Jeremy David Pickett-Heaps 1940–2021†
Peter Beech
A
B
Abstract
Jeremy Pickett-Heaps was a biologist whose acute observational powers were fed by a deep fascination for how cells work; he had an affinity for the myriad diversity of algae and other protists in general and for what they could teach us about all cells. An early adopter of the electron microscope, he made fundamental discoveries in plant cell division and green algal phylogeny that developed into studies on cell division in general: first with the highly-ordered diatoms and other protists, and later with animal cells. In the latter part of his career, Jeremy mastered time-lapse micro-cinematography to document the dynamic lives of cells and, through the resultant movies and his enthusiastic teaching, introduced many to the wonders of microscopic life.
Keywords: algae, botany, cells, cinematography, diatoms, gliding, mitosis, wheat.
The early years
Jeremy Pickett-Heaps (Fig. 1) was born in Bombay (Mumbai), India in June 1940, to an English mother, Edna Azura (née May), and Australian father, Harold Arthur Pickett-Heaps. Harold ran an import/export company, trading goods around the globe, which he named ‘HA Pickett-Heaps and Sons’ in the hope that Jeremy and other potential siblings might one day take over the business. But, in 1941, under the darkening cloud of World War 2 and Edna now pregnant with Jeremy’s younger brother, Tony, the family sailed back to Sydney, where Edna’s mother lived.
With the conclusion of the war, Harold’s business drew the family back to India, this time to Calcutta, in 1946. There, the family lived a comfortable lifestyle with the boys being tutored in the English tradition. Jeremy and Tony seemed to their tutors and parents to be academically gifted, so much so that their father sent them back to Australia in 1949 to board at The Geelong Grammar School, in Corio, Victoria. Apparently Harold had by this time abandoned any hopes of his sons taking over the family import/export business, and was extremely proud of their early aptitude for studies.
With their mother also back in Australia, Harold built a holiday home at Palm Beach, north of Sydney. Palm Beach was where Jeremy came to love the sea and all it offered: playing in the shallows, swimming, fishing and then surf-boarding, all of which undoubtedly stimulated his powers of observation and continual wonderment with the natural world. It became a font of many of his life-long and fondest childhood memories.
Despite disliking the boarding school life, Jeremy noted in an early resumé several ‘teachers of influence’ at Geelong Grammar, namely K. Mappin (his mathematics teacher whom he still visited in later years) and Marjorie Tunbridge (his biology teacher). On Sundays, after chapel service, when the boys were allowed out from school, Jeremy’s favourite escape was to ride a borrowed bicycle to the nearby You Yang Ranges. It was here that he discovered bird watching, a love that he returned to in retirement. Compounding Jeremy’s love of nature was an adolescent year at the school’s Timbertop campus in the Victorian high country. ‘Timbertop’ was established by Geelong Grammar in the mid-1950s to emulate the experiences for young men of the Outward Bound movement. Jeremy explored the high country as one of the very early students to benefit from this outdoor style of education, then novel in Australia. Back at school in Geelong, he excelled at the sports of rowing and Australian Rules football, as well as his studies. Jeremy was initially absent from his final chemistry exam because he was bird watching; a search party eventually found him and, despite being very late for the exam, he won the ‘exhibition’ in chemistry and was Dux of School.
University of Cambridge 1959–65
On arriving at Cambridge with a scholarship to Clare College in 1959, Jeremy took the natural sciences tripos and then part II in biochemistry. Again, he mastered his studies by ‘not swotting for hours on end’ but rather by enhancing student life with activities such as learning to glide in sailplanes (Fig. 2). An evocative, true story is that he emergency landed on the Kings College cricket ground, much to the groundkeeper’s horror, without, apparently, leaving any damage.
In 1963, he was accepted to undertake a PhD under Donald Northcote (FRS 1961) in the biochemistry department at Cambridge; in three years this resulted in a thesis entitled ‘The function of cell organelles in growth and differentiation in wheat’. These were the early days of electron microscopy and, despite being schooled in wet biochemistry, Jeremy was keen to exploit and stretch the powers of electron microscopy to observe the inner workings of intact cells. He decided to look at cell division in a simple system: wheat seedlings germinated on filter paper on the laboratory window ledge. It was hard to not make ground-breaking discoveries in those early days of electron microscopy, but Jeremy’s advances were truly seminal: he showed that Golgi-derived vesicles contribute material to the growing plant cell wall1 and, in two papers that appeared in the first issue of the Journal of Cell Science, he documented his discovery of the pre-prophase band of microtubules, the (still) somewhat enigmatic predictor of plant cell division planes. He initially described these microtubules in meristematic cells of roots and shoots2 and then confirmed their placement during the asymmetric divisions that give rise to the stomatal complex,3 an arrangement of cells that border the openings on leaf surfaces.
The Australian National University (ANU) 1965–70
Keen to return to Australia, Jeremy accepted a fellowship at the ANU’s John Curtin School of Medicine. It was a well-funded appointment that enabled him to pursue whatever interested him; this he did at speed in the school’s recently established electron microscopy unit. Jeremy was an early user of radioactive tracers and autoradiography and was soon able to confirm his earlier theories on plant cell wall development by tracing polysaccharide-laden Golgi vesicles to the developing plant cell wall.4
Green algae
Little was known, then, of the ultrastructure of algae, largely because their often highly vacuolated cells did not preserve well in the osmium-based fixatives used at the time; but Jeremy had the new fixation weapon of glutaraldehyde, which he would use to great effect on wheat seedlings. Stimulated by discussions with colleagues such as Terry O’Brien and Margaret McCully at the ANU’s Research School of Biological Sciences (to which Jeremy shifted his laboratory on acquiring a fellowship there in 1968), and along with his first post-doc, Larry Fowke, and first PhD candidate, Harvey Marchant, he trialled different concentrations and combinations of fixatives and buffers, and various dehydrating agents, embedding materials and stains to produce material that gave the best-possible representation of cells in life. He also quickly realised the benefits of always using either young, actively growing cultures or material collected directly from the wild: tenets that he emphasised to his students thereafter. As any electron microscopist knows, this is painstaking and often frustrating work, but when you get a ‘fix’ that is good, it is a joy to observe the thin-sections and discover as much as possible from the cellular detail that likely nobody has observed before.
With these well-preserved samples, Jeremy initially published a series of four papers on the fine structure of Chara, the most land-plant-like of the green algae, at all stages of growth and development.5 He then proceeded to investigate, with the members of his young research group, numerous and various other green algae—apparently, anything they could get their hands on. It soon became clear that there were clear delineations within the green algae with respect to the way in which they underwent cytokinesis. Jeremy’s key discovery was the identification of a mode of cytokinesis almost identical to that found in land plants, utilising the ‘phragmoplast’, which took place in only Chara and a few other green algae. Phragmoplasts were absent from the remaining large and diverse groups of green algae, such as Chlamydomonas (Chlamydomonas-like organisms were at the time considered to be the direct ancestors of the land plants). The phragmoplast is a precursor to the formation of a cell wall between daughter nuclei following mitosis: after anaphase, spindle microtubules in the phragmoplast hold the nuclei apart while also directing cytoplasmic vesicles into position for wall formation. The alternative mode of cytokinesis, which Jeremy and his laboratory found in all the other groups of green algae that they studied, involves the formation of a ‘phycoplast’. Jeremy coined this term6 to describe the process whereby the anaphase spindle breaks down and the daughter nuclei, adjacent to one another, become separated by new microtubules that form parallel to the plane of cytokinesis [This is different from phragmoplasts in which microtubules are always oriented perpendicular to the plane of cytokinesis]. The phycoplast functions not only to keep the daughter nuclei apart during division but, like the phragmoplast, plays a role in vesicle accumulation and wall formation. Jeremy recognised the phragmoplast as a fundamental feature of land plant development, and its presence in only a small group of green algae made those algae ancestral to the higher plants. Subsequent studies over the following decades, most recently from genetic sequencing data, have confirmed Jeremy’s initial findings as being pivotal in understanding the origins of land plants. His work on many major groups of green algae eventually led him to propose a different phylogeny for the Chlorophyta,7 and he continued to work on green algae even after retirement, producing many exceptional time-lapse videos of the structure, development and reproduction in green algae.
University of Colorado, Boulder 1970–88
Near the end of his fellowships in Canberra, Jeremy was invited by Keith Porter, one of the pioneers of electron microcopy and cell biology, to be an early faculty member at the Department of Molecular, Cellular and Developmental Biology at the University of Colorado, Boulder. This was a perfect place for Jeremy to continue his career: it had one of the two high-voltage electron microscopes in the US at the time, and enabled Jeremy to combine his love of the outdoors while having his own laboratory in an outstanding department. Jeremy continued his green algal work, this time collecting his samples from the Rocky Mountain streams around Boulder.
He amassed such a volume of knowledge that he published this as Green Algae:8 an assembly, in large format book form, of his accumulated thoughts around ‘the phylogeny of cell structures’ borne mainly out of his own published works and supplemented with that of others and with many beautiful electron micrographs (Fig. 3, for example). This was in 1975, when he was thirty five years old: to have stepped into and mastered this field of biology largely unmentored, except by the literature and the organisms, was remarkable.
Cell cleavage and autospore formation in Tetraedron bitridens: phycoplast microtubules (ph), cleavage furrow (cf), centriole (cn), Golgi body (g), parental wall (pw) and pyrenoid (py) (from Pickett-Heaps 1975).
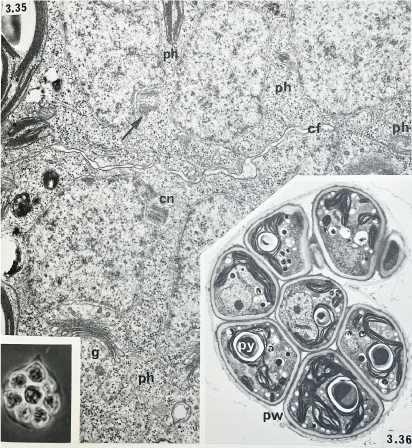
Diatoms
While continuing his interest in green algal evolution and ultrastructure, in the mid-1970s, Jeremy also turned his insight and curiosity to another group of algae, the diatoms. His specific interests centred around diatom cell division, the structures and processes involved in diatom cell wall morphogenesis, and around the mechanisms behind diatom motility. Members of his laboratory were constantly getting water samples from various ponds and creeks in the area to check for diatoms and other algae and brought samples even from far-away places that group members would go to on vacation; one pond in Boulder continues to be a source for diatom research to this day. Jeremy was always captivated by the early microscopy work of the botanist Robert Lauterborn and his exquisitely detailed drawings of the stages of algal development. In an effort to remind the community of often-forgotten great, past works, in 1984 he co-published a translation of Lauterborn’s 1896 treatise, demonstrating how modern optical and electron microscopy simply confirmed the excellent work of Lauterborn9 in understanding the nature of diatom mitosis.10
Jeremy was a master of the transmission electron microscope and the all-important methods that lie behind the generation of a final image of a preserved cell that best reflects the in vivo state. It was noted earlier how he developed techniques for observing the fine structure of green algae and so, too, with diatoms he was able to make significant advances. The hardened silica cell walls of diatoms makes the generation of thin sections of the cells for transmission electron microscopy difficult. Jeremy’s laboratory became expert at generating serial sections of fixed samples to reconstruct the internal cell organisation of diatoms many years before any type of 3D computer reconstruction was possible. This type of analysis became crucial in helping to understand the structure of diatom mitotic spindles (and mitotic spindles in general). In contrast to animal spindles, the highly ordered and localised spindles of diatoms, which are cylindrical and tightly packed, allowed Jeremy and graduate student, David Tippit, to follow the profiles of individual microtubules through an entire spindle by painstakingly comparing the micrographs of one section to those of the adjacent section. This work established that the spindle is in fact composed of two half spindles that interdigitate and overlap in the central region, and that the sliding apart of these interdigitated half-spindles accomplishes much of the pole-pole separation (anaphase B) that occurs during mitosis in diatoms.11 The work also showed that the diatom centrosome, in contrast to that found in most animal cells, is not needed to maintain the organisation of mitotic spindles, but rather is involved in directing microtubules to help in the organisation and placement of cellular structures that form during cell division.12
Jeremy was keenly interested in how chromosomes accurately attach to the mitotic spindle, thus ensuring faithful separation of genetic material during mitosis. He considered proper attachment of the chromosomes to the spindle during prometaphase to be a seminal event in the evolution of all eukaryotic life. Once again, the diatom spindle helped to elucidate the basic functioning of the kinetochore during prometaphase when each chromosome pair attaches to the mitotic spindle. With the use of light and electron microscopy, it was determined that kinetochores function primarily by interacting with and sliding along microtubules from the poles. At prometaphase, one kinetochore of a chromosome pair moves toward either pole, while the second kinetochore is responsible for moving away from that pole, thus ensuring proper separation of the genetic material.13
Time-lapse recording
Jeremy operated with his usual enthusiasm to go beyond the static light or electron microscopic images and worked with the people in his group to record real-time and time-lapse images of numerous diatom processes. This included using 16 mm cine recordings to capture time-lapse well before video or digital time-lapse recording was easily available. By using perfusion chambers to introduce fixatives and drugs while making the live cell observations, then taking electron microscopic images of the fixed and treated cells, Jeremy and his co-workers were able to correlate the presence and localisation of specific cellular structures with dynamic processes. Such methods showed, for example, that a key role of the diatom microtubule organising centre (centrosome) was to help orchestrate the formation of the new cell walls following division.14 Drug treatments of developing diatoms also suggested the requirement for actin as well as microtubules in the localisation of the developing components of the diatom cell wall. Drug perfusion suggested that the sliding of half-spindles in anaphase B actively required energy input, while the movement of split chromosomes to poles (anaphase A) did not.15 Moreover, correlation of live video from high energy ultraviolet light ablation of sections of the diatom spindle, with subsequent electron microscopy by Roger Leslie in the laboratory, suggested that the two interdigitated half-spindles of mitosis were each composed of uniformly oriented microtubules, and that rates of microtubule depolymerisation were distinctly different at the two ends of microtubules.16
Being acutely interested in cell dynamics, Jeremy also had the opportunity to work with Lesley Edgar, a visiting post-doc in his group, to investigate the unresolved problem of gliding motility in diatoms. Using a combination of live microscopy and electron microscopy of the underlying cell ultrastructure, the Edgar/Pickett-Heaps model for motility was developed, in which underlying actin cables provide structure over which trans-cellular components (using myosin) can move down the raphe fissure (a slit in the cell wall of many benthic diatoms) to provide movement.17 While there are still details that remain unresolved, more than forty years later this model continues to be the major working hypothesis for understanding the cellular movement of diatoms with raphes.
As the 1980s progressed, Jeremy found that procuring funding was becoming increasingly difficult in the US, particularly for those cell structure and dynamics labs like his that were not focused on the molecular and genetic aspects of cells. The increasing pressure to obtain external funding sources to continue his work, and his desire to return home to Australia, led to him taking a position at the University of Melbourne in 1988.
University of Melbourne 1988–
Jeremy was hired in 1988 as head of the School of Botany, where he had spent a productive sabbatical with Richard (Rick) Wetherbee in 1984 and very much endeared himself to the students in Rick’s group. There, Jeremy continued to welcome numerous visitors and colleagues to his laboratory, where he encouraged and fostered further ground-breaking work in algal cell biology. For example, a visit in the early 1990s by one of his former PhD students from Boulder, Stan Cohn, prompted a reinvestigation of the diatom motility response18 and reinvigorated investigations into understanding the effects of light on diatom movement.
Animal mitosis
Another visitor to the laboratory in the early 1990s was this Memoir’s second author, Arthur (Art) Forer (Fig. 4) and this led to a collaboration studying animal cell mitosis that was to last some fifteen years. Jeremy’s doctoral studies on cell division and preprophase bands naturally led him to become interested in the workings of mitosis, the separation of chromosomes via the mitotic spindle that happens before division of the cell. At that time, electron microscope studies of mitotic spindles showed that microtubules extend between each chromosome and the spindle pole towards which the chromosome moves; most observers considered that these ‘kinetochore’ microtubules produced the forces that moved the chromosomes poleward. Jeremy was no exception: he studied spindle microtubules in dividing diatoms and other algae, trying to understand how they produced force. Experiments with Tim Spurck (a research assistant who later became his graduate student) in the early 1980s,19 on the diatom Hantzschia, told him that kinetochore microtubules did not produce force but instead acted as guides for the direction in which the chromosomes should move and that the forces that moved the chromosomes were rather produced by a ‘spindle matrix’. In normal Hantzschia cells, kinetochore microtubules are embedded in electron-dense material. He and Spurck depolymerised kinetochore microtubules with colchicine. Spindle structure was maintained when the kinetochore microtubules were depolymerised because the two spindle poles were connected by a separate bundle of microtubules that were not depolymerised by colchicine. When the kinetochore microtubules disappeared, each chromosome moved randomly to one of the two poles (illustrated in Fig. 5). Jeremy and Spurck concluded that something other than microtubules propelled the chromosomes to the poles, a spindle matrix in the form of the electron dense material associated with the kinetochore microtubules. They concluded that microtubules act to guide chromosomes to move in the proper direction and also act as ‘governors’ of speed (since the rate of chromosome movement in untreated cells is governed by the rate of microtubule depolymerisation). This key experiment on diatom mitosis was a basis for Jeremy’s thinking about mitotic mechanisms as he began experiments on animal cells.
After lunch at the Danish Club in Melbourne, ~1995. From L-R: Tim Spurck, Sandra Forer, Art Forer, Jeremy Pickett-Heaps, Julianne Pickett-Heaps. Photographer unknown.
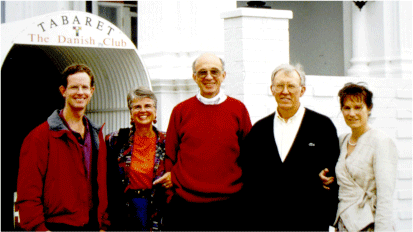
The four electron micrographs are illustrations of mitotic cells before colchicine addition (Image a) and after colchicine addition (Image b); the two images at the bottom are at higher magnification, illustrating the kinetochores at the poles after removal of their connecting microtubules. The kinetochore arrangements at the poles allowed Pickett-Heaps and Spurck to deduce what happened, as illustrated in the coloured cartoons of control and colchicine-treated cells (Pickett-Heaps and Spurck 1982a). In control cells, each chromosome at metaphase is composed of two chromatids that at anaphase split into two chromatids that then move to opposite poles. After colchicine treatment, on the other hand, the chromosomes did not split into two but each one, consisting of two chromatids, moved to one of the two poles at random (Adapted from Pickett-Heaps and Forer 2009).
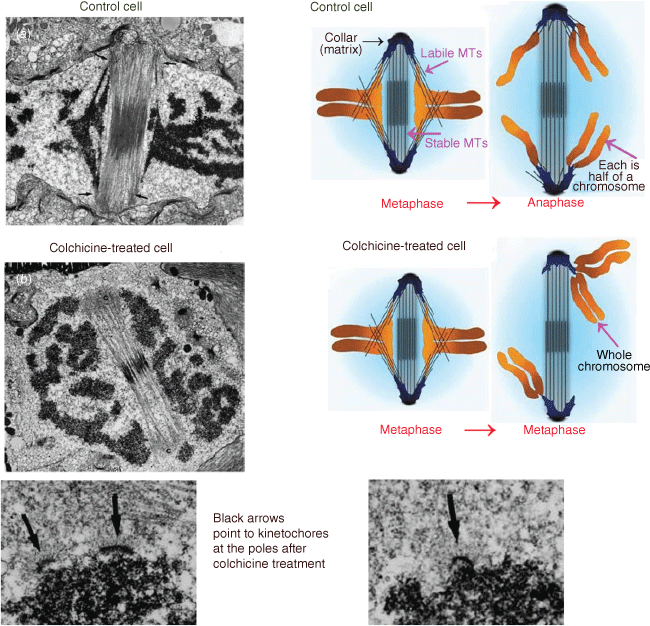
Jeremy began to work with mitotic animal cells around 1985, perhaps to make his interpretations derived from Hantzschia and other ‘odd’, ‘unusual’, non-canonical cells more relevant to colleagues who considered that mitosis occurs only as seen in ‘standard’ animal cells. His early experiments used tissue-cultured marsupial (PtK) cells;20 later he studied cultured newt lung cells. The kinetochore microtubules in these spindles are not embedded in electron-dense material (that is, there is no visible ‘matrix’) but experiments using an focused ultraviolet light to damage kinetochore microtubules confirmed that a spindle matrix functions to move chromosomes in animal cells just as in diatoms. The experiments were these.
Jeremy and colleagues monitored the presence of spindle microtubules in living cells using polarisation microscopy. When a slit-shaped beam of ultraviolet light irradiated across the newt or PtK-cell spindle between the chromosomes and a pole, the birefringence (microtubules) disappeared in the irradiated region, severing the spindle into two pieces—a polar region with microtubules extending toward the equator but ending blindly in the irradiated region, and the rest of the spindle not attached the severed part. The rest of the spindle seemed ‘normal’: severed microtubules extended in one direction from the chromosomes, towards one pole, and ended blindly in the irradiated region while the non-severed microtubules extended to the pole in the other direction and connected the chromosomes to the other pole. Shortly after the microtubules were severed, the ‘amputated’ pole moved towards the chromosomes at the equator. Since this movement occurred in the absence of microtubule connections across the irradiated region, the movements were not caused by microtubules but rather forces for movement were produced by an underlying spindle ‘matrix’. In the article describing these results,21 however, Jeremy and colleagues suggested that the movements of the poles to the equator arose from growth of additional microtubules from the pole; they did not discuss the possibility that movement arose from a spindle matrix. Forer worked in Jeremy’s laboratory in 1993, as a spindle matrix skeptic, and the day he entered Jeremy’s laboratory was challenged by Jeremy with: ‘what causes the pole to move toward the equator when the connecting microtubules are cut?’ Jeremy argued that there must be something underlying the microtubules (a spindle matrix) pulling the pole toward the equator. Challenging Forer forcefully in this way, several times, meant that regardless of what Jeremy wrote in the articles, he personally found this experiment convincing of spindle matrix activity. He did not convince Forer at that time that a spindle matrix produced forces to move chromosomes in animal cells, but he did so later.
One key result arose from using focused ultraviolet light to sever individual kinetochore fibre microtubules during anaphase. Severing kinetochore microtubules should stop the associated chromosomes from moving if the microtubules produce the force, but they did not stop. In newt lung cells, for example, not only did the associated chromosome not stop moving, it accelerated.22 This is exactly what one would expect if an invisible matrix pushed the chromosome poleward: because severing microtubules removes the need to depolymerise them in order for chromosomes to move, cutting the microtubules removes the speed limitation so the movements should accelerate.23 These and related experiments convinced Forer that there were force producers (a spindle matrix) underlying the spindle microtubules. Alas, most colleagues still are not convinced that a spindle matrix or anything other than spindle microtubules produce force.
Some colleagues have accepted that a spindle matrix underlies the microtubule spindle structure, but they say its role is in transporting essential regulatory components;24 only a few considered it to have a possible role in chromosome movement.25 To look for additional evidence, Jeremy and colleagues studied non-microtubule spindle components that might produce forces. The two major motile force producers for intracellular movements are (a) microtubules and their associated motor proteins, and (b) actin and its motor protein myosin. If not microtubules, why not actin? Jeremy and colleagues had found actin in various spindles, including in Oedogonium,26 and they used actin inhibitors to alter mitotic processes.27 From these and other data Jeremy argued that spindles incorporated actin and myosin when they evolved from primitive motile systems.28 Our colleagues by and large remain unconvinced that actin is involved.
Red algae
In the mid-1990s, Jeremy began another important collaboration, with John West, an emeritus professor of phycology who, at that time, had recently moved to Melbourne’s School of Botany from UC, Berkeley. John brought to Melbourne a large collection of red algal cultures and Jeremy immediately pounced on this treasure trove and began filming various unicells and the reproductive spores from multicellular species. Red algae were assumed to have no motile stages because members of the phylum universally lacked flagella. There were a few unsupported claims of motility in the early literature, but it wasn’t until Jeremy filmed the activity of these cells while keeping them alive for many hours in a perfusion chamber that these reports could be confirmed. Of the more than twenty five species that Jeremy filmed, most turned out to have motile stages. These findings were significant because, at the time, phycologists had little to no understanding of red algal motility and the movements shown in his recordings were potentially critical to the survival of species by enabling optimisation of sites for spore germination. As surprising as these findings were, Jeremy’s microcinematography of sexual reproduction in red algae was truly amazing: from spermatial release to nuclear migration and fertilisation, he again observed and beautifully recorded dynamic cellular events that were previously unknown.29
Microcinematography
Jeremy was a great proponent and practitioner of observing living cells, not least to validate what he saw in static electron micrographs. His progression into the world of time-lapse imaging, first using analogue and later using digital microcinematography, can be seen as a complement to his many achievements in electron microscopy. His reasoning for this progression to live imaging may be best summarised by this quote from the introduction to his commercial website Cytographics,30 which he started in the mid 1990s with his wife, Julianne:
As a young scientist, I became fascinated by electron microscopy. In the late sixties, this technique was dramatically transforming our concept of cellular structure. I concentrated upon cell division and morphogenesis in green algae and then diatoms. As this work progressed, I became increasingly frustrated at trying to recreate dynamic cellular events solely from static images. A turning point in my career came when I first saw the extraordinary sight of a live diatom undergoing mitosis at high magnification. After borrowing a 16 mm time-lapse camera, I was soon filming algae doing all the things I had studied with the electron microscope. Since then, I have built up a laboratory devoted to the high-resolution video imaging and recording of all sorts of cells and microscopic organisms going about their complex and extraordinary lives.
This transition to live cell imaging might seem easy when compared to the many innovative techniques he successfully developed for the preparation of plant and algal cells for transmission electron microscopy. But similar innovations were required to prepare living cells for surviving and performing naturally during long periods of time-lapse filming under bright lights, particularly the processes of cell division and morphogenesis for which his resulting films and videos are famous. To this end, Jeremy and Tim Spurck developed the aforementioned micro-chambers to facilitate the high-resolution filming of live cells, including chambers with a continuous flow of media to replenish the cells. Having successfully achieved that, they formed collaborations with a number of local electronic experts to develop a shutter system that acted in concert with the time-lapse apparatus and camera so that cells were only subjected to the light (and resulting heat) for the limited time required for a single frame to be exposed. The only limitation was the need to refocus over long periods of time, as even the best microscopes gradually fade out of focus with time. His students and colleagues remember his mad dashes back to the laboratory after dinner, and into the night, to refocus the microscope during time-lapsed sequences that could last up to 18 h or more, also replenishing the media bottle supplying nourishment to the cells being filmed. Like all of Jeremy’s endeavours, he sought perfection, and he endlessly worked towards finding better ways to keep cells alive and functioning normally, as well as improving the technology for time-lapse microscopy.
In addition to his scientific discoveries published in a range of cell biological and phycological journals, Jeremy was equally interested in inspiring a much broader audience with the wonders and diversity of cells. It was with this impetus that Cytographics was launched. A series of DVDs were produced that have pleased teachers, students and naturalists the world over. DVDs produced include those designed for introductory biology courses, such as Living Cells: Structure, Function and Diversity; for specialists taking courses in phycology or protistology, such as Diatoms: Life in a Glass House and Reproduction in the Red Algae; or cell biology titles such as The Dynamics and Mechanics of Mitosis.
Later in his career, Jeremy collaborated with a former student and friend, Drew Berry (now lead animator at the Walter and Eliza Hall Institute’s WEHI-TV), to combine living-cell sequences with dazzling animations. The link to an edited selection of the many movies made by Jeremy, Tim and Drew is included in Supplementary material.
Many of Jeremy’s long-held and sometimes prescient thoughts around the state of cell biology are documented in the Introduction to Green Algae.31 He despised ‘the dominance of animal and other highly evolved cells in formulating theories and dogmas in cell biology… it is an unbalanced approach, quite dangerous and beset with pitfalls’. A good example is that centrioles, at that time (and, in some quarters, still), were considered by text books to be an essential organiser of the mitotic spindle, yet ‘discerning students and teachers, who perforce must swallow these dogmas, cannot help being mystified by the ability of plant cells to divide perfectly well without centrioles’. He championed the algae as organisms complementary to the animals and plants for studies of cell biology: ‘I believe that algae in general represent a fascinating and vast range of organisms whose potential for the study of fundamental processes of life has been largely unexploited …’, and, ‘this book … hopefully supports my contention that these organisms should command more interest from biologists because they provide excellent material for the deeper investigation of diverse biological phenomena.’
Jeremy’s early work was readily accepted by the field; his later work on spindle matrix and actin was not. Part of the reason may be that much of the work involved ‘crazy’ ideas derived from studies of diatoms and crane-fly spermatocytes, both cell types with non-canonical mitoses, in a world where much of the important research on stereotypical animal cells is directed at medical applications. Also, colleagues may not appreciate how good an observer Jeremy was: one may not understand his conclusions if one is not used to scrutinising cell and organelle behaviour as Jeremy did. Nor are many willing to change direction to consider alternate interpretations when confronted with contrary data from the cell, as Jeremy did.
Family life and Jeremy the man
It was at Cambridge that Jeremy met his first wife, Daphne (‘Chi’, pronounced as the Greek letter is) Charmian Scott, an English girl who worked in a coffee shop he frequented. They shared an affinity for the outdoors, with adventure being an important part of life, and married in 1965 before moving to Canberra soon after. Jeremy often reminisced about the late 1960s in Canberra with his young family (David was born in 1967, Rebecca in 1969). As well as continuing to glide, he recalled that days of discovery in the laboratory were followed by summer evening barbecues with Chi and the children by Lake Burley Griffin, or weekends picnicking beside a mountain stream. The fresh waters which Jeremy fished and explored with his family, and even Sullivan’s Creek flowing through the university campus, were brimming with diverse and mostly green algae, and one can imagine him wondering what these organisms would reveal under the electron microscope. On moving to Boulder in 1970, weekends were again filled with hiking, fly fishing and gliding, and winters offered the bonus of down-hill skiing, in which he was more than proficient.
In the summer of 1971, the family was beset with tragedy. Jeremy celebrated his 31st birthday by taking a newly acquired glider to the air in the fields around Boulder. The sailplane must have seemed in good order, so Chi—who was herself learning to glide—took her first solo flight that day. In what was surely a moment and then seconds of horror, Jeremy witnessed the glider loose a tail fin and plummet to the ground. Chi was killed. The only solace Jeremy could take from this dreadful event was that she was likely to have lost consciousness as soon as the aircraft began to fall. In the immediate aftermath of Chi’s death, Jeremy sought refuge in his work and, in the following year, produced a large number of mostly single-author papers on the comparative structure and phylogeny of green algae.
Now a widower with two young children, Jeremy needed support in order to continue his research career; this is how he met his second wife Julianne (Julie) Francis. She was recovering from her own personal tragedy with the death of her husband in a car accident in Michigan soon after the birth of their first child, Aaron. Julie had moved to Boulder and was staying with her aunt, a neighbour of Jeremy’s, when she offered to help him with the children. Together they coped well with the difficulties life was throwing at them and a deep commitment gradually developed. They married in 1977 when they decided to have a child of their own. Christopher was born in 1978.
In 2002 Jeremy retired from his official duties as head of Botany at the University of Melbourne, but he maintained his beloved research practice as a Senior Research Fellow, and began to spend more and more time at Mallacoota, in far eastern Victoria, where he and Julie had recently acquired a seaside home. Days at Mallacoota were busy: when he and Julie were not fishing, gardening or entertaining family, friends or ex-colleagues, they were editing the many accumulated hours of time-lapse footage to produce the educational videos for Cytographics. And it was at Mallacoota, with sea to one side and national park forest to the other, that Jeremy’s schoolboy past-time of bird watching was reinvigorated, now with the added technology of videography. In this, he once again produced a number of memorable DVDs that he would supply to other twitchers on request, and which he even made available at cost price in the local news agency!
It was in 2006 that Jeremy first showed the signs of Parkinson’s Disease. Thankfully, progression was slow and he could continue for many years—around a decade—to pursue his hobbies and live at home. Eventually, he and Julie moved to Canberra to be closer to medical facilities, and it was there—in a somewhat circular footnote to his well-travelled life—that he passed away in 2021.
Jeremy was not only a gifted scientist but an engaging and inspirational teacher. His lectures were enthralling, and they motivated many to pursue research careers, often in his laboratory. Undergraduates were generally unaware of Jeremy’s achievements in cell biology, but they knew they were listening to someone special whose excitement for the discoveries made sometimes decades earlier had not dimmed with time. He illustrated his lectures not with overcrowded and wordy slides but with an enthralling selection of electron micrographs and clips carefully chosen from his many hours of time-lapse video-microscopy. Jeremy imparted such a deep sense of wonder and enthusiasm for the microscopic world, and astonishment at the beautiful diversity of protists, that his teaching had a lasting impact on many of those lucky enough to experience it.
For Jeremy’s research students, joining his laboratory and learning from his instruction and insights was a rich and happy experience. He gave his students the freedom to design and run their own experiments, while always being present to counsel or correct. He rejoiced in their successes and gently guided when motivation was needed. Perhaps due to the tragic loss of his first wife, Jeremy was acutely aware of the need to develop a laboratory that balanced work and life issues. Stanley Cohn recalls that everyone in the group shared in the joys and pains of other members, and always took time to enjoy life outside the laboratory. This included numerous parties at Jeremy’s house where he would often entertain by his proficient piano playing of ragtime and blues, annual trips up through Rocky Mountain National Park to have a picnic and hike at Grand Lake, or the summer ‘Frosty Friday’ ritual of making homemade ice cream to end each week.
Jeremy is missed not just for his brilliance as an observer of biology and his sharp mind, but for his infectious enthusiasm for the wonders of the natural world and kindness to his fellow man. All of us, and we believe that ‘us’ is likely to include all those who worked with him, feel very fortunate to have been a part of his remarkable life.
Acknowledgements
The authors gratefully acknowledge input to and critical reading of the manuscript by Julianne Pickett-Heaps and Brian Gunning FRS. We also thank the significant contributions from Jeremy’s friend and colleague, Rick Wetherbee (University of Melbourne) and students Drew Berry (Walter and Eliza Hall Institute), Stanley Cohn (de Paul University) and Sarah Wilson (La Trobe University). A link to a video supplement containing edited versions of many movies made by Jeremy and Tim is provided in the Supplementary material for this biographical memoir, where a complete list of Pickett-Heaps’ publications may also be found.
References
Cohn, S. A., and Pickett-Heaps, J. D. (1988) The effects of colchicine and dinitrophenol on the in vivo rates of anaphase A and B in the diatom Surirella, European Journal of Cell Biology, 46, 523-530.
| Google Scholar | PubMed |
Cohn, S. A., Nash, J., and Pickett-Heaps, J. D. (1989) The effect of drugs on diatom valve morphogenesis, Protoplasma, 149, 130-143.
| Crossref | Google Scholar |
Cohn, S. A., Spurck, T. P., and Pickett-Heaps, J. D. (1999) High energy irradiation at the leading tip of moving diatoms causes a rapid change of cell direction, Diatom Research, 14, 193-206.
| Crossref | Google Scholar |
Forer, A., and Pickett-Heaps, J. D. (1998) Cytochalasin D and latrunculin affect chromosome behaviour during meiosis in cranefly spermatocytes, Chromosome Research, 6, 533-549.
| Crossref | Google Scholar | PubMed |
Forer, A., Spurck, T., and Pickett-Heaps, J. D. (2007) Actin and myosin inhibitors block elongation of kinetochore fibre stubs in metaphase crane-fly spermatocytes, Protoplasma, 232, 79-85.
| Crossref | Google Scholar | PubMed |
Johansen, K. M., Forer, A., Yao, C., Girton, J., and Johansen, J. (2011) Do nuclear envelope and intranuclear proteins reorganize during mitosis to form an elastic, hydrogel-like spindle matrix? Chromosome Research, 19, 345-365.
| Crossref | Google Scholar | PubMed |
Leslie, R. J., and Pickett-Heaps, J. D. (1984) Spindle microtubule dynamics following ultraviolet microbeam irradiations of mitotic diatoms, Cell, 36, 717-727.
| Crossref | Google Scholar | PubMed |
Northcote, D. H., and Pickett-Heaps, J. D. (1966) A function of the Golgi apparatus in polysaccharide synthesis and transport in the root-cap cells of wheat, Biochemical Journal, 98, 159-167.
| Crossref | Google Scholar | PubMed |
Pickett-Heaps, J. D. (1966) Incorporation of radioactivity into wheat xylem walls, Planta, 71, 1-14.
| Crossref | Google Scholar | PubMed |
Pickett-Heaps, J. D. (1967a) The use of radioautography for investigating wall secretion in plant cells, Protoplasma, 64, 49-66.
| Crossref | Google Scholar |
Pickett-Heaps, J. D. (1967b) Further observations on the Golgi apparatus and its function in cells of the wheat seedling, Journal of Ultrastructure Research, 18, 287-303.
| Crossref | Google Scholar |
Pickett-Heaps, J. D. (1967c) Ultrastructure and differentiation in Chara sp. I Vegetative cells, Australian Journal of Biological Sciences, 20, 539-552.
| Crossref | Google Scholar |
Pickett-Heaps, J. D. (1967d) Ultrastructure and differentiation in Chara sp. II Mitosis, Australian Journal of Biological Sciences, 20, 883-894.
| Crossref | Google Scholar |
Pickett-Heaps, J. D. (1967e) Ultrastructure and differentiation in Chara sp. III Formation of the antheridium, Australian Journal of Biological Sciences, 21, 255-274.
| Crossref | Google Scholar |
Pickett-Heaps, J. D. (1967f) Ultrastructure and differentiation in Chara sp. III Formation of the antheridium, Australian Journal of Biological Sciences, 21, 255-274.
| Crossref | Google Scholar |
Pickett-Heaps, J. D. (1968) Further ultrastructural observations on polysaccharide localization in plant cells, Journal of Cell Science, 3, 55-64.
| Crossref | Google Scholar | PubMed |
Pickett-Heaps, J. D. (1972) Variation in mitosis and cytokinesis in plant cells: Its significance in phylogeny and evolution of ultrastructural systems, Cytobios, 5, 59-77.
| Google Scholar |
Pickett‐Heaps, J. (1983) Valve morphogenesis and the microtubule center in three species of the diatom Nitzschia, Journal of Phycology, 19(3), 269-281.
| Crossref | Google Scholar |
Pickett-Heaps, J., and Forer, A. (2009) Mitosis: spindle evolution and the matrix model, Protoplasma, 235, 91-99.
| Crossref | Google Scholar | PubMed |
Pickett-Heaps, J. D., Forer, A., and Spurck, T. (1997) Traction fibre: toward a “tensegral” model of the spindle, Cell Motility and the Cytoskeleton, 37, 1-6.
| Crossref | Google Scholar | PubMed |
Pickett-Heaps, J. D., and Northcote, D. H. (1966a) Relationships of cellular organelles to the development and formation of the plant cell wall, Journal of Experimental Botany, 17, 20-26.
| Crossref | Google Scholar |
Pickett-Heaps, J. D., and Northcote, D. H. (1966b) Organization of microtubules and endoplasmic reticulum during mitosis and cytokinesis in wheat meristems, Journal of Cell Science, 1, 109-120.
| Crossref | Google Scholar | PubMed |
Pickett-Heaps, J. D., and Northcote, D. H. (1966c) Cell division in the formation of the stomatal complex of the young leaves of wheat, Journal of Cell Science, 1, 121-128.
| Crossref | Google Scholar | PubMed |
Pickett-Heaps, J. D., and Northcote, D. H. (1966d) Cell division in the formation of the stomatal complex of the young leaves of wheat, Journal of Cell Science, 1(1), 121-128.
| Crossref | Google Scholar |
Pickett-Heaps, J. D., and Spurck, T. P. (1982a) Studies on kinetochore function in mitosis. I. The effects of colchicine and cytochalasin on mitosis in the diatom Hantzschia amphioxys. European Journal of Cell Biology, 28(1), 77-82.
| Google Scholar | PubMed |
Pickett-Heaps, J. D., and Spurck, T. P. (1982b) Studies on kinetochore function in mitosis. II. The effects of metabolic inhibitors on mitosis in the diatom Hanzschia amphioxys, European Journal of Cell Biology, 28(1), 83-91.
| Google Scholar |
Pickett-Heaps, J. D., and Tippit, D. H. (1978) The diatom spindle in perspective, Cell, 14(3), 455-467.
| Crossref | Google Scholar |
Pickett-Heaps, J. D., Schmid, A. M., and Tippit, D. H. (1984) Cell division in diatoms: a translation of part of Robert Lauterborn’s treatise of 1896 with some modern confirmatory observations, Protoplasma, 120, 132-154.
| Crossref | Google Scholar |
Pickett-Heaps, J. D., Tippit, D. H., and Leslie, R. (1980) Light and electron microscopic observations on cell division in two large pennate diatoms. Hantzschia and Nitzschia. II. Ultrastructure. European Journal of Cell Biology, 21(1), 12-27.
| Google Scholar | PubMed |
Pickett-Heaps, J. D., Tippit, D. H., and Porter, K. R. (1982) The diatom spindle in mitosis, Cell, 29, 729-744.
| Google Scholar |
Sampson, K., and Pickett-Heaps, J. D. (2001) Phallacidin stains the kinetochore region in the mitotic spindle of the green algae Oedogonium spp. Protoplasma, 217, 166-176.
| Crossref | Google Scholar | PubMed |
Sampson, K., Pickett-Heaps, J. D., and Forer, A. (1996) Actin is involved in chromosomal attachment to the spindle and possibly anaphase A movement of chromosomes in the green alga Oedogonium, Protoplasma, 192, 130-144.
| Crossref | Google Scholar |
Spurck, T., Forer, A., and Pickett-Heaps, J. (1997) Ultraviolet microbeam irradiations of epithelial and spermatocyte spindles suggest that forces act on the kinetochore fibre, and are not generated by its disassembly, Cell Motility and the Cytoskeleton, 36, 136-148.
| Crossref | Google Scholar | PubMed |
Spurck, T. P., Pickett-Heaps, J. D., and Klymkowsky, M. W. (1986a) Metabolic inhibitors and mitosis. I. Effects of dinitrophenol/deoxyglucose and nocodazole on the live spindle, Protoplasma, 131, 47-59.
| Crossref | Google Scholar |
Spurck, T. P., Pickett-Heaps, J. D., and Klymkowsky, M. W. (1986b) Metabolic inhibitors and mitosis. II. Effects of dinitrophenol/deoxyglucose and nocodazole on the microtubule cytoskeleton, Protoplasma, 131, 60-74.
| Crossref | Google Scholar |
Spurck, T. P., Stonington, O. G., Snyder, J. A., Pickett-Heaps, J. D., Bajer, A., and Mole-Bajer, J. (1990) UV microbeam irradiations of the mitotic spindle. II. Spindle fiber dynamics and force production, The Journal of cell biology, 111, 1505-1518.
| Crossref | Google Scholar | PubMed |
Wilson, S. M., Pickett-Heaps, J. D., and West, J. A. (2002) Fertilization and the cytoskeleton in the red alga Bostrychia moritziana (Rhodomelaceae, Rhodophyta), European Journal of Phycology, 37, 509-522.
| Crossref | Google Scholar |
Yao, C., Wang, C., Li, Y., Zavortink, M., Archambault, V., Girton, J., Johansen, K. M., and Johansen, J. (2018) Evidence for a role of spindle matrix formation in cell cycle progression by antibody perturbation, PLoS One, 13(11), e0208022.
| Crossref | Google Scholar | PubMed |
Footnotes
† This biographical memoir is republished with minor edits from Biographical Memoirs of Fellows of the Royal Society, Volume 77, http://doi.org/10.1098/rsbm.2023.0017.
4 Pickett-Heaps (1966, 1967a, 1967b, 1968).
5 Pickett-Heaps (1967c, 1967d, 1967e, 1967f).
6 Pickett-Heaps (1972, 1975).
11 The work was subsequently reviewed in Pickett-Heaps and Tippit (1978).
30 http://www.cytographics.com/jer.html, viewed June 2024.