Lower limb muscle performance during a closed chain single leg squat and a squat jump in people with leg weakness after stroke: A comparative study
Genevieve Tolé
A Department of Physiotherapy,
B Acquired Brain Injury Unit,
C Department of Physiotherapy,
D Department of Physiotherapy,
E Department of Physiotherapy,
F Department of Respiratory Research@Alfred,
G School of Health and Sports Science,
Abstract
To determine if the intention to perform an exercise at speed leads to beneficial alterations in kinematic and kinetic components of the movement in people with post-stroke hemiplegia.
Comparative study.
Subacute metropolitan rehabilitation hospital.
Convenience sample of patients admitted as an inpatient or outpatient with a diagnosis of stroke with lower limb weakness, functional ambulation category score ≥3, and ability to walk ≥14metres.
Participants performed a single leg squat exercise on their paretic and nonparetic legs on a leg sled under three conditions: 1) self-selected speed (SS), 2) fast speed (FS), 3) jump squat (JS). Measures of displacement, flight time, peak concentric velocity, and muscle excitation (via electromyography) were compared between legs and conditions.
Eleven participants (age: 56 ± 17 years; median time since stroke onset: 3.3 [IQR 3,41] months) were tested. All participants achieved a jump during the JS, as measured by displacement and flight time respectively, on both their paretic (0.25 ± 0.16 m and 0.42 ± 0.18 s) and nonparetic (0.49 ± 0.36 m and 0.73 ± 0.28 s) legs; however it was significantly lower on the non-paretic leg (p < 0.05). Peak concentric velocity increased concordantly with intended movement speed (JS-FS paretic: 0.96 m/s, non-paretic: 0.54 m/s; FS-SS paretic 0.69 m/s, nonparetic 0.38 m/s; JS-SS paretic 1.66 m/s, non-paretic 0.92 m/s). Similarly, muscle excitation increased significantly (p < 0.05) with faster speed for the paretic and nonparetic vastus lateralis. For gastrocnemius, the only significant difference was an increase during nonparetic JS vs. SS and FS.
Speed affects the kinematic and kinetic components of the movement. Performing exercises ballistically may improve training outcomes for people post-stroke.
Keywords: Stroke, resistance training, mobility, rehabilitation, plyometric.
Introduction
Resistance training has been successfully implemented in people following stroke to improve lower limb muscle strength however research to date has demonstrated a limited translation of these strength gains into improved walking capacity (Ada, Dorsch & Canning, 2006; Morris, Dodd & Morris, 2004; Williams, Kahn & Randall, 2014b). The reason for this remains unclear, however one hypothesis is that traditional resistance training programs do not take into consideration the speed at which muscles are required to contract in order to propel the body forward during gait (Williams et al., 2019).
As stated by the American College of Sports Medicine (ACSM), physiological adaptations to resistance exercise are specific to the stimulus applied (Ratamess et al., 2009). That is, when training to improve a specific function, resistance training should consider the muscle actions and range of motion involved, the muscle groups trained, the energy systems involved, and pertinent to this study, the speed of the movement(Ratamess et al., 2009). When developing resistance exercises to improve gait speed after stroke, task-specific programs should consider the velocity at which muscles contract during gait. Take for example the plantarflexors of the ankle, which generate power during push-off at angular velocities of up to 200° per second (Umberger & Martin, 2007). Traditionally, resistance exercises applied to improve gait speed after stroke have focused on maximal force, with little consideration for targeting the velocity component of muscle power, where power = force × velocity.
Power training techniques, such as ballistic and plyometric strengthening, that emphasize movement velocity to maximize power production have been used successfully in healthy subjects and athletes to optimize physical performance (Ratamess et al., 2009). The principles of ballistic resistance training have been applied in recent years in neurological populations (Cordner, Egerton, Schubert, Wijesinghe & Williams, 2021). Little is known however about whether people with stroke are capable of generating higher power via increased movement velocity when performing an exercise at speed or ballistically. Furthermore, to our knowledge, no studies have examined the mechanisms by which these increased movement velocities, if they exist, are generated. For example, doing exercises ballistically may require increases in muscle excitation to achieve greater movement speeds and induce a flight phase, where increased muscle excitation signifies greater activation of the muscle fibers.
The overall aim of this study was to determine whether the intention to perform an exercise at different speeds (self-selected, fast, ballistic) resulted in alterations in kinematic and kinetic components of the movement in people with leg weakness after stroke. The outcome measures used to address this aim were: (i) displacement (primary outcome), (ii) peak concentric velocity, (iii) flight time, and (iv) muscle excitation of the vastus lateralis and gastrocnemius muscles. A secondary aim was to evaluate whether strength of the gastrocnemius or quadriceps muscles was associated with greater peak concentric velocity, in order to determine whether muscle strength is relevant to the ability to generate faster speeds and perform ballistic exercises effectively. Examining these questions may help determine whether this form of resistance training is achievable for people with lower limb weakness after stroke, and therefore guide future research on its applicability to improve muscle power in this population.
Methods
Design
Single-centre comparative study. This study was approved by both the Alfred Health and LaTrobe University Research and Ethics Committees (project number 665/18).
Participants
A convenience sample of patients ≥18 years old who were either admitted as an inpatient to Caulfield Hospital in Melbourne, Australia, for rehabilitation following a stroke or who were enrolled in an outpatient program at Caulfield Hospital were screened for inclusion. Inpatient and outpatient lists were screened, and patients were approached for inclusion if they were able to provide informed consent, presented with lower limb weakness (determined by at least a 10% inter-limb difference of the knee extensors on hand-held dynamometry [HHD] testing) and could mobilize ≥14 m with a functional ambulation category (FAC) score of ≥3, that is, the ability to walk with continuous intermittent or light touch to assist balance or co-ordination (Holden, Gill & Magliozzi, 1986). They were excluded if they had any unstable medical condition, any orthopedic issue or any pain on weight bearing that would limit participation in the trial protocol. Healthy controls were not utilized as it is well established in the literature that this group can achieve higher rates of force production during ballistic exercises (Clark, Bryant & Humphries, 2008; Cormie, McGuigan & Newton, 2010, 2011). Recruitment aimed to encompass participants with a range of FAC scores between 3 and 6 to include varying levels of mobility.
The primary outcome for this trial was peak displacement. A study by Williams, Clark, Hansson and Paterson (2014a) investigated the feasibility of applying ballistic resistance training principles in a mixed neurological population (n = 11). They compared a seated and reclined leg press exercise performed both ballistically and conventionally, measuring peak jump height (analogous to our displacement measure) as an outcome. Post hoc analysis demonstrated a significant effect of the ballistic exercise on peak jump height during reclined leg sled exercise: 12.4 ± 4.1 cm for the ballistic condition, 7.7 ± 4.1 cm for the conventional condition; Cohen’s d effect size = 1.14. Using a power level of 0.80 and statistical significance set at 0.05, a sample size of 11 was therefore required.
Data collection
Demographic data collected included: age, sex, weight, medical co-morbidities, type of stroke (ischemic or hemorrhagic), side of stroke (L/R), time since stroke onset, FAC score and mobility aids used.
Measurements of peak displacement, flight time, peak concentric velocity and peak electromyography (EMG) activity of the gastrocnemius and vastus lateralis muscles were taken with the participant performing the exercises whilst lying supine on a leg sled machine (Fig 1a). The gastrocnemius and vastus lateralis muscles were chosen for EMG measurement as they are prime movers in key functional tasks such as walking (gastrocnemius) (Neptune, Kautz & Zajac, 2001) and stair navigation/rising from a chair (vastus lateralis) (Eriksrud & Bohannon, 2003). Vastus lateralis was chosen to represent the quadriceps femoris group as it is typically the largest of the four synergistic muscles.
(a) Single leg squat on the leg sled, (b) Pressure Mat, (c) String potentiometer, and (d) Quadricep and vastus lateralis EMG placement.
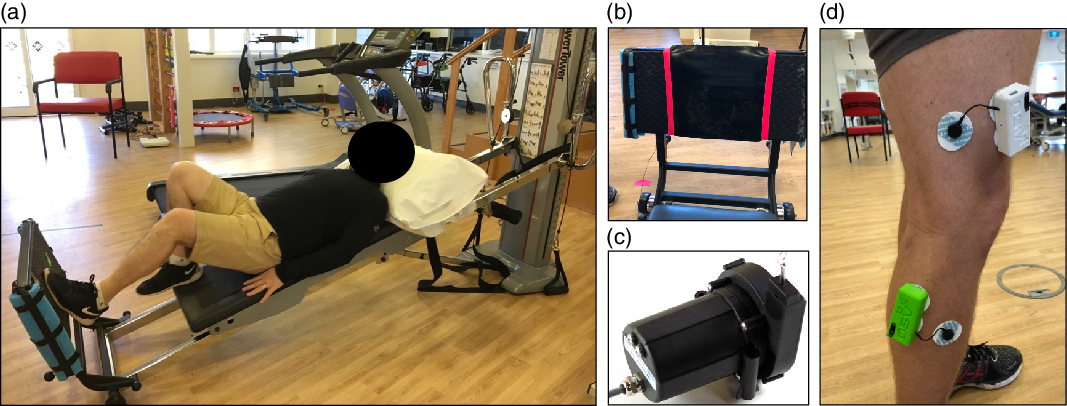
Three conditions were tested on both the paretic and nonparetic legs: 1) Self-selected speed single leg squat (SS), 2) Fast single leg squat (FS), and 3) Single leg squat jump (JS).
The SS squat provided data on the conventional form of the exercise, and was used as a basis for comparison. The FS (i.e. performing a single leg squat as fast as possible without jumping) was performed as a comparator when the intention was to perform the exercise rapidly but without a flight phase. The ‘ballistic’ JS was performed identically to the FS (i.e. as fast as possible) but with the intention to jump as high as possible at the top of the movement. This was performed to determine whether there was additional benefit of including the intention to produce a flight phase.
The tester was provided with a standardized list of instructions to follow for each assessment. Given participants routinely completed squats within their rehabilitation program, no instructions on how to perform a squat were provided. Instructions for each condition were as follows:
Condition 1: “When I say ‘go’ I want you to do a squat as you normally would three times in a row.”
Condition 2: “When I say ‘go’ I want you to do a squat as quickly as you can three times in a row” without jumping.
Condition 3: “When I say ‘go’ I want you to do a squat jump, aiming to jump as high as you possibly can three times in a row.”
Participants lay supine on a leg sled machine with one leg in contact with the footplate (Fig 1a) on top of which was a pressure mat (Fig 1b). Peak displacement was measured using a string potentiometer as described previously (Garcia-Ramos et al., 2016) (Fig 1c). The string potentiometer was attached to the leg sled machine to measure both displacement and velocity of the sled relative to the fixed plate, giving measures of peak displacement and peak concentric velocity. For the purpose of this study, peak concentric velocity was considered an acceptable proxy measure to represent power, where acceleration is directly related to velocity, and where power = mass × acceleration. The pressure mat, which consisted of Velostat pressure sensitive fabric embedded in non-slip plastic sheeting, measured flight time by measuring when the participants’ foot left the footplate and landed back on it during the ballistic JS condition. These data were acquired at 1000 Hz using a CompactDAQ system (National Instruments, USA) and customized LabVIEW software (National Instruments, USA).
Surface EMG was used to measure presence and magnitude of excitation of the vastus lateralis and gastrocnemius muscles under all three test conditions (Fig. 1d). The EMG system was a wireless 12-bit Arduino-based Myoware Muscle Sensor (common mode rejection ration: 110) sampling at 1000 Hz and synchronized with the string potentiometer and pressure mat software. A real-time clock was used to control sampling variability at the microcontroller to within 1 μs of error. Data from the two channels were sent individually to a central control unit using NRF24l+ radio frequency modules. After the skin was cleaned, EMG electrodes were placed over the muscle bellies of the lateral gastrocnemius and the distal one third of the vastus lateralis (Hermens, Freriks, Disselhorst-Klug & Rau, 2000). Placement and data quality were visually inspected via output reading of the EMG during a calf raise for lateral gastrocnemius, and a small squat for vastus lateralis. EMG data were bandpass filtered (Butterworth zero lag 4th order 20–400 Hz), rectified and smoothed using a 40 ms moving average. From these data the peak muscle excitation value during each trial was obtained. These data are the amplified millivolt recordings from the electrodes, and are reported as a unitless value as the raw value is automatically scaled to oscillate around the mid-voltage value of the microcontroller. To ensure that clipping is not present a rotary potentiometer is then adjusted to set the range of the amplified signal, with the data recorded via the Arduino 12-bit ADC in our study. Whilst it is possible to use an oscilloscope to obtain the amplification of the system as built, and we did not adjust the potentiometer between participants, these voltages cannot be compared between studies or across days due to the well-known issues with EMG. We have therefore chosen to report these data as unitless values and only compared within muscle groups, within participant as per recommendations (Vigotsky, Halperin, Lehman, Trajano & Vieira, 2018). Myoware Muscle Sensor-based systems have been validated (Heywood et al., 2018) and used in clinical research studies previously due to their flexibility and customization capability (Kahn et al., 2021).
A hand-held Lafayette dynamometer was used to measure the maximum isometric force of the gastrocnemius and the quadriceps. Testing was completed following the protocol from Mentiplay et al. (2015). Three trials were performed on each leg, one familiarization trial followed by two recorded trials. Measurements were converted to torque (Nm) using the formula: Torque = (peak force [kg] × 9.81)× distance from joint (m). These values were then normalized to body mass by dividing the torque value by body mass (Nm/kg).
Exercise testing was conducted in a single session comparing three variations of the exercise where during the first and second conditions, the foot remains in contact with the footplate, and in the third condition the participant attempts to incorporate a ‘jump’ or period where their foot is not in contact with the footplate. The testing order of the three conditions was randomized by a Microsoft Excel based random-order generator.
Participants were transferred onto the leg sled with their preferred footwear in situ and lay supine. No assistive devices such as AFO or ankle braces were worn. The foot of the leg to be tested was placed flat on the footplate, with the knee fully extended, and the heel in line with the base of the footplate. To standardize the load on the leg sled, the sled was set to level 16 which correlates with approximately 31% of body weight resistance.
The participants performed the exercises three times for each condition – a familiarization trial first, followed by two recorded trials, with a timed 2-min rest in between each trial. For each trial, three repetitions were completed. They performed the exercise on their nonparetic leg first to allow for task familiarization, and then repeated the exercise on their paretic leg. Where necessary, the tester supported the ankle being tested to ensure it landed safely on the footplate, and was supported mediolaterally.
Statistical analysis
Demographic data are presented as mean ± SD, range and number where relevant. For each condition, two trials of three repetitions were recorded. The median scores from each trial were used for the data on peak displacement, peak concentric velocity and EMG data. For HHD the best performance from the two recorded trials was used for analysis. Data were taken for each participant and paired samples t-test were used to compare differences for displacement, flight time and peak concentric velocity. Due to non-normality of data for the EMG and HHD data, a Wilcoxon signed rank test was performed to compare differences in muscle excitation. A Spearman’s rho correlation was used to determine if there was an association between peak concentric velocity during the FS and JS conditions and strength of the gastrocnemius and/or quadriceps muscles. Where missing data were present due to equipment issues, data were not imputed given the small sample size. These trials were removed and the data for that subject not included in the pairwise comparisons for analyses that included that variable. All data were analyzed using SPSS software with a significance level set at p < 0.05.
Results
Between June 2019 and November 2019, 12 participants were recruited (Table 1). Due to equipment issues at commencement of testing, no data were collected for the first participant, who was unavailable to return for testing at a later date, and therefore excluded from reporting. Participants were aged between 36 and 82 years and ranged from 1 month to 27 years since stroke onset. Gait aids used included single point sticks (n = 3), 4-point sticks (n = 3), and 2-wheel frames (n = 2). Four participants required an ankle foot orthosis to mobilize and one participant used a sports ankle brace.
Characteristics of participants (n = 11)
Age, mean (SD), range | 56.4 (16.9), 36–82 | |
Female, n | 6 | |
Type of stroke, infarct/hemorrhage n | 3/8 | |
Side of lesion | ||
Left | 8 | |
Right | 3 | |
Months since stroke onset, median (IQR) | 3.3 (2.6, 40.7) | |
BMI, kg m−2, mean (SD) | 27.6 (4.3) | |
Comorbidities, n | ||
0–1 | 9 | |
2–4 | 2 | |
FAC score | ||
3–4 | 7 | |
5–6 | 4 | |
Muscle strength (Nm/kg), median (IQR) | ||
Gastrocnemius nonparetic | 0.50 (0.40, 0.52) | |
Gastrocnemius paretic | 0.20 (0.15, 0.32) | |
Quadriceps nonparetic | 0.94 (0.79, 1.26) | |
Quadriceps paretic | 0.62 (0.32, 0.75) |
IQR: Interquartile range; BMI: Body mass index.
Peak displacement and flight time
All 11 participants were able to achieve flight phase on both their non-paretic and paretic legs. The paretic leg achieved significantly greater displacement when performing the JS compared with FS and there was a statistically significant difference in displacement between the paretic and non-paretic legs (Table 2). There was also a statistically significant difference in flight time (p = 0.003) between the nonparetic leg (0.73 ± 0.28 s) and paretic leg (0.42 ± 0.18 s).
Difference in displacement (m) between fast squat (FS) and jump squat (JS) conditions
Peak concentric velocity
There was a statistically significant difference in peak concentric velocity on both the paretic and nonparetic legs when comparing each of the three conditions (Table 3). The difference in velocity was significantly greater between the FS and SS conditions, and even greater when comparing JS and FS conditions. A statistically significant difference was also found when comparing the peak concentric velocity differences between paretic and nonparetic legs during both the FS and JS conditions, but not the SS squat condition (Table 3).
Peak concentric velocity (m/s) differences between self-selected speed (SS), fast speed (FS), and jump squat (JS) conditions
SS mean (SD) | FS mean (SD) | JS mean (SD) | FS-SS mean difference, 95% CI | JS-FS mean difference, 95% CI | JS-SS mean difference, 95% CI | ||
---|---|---|---|---|---|---|---|
Peak concentric velocity paretic leg (m/s) | 0.47 (0.24) | 0.85 (0.33) | 1.39 (0.56) | 0.38* (0.22, 0.54) | 0.54* (0.32, 0.76) | 0.92* (0.61, 1.23) | |
Peak concentric velocity non-paretic leg (m/s) | 0.54 (0.22) | 1.23 (0.28) | 2.20 (0.74) | 0.69* (0.50, 0.88) | 0.96* (0.58, 1.35) | 1.66* (1.13, 2.18) | |
Mean Difference 95% CI | 0.07 (−0.09, 0.23) | 0.39 # (0.15, 0.62) | 0.81 # (0.38, 1.23) |
Muscle strength and muscle excitation
No significant association was found between the strength of the gastrocnemius muscle on HHD testing and peak concentric velocity under the FS and JS conditions. However, statistically significant correlations were found between the paretic quadriceps strength and peak concentric velocity of FS, and nonparetic quadriceps strength and peak concentric velocity for JS (Table 4). These correlations however were weak to moderate. There were statistically significant increases in paretic and non-paretic quadriceps excitation, as measured on EMG between all three conditions, and in non-paretic gastrocnemius excitation between the JS-FS and JS-SS squat conditions (Table 5). Due to equipment malfunction there were 6 missing EMG data points across three participants.
Correlation between peak concentric velocity and muscle strength in the gastrocnemius and quadriceps muscles during the fast squat (FS) and jump squat (JS) conditions
Strength paretic gastrocnemius (Nm/kg) | Strength paretic quadriceps (Nm/kg) | Strength nonparetic gastrocnemius (Nm/kg) | Strength nonparetic quadriceps (Nm/kg) | ||
---|---|---|---|---|---|
Peak concentric velocity FS (m/s) | 0.53 (0.096) | 0.61 (0.047*) | 0.36 (0.272) | 0.53 (0.096) | |
Peak concentric velocity JS (m/s) | 0.37 (0.259) | 0.49 (0.125) | 0.47 (0.142) | 0.62 (0.043*) |
Gastrocnemius and soleus muscle excitation# measured using EMG during self-selected speed (SS), fast speed (FS), and jump squat (JS) conditions
Median (IQR) SS | Median (IQR) FS | Median (IQR) JS | FS-SS median difference (IQR) | JS-FS median difference (IQR) | JS-SS median difference (IQR) | ||
---|---|---|---|---|---|---|---|
Peak paretic excitation gastrocnemius | 0.76 (0.24, 2.4), n = 11 | 0.73 (0.25, 2.3), n = 10 | 4.73 (0.89, 9.29), n = 10 | 0.23 (−0.03, 0.77), n = 10 | 1.30 (0.53, 3.03), n = 9 | 3.09 (−0.09, 6.68), n = 10 | |
Peak paretic excitation quadriceps | 2.14 (0.43, 3.94), n = 11 | 2.13 (0.63, 7.30), n = 10 | 5.55 (2.29, 14.80), n = 10 | 0.63* (0.25, 1.25), n = 10 | 25.91* (9.65, 31.82), n = 10 | 27.88* (15.51, 38.20), n = 10 | |
Peak non-paretic excitation gastrocnemius | 1.4 (0.81, 3.45), n = 11 | 2.15 (1.58, 5.05), n = 10 | 30.15 (18.54, 39.87), n = 11 | 1.11 (0.23, 3.68), n = 10 | 1.35* (0.01, 7.56), n = 10 | 3.03* (2.03, 12.49), n = 11 | |
Peak non-paretic excitation quadriceps | 4.97 (2.57, 7.62), n = 11 | 7.20 (2.89, 23.94), n = 10 | 14.85 (2.56, 39.08), n = 11 | 3.39* (0.32, 17.03), n = 10 | 6.04* (0.94, 24.05), n = 10 | 7.76* (0.89, 34.64), n = 11 |
Discussion
This comparative study demonstrated that people with lower limb weakness after stroke could achieve measurable displacement and flight times during a JS exercise. They were able to significantly increase their peak concentric velocity and generate more muscle excitation on demand, and this magnitude increased with increasing speed intention. This performance however was not strongly correlated with muscle strength.
To our knowledge, this is the first study to measure flight time in people with hemiplegia after stroke. Previous small studies have measured jump height (or displacement) (Hendrey et al., 2018; Williams et al., 2014a) however the addition of a pressure mat to demonstrate flight time – when combined with displacement – provides evidence that participants are able to achieve the goal of a ‘jump.’ Importantly, in this study, all eleven participants were able to achieve flight phase. Peak concentric velocity between the paretic and non-paretic legs was greater with increased speed intention (FS-SS and JS-SS), and when intending to jump vs. performing the task at maximal speed without jumping (JS-FS). These results establish that the intent to move more quickly, even if a flight phase does not occur, still results in greater peak concentric velocity. Introducing the intention to jump (JS condition) further increases peak concentric velocity above that which is produced when the intention is to perform the task at maximal speed (FS). It is therefore reasonable to consider the inclusion of ballistic exercises into resistance exercise programs after stroke if the aim is to increase movement velocity during training.
Modest associations were found between isometric strength on HHD testing and performance in the ballistic conditions (FS and JS). Although this demonstrates that isometric strength contributes to performance, it indicates that people with isometric leg weakness after stroke may still be capable of successfully completing ballistic exercises. Clinicians may therefore consider the inclusion of these exercises even in patients who have noticeable isometric strength deficits.
Strengths of this study are that it is the first to examine muscle excitation occurring during ballistic exercises in a clinical population. The EMG data demonstrated that muscle excitation of the quadriceps increased as the intended task speed increased (SS-FS), and again at the same intended task speed but with the addition of the intention to jump (FS-JS). This may be clinically relevant, as increased muscle excitation during an exercise may result in improved rehabilitation outcomes. Support for this was provided in a recent systematic review which reported that plyometric exercises might improve muscle coordination and activation during stretch-shortening cycle activities (Ramirez-Campillo, Gentil, Negra, Grgic & Girard, 2021). Further research however is required to determine if this acute increase in excitation during exercise translates to real-world functional gains. Additionally, further exploration of the most appropriate exercises to implement is indicated if the goal is to address walking, given the lack of increased muscle excitation in the paretic gastrocnemius. This interesting finding could reflect the chosen exercise of a single leg squat, where the knee bends and straightens, therefore biasing the quadriceps. A more task-specific exercise, such as a straight knee ‘hop’ that reduces the involvement of the quadriceps may reveal changes in gastrocnemius excitation levels.
Limitations of this study include the relatively small sample size, and the large range of age and stroke chronicity, however this concern was attenuated by the large magnitude of effect of many of the differences observed. For all conditions, the non-paretic leg was tested first to allow task familiarization, which may have resulted in an order effect. A further limitation was missing data. Due to equipment malfunction, the first consented participant was unable to participate in the assessment and data was therefore not collected and they were excluded from reporting. Further, there were six missing EMG data points across three participants. Whilst problematic, we believe that the integration of EMG with a pressure sensing mat and string potentiometer provided sufficiently important and novel mechanistic information to counterbalance this loss. Our EMG data were reported as unitless, instead of the traditional mV format, due to the amplification method of our EMG device. Whilst this does not allow the mV values to be compared to other studies, nor should any raw EMG values be compared across studies given the inherent limitations (Vigotsky et al., 2018). The criterion-reference validity of this EMG system has previously been established (Heywood et al., 2018).
This study provides evidence to demonstrate that people with lower limb muscle weakness after stroke – even those requiring gait aids or orthoses to mobilize – can generate greater peak concentric velocities and increase muscle excitation when instructed to move at maximal vs. self-selected speed, and that this is enhanced further by the intention to jump. Whether this translates to other ballistic exercises remains to be proven, however the results of this study warrant further exploration of this form of resistance training in stroke to determine whether participants can improve their power generation, and what effect this has on improving walking speed and other mobility tasks.
Conclusion
Participants were successful in achieving flight phase (i.e. jumping) and increasing their peak concentric velocity on both their paretic and non-paretic legs on demand. This demonstrates that the addition of ballistic-style exercises into a resistance training program for people with hemiplegia after stroke may help to address difficulties with power generation due to self-selected velocity or low levels of muscle excitation, which is vital when considering the biomechanical demand of speed on walking and mobility tasks.
Acknowledgements
We would like to thank the participants who volunteered their time to take part in this study.
Financial support
This work was supported by an Australian Government Research Training Program Scholarship.
Ethical standards
The authors assert that all procedures contributing to this work comply with the ethical standards of the relevant national and institutional committees on human experimentation and with the Helsinki Declaration of 1975, as revised in 2008.
References
Ada L., Dorsch S., Canning C. G. (2006) Strengthening interventions increase strength and improve activity after stroke: a systematic review. Australian Journal of Physiotherapy 52(4), 241-248.
| Crossref | Google Scholar |
Clark R., Bryant A., Humphries B. (2008) A comparison of force curve profiles between the bench press and ballistic bench throws. Journal of Strength and Conditioning Research 22(6), 1755-1759.
| Crossref | Google Scholar |
Cordner T., Egerton T., Schubert K., Wijesinghe T., Williams G. (2021) Ballistic resistance training: Feasbility, safety and effectiveness for improving mobility in adults with neurologic conditions: A systematic review. Archives of Physical Medicine and Rehabilitation 102(4), 735-751.
| Crossref | Google Scholar |
Cormie P., McGuigan M. R., Newton R. U. (2010) Adaptations in athletic performance after ballistic power versus strength training. Medicine and Science in Sports and Exercise 42(8), 1582-1598.
| Crossref | Google Scholar |
Cormie P., McGuigan M. R., Newton R. U. (2011) Developing maximal neuromusclar power. Part 2 - training considerations for improving maximal power production. Sports Medicine 41(2), 125-146.
| Crossref | Google Scholar |
Eriksrud O., Bohannon R. (2003) Relationship of knee extension force to independence in sit-to-stand performance in patients receiving acute rehabilitation. Physical Therapy 83(6), 544-551.
| Crossref | Google Scholar |
Garcia-Ramos A., Stirn I., Strojnik V., Padial P., De la Fuente B., Arguelles-Cienfuegos J., Feriche B. (2016) Comparison of the force-, velocity-, and power-time curves recorded with a force plate and a linear velocity transducer. Sports Biomechanics 15(3), 329-341.
| Crossref | Google Scholar |
Hendrey G., Clark R., Holland A., Mentiplay B., Davis C., Windfeld-Lund C., Williams G. (2018) Feasibility of ballistic strength training in subacute stroke: A randomized, controlled, assessor-blinded pilot study. Archives of Physical Medicine and Rehabilitation 99(12), 2430-2446.
| Crossref | Google Scholar |
Hermens H., Freriks B., Disselhorst-Klug C., Rau G. (2000) Development of recommendations for SEMG sensors and sensor placement procedures. Journal of Electromyography and Kinesiology 10(5), 361-374.
| Crossref | Google Scholar |
Heywood S., Pua Y. H., McClelland J., Geigle P., Rahmann A., Bower K., Clark R. (2018) Low-cost electromyography – validation against a commercial system using both manual and automated activation timing thresholds. Journal of Electromyography and Kinesiology 42, 74-80.
| Crossref | Google Scholar |
Holden M., Gill K., Magliozzi M. (1986) Gait assessment for neurologically impaired patients: standards for outcome assessment. Physical Therapy 66, 1530-1539.
| Crossref | Google Scholar |
Kahn M. B., Williams G., Mentiplay B. F., Bower K. J., Olver J., Clark R. A. (2021) Upper limb associated reactions: the relationship between movement kinematics and muscle activity in seated versus walking testing. American Journal of Physical Medicine and Rehabilitation 100(3), 235-242.
| Crossref | Google Scholar |
Mentiplay B.F., Perraton L.G., Bower K.J., Adair B., Pua, Y-H., Williams G., McGaw R., Clark RA. (2015) Assessment of lower limb muscle strength and power using hand-held and fixed dynamometry: a reliability and validity study. PLoS One 10, 1-18.
| Crossref | Google Scholar |
Morris S., Dodd K., Morris M. (2004) Outcomes of progressive resistance strength training following stroke: A systematic review. Clinical Rehabilitation 18(1), 27-39.
| Crossref | Google Scholar |
Neptune R. R., Kautz S. A., Zajac F. E. (2001) Contributions of the individual ankle plantar flexors to support, forward progression and swing initiaition during walking. Journal of Biomechanics 34(11), 1387-1398.
| Crossref | Google Scholar |
Ramirez-Campillo R., Gentil P., Negra Y., Grgic J., Girard O. (2021) Effects of plyometric jump training on repeated sprint ability in athletes: A systematic review and meta-analysis. Sport Medicine 51, 2165-2179.
| Crossref | Google Scholar |
Ratamess N. A., Alvar B. A., Evetoch T. K., Housh T. J., Kibler W. B., Kraemer W. J., Triplett N. T. (2009) American college of sports medicine position stand. Progression models in resistance training for healthy adults. Medicine and Science in Sports and Exercise 41(3), 687-708.
| Crossref | Google Scholar |
Umberger B. R., Martin P. E. (2007) Mechanical power and efficiency of level walking with different stride rates. Journal of Experimental Biology 210(18), 3255-3265.
| Crossref | Google Scholar |
Vigotsky A., Halperin I., Lehman G., Trajano G., Vieira T. (2018) Interpreting signal amplitudes in surface electromyography studies in sport and rehabilitation sciences. Frontiers in Physiology 8, 985.
| Crossref | Google Scholar |
Williams G., Clark R. A., Hansson J., Paterson K. (2014) Feasibiliy of Ballistic Strengthening exercises in neurologic rehabilitation. American Journal of Physical Medicine and Rehabilitation 93(9), 828-833.
| Crossref | Google Scholar |
Williams G., Hassett L., Clark R., Bryant A., Olver J., Morris M., Ada L. (2019) Improving walking ability in people with neurologic conditions: A theoretical framework for biomechanics-driven exercise prescription. Archives of Physical Medicine and Rehabilitation 100(6), 1184-1190.
| Crossref | Google Scholar |
Williams G., Kahn M., Randall A. (2014) Strength training for walking in neurologic rehabilitation is not task specific: A focused review. American Journal of Physical Medicine and Rehabilitation 93(6), 511-522.
| Crossref | Google Scholar |