, and potentially provide forewarning of imminent outbreaks and introduction of new variants into a population.
Wastewater surveillance for SARS-CoV-2 has been an invaluable tool over the many phases of the COVID-19 pandemic for informing public health interventions and stemming community spread. In the current phase of the pandemic, where we have shifted towards endemicity with massively reduced clinical nucleic acid-based testing, wastewater has become an essential tool for understanding the diversity of lineages that are circulating in the community, and their dynamics. The wide-spread adoption of wastewater surveillance practices in more labs than ever before, has established an infrastructure that can readily incorporate the surveillance of other pathogens of public health importance.
Acknowledgements
The author acknowledges SydneyWater for their contribution to wastewater surveillance in NSW, as well as Dr Charles Foster for his assistance in data analysis.
References
1 Gonzalez R et al. (2020) COVID-19 surveillance in southeastern Virginia using wastewater-based epidemiology. Water Res 186, 116296.
| Crossref | Google Scholar | PubMed |
2 Ryu Y et al. (2016) Comparative measurement and quantitative risk assessment of alcohol consumption through wastewater-based epidemiology: an international study in 20 cities. Sci Total Environ 565, 977-983.
| Crossref | Google Scholar | PubMed |
3 Zheng Q et al. (2020) Long-term trends in tobacco use assessed by wastewater-based epidemiology and its relationship with consumption of nicotine containing products. Environ Int 145, 106088.
| Crossref | Google Scholar | PubMed |
4 Hendriksen RS et al. (2019) Global monitoring of antimicrobial resistance based on metagenomics analyses of urban sewage. Nat Commun 10, 1124.
| Crossref | Google Scholar | PubMed |
5 Wolfe MK et al. (2022) Wastewater-based detection of two influenza outbreaks. Environ Sci Technol Lett 9, 687-692.
| Crossref | Google Scholar |
6 Hughes B et al. (2022) Respiratory syncytial virus (RSV) RNA in wastewater settled solids reflects RSV clinical positivity rates. Environ Sci Technol Lett 9, 173-178.
| Crossref | Google Scholar |
7 McCall C et al. (2020) Identification of multiple potential viral diseases in a large urban center using wastewater surveillance. Water Res 184, 116160.
| Crossref | Google Scholar | PubMed |
8 Wang W et al. (2020) Detection of SARS-CoV-2 in different types of clinical specimens. JAMA 323, 1843-1844.
| Crossref | Google Scholar | PubMed |
9 Xiao F et al. (2020) Infectious SARS-CoV-2 in feces of patient with severe COVID-19. Emerg Infect Dis 26, 1920-1922.
| Crossref | Google Scholar | PubMed |
10 Brönimann S et al. (2020) Secretion of severe acute respiratory syndrome coronavirus 2 in urine. Curr Opin Urol 30, 735-739.
| Crossref | Google Scholar | PubMed |
11 Ahmed W et al. (2020) First confirmed detection of SARS-CoV-2 in untreated wastewater in Australia: a proof of concept for the wastewater surveillance of COVID-19 in the community. Sci Total Environ 728, 138764.
| Crossref | Google Scholar | PubMed |
12 Sherchan SP et al. (2020) First detection of SARS-CoV-2 RNA in wastewater in North America: a study in Louisiana, USA. Sci Total Environ 743, 140621.
| Crossref | Google Scholar | PubMed |
13 Medema G et al. (2020) Presence of SARS-Coronavirus-2 RNA in sewage and correlation with reported COVID-19 prevalence in the early stage of the epidemic in the Netherlands. Environ Sci Technol Lett 7, 511-516.
| Crossref | Google Scholar | PubMed |
14 Haramoto E et al. (2020) First environmental surveillance for the presence of SARS-CoV-2 RNA in wastewater and river water in Japan. Sci Total Environ 737, 140405.
| Crossref | Google Scholar | PubMed |
15 Chen Y et al. (2020) The presence of SARS-CoV-2 RNA in the feces of COVID-19 patients. J Med Virol 92, 833-840.
| Crossref | Google Scholar | PubMed |
16 Shah S et al. (2022) Wastewater surveillance to infer COVID-19 transmission: a systematic review. Sci Total Environ 804, 150060.
| Crossref | Google Scholar | PubMed |
17 Trottier J et al. (2020) Post-lockdown detection of SARS-CoV-2 RNA in the wastewater of Montpellier, France. One Health 10, 100157.
| Crossref | Google Scholar | PubMed |
18 Street R et al. (2020) Wastewater surveillance for Covid-19: an African perspective. Sci Total Environ 743, 140719.
| Crossref | Google Scholar | PubMed |
19 Gibas C et al. (2021) Implementing building-level SARS-CoV-2 wastewater surveillance on a university campus. Sci Total Environ 782, 146749.
| Crossref | Google Scholar | PubMed |
20 Daughton CG (2020) Wastewater surveillance for population-wide Covid-19: the present and future. Sci Total Environ 736, 139631.
| Crossref | Google Scholar | PubMed |
21 Agrawal S et al. (2022) Genome sequencing of wastewater confirms the arrival of the SARS-CoV-2 Omicron variant at Frankfurt airport but limited spread in the city of Frankfurt, Germany, in November 2021. Microbiol Resour Announc 11, e01229-21.
| Crossref | Google Scholar | PubMed |
22 Ahmed W et al. (2020) Detection of SARS-CoV-2 RNA in commercial passenger aircraft and cruise ship wastewater: a surveillance tool for assessing the presence of COVID-19 infected travellers. J Travel Med 27, taaa116.
| Crossref | Google Scholar | PubMed |
23 Hassard F et al. (2022) Wastewater surveillance for rapid identification of infectious diseases in prisons. Lancet Microbe 3, e556-e557.
| Crossref | Google Scholar | PubMed |
24 Wu F et al. (2022) Making waves: wastewater surveillance of SARS-CoV-2 in an endemic future. Water Res 219, 118535.
| Crossref | Google Scholar | PubMed |
25 Ahmed W et al. (2022) Minimizing errors in RT-PCR detection and quantification of SARS-CoV-2 RNA for wastewater surveillance. Sci Total Environ 805, 149877.
| Crossref | Google Scholar | PubMed |
26 Child HT et al. (2023) Comparison of metagenomic and targeted methods for sequencing human pathogenic viruses from wastewater. mBio 14, e01468-23.
| Crossref | Google Scholar | PubMed |
27 Ahmed W et al. (2020) Surveillance of SARS-CoV-2 RNA in wastewater: methods optimisation and quality control are crucial for generating reliable public health information. Curr Opin Environ Sci Health 17, 82-93.
| Crossref | Google Scholar | PubMed |
28 Ye Y et al. (2016) Survivability, partitioning, and recovery of enveloped viruses in untreated municipal wastewater. Environ Sci Technol 50, 5077-5085.
| Crossref | Google Scholar | PubMed |
29 Karthikeyan S et al. (2022) Wastewater sequencing reveals early cryptic SARS-CoV-2 variant transmission. Nature 609, 101-108.
| Crossref | Google Scholar | PubMed |
30 Gupta P et al. (2023) Wastewater genomic surveillance captures early detection of Omicron in Utah. Microbiol Spectr 11, e00391-23.
| Crossref | Google Scholar | PubMed |
31 Espinosa-Gongora C et al. (2023) Early detection of the emerging SARS-CoV-2 BA.2.86 lineage through integrated genomic surveillance of wastewater and COVID-19 cases in Sweden, weeks 31 to 38 2023. Euro Surveill 28, pii=2300595.
| Crossref | Google Scholar | PubMed |
32 Kirby AE et al. (2022) Notes from the field: early evidence of the SARS-CoV-2 B.1.1.529 (Omicron) variant in community wastewater — United States, November–December 2021. MMWR Morb Mortal Wkly Rep 71, 103-105.
| Crossref | Google Scholar | PubMed |