Evolutionary history of Neotropical butterflies of the infratribe Neosatyriti based on target enrichment (Lepidoptera: Nymphalidae, Satyrinae, Satyrini, Pronophilina)
Tomasz W. Pyrcz A , Oscar Mahecha-J.






A
B
C
D
E
F
G
H
I
Abstract
The infratribe Neosatyriti is a section of the entirely Neotropical subtribe Pronophilina, accounting for 57 species distributed from southern Patagonia to the Andes of northern Peru, and along the Atlantic coast, with the highest diversity in central Chile. They are found in two main types of habitats, i.e. puna, pampas and subantarctic grasslands, and Valdivian forests and Chilean matorral, from sea level to nearly 5000 m above sea level. We propose a phylogenetic hypothesis of the infratribe based on molecular data obtained by target enrichment (TE) of 621 nuclear loci, totalling 248,373 base pairs, from 53 species of this infratribe and 12 outgroups. Our analysis confirms that Neosatyriti is monophyletic with full support. Based on these results, we propose eight new combinations and two status reinstatements. Molecular data are congruent with morphological characters except for Homoeonympha which appears to be paraphyletic, thus almost all the genera described originally by L. Herrera, K. Hayward and W. Heimlich in the Twentieth century based only on morphological characters are confirmed as valid, except for Haywardella that is synonymised with Pampasatyrus. Neosatyriti diverged from other Pronophilina some 23 Ma and split into two major lineages some 20 Ma. Our reconstructions do not indicate a single area of origin, rather a multisource origin, but they suggest the Neosatyriti originated in the lowlands, and that their ancestral plants were non-bambusoid grasses. Major divergence of the infratribe started some 12–11 Ma when it split into the ‘Neomaenas clade’ (9 genera), the ‘Pampasatyrus clade’ (4 genera) and the ‘Neosatyrus clade’ (5 genera). The next main radiation took place some 7–5 Ma with the switch to bamboo host plants and the colonisation of Valdivian forests. The final dispersal of the tribe was associated with the colonisation of Mata Atlantica some 6–5 Ma, and high Andean puna in Peru in the Early Pleistocene.
ZooBank: urn:lsid:zoobank.org:pub:29A961D1-99EF-465C-87E7-FB5B3570E3AE
Keywords: Andes, biogeography, coevolution, host-plants, new combination, reinstated genus, systematics, taxonomy, Valdivian forests.
Introduction
Molecular techniques, such as target enrichment, have become increasingly standard in phylogenetic studies over recent years (Espeland et al. 2018, 2019; Mayer et al. 2021; Zhang et al. 2021; Pyrcz et al. 2022). These methods provide new insights into the relationships of organisms across various systematic levels, particularly for diverse taxa comprising numerous morphologically similar and closely related species. Such groups often cannot be adequately studied using only morphological or other traditional characters (Fišer et al. 2018; Williams et al. 2022). Target enrichment is especially useful for obtaining high-quality DNA sequences from old museum specimens, addressing one of the major limitations of molecular phylogenetics reliant on Sanger sequencing (Call et al. 2021; Mayer et al. 2021).
However, robust alpha taxonomy remains the foundation of any phylogenetic analysis, continuing to rely heavily on comprehensive non-genetic data, particularly morphology. Although COI barcoding has proven efficient in this domain (Silva-Brandão et al. 2009; Marín et al. 2021), it is not without limitations (e.g. Chac and Thinh 2023; Dietz et al. 2023; Khan et al. 2024). In Lepidoptera systematics, genital morphology, an organ directly involved in sexual selection, provides critical information on alpha taxonomy and infrageneric relationships, especially when other characters are overly conservative or, conversely, too variable (Pyrcz 2010; Sachanowicz 2013; Seraphim et al. 2014; Zubek et al. 2015).
Within this context, the butterfly infratribe Neosatyriti (sensu Matz and Brower 2016) represents a particularly challenging taxon meriting a comprehensive phylogenetic analysis using high-throughput sequencing techniques. Its genus-level taxonomy remains highly unstable, with between 10 and 19 genera recognised by different authors (Lamas and Viloria 2004; Pyrcz 2010; Benyamini et al. 2014; Matz and Brower 2016). The group comprises ~50 species, many of which exhibit substantial intraspecific variability while often showing minimal differences at the interspecific level. Most species are found in Chile (41 species) and Argentina (35 species), regions where prolific lepidopterists such as Kenneth J. Hayward (1891–1972) and José Herrera (1913–1992) conducted their studies. Consequently, Neosatyriti has been one of the most intensively studied groups of Neotropical Satyrinae taxa during the 20th Century (for a historical overview, see Matz and Brower 2016).
Hayward and Herrera described 10 genera, some of which were monobasic (=a genus including only a single species), based solely on morphological traits, in particular wing venation and, to a lesser extent, male genitalia. Most genera studied in this research were initially placed in the ‘Elina series’, a group of undefined systematic status within the tribe Pronophilini, by Miller (1968) in his world revision of what was then the family Satyridae. However, other genera studied here were associated by Miller (1968) with the predominantly tropical ‘Lymanopoda series’. Viloria (2004) later grouped most of these genera within the so-called ‘Temperate Neotropical Satyrinae’, removing them from Pronophilini and assigning them to the Australian tribe Hypocystini (currently an infratribe of Coenonymphina; Zhang et al. 2021). Viloria also placed the genera Neomaniola and Stuardosatyrus within the Holarctic subtribe Erebiina based on morphological evidence, a classification reflected in the Neotropical butterfly catalogue (Lamas and Viloria 2004). Pyrcz (2010) subsequently recognised this group as a distinct lineage, the ‘Austral Pronophilini’, including two genera previously excluded by Viloria (2004). Matz and Brower (2016) confirmed the monophyly of this group within the subtribe Pronophilina using two mitochondrial and two nuclear genes and introduced the term ‘Neosatyriti’ to describe this lineage. We acknowledge that previous terms such as ‘Elina series’ and ‘Temperate Neotropical Satyrinae’ lack inclusivity, and ‘Austral Pronophilini’ refers to a non-recognised systematic category, we recognise ‘Neosatyriti’ as a formal and adequate infratribal designation. The name follows the convention of derivation from the oldest generic name within the group, although Elina (Miller, 1968) and Argyrophorus (Blanchard, 1852) predate Neosatyrus (Wallengren, 1858). However, to maintain nomenclatural stability, we retain ‘Neosatyriti’ without proposing a replacement name.
Recent contributions have advanced our understanding of various Neosatyriti clades, including phylogenetic studies of Pampasatyrus (Zacca et al. 2017), high-altitude Satyrinae from southern Peru (Cerdeña et al. 2014), and taxonomic reviews and new species descriptions within Faunula (=Tetraphlebia) (Pyrcz 2012), Chilean Satyrinae (Benyamini et al. 2014) and Argyrophorus (Pyrcz and Wojtusiak 2010; Pyrcz et al. 2019). Additionally, the genus Punargentus was recently revised (Pyrcz et al. 2023). Three broader phylogenetic studies using molecular markers concerned partly (Peña et al. 2006, 2011) or entirely (Matz and Brower 2016) with the Neosatyriti were published, as well as one using chromosomal numbers (Brown et al. 2007). Peña et al. (2006, 2011) examined 16 species belonging to 12 genera, using three and five genetic markers respectively. More recently, Matz and Brower (2016) examined 26 species classified within 11 genera (some synonymised) using four genetic markers (two mitochondrial and two nuclear). Both molecular studies yielded one major conclusion: Austral Pronophilina (Neosatyriti) forms a monophyletic group. However, whereas Peña et al. (2011) showed they form a clade within the entirely Neotropical subtribe Pronophilina, Matz and Brower (2016), by contrast, indicated that the subtribe Pronophilina is monophyletic only if the subtribes Euptychiina and Maniolina are included, and the genus Eretris Thieme is excluded. Recent molecular phylogenies of the subtribe Euptychiina demonstrated that this subtribe is monophyletic and does not include any genus traditionally associated with the Pronophilina (Espeland et al. 2019, 2023), and also found a monophyletic Pronophilina, but with low taxa sampling for this subtribe. Although the monophyly of Neosatyriti does not raise any controversy, their internal phylogenetic relationships and the validity of many genera are arguable, and so far, have not been entirely resolvable due to insufficient sampling in previous studies. Here, we analyze the most comprehensive Neosatyriti dataset so far, consisting of twice as many species as in the latest study, 53 out of 57 species of the entire group, and all recognised genera, with dramatically increased gene sampling using target enrichment.
Material and methods
Material
Adult individuals were collected by Tomasz Pyrcz, Pierre Boyer and Christer Fahraeus in Peru, Bolivia, Chile, Argentina and Brazil from 1998 onward. Most specimens used for morphological comparisons in this study, representing all existing species and subspecies of the Neosatyriti, are deposited at the Nature Education Centre of the Jagiellonian University, Kraków, Poland (CEP-UJ). For species identification, additional specimens from major European and American collections were examined, including historical specimens and type material when available. These collections included the Natural History Museum, London, UK (NHMUK); Museo de Historia Natural de la Universidad Nacional de San Agustín, Arequipa, Peru (MUSA); Museum für Naturkunde, Berlin, Germany (ZMB); Senckenberg Naturhistorische Sammlungen, Dresden, Germany (MTD); Florida Museum of Natural History, University of Florida, Gainesville, USA (FLMNH); and Museo de Historia Natural de la Universidad Nacional Mayor de San Marcos, Lima, Peru (MUSM).
Morphology
External morphological characters of adults, particularly wing colour patterns, venation and head parts, were examined and compared using a Prolab stereomicroscope. Male and female genitalia were dissected, examined and compared (see Supplementary Table S1 for the list of genital dissections). Genital parts were removed from abdomens and soaked in 10% KOH solution for 5–10 min. Subsequently, soft tissue was removed in water to expose genital structures. Female abdomens were stained with chlorazol black to facilitate the identification of soft genital parts. Dissected genitalia were dehydrated using 90 and 95% ethanol solutions. Head parts and other external characters were examined with a Delta Optical SZ-450T stereomicroscope. A Nikon DS-Fi1 digital camera was used to capture images of dissections, which were processed using CombineZP (ver. 1.0, see https://combinezp.software.informer.com/#google_vignette) and Corel PHOTO-PAINT X3 (ver. 13.0, see https://www.coreldraw.com) to enhance focus and quality. Dissected genitalia were preserved in glycerol vials pinned alongside corresponding specimens. Terminology for genital structures follows Klots (1956) and Razowski (1996).
Molecular studies
Genomic DNA was extracted from two legs of each specimen using the NucleoSpin Tissue Kit (Macherey–Nagel, Germany) and eluted in 100 μL of molecular-grade H2O. We used the target enrichment kit for Lepidoptera developed by Mayer et al. (2021), and molecular protocols followed those described in that study.
Bioinformatics and phylogenetic analysis
Raw sequence data were processed using the TenriAn workflow developed by Mayer et al. (2021) and available at https://gitlab.leibniz-lib.de/smartin/lepi_te_workflow. In short, raw data were trimmed using fastp (ver. 2.0, see https://github.com/OpenGene/fastp Chen et al. 2018) and assembled using TRINITY (ver. 2.11, see https://github.com/trinityrnaseq/trinityrnaseq/releases; Haas et al. 2013) or SPAdes (ver. 3.15.0, see https://github.com/ablab/spades; Bankevich et al. 2012). Possible cross-contamination was filtered using a Python script available as part of the workflow and the orthology assignment was done using Orthograph (ver. 0.7.1, see https://mptrsen.github.io/Orthograph; Petersen et al. 2017). Orthologous sequences of individual loci were aligned using HMMALIGN from the HMMER package (ver. 3.3.2, see http://hmmer.org) and subsequent filtering was done using multiple criteria to remove outliers and randomly evolving sites. Loci with a GC content of 60% or higher, those shorter than 250 base pairs (bp), and those containing less than 30 samples were removed. Finally, all loci were manually checked to remove possibly remaining contamination or poorly aligned sequences. The full dataset contained 65 taxa, 621 loci, and had a length of 248,373 bp, with a total of 21.84% missing data. These loci were concatenated using AMAS (ver. 0.94, see https://github.com/marekborowiec/AMAS; Borowiec 2016).
Phylogenetic trees were inferred using IQ-TREE (ver. 2.1.3, see http://www.iqtree.org/; Minh et al. 2020) on the concatenated data. Data were partitioned to locus, and substitution models were selected using ModelFinder (ver. 2.1.3 see http://www.iqtree.org/ModelFinder/; Kalyaanamoorthy et al. 2017). We performed 20 likelihood searches with 1000 ultrafast bootstrap replicates (with the -bnni option to reduce model violations) and the SH-like approximate likelihood ratio test (1000 replicates) (Guindon et al. 2010) to assess support. Bioinformatic processing and maximum likelihood analyses were done on the Leibniz Institute for the Analysis of Biodiversity Change (LIB) Rocks Cluster.
Divergence time estimation
We conducted divergence time estimation using Bayesian analysis. Owing to computational constraints, we reduced the dataset using the ‘gene shopping’ method in the Python package SortaDate (ver. 2.7, see https://github.com/FePhyFoFum/sortadate; Smith et al. 2018), selecting the 50 most informative loci from our original 621 loci. This package determines which gene trees are clock-like, have the least topological conflict with the species tree, and have informative branch lengths (Stubbs et al. 2020). The divergence time estimation was performed using the Standard mode in BEAST (ver. 2.6.3, see https://www.beast2.org; Bouckaert et al. 2019), and the evolutionary model used for each partition was selected by BEAST–ModelTest (bModelTest, ver. 1.2.1, see https://github.com/BEAST2-Dev/bModelTest; Bouckaert and Drummond 2017). The ML tree was used as the starting tree in the analysis. The MCMC chains were run for 400 million generations and sampled once every 50,000. A relaxed clock lognormal model and birth–death tree prior to the analysis were used. Three independent runs were started and combined with LogCombiner (ver. 2.6.3, see https://beast.community/logcombiner; Bouckaert et al. 2019). The posterior distribution of trees from the analysis was then summarised in TreeAnnotator (ver. 2.6.3, see https://www.beast2.org/treeannotator/; Bouckaert et al. 2019) with a selected burn-in of 20% to build the maximum clade credibility tree, and posterior probabilities (pp) were calculated as branch support. Chain convergence was further assessed with Tracer (ver. 1.6, A. Rambaut, M. Suchard, D. Xie and A. J. Drummond, see https://github.com/beast-dev/tracer/releases/tag/v1.6) to confirm sufficient effective sampling size (ESS > 200) (Rambaut 2017). Branch support was defined as low (pp ≤ 0.75), moderate (pp = 0.75–0.95) and strong (pp ≥ 0.95). Divergence dating was performed using the NSF CIPRES portal (see https://www.phylo.org, Miller et al. 2010).
In the absence of described fossils of Pronophilina, we relied on secondary calibrations to time-calibrate the species tree. For that reason, based on Espeland et al. (2018), the selected calibration points (following the normal distribution) were applied for the crown age of the Pronophilina + Euptychiina at 30 Ma (22.3–37.8 Ma, Sigma = 4), Euptychiina at 26 Ma (18–33.1 Ma, Sigma = 3.6) and Pronophilina at 28 Ma (19.6–34.4 Ma, Sigma = 3.7). The tree was visualised using FigTree (ver. 1.4, see https://github.com/rambaut/figtree/releases). To plot the ultrametric tree against stratigraphy, the R package Strap (ver. 1.6.1, see https://cran.r-project.org/web/packages/strap/index.html; Bell and Lloyd 2015) was used, and the resulting trees were refined in Inkscape (ver. 1.3, see https://www.inkscape.org).
Raw sequence data are available in the NCBI Short Read Archive. Accession numbers can be found in Supplementary Table S2. Additional data, including alignments, tree files and input files, are available on Zenodo (Pyrcz et al. 2024a). Brazilian specimens are registered under the SISGEN: AD0AE4D.
Biogeography and ecology
Biogeographic regionalisation identified nine ecoregions where Neosatyriti species are present, based on classifications from www.oneearth.org: NT1_1: subantarctic forests (A); NT1_2: mixed Valdivian forests (B); NT2: Patagonia Steppe and Low Mountains (C); NT3: Rio de La Plata Grasslands (D); NT4: Chaco Grasslands (E); NT5_1/4: southern puna (F); NT5_5: Peruvian, northern puna (G); NT6: Chilean Matorral Shrublands and Savanna (H); NT14: Brazilian Atlantic Moist Forests and grasslands (I). All the species are assigned to the above categories.
Ancestral areas reconstruction was carried out using the R package BioGeoBEARS (ver. 1.1.3, N. J. Matzke, see http://phylo.wikidot.com/biogeobears; Matzke 2013, 2018) based on the dated tree retaining only members of the Neosatyriti. The maximum range size was set to four, which was the maximal number of areas found in any of the included species. We fitted the DEC (dispersal, extinction, cladogenesis, Ree and Smith 2008), DIVAlike (likelihood implementation of dispersal–vicariance analysis, Ronquist 1997) and BAYAREAlike (likelihood implementation of BayArea, Landis et al. 2013) models with and without the jump-dispersal parameter J, and the BAYAREA + J model was found to have the best fit (Supplementary Table S3) when comparing models using the corrected Akaike information criterion (AICc) (Matzke 2018).
Host plants of species of the Neosatyriti were identified based on published data (Beccaloni et al. 2008), and unpublished, personal observations of egg-laying behaviour and habitat preferences carried out by the authors in the field from 1988 to 2022. Host plants are divided into two taxonomic and ecological categories: bamboos (chiefly of the genus Chusquea) associated with closed forest and non-bambusoid grasses associated with open formations (mostly Stipa, Pappostipa, Festuca, Calamagrostis). Elevation was binned in three discrete categories, low (L, 0–1499 m) corresponding with lowland and premontane forests, pampas and subantarctic steppe; intermediate (I, 1500–2999 m) corresponding with montane forests and shrubs; and high (H, 3000 m and above) corresponding with puna grasslands. Marginal ancestral state reconstruction for elevation and host plants (same tree as for the ancestral state reconstruction above) was carried out using functions in the R package phytools (ver. 2.4.4, see https://cran.r-project.org/package=phytools/; Revell 2024). For the two-state host plant data without any polymorphism we first fitted equal-rates (ER) and all-rates-different (ARD) Mk models (Lewis 2001) using the function ‘fitMk’ in phytools with the prior distribution set to ‘fitzjohn’ (FitzJohn et al. 2009). Model comparison was done using AICc and the ER model was shown to have the best fit (Supplementary Table S4), and was used for ancestral state reconstruction using the function ‘ancr’ also in phytools. For the three-state elevation data, we applied the polymorphism-aware Mk model (function ‘fitpolyMk’) since several species occur in more than one binned category. This implies that the transition from one state to another can only go through the polymorphic state. Elevation was treated as an ordered character since this lets us disallow biologically implausible polymorphic states such as L + H. We fitted the ER, ARD, SYM (symmetric rates) and transient (gain of polymorphism occurs at a different rate than its loss) models. The prior distribution was set to ‘fitzjohn’ as above. The SYM model was shown to have the best fit based on AICc (Supplementary Table S5) and used for marginal ancestral state reconstruction with ‘ancr’. Results were plotted using the plot function in R.
Results
Phylogeny based on target enrichment
The infratribe Neosatyriti is confirmed as monophyletic and fully supported (Fig. 1). It appears to be sister to a clade composed of three entirely tropical genera of Pronophilina, namely Eretris + (Lymanopoda + Manerebia). The infratribe can be divided into three major, fully supported clades, for convenience called here after the most diverse genus of each clade. A detailed overview of the classification of Neosatyriti species and combinations proposed in the most recent major work, including Lamas and Viloria (2004), Pyrcz (2010), Pyrcz et al. (2016), Matz and Brower (2016) can be found in Supplementary Table S6. The ‘Neomaenas clade’ contains nine genera, but five are monobasic, and a further three are represented by two species only. The ‘Pampasatyrus clade’ contains four genera, two of which have more than five representatives (seven out of nine extant species of Pampasatyrus Hayward, 1953, were examined here, except P. yacantoensis (Köhler, 1939) and P. karri Pyrcz, Cerdeña & Boyer, 2023). The synonymy of the monobasic genus Haywardella Herrera, 1966 (type-species Epinephele edmondsii Butler, 1881) proposed by Zacca et al. (2017) is confirmed here. Finally, the ‘Neosatyrus clade’ contains five genera, including one monobasic, Neosatyrus Wallengren, 1858, from which the name of the infratribe is derived. The genus Tetraphlebia C. Felder & R. Felder, [1867], is the most species-rich within this clade with five representatives. The genus Homoeonympha C. Felder & R. Felder, [1867] is paraphyletic and only recovers as monophyletic when restricted to H. boisduvalii (Blanchard, 1852) with other species previously associated with Homoeonympha by Pyrcz et al. (2016) reassigned to Nelia Hayward, 1953 (Fig. 1e–i).
Phylogeny of the infratribe Neosatyriti based on target enrichment outlining three main clades, with adults representatives of most genera, including Haywardella = Neomaenas, except Pamperis, and (dorsal/ventral), male genitalia (in lateral view, excluding aedeagus, scale bar: 1 mm). SH, Shimodaira–Hasegawa; UF, Ultrafast bootstrap supports.
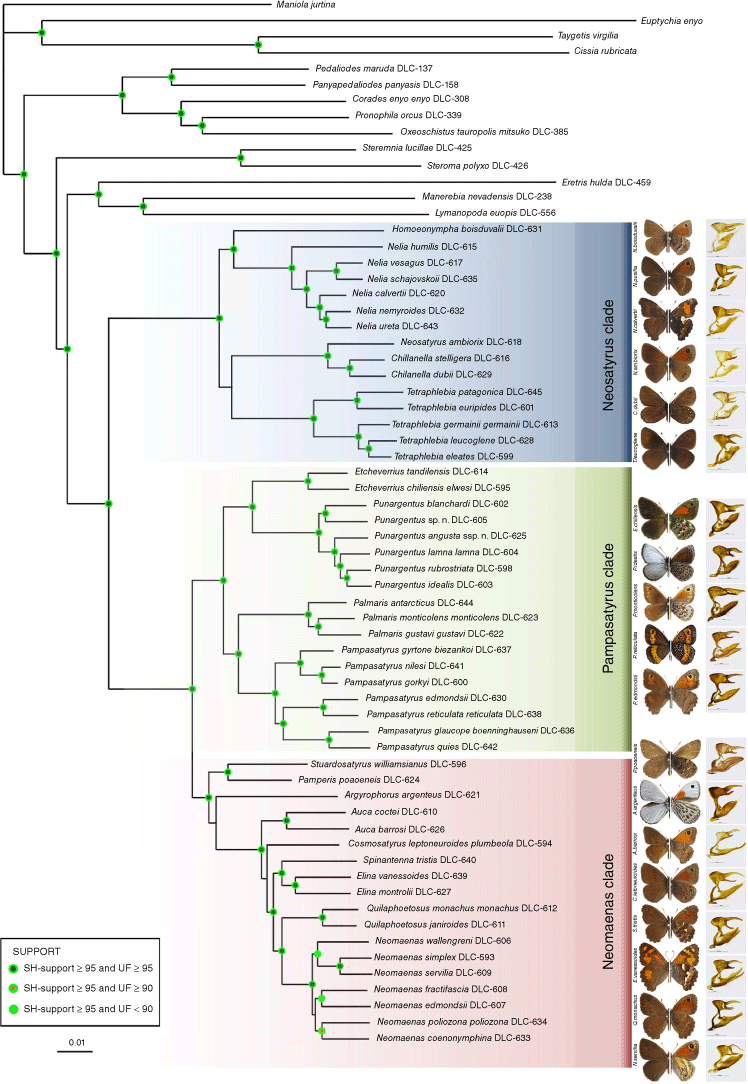
Based on extant species, we can hypothesise that Neosatyriti diverged from other Pronophilina c. 27 Ma (95% HPD: 22.82–31.54 Ma) during the Chattian Age of the Oligocene. The infratribe split into two main lineages (Neosatyrus clade and Neomaenas + Pampasatyrus clades) c. 20 Ma (95% HPD: 15.36–23.81 Ma), during the end of the Burdigalian Age of the Miocene. The Neosatyrus clade, consisting of 5 extant genera, diverged c. 12 Ma (95% HPD: 9.77–14.47 Ma), and the Neomaenas + Pampasatyrus clades, consisting of 13 extant genera, separated c. 13 Ma (95% HPD: 10.32–15.05 Ma) during the Serravallian Age of the Miocene. Finally, the major diversification of the Neosatyriti occurred during the Pliocene and at the beginning of the Pleistocene (Fig. 2).
Neosatyrus clade
This is a highly supported clade comprising 5 genera and 15 species, sister to the remaining Neosatyriti (Fig. 1). Male genitalia are rather compact, with stout uncus and gnathos, the valvae are characteristically compressed in the middle and tapering to a blunt tip (Fig. 3). Female genitalia are characterised by sparse and usually short hair on papillae anales, long posterior apophysis, large, pocket-like postvaginal lamellae, short ductus bursae, medium-sized bursae with two, short and wide signa (Fig. 4).
Male genitalia, ‘Neosatyrus clade’ (a, lateral view; b, dorsal view; c, aedeagus in lateral view; d, aedeagus in dorsal view): (a) Homoeonympha boisduvalii boisduvalii; (b) Nelia calvertii; (c) Nelia humilis humilis; (d) Neosatyrus ambiorix ambiorix; (e) Chillanella stelligera; (f) Chillanella dubii.
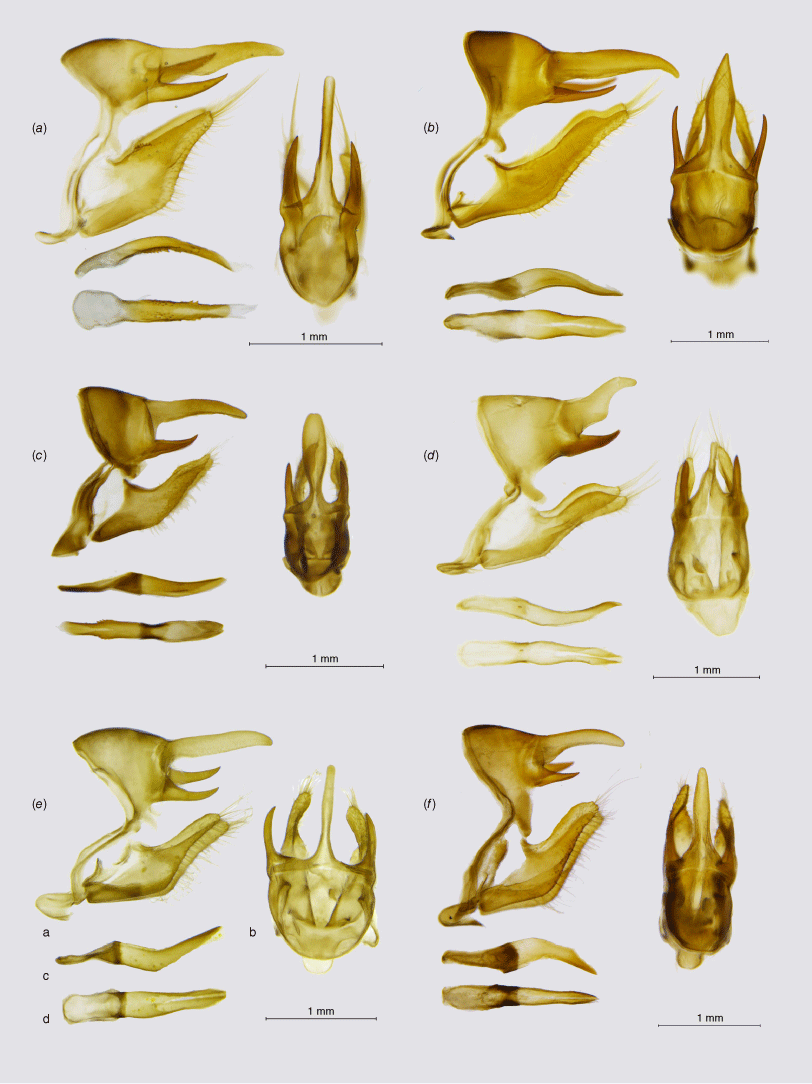
Female genitalia (lateral view): (a–d) (‘Pampasatyrus clade’): (a) Etcheverrius chiliensis chiliensis; (b) Palmaris monticolens monticolens; (c) Pampasatyrus edmondsii; (d) Punargentus lamna cuzcoensis; (e–i) (‘Neosatyrus clade’): (e) Tetraphlebia eleates; (f) Homoeonympha boisduvalii boisduvalii; (g) Neosatyrus ambiorix ambiorix; (h) Chillanella stelligera; (i) Nelia ureta.
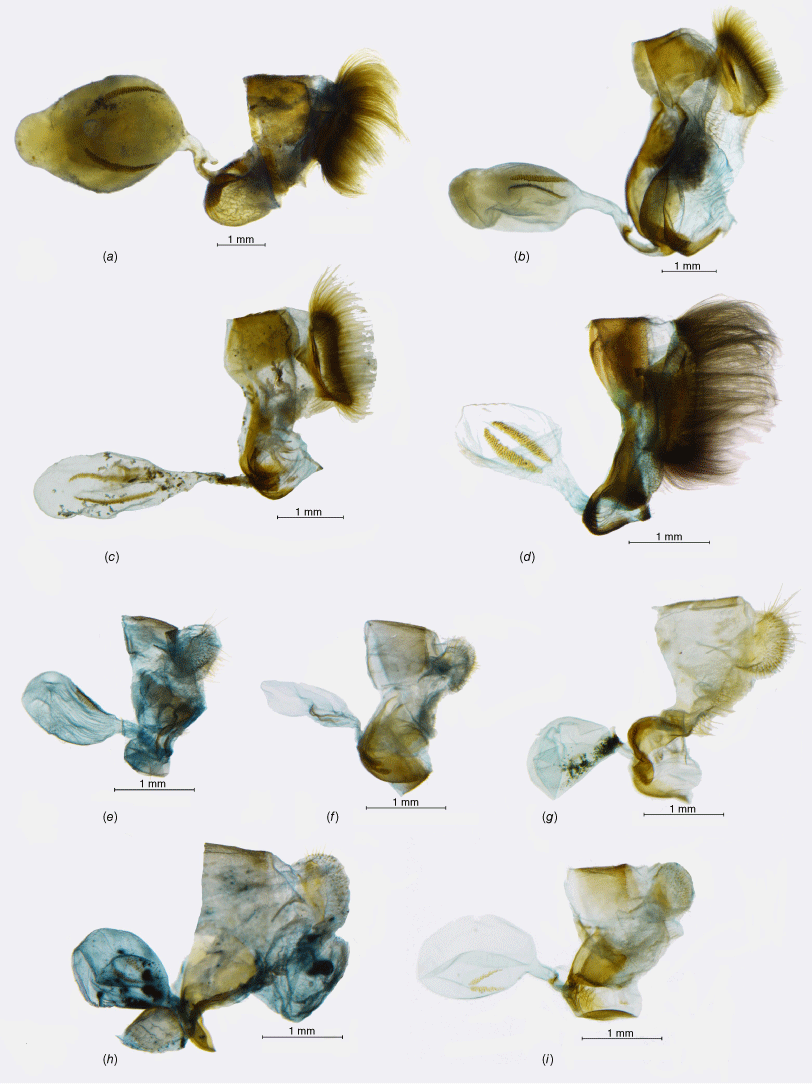
Tetraphlebia C. Felder & R. Felder, 1867
[Faunula C. Felder & R. Felder, 1867; Matz & Brower, 2016, syn.]
[Neomaniola Hayward, 1949, syn.]
The genera Faunula and Tetraphlebia were described simultaneously by C. Felder & R. Felder for two new species, the former for Faunula leucoglene C. Felder & R. Felder, 1867, and the latter for Tetraphlebia germainii C. Felder & R. Felder, 1867. These two species share some similarities in colour patterns, but T. germainii is a much bigger butterfly. The taxonomy of Faunula underwent a lot of changes, with species placed in it or removed (Lamas and Viloria 2004; Pyrcz 2010; Benyamini et al. 2014). A checklist was elaborated by Pyrcz (2012), including a new species, Faunula dubii Pyrcz, here removed to Chillanella Hayward. Matz and Brower (2016) lumped Faunula with Tetraphlebia C. Felder & R. Felder, 1867, based on their phylogenetic study. Even though we confirm here that the two genera are synonymous, in our opinion, the selection of Tetraphlebia as a priority name over Faunula was an unfortunate decision. At that moment Faunula comprised four species, whereas Tetraphlebia was monobasic. Faunula was a much better-known name, with several articles dedicated to its taxonomy, whereas Tetraphlebia was only mentioned a couple of times in scientific literature. It would have been good practice to consider the monobasic genus to be the junior synonym of the more diverse one, as it would allow the better fixation of diagnostic morphological characters of the revised genus, and restrict the number of necessary new combinations.
The genus Neomaniola Hayward, 1949, was proposed as a replacement name for Pseudomaniola Weymer, 1890, for Neomaniola euripides, which was placed in Faunula by Pyrcz (2010), an issue discussed more extensively by Pyrcz (2012). That decision is confirmed by the results of this study. Faunula eleates was originally described as a subspecies of F. leucoglene but more recent studies all treat it as a separate species, although clearly sister to F. leucoglene (Pyrcz 2012; Pyrcz et al. 2016).
All the species of Tetraphlebia occur in open habitats, either in high altitude puna (T. euripides, T. leucoglene), temperate pampas and mid-elevation grasslands (F. eleates, T. germainii) or subantarctic steppe (T. patagonica), in the bioregions NT1, NT2, NT5 and NT6. They are found from sea level to nearly 5000 m (F. euripides). A new subspecies of F. euripides from southern Peru was recently identified, it will be described in a separate paper.
We were only unable to obtain DNA data for Tetraphlebia germainii argentina Hayward, 1962, but considering its morphology it is reasonable to retain its subspecific status.
Chillanella Hayward, 1966
The genus Chillanella, described as monobasic for C. stelligera (Butler, 1881), belongs to a highly supported clade, also including the monobasic Neosatyrus, altogether comprising three species placed previously in as many as five different genera (Pyrcz 2010; Matz and Brower 2016; Pyrcz et al. 2016). Considering important morphological differences in wing shape, colour patterns, and male genitalia between the two species placed here in Chillanella, and Neosatyrus ambiorix, we postulate retaining their separate generic status. Male genitalia of Chillanella resembles Homoeonympha, especially the valvae, and the widely open uncus and subunci, whereas the tegumen is less bulky. The uncus is stout and straight differing sharply from the uplifted uncus of Neosatyrus (Fig. 3).
We propose a new generic status for Chillanella dubii comb. nov., based on colour pattern similarities, single forewing subapical ocellus and a row of prominent white hindwing ventral submarginal dots, and molecular evidence. Our findings contradict Matz and Brower (2016) who treat C. stelligera and C. dubii as belonging to Cosmosatyrus, a genus situated, in fact, in a completely different clade of Neosatyriti (Fig. 1).
The species of Chillanella occur in temperate (C. stelligera) and subantarctic (C. dubii) grasslands in the bioregions NT1 and NT6, from sea level to mid-elevations to 2000 m.
Neosatyrus Wallengren, 1858
This genus was erected for Neosatyrus ambiorix Wallengren, 1860, a species formally described 2 years later, which was associated with several other genera, recently with Neomaenas (Pyrcz 2010; Pyrcz et al. 2019). Matz and Brower (2016) did not examine the DNA of N. ambiorix but placed one additional species, H. humilis C. Felder & R. Felder, [1867], in the genus Neosatyrus, which is externally confusingly similar but has quite different genitalia (see Pyrcz et al. 2016), and according to our study is relatively distantly related to N. ambiorix, clustering with Homoeonympha and Nelia (Fig. 1). Therefore, the genus Neosatyrus is considered here as monobasic. The male genitalia, although quite simple and generally not unlike many other genera of the infratribe, are unique by presenting a massive, uplifted – elephant trunk like – uncus, as well as slender, arched valvae with a particularly elongated basal part.
The only representative of Neosatyrus occurs in temperate forests, in the bioregion NT2 (N. ambiorix obscuratior) and NT6 (N. ambiorix ambiorix), from sea level to mid-elevations, as high as 1800 m (N. ambiorix obscuratior).
Homoeonympha C. Felder & R. Felder, [1867] rev. stat.
[Stygnus C. Felder & R. Felder, [1867] (preocc. Stygnus Perty, 1833)]
[Stygnolepsis Strand, 1942 (repl. name for Stygnus)]
[Erebina Bryk, 1944?]
The genus Homoeonympha C. Felder & R. Felder, [1867] was described for H. pusilla C. Felder & R. Felder, considered here and, elsewhere by Matz and Brower (2016), as a subspecies of H. boisduvalii (Blanchard, 1852).
According to the results of our molecular study, Homoeonympha appears as paraphyletic. Considering this, three species associated so far with Homoeonympha (H. schajovskoii, H. humilis and H. vesagus) are removed from the genus Nelia, even though H. boisduvalii closely resembles the three in colour patterns and genitalia (Fig. 3). In consequence, Homoenympha rev. stat. is restricted to H. boisduvalii and becomes monobasic, sister to Nelia on a very long branch, suggesting a divergence time at 10.8 Ma (95% HPD: 8.81–13 Ma).
Homoeonympha individuals are small, inconspicuous butterflies with barely patterned, rounded wings, difficult to recognise from each other based on colour patterns only. Their male genitalia is simple, with a massive tegumen, a long uncus flattened dorso-ventrally, terminated by a blunt tip; rather short but stout, acute subunci, fairly short but stout valvae with a nearly straight, partly serrate dorsal surface, and a short, arched aedeagus with a wide proximal opening, gradually tapering to a blunt tip, with lateral cornuti. We need to point out that Nelia humilis (C. Felder & R. Felder, [1867]) comb. nov. is externally extremely similar to Neosatyrus ambiorix, the two are almost impossible to recognise without recurring to male genitalia.
Importantly, the larvae of H. boisduvalii feed on non-bambusoid grasses, contrary to other species of the clade. The nominate H. boisduvalii is found in the ecoregion NT2 whereas H. boisduvalii pusilla is also in NT6.
Nelia Hayward, 1953, rev. stat.
The genus Nelia was described by Hayward (1953) for two species previously placed in Elina, 1852, N. calvertii and N. nemyroides. Its validity was upheld by Pyrcz (2010) based on distinctive external characters mostly, and features of male genitalia, and also by Matz and Brower (2016) who placed the only examined species – N. nemyroides – as sister to ‘Neosatyrus’ humilis. Nelia recovers as fully supported when, three species previously associated with Homoeonympha, Neosatyrus or Neomaenas are added, namely N. schajovskoii comb. nov., N. humilis comb. nov. and N. vesagus comb. nov. (Fig. 1)
The genus Nelia cannot be readily identified based on morphology. On one hand, three species previously constituting Nelia are recognised from other Neosatyriti by their produced forewing apex and falcate hindwings, similar to some extent only to Auca or Elina that are situated in a completely different clade of the subtribe. On the other hand, three other species that moved here to Nelia are externally more alike Homoeonympha, Neosatyrus and Tetraphlebia with rounded wings and large FWV subapical ocelli. Male genitalia does show some similarities, in particular a humped tegumen and proportionally longer uncus not produced basally as in Homoeonympha, and a slender valva produced dorsally before apex. Additionally, the aedeagus is almost straight, or gently s-shaped with smooth edges, similar to Chillanella and Neosatyrus, compared to an arched aedeagus of Homoeonympha with a broad proximal opening and lateral cornuti.
We reject the new combination proposed by Matz and Brower (2016) associating N. schajovskoii, N. humilis and H. boisduvalii with Neosatyrus as it does not agree with the results of our phylogenetic analysis (Fig. 1). In fact, Neosatyrus belongs in the sister clade of Homoeonympha + Nelia composed of, apart from Neosatyrus, Chillanella and Tetraphlebia.
Species of Nelia are associated exclusively with humid Valdivian forests (NT1) and NT6 with host plants among Chusquea bamboos (N. vesagus comb. nov., N. shajovskoii comb. nov. and N. humilis comb. nov.), although some of them, i.e. N. calvertii, were observed to tolerate highly disturbed habitats where natural forests are replaced with eucalypts.
10. Nelia vesagus (Doubleday, [1849]) comb. nov.
[Neosatyrus ochreivittatus Butler, 1881, syn.]
[Neosatyrus violaceus Butler, 1881, syn.]
Pampasatyrus clade
The second, fully supported main branch of the Neosatyriti comprises the genus Pampasatyrus Hayward, 1953, and three genera which have been previously placed by Pyrcz et al. (2016) in the synonymy of Argyrophorus, namely Punargentus Heimlich, 1963, Palmaris Etcheverry, 1965 and Etcheverrius Herrera, 1965 (Fig. 1). Male genitalia is characterised by the elongated valvae with smooth dorsa. The uncus and the subunci are, however, rather variable (Fig. 5). All the genera of this clade share a unique character of female genitalia: papillae anales covered with dense and long hair, accompanied by a short, blunt posterior apophysis, large, pocket-like postvaginal lamellae, moderately sclerotised, rather long ductus bursae, strongly sclerotised in basal half, pear-like, large corpus bursae with two, long signa (Fig. 4a–d).
Male genitalia, ‘Pampasatyrus clade’ (a, lateral view; b, dorsal view; c, aedeagus in lateral view; d, aedeagus in dorsal view): (a) Etcheverrius tandilensis; (b) Palmaris gustavi gustavi; (c) Punargentus heimlichi; (d) Punargentus atusparia atusparia; (e) Pampasatyrus karri; (f) Pampasatyrus yacantoensis.
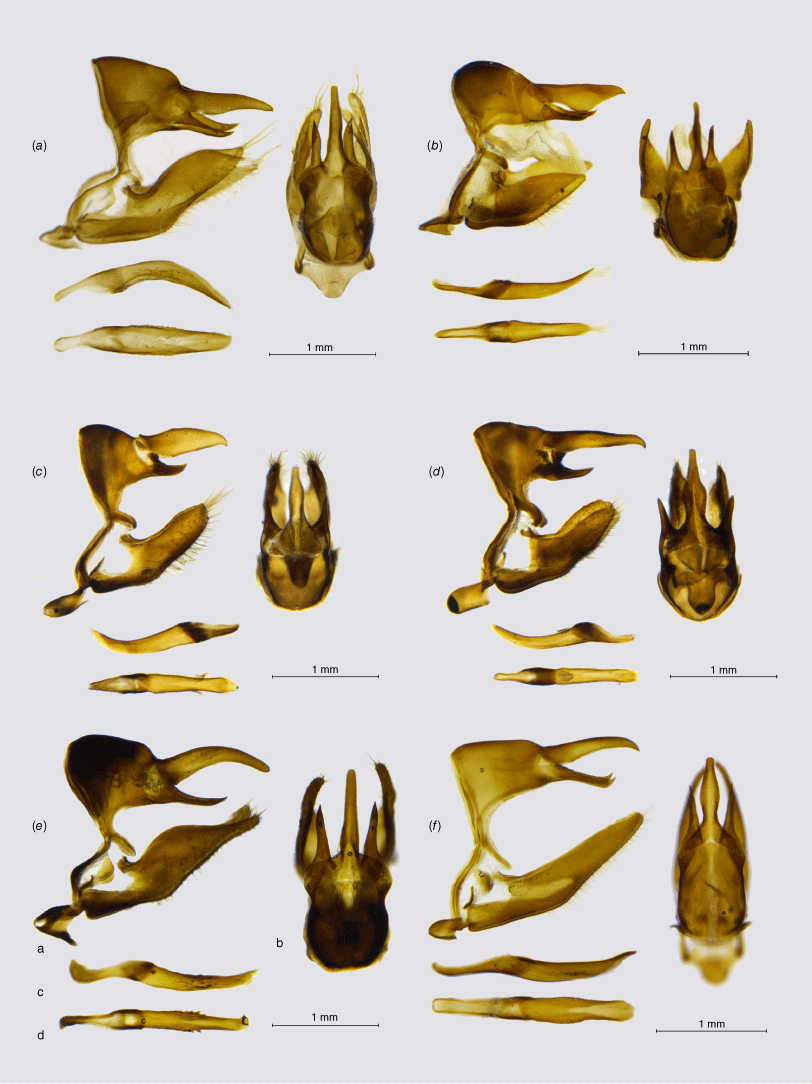
Pampasatyrus Hayward, 1953
[Haywardella Hayward, 1966, syn.]
[Pseudocercyonis Miller & Emmel, 1971, syn.]
The genus Pampasatyrus was revised by Zacca et al. (2017), based on a thorough analysis of morphological characters, who identified eight species in the genus. It upheld the monobasic genus Haywardella Hayward as sister to Pampasatyrus, even though Pyrcz (2010) and then Matz and Brower (2016) sank it as its junior synonym. Pyrcz et al. (2023) described a new species, P. karri Pyrcz, Boyer & Cerdeña, sister to P. gorkyi Pyrcz, Cerdeña & Zacca, and revised again the relationships within the genus based on COI barcodes, showing that its clusters into two well-defined clades, one of these including three Andean species and P. gyrtone, and the other mostly Brazilian and north Argentinian species. Our results based on target enrichment support the monophyly of Pampasatyrus, also indicating the internal position of P. edmondsii in the Pampasatyrus clade and its sister position to P. reticulata. No DNA sequences of P. yacantoensis were available for this study but morphological data support the generic position of this species, as suggested by Zacca et al. (2017).
The genus Pampasatyrus is found predominantly in the bioregions NT2, NT3, NT4 and NT14, in south Brazilian and Argentine mid-elevation to lowland pampas and the northern part of the subantarctic steppe, but three species are found in high altitude puna from south-central Peru to northern Argentina NT5, as high as 3800–4000 m, namely P. nilesi, P. gorkyi (Cerdeña et al. 2014) and P. karri (Pyrcz et al. 2023). All species are univoltine, flying during the austral summer. Rosa et al. (2020) described the population parameters of two Brazilian species. Hostplants are unknown, but larvae of P. glaucope and P. gyrtone accepted grasses in the laboratory, growing up to the fourth instar (Freitas et al., unpubl. data).
18. Pampasatyrus glaucope (C. Felder & R. Felder, 1867)]
Palmaris Herrera, 1965, stat. reinst.
The genus Palmaris was described by Herrera (1965) as monobasic for Hipparchia monticolens Butler, 1881, based mostly on male genitalia characters and venation. Lamas and Viloria (2004) included in Palmaris two other species – P. gustavi and P. antartictus – both closely related allopatric allies. Palmaris was synonymised with Argyrophorus by Pyrcz (2010) and Pyrcz et al. (2016), who also downgraded Palmaris penai, originally described in Punargentus, probably because of its silver upperside colour, to the rank of a subspecies of P. gustavi. Matz and Brower (2016) suggested the sister position of P. chiliensis and P. monticolens and placed the two in Punargentus. Both actions are disproved here, and the genus Palmaris stat. reinst. is formally reinstated based on our data (Fig. 1). First, Palmaris does not cluster with Argyrophorus, which is restricted to A. argenteus (as already suggested by Matz and Brower 2016), and secondly, the two species (P. monticolens and E. chiliensis) are not closely related and situate in different clades of Neosatyriti. The genitalia of Palmaris is characterised mainly by the massive, unusually shaped uncus, inflated basally. Valvae are simple and elongated with a smooth dorsal surface (Fig. 5). According to our study, Palmaris is fully supported and sister to Pampasatyrus.
The genus is found in southernmost Peru (Talca, Puno) and northern Chile within the bioregion NT5, where it occurs in high elevation puna, up to nearly 5000 m, to central Argentina NT4 and southern Patagonia NT2 where it flies in lowland subantarctic steppes.
Punargentus Heimlich, 1963
This genus was raised by Heimlich (1963) for Argyrophorus angusta. It was synonymised with Argyrophorus (Pyrcz and Wojtusiak 2010), but reinstated by Matz and Brower (2016). That decision, supported by the present study, was weakly supported in their study by combining only one species originally placed in this genus, P. lamna, with another, undescribed one. Also, Matz and Brower (2016) included in Punargentus two other species, Etcheverrius chiliensis and Palmaris monticolens, which proves incorrect, with the latter belonging to another branch of the Neosatyriti. The genus Punargentus is one of the most diverse among the Neosatyriti and comprises seven, frequently polytypic species, two of which were described recently by Pyrcz et al. (2023). That study shows Punargentus clustering into two well-defined phylogenetic and ecological groups. Punargentus are quite homogenous in external morphology, in particular by the wings marked in most species by silver dorsal markings. All the species of Punargentus also share quite similar male genitalia with a dome-shaped tegumen, a stout uncus humped in the middle and an acute apex, and thin, sharp subunci. The valvae are stout with a smooth dorsum and blunt apex, similar to Palmaris, Etcheverrius and Argryrophorus, which was one of the reasons for considering these taxa as congeneric (Pyrcz 2010; Pyrcz et al. 2016). Several new subspecies of P. lamna, as well as one of P. rubrostriata and P. angusta were identified and will be described in a separate paper (Pyrcz et al., in prep.).
Punargentus is the only genus of Neosatyriti nearly endemic to Peru, with two species, P. lamna and P. angusta marginally penetrating Bolivia, and it is found entirely within the bioregion NT5. It is also the most northerly representative of the infratribe occurring as far as northern Cajamarca. The Chamaya–Huancabamba deflection seems to be the northern distribution limit of Punargentus and thus, also, the infratribe Neosatyriti. Punargentus is strictly high Andean and associated with dry puna grasslands from 3200 m (P. lamna in Huanuco) to 4800 m (P. angusta in Ayacucho).
Etcheverrius Herrera, 1965
This genus was raised by Herrera (1965) for the single species, Cosmosatyrus chiliensis Guérin-Ménéville, 1830. It was later synonymised with Argyrophorus by Pyrcz (2010). Matz and Brower (2016) sustained that Etcheverrius is an invalid synonym but, at the same time, moved Etcheverrius chiliensis to Punargentus as sister to P. monticolens. Our results disprove both actions. We show that P. monticolens belongs to the genus Palmaris (Fig. 1). We also confirm the validity of Etcheverrius as a separate genus, sister to Punargentus. We also show that E. tandilensis comb. nov., which has not been sequenced before this study, forms a monophyletic clade with E. chiliensis. Etcheverrius can be separated easily from Punargentus by external morphology, and, especially, the male genitalia. Etcheverrius is recognised by a massive, arched uncus with an uplifted acute tip and produced basally in the middle. Our results support the placement of Argyrophorus tandilensis in Etcheverrrius as already pointed out by Pyrcz et al. (2023) (Fig. 1).
Etcheverrius chiliensis is widely polytypic, and considerable infrasubspecific variation occurs making a confident identification of the subspecies difficult. It concerns, in particular, the expression of the forewing underside basal orange or red patch, its size and colours. This issue was discussed in some detail by Pyrcz et al. (2016).
The genus Etcheverrius is found from sea level to mid, and occasionally high elevation up to 3000 m to sea level in temperate and subantarctic grassland and Patagonian shrubland from central Chile throughout most of Argentina south of the Río Salado in the bioregions NT2, NT3 and NT4.
35. Etcheverrius chiliensis (Guérin-Ménéville, 1830)
Neomaenas clade
The third, and the most diverse clade of Neosatyriti comprises eight genera. It is highly supported, and sister to the ‘Pampasatyrus clade’ (Fig. 1). Stuardosatyrus and Pamperis appear to form the sister group to the remainder of the clade, although we must point out that the position of Argyrophorus in this clade relative to other genera is weakly supported (Fig. 1). Male genitalia are variable within this clade and difficult to characterise. Those of Argyrophorus and Pamperis closely resemble the genera of the Pampasatyrus clade. Those Auca are unique for the thin, extremely elongated valvae, which are long and slender also in Elina, Quilaphoetosus, and Cosmosatyrus, but the latter genus is additionally recognisable by the particularly long uncus. By contrast, the species of Neomaenas has massive valvae produced dorsally, and a characteristic upcurved, wavy uncus (Fig. 6). Female genitalia are also more diverse than in the other two clades but can be characterised by short or very short hair on the papillae anales, moderately long posterior apophysis, massive postvaginal lamellae, moderately sclerotised with a rippled surface, except for Neomaenas, which genitalia are standing apart from other genera of this clade, by the very long posterior apophysis, smooth and rather narrow postvaginal lamella and a very long ductus bursa (Fig. 7).
Male genitalia, ‘Neomaenas clade’ (a, lateral view; b, dorsal view; c, aedeagus in lateral view; d, aedeagus in dorsal view): (a) Argyrophorus argenteus argenteus; (b) Stuardosatyrus williamsianus; (c) Auca coctei coctei; (d) Cosmosatyrus leeptoneuroides plumbeola; (e) Neomaenas servilia; (f) Quilaphoetosus monachus monachus.
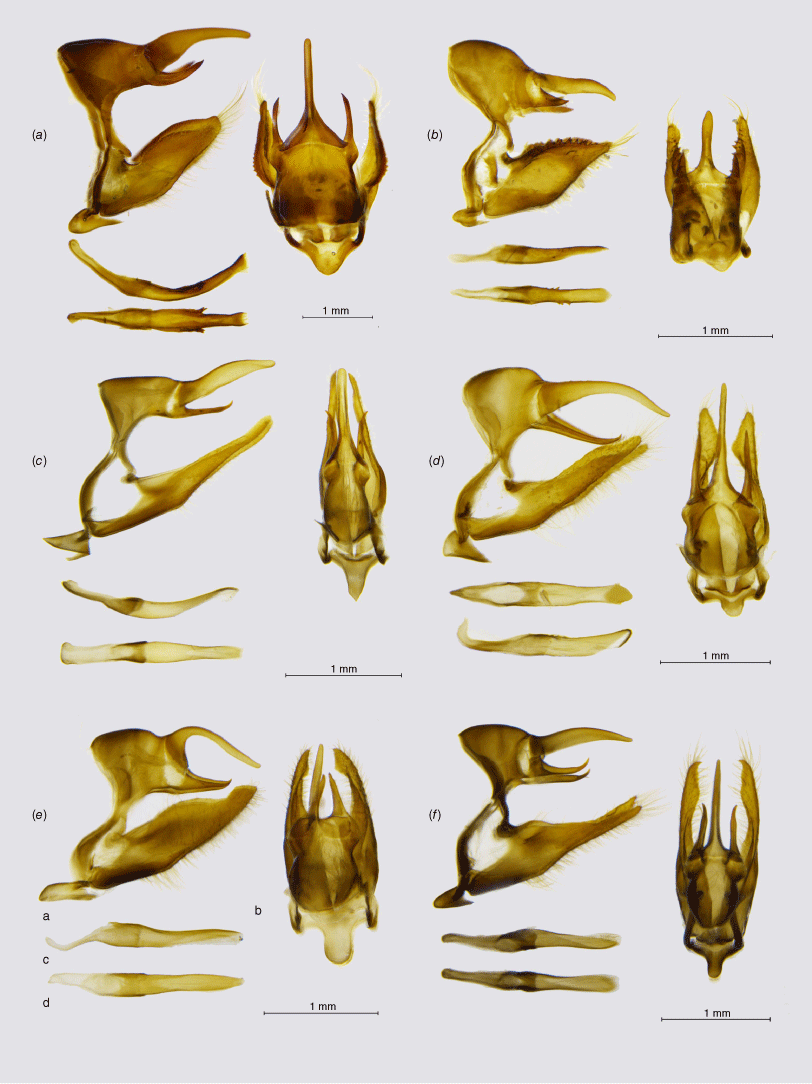
Pamperis Heimlich, 1959
This is, arguably, the least known genus of the entire infratribe. Pamperis was erected by Heimlich (1959) for his new species Pamperis poaoeneis Heimlich, 1959. Its description was actually, partly based on the incorrect drawing of wing venation made by Heimlich (1959), which shows a completely aberrant pattern. That mistake confused Miller (1968) who even doubted if the genus Pamperis belonged in the (by then) tribe Pronophilini. This issue was discussed in some detail by Pyrcz et al. (2016), based on the rediscovery of P. poaoeneis by P. Boyer in Argentina, considerably farther southwards than its type locality, Volcán Osorno. We should also mention that the illustration of P. poaoeneis in Peña et al. (1996) guide on Chilean butterflies is very unrealistic and does not depict correctly its natural appearance. The genus Pamperis was incorrectly synonymised with Argyrophorus by Pyrcz (2010) but reinstated by Matz and Brower (2016) as a sister to Argyrophorus.
Pamperis are inhabitants of Patagonian and subantarctic grassland in the bioregion NT1. They are found at mid elevations ~1200–1800 m.
Stuardosatyrus Herrera & Etcheverry, 1965
The monobasic genus Stuardosatyrus was described by Herrera and Etcheverry (1965) for Argyrophorus williamsianus, confused by some authors with Palmaris antarcticus a superficially similar subantarctic species belonging actually to a different clade of the infratribe, as explained by Pyrcz et al. (2016). It has to be pointed out that Stuardosatyrus was removed from Pronophilina by Viloria (2004) to the Australian subtribe Hypocystina, a decision reflected in the catalogue of Neotropical butterflies (Lamas and Viloria 2004). This was proved to be incorrect by Peña et al. (2006), and was confirmed by Pyrcz (2010), who however placed this species in the genus Argyrophorus. Matz and Brower (2016) did not examine S. williamsianus. In the TE tree (Fig. 1), the genera Pamperis and Stuardosatyrus are in sister position but on a long branch, which indicates their rather long-dated divergence, according to our study some 8.5 Ma (95% HPD: 6.57–10.4 Ma) (Fig. 2), therefore we decide to retain their separate status. The two share approximately similar, and male genitalia characterised by massive, constricted valvae with a serrate dorsal surface (Fig. 3).
Stuardosatyrus is an inhabitant of Patagonian and subantarctic grassland in the bioregion NT1. It is found from sea level to mid elevations ~1200–1800 m.
Argyrophorus Blanchard, 1852
Argyrophorus is considered here as monobasic, including only the nominal species A. argenteus Blanchard, 1852. The genus Argyrophorus was greatly expanded by Pyrcz (2010) and Pyrcz et al. (2016) with many genera placed as its junior synonyms, namely Etcheverrius, Pamperis, Palmaris and Punargentus based on a comparative study using adult morphology. Matz and Brower (2016) confined Argyrophorus again to A. argenteus. Based mostly on our target enrichment data we concur with that decision. Male genitalia are most similar to Pampasatyrus (Fig. 5).
Argyrophorus has a particularly wide altitudinal range, occurring from sea level to 3000 m in temperate grassland and Patagonian Steppe, where it feeds on grasses (Shapiro 1982; Henry 1992) in the bioregions NT2 and NT6.
Auca Hayward, 1953
The genus Auca was raised by Hayward (1953) and its status was not questioned by subsequent researchers (Lamas and Viloria 2004; Pyrcz 2010; Pyrcz et al. 2023). Also, Matz and Brower (2016) confirmed its monophyly and somewhat closer affinities with Cosmosatyrus. The alpha taxonomy of the genus was revised by Pyrcz et al. (2016) who recognised two species and thoroughly discussed their morphological differences, and the status of A. pales. A. coctei nycteropus stat. reinst. is considered here, following Pyrcz and Wojtusiak (2010), as the morphologically separable northern subspecies of A. coctei, and its placement as a junior synonym of the nominate A. coctei by Matz and Brower (2016) is hereby rejected. Auca forms a fully supported branch sister to the large clade comprising Cosmosatyrus, Spinantenna, Elina, Quilaphoetosus and Neomaenas.
Auca are associated with grasslands, either temperate meadows or clearings within mostly forested areas, from north-central Chile, A. coctei nycteropus within the bioregion NT6, to open habitats in south-central Patagonian bioregion NT2, A. coctei confusa. They are found from sea level to mid-elevation (1600 m).
41. Auca coctei (Guérin-Ménéville, [1838])
Neomaenas Wallengren, 1858
[Stibomorpha Butler, 1874, syn.]
[Erebina Bryk, 1944, syn.?]
Neomaenas belongs to a large, fully supported clade comprising six genera. It is the most species-rich genus of Neosatyriti with, as currently recognised, nine species. All of them were placed in this genus by Pyrcz (2010), together with Neosatyrus ambiorix, which is shown here to belong to a separate monobasic genus. Five species of Neomaenas were included in the phylogenetic hypothesis by Matz and Brower (2016). Its alpha taxonomy was revised by Pyrcz et al. (2016).
We, hereby, reject the synonymy of Quilaphoetosus with Neomaenas proposed by Matz and Brower (2016) because the two species of the former genus form a well-supported clade sister to Neomaenas and morphologically they are easily recognised, and demonstrate one salient synapomorphy, as discussed below. The male genitalia of Neomaenas is immediately recognised by a thin, long-arched uncus, and fairly short subunci with a wide base, both widely separated. The valvae are large, generally constricted towards the apex, with a serrate dorsal surface (Fig. 6).
The status of N. poliozona reedi originally described as Neosatyrus reedi Butler, 1881 needs some explanation, due to the confusion in Matz and Brower (2016), who considered it as a junior homonym of Neomaenas reedi Reed, 1877 a taxon described originally as Stibomorpha reedi Butler, 1874, and a junior synonym of Spinantenna tristis. Matz and Brower (2016) thus sank Spinantenna as a junior synonym of Neomaenas. According to our study, however, both Spinantenna and Neomaenas are valid, genera, hence no case for a homonymy and a replacement name.
The genus Neomaenas is a typical representative of temperate forest of the bioregion NT1 and NT6, and a nearly endemic to central and south-central Chile, marginally penetrating western Argentina. The species of Neomaenas occur from sea level to ~1600 m.
48. Neomaenas poliozona (C. Felder & R. Felder, [1867])
[Satyrus valdivianus Reed, 1877, nomen nudum]
[Satyrus thelxiope Reed, 1877, nomen nudum]
Quilaphoetosus Herrera, 1966
Herrera (1966) described Quilaphoetosus as two morphologically similar species previously associated with the genera Elina and Auca. Quilaphoetosus is fully supported and sister to Neomaenas. We reject the synonymy of Quilaphoetosus with Neomaenas proposed by Matz and Brower (2016), based on our molecular data, and important differences in adult morphology, in particular, the salient synapomorphy of Quilaphoetosus: the setose eyes, a unique character within Neosatyriti, whereas all other genera have naked eyes (Forster 1964; Hodór 2020) (Fig. 8). Also, the male genitalia of Quilaphoetosus are consistently different from Neomaenas, described above, and similar in most respect to the next genus, Elina, in particular, characterised by a stout, and only slightly arched uncus, with a narrower opening between the uncus and subunci. The valvae are thicker in basal part, narrowing towards apex. The aedeagus is rather simple and similar to Quilaphoetosus and Neomaenas.
Left, naked eyes of Argyrophorus argenteus argenteus; right, hairy eye of Quilaphoetosus monachus monachus (Hodór 2020).
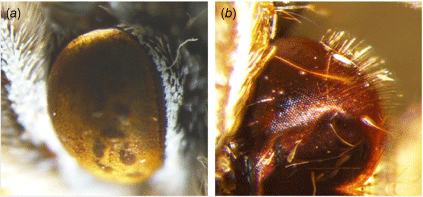
The two species occur in temperate to subantarctic forests in the bioregions NT1 and NT6, always in association with Chusquea bamboo stands.
51. Quilaphoetosus janirioides (Blanchard, 1852)
[Satyrus limonias Philippi, 1860, syn.]
[Epinephele dryas C. Felder & R. Felder, 1867, syn.]
[Epinephele blanchardi Kirby, 1871, repl. name, syn.]
[Epinephele limonias var. quinquepunctata Silva, 1916, syn.]
52. Quilaphoetosus monachus (Blanchard, 1852)
Elina Blanchard, 1852
This is a well-differentiated genus described by Blanchard (1852) for E. vanessoides and comprising, as currently recognised, two, large, conspicuously marked species. Although its separate generic status has never been challenged (Lamas and Viloria 2004; Pyrcz 2010; Matz and Brower 2016; Pyrcz et al. 2023), at some stage it also included the species currently placed in Nelia (Lewis 1974). The monophyly of Elina is fully supported (Fig. 1). Its sister genus is Spinantenna Hayward, 1953. The two taxa are considered separate genera based on differences in external morphology. The tegumen, uncus, and subunci are similar to several species, in particular, Pampasatyrus, but its valvae are much longer, and slender than in other members of the Neosatyriti, except Auca.
Both species of Elina, similarly to Neomaenas, are associated with dense bamboo thickets and occur in humid temperate forests at low elevations below 1000 m ASL.
53. Elina montrolii (Feisthamel, 1839)
[Satyrus lefebvrii Guérin-Ménéville, name preocc. Boisduval, 1828]
Spinantenna Hayward, 1953
This is a monobasic genus, whose representative, S. tristis Guérin-Ménéville, is closely related to Elina, as pointed out above. Externally, however, S. tristis is unlike any other genus of the tribe, with a usually checkered hindwing underside colour pattern. It is much smaller than the two species of Elina, approximately the size of mid-size Neomaenas. The male genitalia of Spinantenna is broadly similar to other genera of the infratribe but characterised by a very long uncus accompanied by a long and thin subuncus separated by a wide gap. The valvae are fairly long with a smooth dorsal surface, featureless and similar to many other genera, however, notably not to Elina.
Spinantenna occurs in grassland meadows within the temperate forest belt, in similar habitats as Auca in the bioregions NT1 and NT6.
55. Spinantenna tristis (Guérin-Ménéville, [1838])
[Satyrus flora Philippi, 1859, syn.]
[Pedaliodes oaxes Butler, 1870, syn.]
[Stibomorpha reedi Butler, 1874, syn.]
Cosmosatyrus C. Felder & R. Felder, [1867]
The genus Cosmosatyrus was raised for C. leptoneuroides but the scope of the genus was later vastly expanded by including several species, morphologically widely different and distantly related, associated currently with Palmaris, Stuardosatyrus or Etcheverrius (Elwes 1903; Gaede 1931; Bryk 1944; Herrera and Pérez 1989). Cosmosatyrus was restricted to only the nominal species by Pyrcz (2010). Its validity was upheld by Matz and Brower (2016) who, however, added S. stelligera, which according to our results belongs to a different clade of the infratribe, therefore that move is discarded. Cosmosatyrus is currently considered monobasic and represented by a polytypic species, C. leptoneuroides. The male genitalia of Cosmosatyrus is immediately recognised by the very long, arched and thin uncus, accompanied by long and thin subunci converging at a narrow-angle. The valvae are as long as in Elina, gradually tapering towards the apex (Fig. 8).
Cosmosatyrus leptoneuroides is associated with temperate and subantarctic grassland. The nominate subspecies occurs in temperate forests of central Chile within the bioregion NT6, replaced southwards by C. leptoneuroides plumbeola, which reaches as far as Tierra del Fuego in the bioregion NT1 and NT2. Cosmosatyrus leptoneuroides ssp. is a local, montane subspecies occurring on the Argentinian slopes.
56. Cosmosatyrus leptoneuroides C. Felder & R. Felder, [1867]
[Satyrus morania Berg, 1877, syn.]
[Erebia plumbeola var. duseni Staudinger, 1899, syn.]
[Cosmosatyrus statia Weymer, 1911, syn.]
The highest species richness of the Neosatyriti infratribe is found in two bioregions: NT6 (Chilean Matorral Shrublands) in central Chile, and NT1_2 (Valdivian Temperate Forests) in south-central Chile, hosting 27 and 29 species respectively. These regions together encompass approximately half of all Neosatyriti species. The third richest bioregion is NT2 (Patagonian Steppe and Low Mountains) in southern Argentina, with 14 species. The other six bioregions show considerably lower and fairly uniform species richness, ranging between four and nine species.
The altitudinal distribution of Neosatyriti spans an exceptional range (Fig. 9), from sea level to nearly 5000 m above sea level (ASL), exceeding most other Pronophilina groups and clades (e.g. Lymanopoda: 800–4000 m; Manerebia: 800–3600 m; Eretris: 800–3800 m). The ‘Pedaliodes clade’ is similarly extensive, occurring from sea level (Praepedaliodes Forster; Pyrcz et al. 2018) to 4800 m (Nivaliodes Pyrcz & Espeland, 2024; Pyrcz et al. 2024b). The genus Punargentus is unique as an exclusively high-altitude representative, occurring at 2600–4600 m. Several temperate genera, such as Neomaenas, Homoeonympha, Nelia and Elina, are typically lowland to premontane dwellers. The genus Pampasatyrus has the broadest altitudinal span, ranging from sea level to 4000 m.
Altitudinal distributions of the species of Neosatyriti. The bar represents the maximum and minimum elevation recorded for each species.
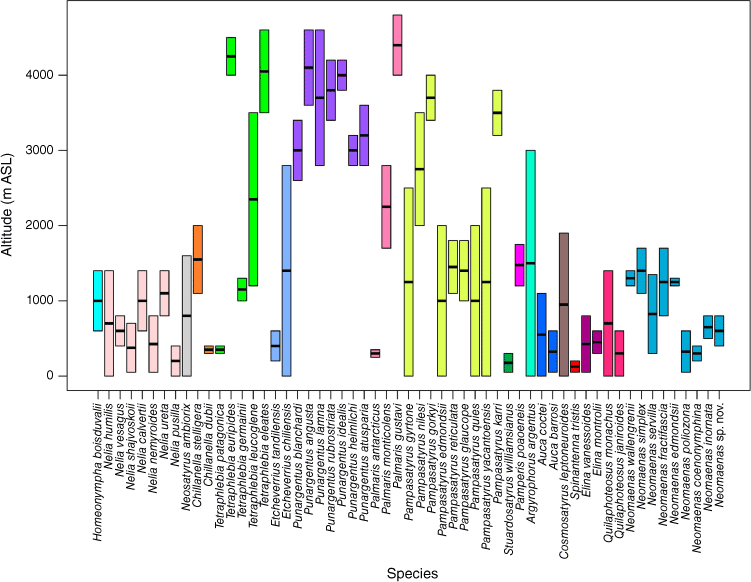
Individual species exhibit much narrower altitudinal ranges, especially lowland species, which are typically confined from a few hundred to 1000 m. A notable exception is Argyrophorus argenteus, which occurs from near sea level to above 3000 m. Although not rigorously tested statistically, available data suggest that the mean elevational range of Neosatyriti (917 m) is broader than other Pronophilina groups. Ancestral elevation reconstructions, categorised as low, intermediate or high (Fig. 10), suggest a probable low to low–intermediate-altitude origin for the infratribe. The Neomaenas clade retained a preference for lowlands, whereas the Neosatyrus clade predominantly inhabited lowlands before transitioning to higher elevations in Tetraphlebia. The Pampasatyrus clade colonised intermediate and eventually high elevations, notably through Pampasatyrus and Punargentus.
Ancestral character reconstruction of elevation and larval host plants of Neosatyriti. Low elevation = 0–1499 m, intermediate elevation = 1500–2999 m, and high elevation ≥ 3000 m.
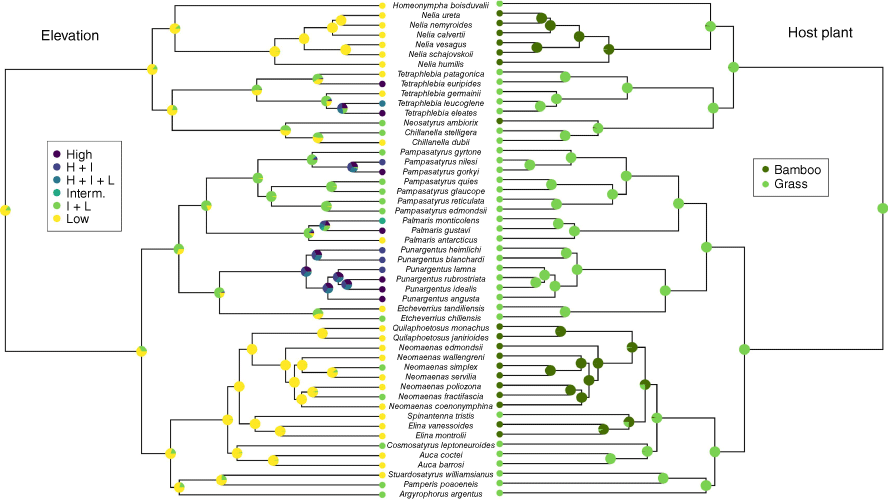
Ancestral area reconstruction (Fig. 11, 12) did not yield conclusive results, indicating, however, a higher probability that Neosatyriti originated in NT1_2, NT2, and NT6. Conversely, NT14 and NT5_5 appear to have been colonised later, with four independent colonisations of NT5_5 and a single colonisation of NT14, followed by dispersal to other bioregions, particularly NT3 and NT5_1/4. NT1_1 (Subantarctic Forest) was independently colonised by different clades no earlier than 3 Ma (Fig. 13).
Ancestral area reconstruction using the BAYAREA + J model in BioGeoBEARS (log-likelihood = −177.41, dispersal (d) = 0.006, extinction (e) = 0.0324, jump parameter (j) = 0.0256). Only the most probable area or area combination is shown at nodes and branches. A, subantarctic forests (NT1_1); B, mixed Valdivian forests (NT1_2); C, Patagonia Steppe and low mountains (NT2); D, Rio de La Plata Grasslands (NT3); E, Chaco Grasslands (NT4); F, southern Puna (NT5_1/4); G, Peruvian, northern Puna (NT5_5); H, Chilean Matorral Shrublands and Savanna (NT6); and I, Brazilian Atlantic Moist Forests and grasslands (NT14). See the map in Fig. 13. Regions are taken from www.oneearth.org/.
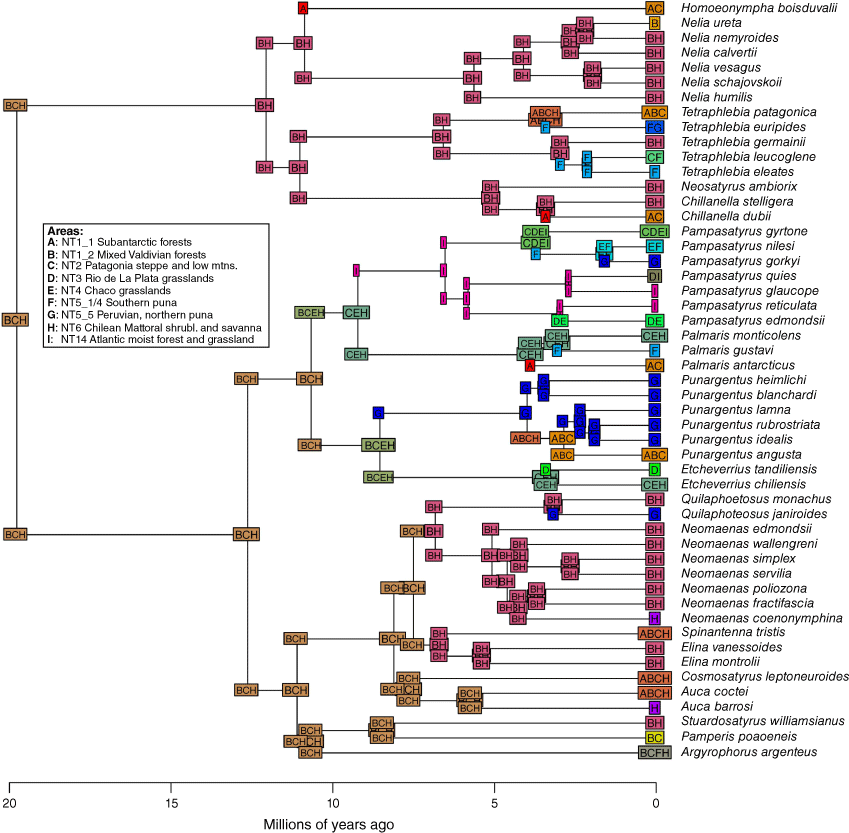
Ancestral area reconstruction using the BAYAREA+J model in BioGeoBEARS (Log-likelihood = −177.41, dispersal (d) = 0.006, extinction (e) = 0.0324, jump parameter (j) = 0.0256. Pie charts indicate the probability of each area or area combination. A = subantarctic forests (NT1_1), B, mixed Valdivian forests (NT1_2); C, Patagonia Steppe and low mountains (NT2), D = Rio de La Plata Grasslands (NT3), E = Chaco Grasslands (NT4), F = southern Puna (NT5_1/4), G = Peruvian, northern Puna (NT5_5), H = Chilean Matorral Shrublands and Savanna (NT6), and I = Brazilian Atlantic Moist Forests and grasslands (NT14). See map in Fig 13. Regions are taken from www.oneearth.org/.
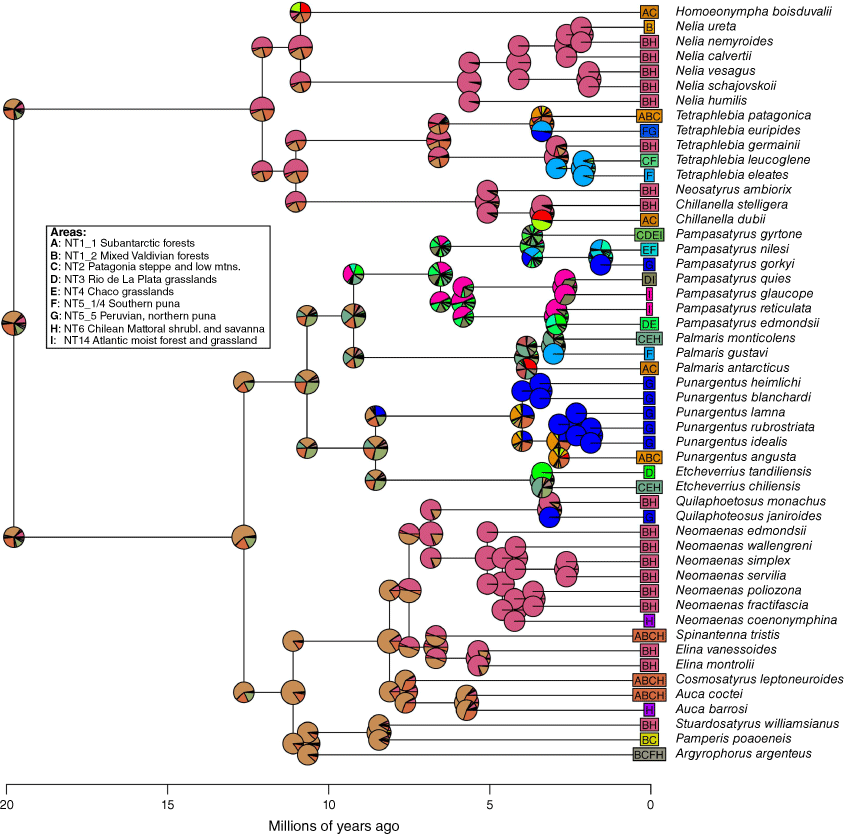
Hypothetical timing and routes of dispersal of Neosatyriti across the bioregions of the Neotropical Realm (www.oneearth.org, modified).
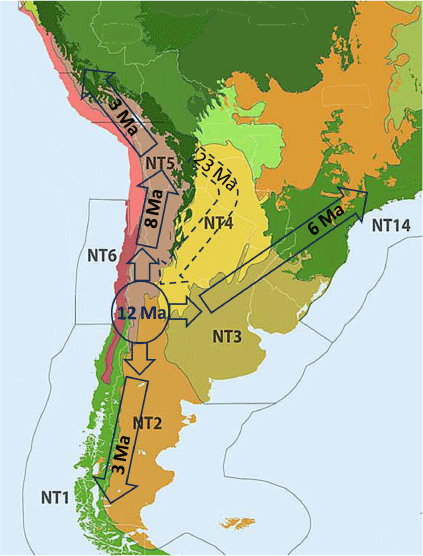
The biology of most Neosatyriti species remains poorly known, though the early stages of several species are currently being described (D. Benyamini, pers. comm.). Their larval host plants belong to two categories within Poaceae: bamboos (chiefly Chusquea) associated with Valdivian forests and shrubs in the bioregions NT1_1, NT1_2, and NT6 (Fig. 14), and non-bambusoid grasses (e.g. Stipa, Pappostipa, Calamagrostis, Festuca) associated with open habitats in NT2, NT3, NT4, NT5_5, NT5_1/4, and NT14_2/4 (Fig. 15–17). However, NT14 is misleading, as Neosatyriti species occur exclusively in pockets of pampas habitats within areas otherwise dominated by the Atlantic Forest (Fig. 15). Of the total species, 21 feed on bamboos and 36 on grasses. Ancestral host plant reconstructions indicate a non-bambusoid grass-feeding ancestor (Fig. 10). Host plant shifts to bamboos occurred independently in the Homoeonympha–Nelia clade, the Neomaenas–Elina clade (except S. tristis), and in the isolated case of Neosatyrus ambiorix within the Neosatyrus clade. The Pampasatyrus–Punargentus clade remained conservative in its use of non-bambusoid grasses.
Bioregions: (a) NT1_1: Laguna Parrillar, Magallanes, Chile, habitat of Quilaphoetosus monachus valdiviae; (b) NT1_1: Ushuaia – Lago Escondido, Tierra del Fuego, Argentina, habitat of Cosmosatyrus leptoneuroides plumbeola; (c) NT1_2: Lago Llanquihue, Los Lagos, Chile, habitat of Nelia nemyroides, Homeonympha schajovskoii; (d) NT1_2: San Carlos de Bariloche, Rio Negro, Argentina, habitat of Neosatyrus ambiorix obscuratior; (e) NT6: Volcan Llaima, Araucania, Chile, habitat of Quilaphoetosus monachus monachus, Quilaphoetosus janirioides, Neomaenas fractifascia; (f) NT6: Chillan, Ñuble, Chile, habitat of Neosatyrus ambiorix, Auca coctei coctei, Neomaenas servilia; (g) NT6: Vallecitos, Chile, habitat of Etcheverrius chiliensis ssp., Palmaris monticolens pintatus; (h) NT6: Termas de Chillan, Ńuble, Chile, habitat of Tetraphlebia germainii germainii, Neomaenas wallengrenii, Neomaenas edmondsii.
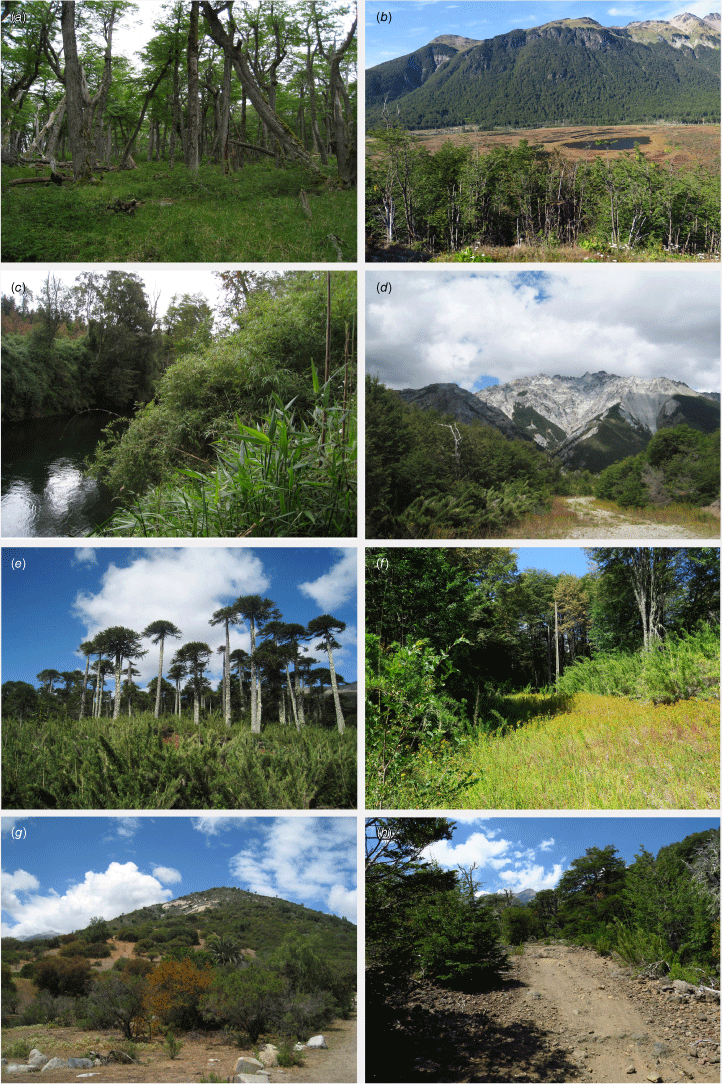
Bioregions: (a) NT14: Serra da Caparaó, Minas Gerais, Brazil, habitat of Pampasatyrus gyrtone gyrtone; (b) NT14: Serra dos Orgãos, Rio de Janeiro, Brazil, habitat of Pampasatyrus reticulata reticulata, Pampasaturus glaucope boenninghauseni; (c) NT14: Urupema, Santa Catarina, Brazil, habitat of Pampasatyrus gyrtone biezankoi; (d) NT14: Alto de Quiriri, Paraná, Brazil; (e) NT14: Urubici, Santa Catarina, Brazil, habitat of Pampasatyrus quies; (f) NT14: Serra da Bocaina, São Paulo, Brazil, habitat of Pampasaturys glaucope eberti; (g) NT3: Villa Ventana, Buenos Aires, Argentina, habitat of Etcheverrius tandilensis; (h) NT3: Parque Provincial Luro, La Pampa, Argentina, habitat of Pampasatyrus yacantoensis.
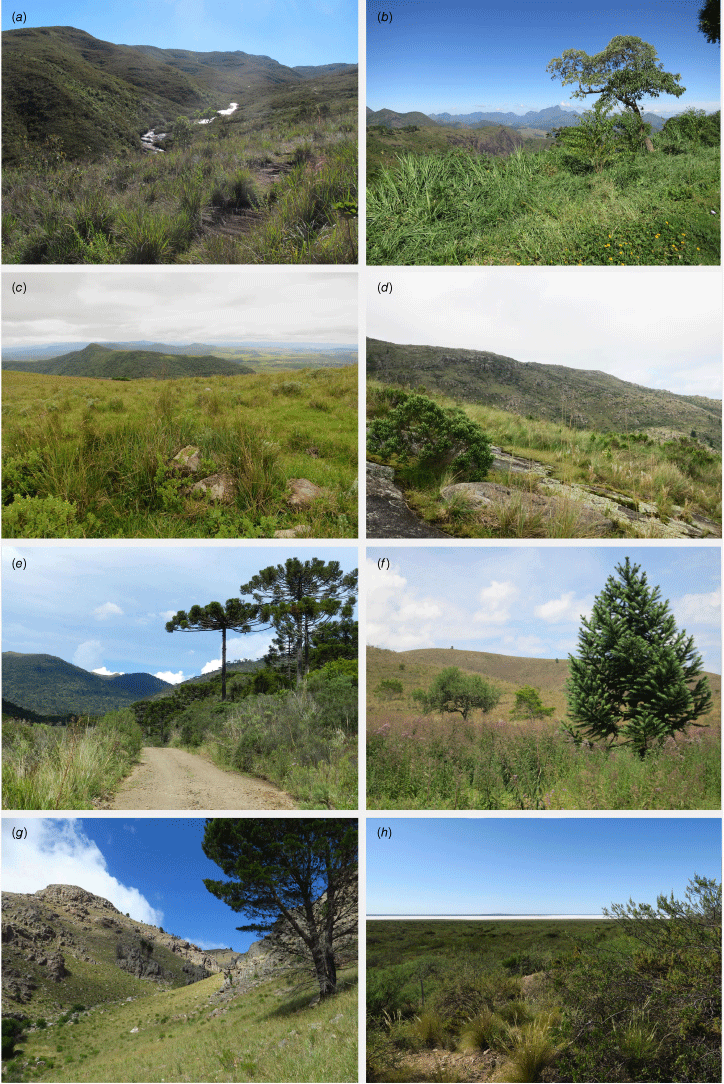
Bioregions: (a) NT5_1, Huaylas, Ancash, Peru, habitat of Punargentus atusparia atusparia; (b) NT5_1 Tayabamba, La Libertad, Peru, habitat of Punargentus lamna ssp. (c) NT5_1 Aylambo, Cajamarca, Peru, habitat of Punargentus blanchardi blanchardi; (d) NT5_1 Cocharcas, Ayacucho, Peru, habitat of Pampasatyrus karri; (e) NT5_1, Ocros, Ancash, Peru, habitat of Punargentus idealis idealis; (f) NT5_1, Pastoruri, Ancash, Peru, habitat of Punargentus rubrostriata; (g) NT5_1-4 Salar de Huasco, Tarapaca, Chile, habitat of Palmaris gustavi penai; (h) NT5_1-4 Putre, Arica, Chile, habitat of Palmaris gustavi gustavi.
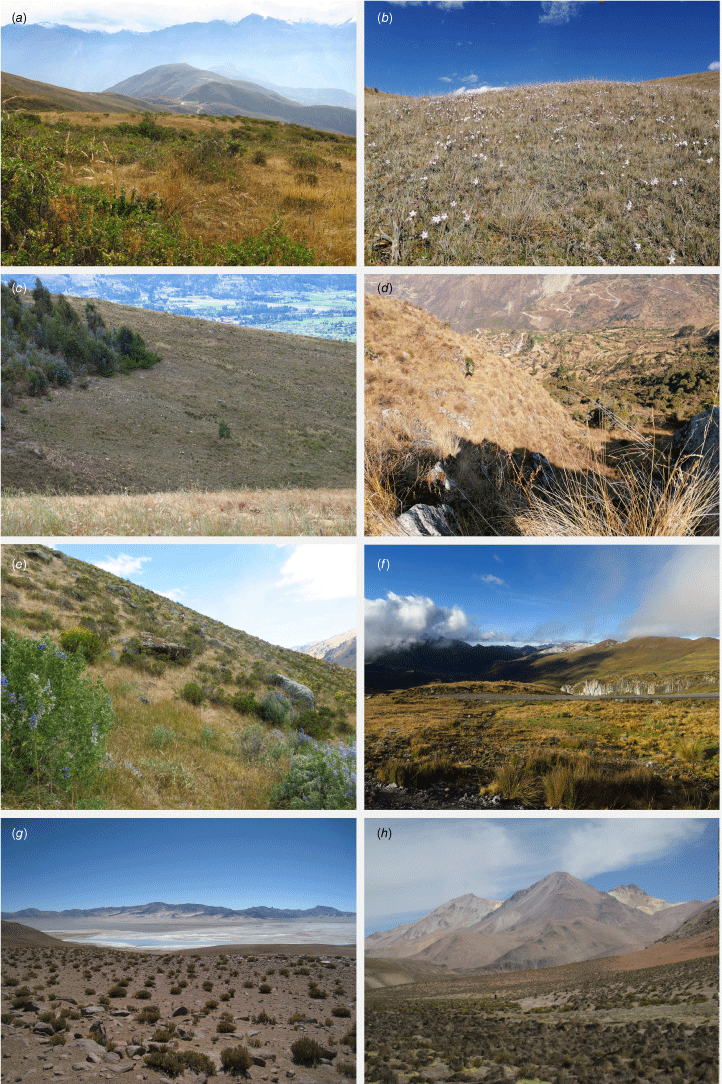
Bioregions: (a) NT2: Cumbres de San Gregorio, Magallanes, Chile, habitat of Homeonympha boisduvalii boisduvalii, Cosmosatyrus leptoneuroides plumbeola; (b) NT2: Peninsula Valdes, Chubut, Argentina, habitat of Etcheverrius chiliensis chiliensis; (c) NT2: Cerro Fitz Roy, Santa Cruz, Argentina, habitat of Palmaris antarcticus; (d) NT2: Perito Moreno, Santa Cruz, Argentina, habitat of Stuardosatyrus williamsianus; (e) NT2: Bajo Caracoles, Santa Cruz, Argentina, habitat of Stuardosatyrus williamsianus; (f) NT2: El Calafate, Santa Cruz, habitat of Etcheverrius chiliensis magallanicus, Argyrophorus argenteus argenteus; (g) NT2: road to Villa Pehuenia, Neuquen, Argentina, habitat of Etcheverrius chiliensis chiliensis, Tetraphlebia patagonica; (h) NT2: Villa Pehuenia, Neuquen, Argentina, habitat of Chillanella stelligera.
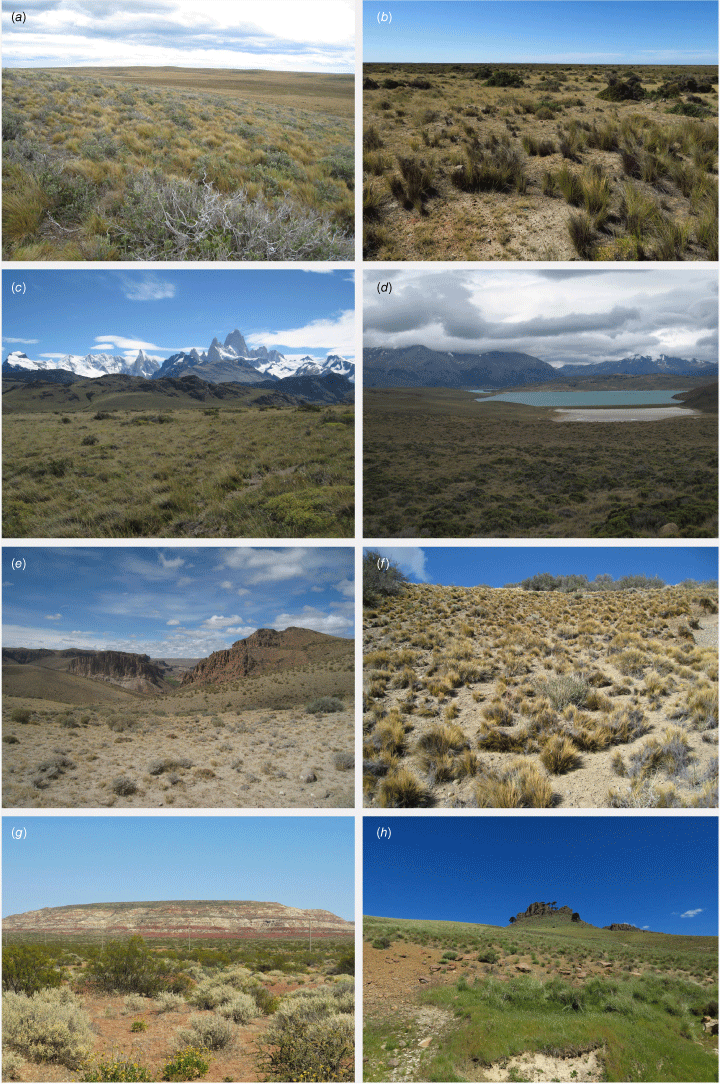
Discussion
Our phylogenetic analysis, based on comprehensive molecular datasets from 53 of 57 Neosatyriti species, confirms the infratribe as a highly supported monophyletic clade within Pronophilina. This result aligns with earlier studies using smaller datasets (Peña et al. 2011; Matz and Brower 2016).
Three main clades within Neosatyriti are fully supported. Their representatives also present some common characters of male and female genitalia, some of which are unique and allow an immediate assignation to one of the clades. The most diverse, in colour patterns, wings shapes, as well as male and female genitalia is the ‘Neomaenas clade’, with highly specialised structures, absent in other genera, e.g. in Auca male genitalia or Neomaenas female genitalia. However, the position within this clade of three genera, Pamperis, Stuardosatyrus and Argyrophorus, requires, in our opinion, more explanation. Both externally and in male genitalia, the three genera present many more common characters with the species of the sister Pampasatyrus clade. Also, the case of Homoeonympha, which in this study appears as paraphyletic needs some further research. It is remarkable, however, that nearly all the genera proposed by L. Herrera (1965, 1966, 1974, 1986, 1989), K. Hayward (1953, 1954, 1958a, 1958b, 1958c, 1958d, 1963, 1964, 1967) and W. Heimlich (1959, 1962, 1963, 1969, 1972), which were described only based on morphological characters, such as colour patterns, venation, legs or male genitalia, and at some stage synonymised or disregarded, are shown here to actually be valid. In particular, the synonymy proposed, at different stages, of several genera with Argyrophorus, namely Pamperis, Palmaris, Punargentus and Stuardosatyrus and Etcheverrius (see Pyrcz 2010) is not supported by our molecular data presented here. The only invalidated genus is Haywardella, which Zacca et al. (2017) sustained as valid and sister to Pampasatyrus after it had been synonymised by Pyrcz (2010). Twentieth-century authors heavily relied on a comparative analysis of wing venation patterns in identifying the genera. It was shown that although wing venation is generally quite conservative, in some cases it shows fast evolutionary rates even between closely related genera, and can even show intraspecific variation a matter discussed in some detail by Pyrcz and Wojtusiak (2010). Interestingly, even though some of the venation patterns (or genitalia) that were used as diagnostic for the description of some genera did not prove taxonomically valuable or informative, or simply erroneously depicted (e.g. Pamperis) (Heimlich 1959), the genera themselves prove to be valid from a molecular perspective.
Our tree topology shows two, almost simultaneous divergence events in the Late Oligocene, first the splitting of the Steroma–Steremnia clade at c. 25 Ma (95% HPD: 20.53–28.72 Ma), and shortly afterward the divergence of Neosatyriti from a common block composed of Lymanopoda + Eretris + Manerebia at 24 Ma (95% HPD: 19.73–27.59 Ma). This indicates that the relationships of Neosatyriti and its closest relatives within the subtribe are entirely resolved. However, there are other data than the molecular that, in our opinion, shed some light on this issue. The genera Lymanopoda, Eretris and Manerebia are immediately identifiable by several salient synapomorphies i.e. wing shapes and colour patterns, and genitalia with highly specialised sclerites that are markedly different from those of other Pronophilina, and especially Neosatyriti (Pyrcz et al. 2018; Mahecha-J. et al., in prep.). They are also associated exclusively with the Andean tropical montane forests, therefore ecologically and geographically showing little affinity with this infratribe. By contrast, the genus Steremnia, in particular, presents several morphological similarities with Neosatyriti; the shape of the androconial patch, or male genitalia that are very similar to genera such as Quilaphoetosus and Cosmosatyrus. Steremnia also contains some open habitats of dry puna–Andean western slopes specialist (S. rugilas, S. umbracina), frequently occurring in the same kind of habitats as some Neosatyriti, such as Punargentus or Tetraphlebia, where their larvae also feed on non-bambusoid grasses.
The initial stage of the evolution of the Neosatyriti is congruent with the appearance of the first C3 temperate grasslands habitats in the Neotropical Realm in the early Miocene (Stromberg 2011). Our reconstructions indicate that they originated in the lowlands, from non-bambusoid grass-feeding species, even though all other Pronophilina are montane and in vast majority bamboo dependent, with notable few exceptions such as Steremnia (and some other little diverse genera, e.g. some Steromapedaliodes, Pyrcz et al. 2018, Punapedaliodes, Parapedaliodes and Diaphanos, Beccaloni et al. 2008). Also, our ancestral area analysis does not indicate one particular area of origin, but rather a multisource origin with a higher probability pointing out three regions–ecozones: mixed Valdivian forests, Patagonia Steppe and low mountains, and Chilean Matorral Shrublands and Savanna where the first important radiation of Neosatyriti into major clades occurred some 12–10 Ma. These areas correspond with the highest extant species per genus diversity of the infratribe. Considering, however, the fact that Neosatyriti radiated from Pronophilina, which at 23 Ma already presented some diversity limited to the tropical Andes, we can speculate that the ancestor of Neosatyriti likely originated in the foothills of the southern tropical Andes where first larger grassland communities were constituted (Latrubesse et al. 2010) (Fig. 12).
Another major main radiation of Neosatyriti took place from the mid to late Miocene, some 7–5 Ma, and corresponded with the appearance of the most diverse genus Neomaenas, as well as Quilaphoetosus, Neosatyrus, and Nelia. This occurred alongside their switch back to the original Pronophlina host plants, Chusquea bamboos, and allowed the colonisation of close forest communities dominated by the Valdivian forests and Chilean shrublands. Intriguingly, such a switchback did not occur in the mountains of SE Brazil, which were colonised at a later stage, some 6–5 Ma (Fig. 12), despite the high availability of bamboo in the humid montane Atlantic Forest, as local Pampasatyrus are all associated with open high-altitude grasslands, they maintained the same ancestral use of non-bambusoid grasses, although most of the surrounding areas are covered mostly with dense forests rich of bamboos. The adaptation of Neosatyriti to cold, grassland environments enabled them to colonise the subantarctic grasslands all the way to the Tierra del Fuego and extend their range northwards along the puna habitats of the high Andes, reaching as far as northern Peru, which was the latest stage of dispersal of Neosatyriti, in the Early Pleistocene, some 2 Ma (Fig. 12). Incidentally, the colonisation of central Peruvian puna by the Neosatyriti coincides in time with that of Anthus, an obligate grassland group of Neotropical passerine birds (van Els et al. 2019), a phylogenetically a distant taxon, which possibly indicates a wider scale biogeographical pattern in this region of the Neotropical Realm.
Conclusions
The infratribe Neosatyriti encompasses 57 species distributed from southern Patagonia to northern Peru along the Atlantic coast, with central Chile as its diversity hotspot, occupying habitats ranging from grasslands (puna, pampas, and subantarctic) to Valdivian forests and Chilean matorral. Based on molecular data from target enrichment, our phylogenetic analysis confirms the monophyly of Neosatyriti and supports eight new taxonomic combinations and two status reinstatements. Although morphological data largely align with molecular findings, Homoeonympha appears paraphyletic and Haywardella is synonymised with Pampasatyrus.
Finally, Neosatyriti diverged from other Pronophilina at c. 23 Ma and split into two major lineages at c. 20 Ma. The evolutionary history indicates a multisource origin, with ancestral associations to lowland habitats and non-bambusoid grasses. Major diversification occurred 12–11 Ma, leading to the formation of three main clades: ‘Neomaenas’, ‘Pampasatyrus’ and ‘Neosatyrus’. Subsequent radiations, including a shift to bamboo host plants and colonisation of Valdivian forests, occurred 7–5 Ma. The group later expanded into the Atlantic Forest and high Andean puna during the Early Pleistocene. This evolutionary timeline reflects the dynamic interplay between habitat changes and diversification in this unique infratribe.
Data availability
The sequences that support this study are available in NCBI, with corresponding accession numbers listed in Supplementary Table S2, and the list of genital dissections in Supplementary Table S1. Alignments, selected models, tree files, and input files used for ancestral states and ancestral area reconstruction are available on Zenodo (Pyrcz et al. 2024a).
Declaration of funding
This work was supported and founded by Narodowe Centrum Nauki grant Harmonia-10 2018/30/M/NZ8/00293 ‘Evolutionary biogeography and diversification of the predominantly Andean butterfly subtribe Pronophilina (Nymphalidae, Satyrinae) based on phylogenetic data generated using modern molecular methods’ (to all authors of this paper). Andre Victor Lucci Freitas received a fellowship (304291/2020-0) from Conselho Nacional de Desenvolvimento Científico e Tecnológico (CNPq) and a grant (2021/03868-8) from Fundação de Amparo à Pesquisa do Estado de São Paulo (FAPESP).
Acknowledgments
We kindly thank Narodowe Centrum Nauki and Jagiellonian University from Poland for their support and are grateful to Sebastian Martin (LIB) for uploading sequences to NCBI and troubleshooting with the TenriAn workflow. Andre Victor Lucci Freitas thanks CNPq for the fellowship and the FAPESP for the grant. Oscar Mahecha-J. thanks the Universidad Distrital Francisco José de Caldas from Bogotá-Colombia and Miguel Gonzalo Andrade Correa, head of Instituto de Ciencias Naturales ICN at Universidad Nacional de Colombia for their support.
References
Bankevich A, Nurk S, Antipov D, Gurevich AA, Dvorkin M, Kulikov AS, et al. (2012) SPAdes: a new genome assembly algorithm and its applications to single-cell sequencing. Journal of Computational Biology 19, 455-477.
| Crossref | Google Scholar | PubMed |
Beccaloni GG, Viloria AL, Hall SK, Robinson GS (2008) Catalogue of the Hostplans of Neotropical Butterflies. Monografias Tercer Milenio Vol. 8. (Sociedad Entomológica Aragonesa; Red Iberoamericana de Biogeografía y Entomología Sistemática; Ciencia y Tecnología para el Desarrollo; Natural History Museum, London, UK; and Instituto Venezolano de Investigaciones Científicas, Venezuela)
Bell MA, Lloyd GT (2015) Strap: an R package for plotting phylogenies against stratigraphy and assessing their stratigraphic congruence. Palaeontology 58, 379-389.
| Crossref | Google Scholar |
Benyamini D, Ugarte A, Shapiro AM, Hendrik Mielke OH, Pyrcz T, Bálint Z (2014) Una lista actualizada de las mariposas de Chile (Lepidoptera, Papilionoidea and Hesperioidea) incluyendo distribución, período de vuelo y estado de conservación. Parte I incluyendo las Fa- milias: Papilionidae, Pieridae, Nymphalidae (en parte) y Hesperiid. Boletín Museo Nacional de Historia Natural 63, 9-31 [In Spanish].
| Crossref | Google Scholar |
Blanchard E (1852) Orden VI. Lepidópteris. In‘Historia Física y Política de Chile según documentos adquiridos en esta república durante doce años de residencia en ella y publicada bajo los auspicios del Supremo Gobierno, Tomo Tercero: Zoologia’. (Ed C Gay) pp. 1–112. (E. Thunot: Paris, France) [In Spanish] 10.5962/bhl.title.16172
Borowiec ML (2016) AMAS: a fast tool for alignment manipulation and computing of summary statistics. PeerJ 4, e1660.
| Crossref | Google Scholar | PubMed |
Bouckaert RR, Drummond AJ (2017) bModelTest: Bayesian phylogenetic site model averaging and model comparison. BMC Evolutionary Biology 17, 42.
| Crossref | Google Scholar | PubMed |
Bouckaert R, Vaughan TG, Barido-Sottani J, Duchêne S, Fourment M, Gavryushkina A, et al. (2019) BEAST 2.5: an advanced software platform for Bayesian evolutionary analysis. PLoS Computational Biology 15, 1006650.
| Crossref | Google Scholar | PubMed |
Brown KS, Freitas AVL, von Schoultz B, Saura AO, Saura AB (2007) Chromosomal evolution of South American frugivorous butterflies in the Satyroid clade (Nymphalidae: Charaxinae, Morphinae and Satyrinae). Biological Journal of the Linnean Society 92, 467-481.
| Crossref | Google Scholar |
Bryk F (1944) Über die Schmetterlingsausbeute der Schwedischen wissenschaftlichen Expedition nach Patagonien 1932–1934. Arkiv för Zoologi 36A(3), 1-30 [In German].
| Google Scholar |
Call E, Mayer C, Twort V, Dietz L, Wahlberg N, Espeland M (2021) Museomics: phylogenomics of the moth family Epicopeiidae (Lepidoptera) using target enrichment. Insect Systematics and Diversity 5, 6.
| Crossref | Google Scholar |
Cerdeña JA, Pyrcz TW, Zacca T (2014) Mariposas altoandinas del sur del Peru, I. Satyrinae de la puna xerofitica, con la descripción de dos nuevos taxones y tres nuevos registros para Peru (Lepidoptera: Nymphalidae). Revista Peruana de Biologia 21(3), 213-222 [In Spanish].
| Crossref | Google Scholar |
Chac LD, Thinh BB (2023) Species identification through DNA barcoding and its applications: a review. Biology Bulletin 50(6), 1143-1156.
| Crossref | Google Scholar |
Chen S, Zhou Y, Chen Y, Gu J (2018) fastp: an ultra-fast all-in-one FASTQ preprocessor. Bioinformatics 34, i884-i890.
| Crossref | Google Scholar | PubMed |
Dietz L, Eberle J, Mayer C, Kukowka S, Bohacz C, Baur H, et al. (2023) Standardized nuclear markers improve and homogenize species delimitation in Metazoa. Methods in Ecology and Evolution 14, 543-555.
| Crossref | Google Scholar |
Elwes HJ (1903) XVI. The Butterflies of Chile. Transactions of the Royal Entomological Society of London 51(3), 263-301.
| Google Scholar |
Espeland M, Breinholt J, Willmott KR, Warren AD, Vila R, Toussaint EFA, et al. (2018) A comprehensive and dated phylogenomic analysis of butterflies. Current Biology 28, 770-778.e5.
| Crossref | Google Scholar | PubMed |
Espeland M, Breinholt JW, Barbosa EP, Casagrande MM, Huertas B, Lamas G, Marín MA, Mielke OHH, Miller JY, Nakahara S, Tan D, Warren AD, Zacca T, Kawahara AY, Freitas AVL, Willmott KR (2019) Four hundred shades of brown: Higher level phylogeny of the problematic Euptychiina (Lepidoptera, Nymphalidae, Satyrinae) based on hybrid enrichment data. Molecular Phylogenetics and Evolution 131, 116-124.
| Crossref | Google Scholar | PubMed |
Espeland M, Nakahara S, Zacca T, Barbosa EP, Huertas B, Marín MA, Lamas G, Benmesbah M, Brévignon C, Casagrande MM, Fåhraeus C, Grishin N, Kawahara AY, Mielke OHH, Miller JY, Nakamura I, Navas V, Patrusky B, Pyrcz TW, Richards L, Tan D, Tyler S, Viloria , Warren AD, Xiao L, Freitas AVL, Willmott KR (2023) Combining target enrichment and Sanger sequencing data to clarify the systematics of the diverse Neotropical butterfly subtribe Euptychiina (Nymphalidae, Satyrinae). Systematic Entomology 48(4), 498-570.
| Crossref | Google Scholar |
Fišer C, Robinson CT, Malard F (2018) Cryptic species as a window into the paradigm shift of the species concept. Molecular Ecology 27, 613-635.
| Crossref | Google Scholar | PubMed |
FitzJohn RG, Maddison WP, Otto SP (2009) Estimating trait-dependent speciation and extinction rates from incompletely resolved phylogenies. Systematic Biology 58, 595-611.
| Crossref | Google Scholar |
Forster W (1964) Beitrage zur Kenntnis der Insektenfauna Boliviens XIX. Lepidoptera III. Satyridae. Veroffentlichungen der zoologischen Staatsammlung Munchen 8, 51-188 [In German].
| Google Scholar |
Guindon S, Dufayard JF, Lefort V, Anisimova M, Hordijk W, Gascuel O (2010) New algorithms and methods to estimate maximum-likelihood phylogenies: assessing the performance of PhyML 3.0. Systematic Biology 59, 307-321.
| Crossref | Google Scholar | PubMed |
Haas BJ, Papanicolaou A, Yassour M, Grabherr M, Blood PD, Bowden J, et al. (2013) De novo transcript sequence reconstruction from RNA-seq using the Trinity platform for reference generation and analysis. Nature Protocols 8, 1494-1512.
| Crossref | Google Scholar | PubMed |
Hayward KJ (1953) Satíridos argentinos (Lep. Rhop. Satyridae) I. Los géneros (excluídos Euptychia y Neomaniola). Acta Zoologica Lilloana 13, 5-66 [In Spanish].
| Google Scholar |
Hayward KJ (1954) Tres satiridos nuevos (Lepidoptera, Satyridae). Revista de la Sociedad Entomológica Argentina 17(1/2), 15-18 [In Spanish].
| Google Scholar |
Hayward KJ (1958a) Satíridos argentinos (Lep., Rhop., Satyridae) II. Los géneros (continuación). Acta Zoologica Lilloana 15, 161-181 [In Spanish].
| Google Scholar |
Hayward KJ (1958b) Satíridos argentinos (Lep. Rhop. Satyridae) III. Guía para su clasificación. Acta Zoologica Lilloana 15, 199-296 [In Spanish].
| Google Scholar |
Hayward KJ (1958c) Catalogo sinonimico de los ropalóceros argentinos excluyendo Hesperiidae (segundo suplemento). Acta Zoologica Lilloana 16, 12-21 [In Spanish].
| Google Scholar |
Hayward KJ (1958d) Dibujos de los genitales masculinos de algunos satiridos neotropicales (Lep., Rhop., Satyridae). Acta Zoologica Lilloana 16, 61-81 [In Spanish].
| Google Scholar |
Hayward KJ (1963) Dos satíridos neotropicales nuevos (Lep. Rhop. Satyridae). Revista de la Sociedad Entomológica Argentina 26(1/4), 45-46 [In Spanish].
| Google Scholar |
Hayward KJ (1964) Catálogo sinonímico de ropalóceros argentinos, excluyendo Hesperiidae (cuarto suplemento). Acta Zoologica Lilloana 19, 497-502 [In Spanish].
| Google Scholar |
Hayward KJ (1967) Tres satíridos neotropicales nuevos (Lep. Rhop. Satyridae). Acta Zoologica Lilloana 21, 19-24 [In Spanish].
| Google Scholar |
Heimlich W (1959) Eine neue Satyride aus Chile. Entomologische Zeitschrift 69(16), 173-179 [In German].
| Google Scholar |
Heimlich W (1962) Neomaenas poliozona n. comb. Entomologische Zeitschrift 72(11), 113-115 [In German].
| Google Scholar |
Heimlich W (1963) Die Gattung Argyrophorus Blanchard (Lep., Satyridae). Mitteilungen der münchner Entomologischen Gesellschaf 53, 70-79 [In German].
| Google Scholar |
Heimlich W (1969) Consideraciones sobre algunos géneros de satíridos chilenos (Lepidoptera). Boletín de la Sociedad de Biología de Concepción 41, 79-84 [In Spanish].
| Google Scholar |
Heimlich W (1972) Satyridae der südlichen Neotropis und Subantarktis (Lepi-doptera: Satyridae). Beiträge zur Entomologie 22(3/6), 149-197 [In German].
| Google Scholar |
Henry AA (1992) Redescripción del huevo, último estadio larval y la pupa de Argyrophorus argenteus Blanchard, 1852 y la descripción del último estadio larval y pupa de Etcheverrius chiliensis (Guérin-Méneville, 1832) y Auca nycteropus (Reed, 1877) (Lepidoptera: Nymphalidae). Acta Entomológica Chilena 17, 187-193 [In Spanish].
| Google Scholar |
Herrera JV (1965) Etcheverrius y Palmaris, nuevos géneros de Satyridae andinos (Lepidoptera). Publicaciones del Centro de Estudios Entomológicos. Universidad de Chile 7, 57-73 [In Spanish].
| Google Scholar |
Herrera JV (1966) Quilaphoetosus, Chillanella y Haywardella nuevos géneros de Satyridae andinos (Lepidoptera). Publicaciones del Centro de Estudios Entomológicos Andinos (Lepidoptera). Publicaciones del Centro de Estudios entomológicos Universidad de Chile 8, 69-72 [In Spanish].
| Google Scholar |
Herrera JV (1974) Auca delessei n. sp., especie gemela de Auca coctei Guerin; genitalia y cariotipos de las spp. de Auca (Lepidoptera, Satyridae). Publicaciones entomológicas. Universidad de Chile 11, 22-32 [In Spanish].
| Google Scholar |
Herrera JV (1986) Pamperis poaoeneis Heimlich (Lep., Satyridae). Observaciones, críticas y rectificaciones de la venación alar y genitalia de esta especie. Acta entomológica Chilena 13, 161-166 [In Spanish].
| Google Scholar |
Herrera JV, Etcheverry M (1965) Stuardosatyrus nuevo género de Satyridae y revalidación de la especie williamsianus Butler 1868. Publicaciones del Centro de Estudios Entomológicos. Universidad de Chile 7, 74-77 [In Spanish].
| Google Scholar |
Herrera JV, Pérez V (1989) Hallazgo en Chile de Stuardosatyrus williamsianus (Butler), 1868, y consideraciones sobre el género (Lepidoptera: Satyrinae). Acta Entomológia Chilena 15, 171-195 [In Spanish].
| Google Scholar |
Hodór D (2020) Zróżnicowanie morfologiczne motyli z podrodziny oczennicowatych (Satyrinae) na obszarze pampy i lasów waldiwijskich. Morphological variability of Satyrinae butterflies in pampa and Valdivian forests habitats. Lic. thesis, Uniwersytet Jagielloński, Poland. Available at https://ruj.uj.edu.pl/entities/publication/923fe290-25e9-4649-8c71-362149a9eb87 [In Polish with title and abstract in Polish and English]
Kalyaanamoorthy S, Minh BQ, Wong TK, Von Haeseler A, Jermiin L S (2017) ModelFinder: fast model selection for accurate phylogenetic estimates. Nature Methods 14, 587-589.
| Crossref | Google Scholar | PubMed |
Khan M, Joshi M, Espeland M, Huemer P, Lopez-Vaamonde C, Mutanen M (2024) Patterns of speciation in a parapatric pair of Saturnia moths as revealed by target capture. Molecular Ecology 33, e17194.
| Crossref | Google Scholar | PubMed |
Lamas G (2010) Nomenclatural notes on Satyrinae (Lepidoptera: Nymphalidae). SHILAP Revista Lepidoptera 38, 197-204 [In Spanish].
| Google Scholar |
Landis MJ, Matzke NJ, Moore BR, Huelsenbeck JP (2013) Bayesian analysis of biogeography when the number of areas is large. Systematic Biology 62, 789-804.
| Crossref | Google Scholar | PubMed |
Latrubesse EM, Cozzuol M, da Silva-Caminha SAF, Rigsby CA, Absy ML, Jaramillo C (2010) The Late Miocene paleogeography of the Amazon Basin and the evolution of the Amazon River system. Earth and Science Review 99, 99-124.
| Crossref | Google Scholar |
Lewis PO (2001) A likelihood approach to estimating phylogeny from discrete morphological character data. Systematic Biology 50, 913-925.
| Crossref | Google Scholar | PubMed |
Marín MA, López-Rubio A, Clavijo A, Pyrcz TW, Freitas AVL, Uribe SI, Álvarez CF (2021) Use of species delimitation approaches to tackle the cryptic diversity of an assemblage of high Andean butterflies (Lepidoptera: Papilionoidea). Genome 64, 937-949.
| Crossref | Google Scholar | PubMed |
Matz J, Brower AVZ (2016) The South Temperate Pronophilina (Lepidoptera: Nymphalidae: Satyrinae): a phylogenetic hypothesis, redescriptions and revisionary notes. Zootaxa 4125, 1-108.
| Crossref | Google Scholar | PubMed |
Matzke NJ (2013) Probabilistic historical biogeography: new models for founder-event speciation, imperfect detection, and fossils allow improved accuracy and model testing. Frontiers of Biogeography 5, 242-248.
| Crossref | Google Scholar |
Matzke NJ (2018) BioGeoBEARS: biogeography with Bayesian (and likelihood) evolutionary analysis with R scripts. Version 1.1.3. Zenodo 2018, v1.1.1 [Data files, published 6 November 2018].
| Crossref | Google Scholar |
Mayer C, Dietz L, Call E, Kukowka S, Martin S, Espeland M (2021) Adding leaves to the Lepidoptera tree: capturing hundreds of nuclear genes from old museum specimens. Systematic Entomology 46, 649-671.
| Crossref | Google Scholar |
Miller LD (1968) The higher classification, phylogeny, and zoogeography of the Satyridae (Lepidoptera). American Entomological Society 24, 1-174.
| Google Scholar |
Miller MA, Pfeiffer W, Schwartz T (2010) Creating the CIPRES Science Gateway for Inference of Large Phylogenetic Trees. In ‘Proceedings of the Gateway Computing Environments Workshop (GCE)’, 14 November 2010, New Orleans, LA, USA. INSPEC Accession Number 11705685. (IEEE) 10.1109/GCE.2010.5676129
Minh BQ, Schmidt HA, Chernomor O, Schrempf D, Woodhams MD, Von Haeseler A, Lanfear R (2020) IQ-TREE 2: new models and efficient methods for phylogenetic inference in the genomic era. Molecular Biology and Evolution 37, 1530-1534.
| Crossref | Google Scholar | PubMed |
Peña C, Wahlberg N, Weingartner E, Kodandaramaiah U, Nylin S, Freitas AVL, Brower AVZ (2006) Higher level phylogeny of Satyrinae butterlies (Lepidoptera: Nymphalidae) based on DNA sequence data. Molecular Phylogenetics and Evolution 40, 29-49.
| Crossref | Google Scholar | PubMed |
Peña C, Nylin S, Wahlberg N (2011) The radiation of Satyrini butterflies (Nymphalidae: Satyrinae): a challenge for phylogenetic methods. Zoological Journal of the Linnean Society 161(1), 64-87.
| Crossref | Google Scholar |
Petersen M, Meusemann K, Donath A, Dowling D, Liu S, Peters RS, et al. (2017) Orthograph: a versatile tool for mapping coding nucleotide sequences to clusters of orthologous genes. Bmc Bioinformatics 18, 1-10.
| Crossref | Google Scholar |
Pyrcz TW (2012) A new species of Satyrine butterfly from Patagonia in more than a century and revisional notes on the genus Faunula C. Felder & R. Felder (Lepidoptera: Nymphalidae: Satyrinae). Zootaxa 3342, 60-68.
| Google Scholar |
Pyrcz TW, Wojtusiak J (2010) A new species of Argyrophorus Blanchard from northern Peru and considerations on the value of wing venation as a source of synapomorphies in some temperate Neotropical Satyrinae (Lepidoptera: Nymphalidae). Genus 21(4), 605-613.
| Google Scholar |
Pyrcz TW, Ugarte A, Boyer P, Shapiro AM, Benyamini D (2016) An updated list of the butterflies of Chile (Lepidoptera, Papilionoidea) including distribution, flight periods and conservation status. Part II, subfamily Satyrinae (Nymphalidae), with the descriptions of new taxa. Boletín del Museo Nacional de Historia Natural, Chile 65, 31-67.
| Google Scholar |
Pyrcz TW, Prieto C, Boyer P, Lorenc-Brudecka J (2018) Discovery of a remarkable new species of Lymanopoda (Lepidoptera: Nymphalidae: Satyrinae) and considerations of its phylogenetic position: an integrative taxonomic approach. European Journal of Entomology 115, 387-399.
| Crossref | Google Scholar |
Pyrcz TW, Cerdeña J, Florczyk K (2019) Two remarkable new species of Argyrophorus Blanchard from the Peruvian high Andes (Lepidoptera, Nymphalidae, Satyrinae). Zootaxa 4656(2), 375-383.
| Crossref | Google Scholar |
Pyrcz TW, Lachowska‐Cierlik D, Willmott KR, Mrozek A, Mahecha‐Jiménez O, Fåhraeus C, Boyer P, Martín S, Espeland M (2022) A new genus in the diverse Andean Pedaliodes complex uncovered using target enrichment (Lepidoptera, Nymphalidae). Systematic Entomology 48(1), 163-177.
| Crossref | Google Scholar |
Pyrcz TW, Boyer P, Cerdeña Gutierrez JA, Mahecha Jiménez O, Lorenc-Brudecka J, Zajac KS, Garlacz R, Mrozek A, Lachowska-Clierlik D, Farfán J, Fahraeus C, Lamas G, Espeland M (2023) Diversity and relationships between Andean shrubland puna butterflies in the genus Punargentus (Lepidoptera: Nymphalidae: Satyrinae). European Journal of Entomology 120, 324-337.
| Crossref | Google Scholar |
Pyrcz TW, Mahecha-Jimenez O, Lachowska-Cierlik D, Boyer P, Freitas AVL, Florczyk K, Fåhraeus C, Cerdeña J, Núñez-Bustos EO, Espeland M (2024a) Data from: Evolutionary history of Neotropical butterflies of the infratribe Neosatyriti based on target enrichment (Lepidoptera: Nymphalidae, Satyrinae, Satyrini). Zenodo 2024, version v1 [Dataset, published 30 July 2024].
| Crossref | Google Scholar |
Pyrcz T, Mahecha-J O, Boyer P, Lachowska-Cielik D, Cerdeña J, Farfán J, Garlacz R, Lorenc-Brudecka J, Bálint Z, Fåhraeus C, Zając-Garlacz KS, Espeland M (2024b) A snow-dwelling tropical butterfly? An unprecedented discovery of a new genus of the Pedaliodes clade in an extreme, high-altitude Andean environment (Lepidoptera: Nymphalidae: Satyrinae). Zoological Journal of the Linnean Society 202(2), zlae112.
| Crossref | Google Scholar |
Rambaut A (2017) Effective sample size (ESS). BEAST documentation. (BEAST Developers) Available at https://beast.community/ess_tutorial
Ree RH, Smith SA (2008) Maximum likelihood inference of geographic range evolution by dispersal, local extinction, and cladogenesis. Systematic Biology 57, 4-14.
| Crossref | Google Scholar | PubMed |
Revell LJ (2024) phytools 2.0: an updated R ecosystem for phylogenetic comparative methods (and other things). PeerJ 12, e16505.
| Crossref | Google Scholar | PubMed |
Ronquist F (1997) Dispersal–vicariance analysis: a new approach to the quantification of historical biogeography. Systematic Biology 46, 195-203.
| Google Scholar |
Rosa AHB, Ribeiro DB, Freitas AVL (2020) Population biology, natural history and conservation of two endangered high elevation Neotropical butterflies. Journal of Insect Conservation 24(4), 681-694.
| Crossref | Google Scholar |
Sachanowicz K (2013) Separation possibilities and genital measurement variations in two cryptic species of European pierid butterflies, Leptidea juvernica Williams, 1946 and L. sinapis (Linnaeus, 1758). Zoology 116(4), 215-23.
| Crossref | Google Scholar | PubMed |
Seraphim N, Marín MA, Freitas AVL, Silva-Brandão KL (2014) Morphological and molecular marker contributions to disentangling the cryptic Hermeuptychia hermes species complex (Nymphalidae: Satyrinae: Euptychiina). Molecular Ecology Resources 14, 39-49.
| Crossref | Google Scholar | PubMed |
Shapiro AM (1982) Notas sobre los estadios inmaduros de la mariposa plateada, Argyrophorus argenteus Blanchard (Lepidoptera: Satyridae). Revista de la Sociedad Mexicana de Lepidopterología 7(1), 29-31 [In Spanish].
| Google Scholar |
Silva-Brandão KL, Lyra ML, Freitas AVL (2009) Barcoding Lepidoptera: current situation and perspectives on the usefulness of a contentious technique. Neotropical Entomology 38(4), 441-451.
| Crossref | Google Scholar | PubMed |
Smith S, Brown JW, Walker JF (2018) So many genes, so little time: comments on divergence-time estimation in the genomic era. PLoS ONE 13(5), e0197433.
| Crossref | Google Scholar | PubMed |
Stromberg C (2011) Evolution of grasses and grassland ecosystems. Annual Review of Earth and Planetary Sciences 39(1), 517-544.
| Crossref | Google Scholar |
Stubbs RL, Folk RA, Xiang CL, Chen S, Soltis DE, Cellinese N (2020) A phylogenomic perspective on evolution and discordance in the alpine–arctic plant clade Micranthes (Saxifragaceae). Frontiers in Plant Science 10, 1773.
| Crossref | Google Scholar | PubMed |
Ureta E (1956) Nuevos rhopalóceros (Lep.) de Chile. Boletín del Museo Nacional de Historia Natural 26(6), 159-185 [In Spanish].
| Google Scholar |
van Els P, Norambuena HV, Etienne RS (2019) From pampa to puna: biogeography and diversification of a group of Neotropical obligate grassland birds (Anthus: Motacillidae). Journal of Zoological Systematics and Evolutionary Research 57(3), 485-496.
| Crossref | Google Scholar |
Viloria AL (2004) Some Gondwanan and Laurasian elements in the Satyrine fauna of South America (Lepidoptera: Nymphalidae: Satyrinae). Tropical Lepidoptera Research 15(1–2), 53-55.
| Google Scholar |
Williams JL, Zhang YM, LaPolla JS, Schultz TR, Lucky A (2022) Phylogenomic delimitation of morphologically cryptic species in globetrotting Nylanderia (Hymenoptera: Formicidae) species complexes. Insect Systematics and Diversity 6(1), 10.
| Crossref | Google Scholar |
Zacca T, Mielke OHH, Pyrcz TW, Dias FMS, Casagrande MM, Boyer P (2017) Systematics of the Neotropical genus Pampasatyrus (Lepidoptera: Satyrinae: Pronophilina), with description of three new taxa. Insect Systematics & Evolution 48(3), 201-255.
| Crossref | Google Scholar |
Zhang J, Cong Q, Shen J, Opler PA, Grishin NV (2021) Genomics-guided refinement of butterfly taxonomy. The Taxonomic Report of the International Lepidoptera Survey 9, 1-55.
| Crossref | Google Scholar | PubMed |
Zubek A, Lorenc-Brudecka J, Pyrcz TW (2015) Hypandrium as a key character in resolving species-level taxonomy on the example of Perisama oppelii (Latreille) (Lepidoptera: Nymphalidae, Biblidinae). Zootaxa 3990(1), 32-40.
| Crossref | Google Scholar | PubMed |