Ian McDougall 1935–2018
David Phillips
A
Abstract
Ian McDougall was a renowned Earth scientist who gained worldwide distinction for his research in the fields of K-Ar and 40Ar/39Ar geochronology. He was born in Hobart, Tasmania and obtained a BSc (Hons) at the University of Tasmania and a PhD at the Australian National University in Canberra. Following his PhD, he was introduced to the emerging field of K-Ar geochronology during a postdoctoral year at the University of Berkeley in 1961. On his return to Australia, Ian accepted a position in the newly established K-Ar laboratory at the Australian National University. He pioneered the application of the K-Ar dating method to young volcanic rocks and played a pivotal role in developing the geomagnetic timescale. These findings provided crucial evidence in support of the ‘hot spot’ (mantle plume) model and the emerging theory of plate tectonics. He subsequently established the 40Ar/39Ar geochronology method at the Australian National University and gained an international reputation for meticulous experimental work. In the 1980s, he resolved a significant controversy with regard to the age of hominin fossils and artefacts in the Turkana Basin, Kenya. Over the following four decades, he developed a comprehensive geochronological framework for volcanism across the Turkana Basin, providing the basis for current interpretations of early human evolution in the region. Other notable collaborative accomplishments include the application of the 40Ar/39Ar method to thermal history studies and noble gas geochemistry studies of volcanic glasses and mantle material, which provided insights into the evolution of Earth’s atmosphere and interior. Ian’s scientific contributions were recognised with numerous honours and awards, including being elected a Fellow of the American Geophysical Union, a Fellow of the Australian Academy of Science, and receiving the Jaeger Medal from the Australian Academy of Science and the Centenary Medal for ‘service to Australian society and science in geochronology’.
Keywords: Earth Sciences, geology, memoir.
Early life and family history
Ian McDougall (Fig. 1) was born on 24 May 1935, in Hobart, Tasmania, to parents Dugald and Caroline McDougall. Ian was the middle child in a family of five children, with both an older and younger brother and sister. Together Ian and his siblings attended the independent Friends’ School from kindergarten to matriculation. The family resided within a short walking distance from the school, and the children would return home each day for ‘middle day dinner’. Ian’s parents were devoted members of the local Anglican church and attending Sunday church service was non-negotiable for the family. All the children were part of the Sea Scouts or Guides, and each one earned Queen’s Scout or Guide badges. At school, Ian excelled at physics, chemistry and mathematics, but found languages a little more challenging.
In 1951, at the age of sixteen, Ian embarked on a journey to Austria with a group of Australian Scouts to participate in the 7th World Scout Jamboree. Set amidst the scenic mountains of the Salzkammergut region, near the small village of Bad Ischl, this memorable adventure included a slow boat trip to Europe. Over the course of the voyage, the scouts developed firm friendships. The jamboree, with a limited attendance of 15 000 scouts due to cost constraints in a country still under military control, welcomed attendees from across the world. Ian and his compatriots enjoyed an unparalleled camping experience and were exposed to many other cultures and languages. Upon returning to Australia, Ian completed his matriculation year at the Friends’ School and subsequently applied for enrolment at the University of Tasmania.
Although Ian had little interest in conventional team sports he enjoyed a game of tennis and harboured a passion for sailing. As a teenager, sailing became his primary interest and he was able to return to the sport in Canberra following the construction of the Scrivener Dam on the Molonglo river, which created Lake Burley Griffin. Ian passed on his sailing skills to his three children, and over the years, the family travelled to various sailing championships, equipped with an assortment of boats and camping gear.
Education: becoming a scientist
Ian embarked on his undergraduate studies at the University of Tasmania, earning a BSc (Hons) degree in 1957. During this period, he was introduced to the novel concepts of continental drift and an expanding Earth, by S. Warren Carey, a prominent advocate of these theories. Following his graduation, Ian pursued a doctoral program at the Department of Geophysics in the Australian National University, under the supervision of Germaine (Joppy) Joplin. His PhD thesis focused on the petrology and geochemistry of magmatic intrusive rocks in Tasmania known as the Tasmanian Dolerites, intrusive magmatic rocks of significant geological importance because they resulted from the break-up of the super-continent Gondwanaland. Ian’s comprehensive fieldwork, along with meticulous mineralogical and geochemical studies of the Red Hill dolerite intrusion, revealed its highly differentiated nature and tholeiitic basalt composition, similar to the Karroo dolerites in southern Africa.1 The field and laboratory skills that Ian acquired during his PhD studies proved instrumental in shaping the next stages of his academic career. He completed his PhD in 1960 and, except for a post-doctoral year at the University of California, Berkeley and post-retirement positions at the University of Queensland, he remained at the Australian National University (ANU) for the entirety of his academic career.
Academic career
In 1952, John Conrad Jaeger, the foundation Professor of Geophysics at ANU, was appointed inaugural Director of the Department of Geophysics, within the Research School of Physical Sciences (RSPhysS). Jaeger, a prominent scientist renowned for his significant contributions to applied mathematics, earth sciences and engineering, held a visionary outlook for the department, focussing on emerging quantitative technologies and techniques in the field of earth sciences. In 1959, when the Department of Radiochemistry in the Research School disbanded, Jaeger seized the opportunity to establish a research presence in radiometric dating. Key personnel, including John Richards, along with equipment such as the AEI MS2 gas-source mass spectrometer, were incorporated into the Department of Geophysics. These developments marked ANU’s entry into the field of isotope geochemistry, a domain that would become closely associated with the institution and Ian’s distinguished career.
During this time, Jaeger became enthused by the potential of the emerging potassium-argon (K-Ar) dating method, and the noble gas mass spectrometer developed by John Reynolds at the University of California, Berkeley. The K-Ar technique is based on the natural decay of 40K to 40Ar (with a half-life of approximately 1250 million years), where the age is proportional to the 40Arradiogenic/40K ratio after accounting for the presence of atmospheric 40Ar (40Ar/36Arair = 298.56). Jaeger made the decision to establish a K-Ar laboratory within the department, and involved John Richards in this endeavour. In 1960, Jaeger arranged for Jack Evernden from Berkeley to set up facilities for potassium and argon analysis in Canberra, utilising a Reynolds gas-source mass spectrometer procured from Berkeley. The collaboration between Evernden and Richards eventually led to the application of the K-Ar method for dating granites in south-eastern Australia,2 in partnership with the Bureau of Mineral Resources (BMR), that would later became the Australian Geological Survey Organisation and then Geoscience Australia.
Around the same time, Jaeger persuaded Ian to become involved with the K-Ar method and facilitated his postdoctoral fellowship year in John Reynold’s K-Ar laboratory, where he worked closely with Jack Evernden and Garniss Curtis. Upon Ian’s return to ANU (via Hawaii) in 1961, he was appointed to a research fellow position in the department, with the objective of further developing and applying the K-Ar dating method (Fig. 2). These events marked the foundation for Ian’s long and illustrious academic career at ANU, where he was promoted to fellow in 1964 and senior fellow in 1968. In 1973, the Department of Geophysics separated from the Research School of Physical Sciences to become the new Research School of Earth Sciences, with Professor Anton L. Hales serving as its inaugural director. Ian was promoted to professor in the research school in 1991.
Ian McDougall operating the MS-10 mass spectrometer used for K-Ar dating analyses (circa 1980) (Photographer: Rob Little, ANU Photographic Services).
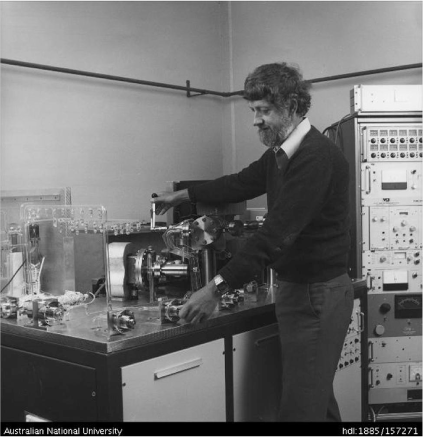
Following his formal retirement in 2001, Ian was appointed emeritus professor at ANU, where he continued his research until his laboratory was closed prematurely in 2006. Ian then accepted adjunct professor (2007–10) and honorary professor (2010–18) appointments in the School of Earth Sciences at the University of Queensland. These positions allowed Ian to continue his dating work in Professor Paulo Vasconcelos’ laboratory. Despite declining health, Ian remained active as a scientist until close to the time of his death in 2018.
K-Ar dating, plate tectonics and the geomagnetic timescale
The 1960s proved to be an exciting period for the field of geoscience. During this time, Ian published a series of ground-breaking articles that played a significant role in the development of the plate tectonics hypothesis, a theory that revolutionised understanding of Earth’s geodynamic history. Ian’s contributions and experiences during this crucial era are chronicled in his own, understated words,3 and further immortalised in the classic book by William Glen, entitled The Road to Jaramillo: Critical Years in the Revolution in Earth Science.4
In retrospect, it is clear that Ian’s PhD work provided him with the critical training necessary for his momentous contributions to K-Ar dating, the development of the plate tectonic theory, and establishment of the geomagnetic timescale. Early in his PhD project, Ian was introduced to rock magnetism and produced his first journal paper,5 which describes the use of geomagnetic data to determine the boundary between dolerite rock and overlying talus material. His study of the Tasmanian Dolerites provided him with valuable field experience and insights into the mineralogy and geochemistry of magmatic rocks. Ian went on to publish several articles on the Red Hill Dolerite, including a significant paper in Nature that reported K-Ar ages for various minerals from the intrusion.6 This paper was important because it demonstrated the feasibility of dating low-K minerals (such as plagioclase feldspar) and unaltered (as perceived by his petrologist’s eye) whole rock material using the K-Ar method. It also provided the first ages for the Tasmanian Dolerites.7
Ian had long held an interest in the Hawaiian volcanoes, inspired by Dana’s 1849 observations that the island chain became more eroded towards the WNW, suggesting a transition in the source of the volcanism along the chain over time.8 On his way to Berkeley, accompanied by his new wife Pam, Ian took the opportunity to visit Dr Don Richter at the United States Geological Survey (USGS) Kilauea Observatory and explored several volcanic sites. This excursion led Ian to consider the possibility of dating the young (<4 Ma) Hawaiian volcanoes using the K-Ar method, despite the prevailing scepticism surrounding the dating of such young rocks using this technique. In Ian’s own words: ‘most people at the time were very pessimistic about dating such young rocks’.9 This was because: (i) basaltic rocks contain low potassium contents, which limits the amount of radiogenic 40Ar produced from 40K; (ii) the younger the age, the less radiogenic argon is produced; and (iii) analysing low-potassium, young rocks requires large sample volumes (10–15 g), leading to increased atmospheric 40Ar contamination. The prevailing view at the time was that atmospheric argon contamination would swamp the small amounts of radiogenic 40Ar. On his way back from Berkeley, Ian secured a travel grant from Jaeger to sample volcanic rocks across the Hawaiin islands, from Kauai to Hawaii, a distance of approximately 500 km. The objective of the sampling program, was to test the potential of the K-Ar method to date the ESE to WNW progression of Hawaiian magmatism.10 Ian was careful to collect unaltered, crystalline volcanic rocks, including typical ‘tholeiitic basalts’, as well as ‘alkalic basalts’ with elevated potassium levels. This meticulous sampling approach, coupled with careful petrographic examination, proved critical to Ian’s subsequent K-Ar analyses, and is still employed in current research. During this trip, Ian also met with Don Tarling, a PhD student from ANU, who was sampling similar volcanic rocks for geomagnetic measurements.
Back in Canberra, Ian constructed a new extraction line for argon analyses and began dating Gondwana dolerite samples from Antarctica and South Africa.11 This work was significant, not only for confirming the similarity in age across the Gondwana flood basalt province, but also for fine-tuning methods of dating basaltic material. In 1962, Ian carried out his first K-Ar analyses on a Hawaiian sample, specifically a fresh alkali basalt (mugearite) sample (with approximately 2% potassium), collected from West Maui. This analysis unequivocally demonstrated the feasibility of dating young rocks with relatively high precision (Fig. 3).12 Ian then extended these analyses to other samples and, through careful experimental work, confirmed a distinct age progression from Kauai in the northwest (3.9–5.8 Ma) to volcanoes less than 1 million years old on Hawaii (Fig. 4).13 This was an momentous result, as it supported the age migration theory and also quantified the rate of volcanic migration. Furthermore, Ian’s findings provided support for the new mantle convection theory proposed by Canadian Physicist Tuzo Wilson,14 which later became known as the ‘hotspot’ model. According to this model, the movement of the Pacific ocean plate transitioning across a more or less stationary mantle convection plume produces an age progressive array of volcanic islands, such as the Hawaiian chain. Subsequently, Ian and his PhD student Robert Duncan, extended the K-Ar dating studies to other island/seamount chains, confirming similar age progressions, motion directions and migration rates across of the Pacific plate.15
Sketch from the original chart recording showing argon isotopes from the first Hawaiian basalt sample measured by Ian McDougall. The vertical axis shows argon peak heights (40Ar, 38Ar, 36Ar) measured at different recorder ranges, such that maximum values equate to a 40Ar/36Ar ratio of ~300, which is the value for atmospheric argon. The fact that the 36Ar peak height is ~50% that of 40Ar showed that the amount of radiogenic argon present in the sample was ~50%, thus demonstrating that young (<5 Ma) basalts could be dated by the K-Ar method. Modified from McDougall (2013).
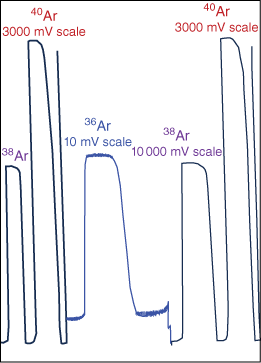
Map of the Hawaiian island chain from Niihau to Hawaii showing increasing K-Ar ages with distance to the WNW. Modified from McDougall (2013) and McDougall (1964).
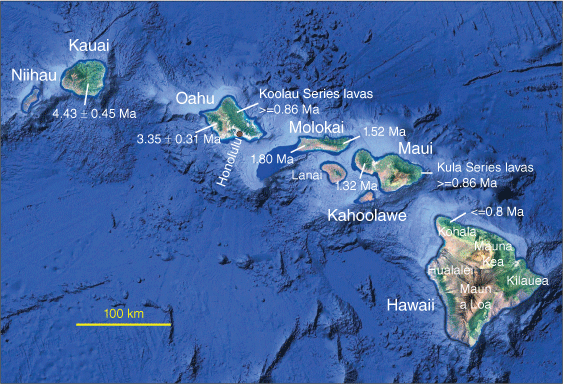
After successfully dating the Hawaiian basalts, Ian collaborated with Don Tarling to investigate geomagnetic evidence, which implied that rocks may undergo ‘self-reversal’, or that Earth’s magnetic field may have reversed polarity in the past. To test the hypothesis, Ian argued that it was necessary to compare paleomagnetic data from different parts of the world in rocks that could be accurately dated. Comparisons of paleomagnetic data obtained on Hawaiian lavas dated by the K-Ar method, revealed several polarity reversals spanning approximately 5.5 million years (Fig. 5).16 These findings were broadly consistent with similar studies in California and France.17 This work demonstrated that Earth’s polarity switched from Normal to Reversed at approximately 1.0 million years and then back to Normal at approximately 2.5 million years. These revelations were ground-breaking as they provided evidence supporting the notion that Earth’s magnetic field had changed polarity in the past, and they also showed that these changes were irregular. The combined studies of the ANU and United State Geological Survey research groups ultimately led to the development of the Geomagnetic Polarity Time-Scale (GPTS), that continues to be refined today. At more or less the same time, Vine and Matthews (1963) observed a pattern of alternating normal and reversed magnetic ‘stripes’ flanking mid-ocean ridges.18 The geomagnetic timescale developed for terrestrial samples provided the means to date the ‘stripes’ and confirmed that they were caused by volcanic upwelling at mid-ocean ridges coupled with sea-floor spreading. In other words, Ian’s research provided crucial evidence to support modern plate tectonic theory, as outlined in the books by H. R. Frankel (The Continental Drift Controversy, vol. 2 Paleomagnetism and Confirmation of Drift),19 H.W. Menard (The Ocean of Truth),20 and W. Glen (Continental Drift and Plate Tectonics).21
Compilation of geomagnetic data and K-Ar ages from Hawaii (Modified from McDougall and Tarling (1964) and McDougall (2013).
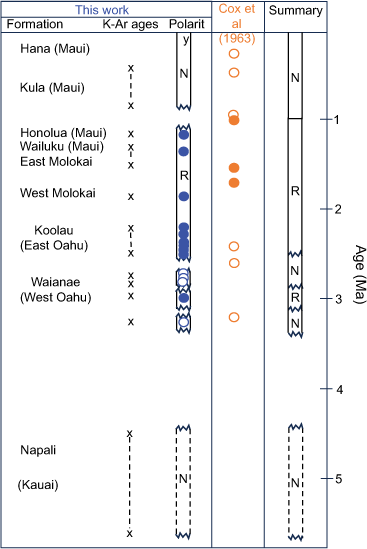
In subsequent years, Ian continued his K-Ar dating and paleomagnetic research on various island/seamount chains, including Fiji,22 Iceland,23 Reunion,24 and Mauritius.25 A significant contribution was the 1973 paper by McDougall and Watkins,26 that identified and constrained the timing of the short-lived (10 000–50 000 year) Reunion polarity excursion at 2.02 ± 0.02 million years. Together with PhD students Aziz-Ur-Rahman and Peter Wellman, Ian further extended his K-Ar dating studies to the Cainozoic volcanoes of eastern Australia. These studies were important for identifying a north-south oriented ‘hotspot’ track along the eastern margin of Australia, indicating northward migration of the Australian plate after separation from Antarctica.27
40Ar/39Ar dating—a new direction
The introduction of the 40Ar/39Ar variant of the K-Ar dating method by Merrihue and Turner in 1966 marked an exciting new development in K-Ar geochronology.28 This method addressed many of the limitations of traditional K-Ar dating, such as the need to analyse potassium and argon using different techniques (flame photometry versus mass spectrometry), which compounded uncertainties. The 40Ar/39Ar method, however, had its own drawbacks, including the requirement to irradiate samples in a nuclear reactor to convert a portion of the 39K to 39Ar (to measure the potassium content), as well as the need to co-irradiate samples with a fluence monitor mineral of known age. Despite these challenges, the 40Ar/39Ar dating method was quickly adopted by most K-Ar laboratories and remains one of the more popular dating methods in use today.
From the late 1960s, Ian set about establishing the 40Ar/39Ar method at ANU. Working with PhD student Neil Tetley, they contacted the Australian Atomic Energy Commission to explore the feasibility of irradiating samples in the Australian nuclear reactor, HIFAR. After several years of experimentation, McDougall and Zarko Roksandic published the first 40Ar/39Ar ages from the new laboratory in 1974.29 This study utilised Ian’s K-Ar standard, GA1550 biotite (from Mount Dromedary, NSW), as a fluence monitor and measured 40Ar/39Ar ages for various samples previously dated by the K-Ar method. Although results were similar, the study revealed large neutron fluence gradients across the sample cans (~30%), which restricted the achievable precision. These investigations also confirmed several isotopic interferences that required correction. Despite the limitations of the HIFAR reactor, Ian persisted and developed an innovative approach to mitigate the problems. This involved inverting the sample cans several times during irradiation to moderate neutron flux gradients and the use of cadmium foil as shielding to filter slow neutrons.30 These measures eventually became standard practise, reducing thermal neutron fluence gradients and nuclear interferences by two orders of magnitude. In addition, GA1550 biotite emerged as one of the few internationally calibrated fluence monitors and remains the preferred standard in several laboratories.
The initial 40Ar/39Ar analyses were conducted using a small AEI MS-10 mass spectrometer until the late 1970s (Fig. 2). In 1975, a Micromass 12 cm gas-source mass spectrometer (MM1200) was acquired and, after a protracted period of commissioning, became the primary instrument for 40Ar/39Ar dating. Eventually, this instrument was replaced by a VG3600 mass spectrometer, purchased in 1990 and commissioned in 1992. The VG3600 represented a significant advancement in analytical capability and featured laser microprobe and furnace systems of small sample analysis, including single feldspar grains from Turkana Basin volcanic ash-beds (tuffs). Over time, the VG3600 system underwent full automation to enhance throughput and became the preferred choice for high precision 40Ar/39Ar geochronology.31
The infamous ‘KBS Tuff Controversy’
In the late 1960s, events in remote northwest Kenya redirected Ian’s research for the next four decades. Starting in 1968, the National Museum of Kenya organised a series of archaeological expeditions to the east side of Lake Rudolf (now Lake Turkana), led by the late Richard Leakey. These expeditions yielded a remarkable collection of early hominin fossils and stone tools found in close proximity to a volcanic ash-bed named the Kay Behrensmeyer Site (KBS) Tuff. Determining the age of this tuff became crucial for constraining the timing of these fossils and artefacts. Initial 40Ar/39Ar analyses of KBS Tuff samples were carried out by Frank Fitch at Birckbeck College (London) and Jack Miller at the University of Cambridge, who reported an age of 2.61 ± 0.26 million years.32 However, this result was soon questioned on the basis of bio-stratigraphic correlations, which indicated an age less than about 2.0 million years.33 Subsequent analyses of KBS samples by the K-Ar laboratory at the University of California, Berkeley produced younger ages of 1.6 and 1.8 million.34 This disagreement led to the so-called ‘KBS Tuff Controversy’, extensively documented in Roger Lewin’s 1987 book entitled Bones of Contention: Controversies in the Search for Human Origins.35 By this stage, Ian had already established an international reputation for meticulous experimental work, making him the ideal candidate to provide an independent age for the KBS Tuff. Ian’s analyses yielded consistent K-Ar and 40Ar/39Ar results, indicating an age of approximately 1.88 million years for the KBS Tuff.36 This finding was supported by additional K-Ar analyses by the Berkeley laboratory,37 and fission track zircon ages,38 effectively resolving the controversy.
In the following years, Ian expanded his exacting dating work to numerous volcanic tuffs and basalt lavas across the Omo-Turkana Basin in Kenya and Ethiopia, in collaboration with the late Frank Brown, Meave Leakey, Craig Feibel and many others (Fig. 6). Early K-Ar analyses eventually gave way to single crystal 40Ar/39Ar dating analyses, which provided highly precise ages with uncertainties of approximately 20 000 years.39 The application of this approach to the KBS Tuff improved the precision of its age to 1.873 ± 0.028 million years.40 Ian’s compilation of K-Ar and 40Ar/39Ar ages established an exceptional chronological framework for the stratigraphy of the Turkana Basin, forming the basis for current interpretations of hominin evolution in the region. Ian’s contributions to Turkana Basin research are probably best summarised in the words of Meave Leakey in a letter to his wife Pam:
Without Ian’s input, we would have struggled to find meaning in the stratigraphy and dating of the Turkana sediments that hold such an important record of our past. We, and future generations will continue to benefit from his dedicated analyses and the resulting sound chronological sequence of the hominins, tools, and ancient faunas that we have recovered and continue to recover. Ian will always be remembered for his essential contributions over almost half a century of research.41
Important developments in noble gas thermochronology
Over the years, Ian’s research focussed primarily on K-Ar and 40Ar/39Ar geochronology. However, he also developed an interest in argon diffusion behaviour in minerals and the potential use of the 40Ar/39Ar method to unravel the thermal history of rocks and minerals, a field known as ‘thermochronology’. From 1978 Ian supervised several graduate student and post-doctoral research projects related to thermochronology, leading to significant advancements in the field. These included prominent studies with Mark Harrison on diffusion coefficients in minerals and the application of diffusion theory to construct quantitative thermal history information on minerals from slowly cooled terranes and magma intrusions. Much of this work is summarised in the book by McDougall and Harrison Geochronology and Thermochronology by the 40Ar/39Ar Method,42 which has become the preferred textbook for students and researchers in the field.
Another important example in this area resulted from Ian’s collaboration with Research Fellow Peter Zeitler. In 1987, they published a ground-breaking article demonstrating the potential of the U-Th/He apatite thermochronology method for studying low-temperature thermal histories.43 This publication is widely regarded as having revitalised interest in a previously overlooked technique. Since then, U-Th/He thermochronology has been extended to various minerals, such as zircon and monazite. However, apatite remains the preferred mineral, particularly due its complementary relationship with apatite fission track thermochronology.
Diversifying into noble gas geochemistry
Following feedback from a school review, Ian set about establishing noble gas geochemistry at ANU. To accomplish this, a suitable high mass resolution VG5400 mass spectrometer was purchased. In 1986, Masahiko Honda, previously employed in Reynolds' Berkeley laboratory, joined the group as a research fellow and was assigned the task of implementing a noble gas research program (Fig. 7). In Honda, Ian found a colleague who shared his meticulous and exacting approach to analytical research. A lengthy period ensued, during which the noble gas extraction line was painstakingly designed, constructed and tested. Finally, in 1989, the first noble gas analyses of submarine basalt glasses were completed, and the first manuscript was published in 1990 based on data from Des Patterson’s PhD thesis.44
Masahiko Honda and Ian McDougall discuss the new MM5400 mass spectrometer gas extraction line in 1991 (ANU Photographic Services).
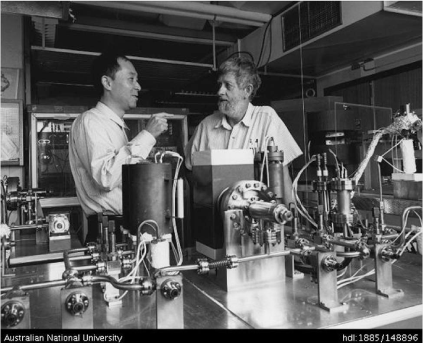
Over the years, the noble gas laboratory gained a reputation as one of the world’s premier noble gas facilities. Significant advances in the field included evidence from Hawaiian basalt glasses indicating a primordial solar noble gas component in the deep earth,45 and the utilisation of neon isotopes in apatite to identify contributions from upwelling mantle plume to volcanism in south-eastern Australia.46 In later years, Honda diversified his research program to include the refinement of cosmogenic He and Ne exposure dating methods,47 as well as noble gas geochemical and geochronological studies on diamonds;48 areas more removed from Ian’s primary interests.
A research career in retirement
Upon his retirement in 2001, Ian was appointed emeritus professor at ANU and remained an active researcher until ill health intervened. Unfortunately, Ian’s analytical work at the ANU was cut short in 2006, when the VG3600 mass spectrometer system in his laboratory was disassembled without his consent, in order to address a human resources conflict. This unfortunate event prompted offers of support from the small 40Ar/39Ar community in Australia, and Ian accepted adjunct and honorary professorships at the University of Queensland to continue his dating work in Paulo Vasconcelos’ laboratory.
With advancing years, Ian became increasingly committed to completing and publishing unfinished projects initiated prior to his retirement. One particular focus was his 40Ar/39Ar dating work on volcanic sequences across the Turkana Basin, resulting in more than fifteen journal articles published after 2001. Collaborating with Terry Spell and David Gray, Ian also finalised manuscripts on 40Ar/39Ar fluence monitors,49 and compiled previously unpublished K-Ar ages for basalts in Victoria.50
During the last decade of Ian’s life, instrument manufacturers introduced a new generation of multi-collector mass spectrometers, capable of significantly improved precision levels for 40Ar/39Ar geochronology. The 40Ar/39Ar laboratories in Australia were early adopters of these new instruments, including a revitalised ANU laboratory set up by Marnie Foster (Fig. 8). As expected, Ian took a keen interest in these new instruments and his expert advice proved invaluable in establishing instrumental and analytical protocols for the 40Ar/39Ar laboratories in Melbourne, Perth and Canberra.
Service to the discipline, honours and awards
Throughout his illustrious career, Ian served in various capacities on numerous committees and associations. These include the Australian Academy of Science National Committee for Geological Sciences (1972–9), vice-president of the International Association of Volcanology and Chemistry of the Earth’s Interior (1975–83), chair of the Australian Capital Territory (ACT) branch of the Geological Society of Australia, vice-chairman for the Organising Committee of the International Association of Volcanology and Chemistry of the Earth’s Interior General Assembly in Canberra (1993), and Treasurer of the Australian Academy of Science (2001–5). He also served on the editorial board of the Australian Journal of Earth Sciences (1979–87).
Ian received numerous awards and accolades for his immense contributions to Earth sciences. In 1997, he was elected a fellow of the American Geophysical Union and a fellow of the Australian Academy of Science in 1998. Notable awards include the Centenary Medal awarded in 2001 for ‘service to Australian society and science in geochronology’, the Jaeger Medal from the Australian Academy of Science in 2007, a DSc honoris causa from the University of Glasgow, UK in 2009, and a Gold Award from the Australian Institute of Nuclear Science and Engineering in 2013.
Final words
Ian will be remembered as a passionate and insightful researcher, with an uncompromising commitment to research integrity. There is no question that he set the benchmark for scientific excellence in the field of K-Ar and 40Ar/39Ar geochronology. To his graduate students and postdoctoral fellows, Ian was an ‘exacting mentor’, who insisted on ‘doing the sums’ and ‘getting things right’.51 Many of these students and fellows have gone on to pursue high-profile academic careers in the field, which is an ongoing testament to Ian’s influence.
Ian died on 10 November 2018 after a long illness. He is survived by his wife Pam, his children, Scott, Geoffrey, and Sandra, and grandchildren Islay, Faye, Angus, and Ellaine.
Acknowledgements
The author gratefully acknowledges the assistance received from Pam McDougall in the writing of this memoir. The memoir also benefitted from formal reviews by K. Lambeck and an anonymous reviewer. The Australian National University is acknowledged for permission to publish photographs from its archives.
References
Chamalaun, F. H., and McDougall, I. (1966) Dating geomagnetic polarity epochs in réunion, Nature, 210(5042), 1212-1214.
| Crossref | Google Scholar |
Cooke, H. B. S. and Maglio, V. J. (1972) ‘Plio-Pleistocene stratigraphy in East Africa in relation to proboscidean and suid evolution’, in Calibration of Hominoid Evolution, eds W. W. Bishop and J. A. Miller, Scottish Academic Press (for Wenner-Gren Foundation for Anthropological Research, New York), Edinburgh, pp. 303–329.
Cox, A., Doell, R. R., and Dalrymple, G. B. (1963) Geomagnetic polarity epochs and Pleistocene geochronometry, Nature, 198, 1049-1051.
| Crossref | Google Scholar |
Cox, A., Doell, R. R., and Dalrymple, G. B. (1964) Reversals of the Earth’s magnetic field, Science, 144, 1537-1543.
| Crossref | Google Scholar | PubMed |
Curtis, G. H., Cerling, D. T., and Hampel, (1975) Age of KBS Tuff in Koobi Fora formation, East Rudolf, Kenya, Nature, 258, 395-398.
| Crossref | Google Scholar |
Drake, R. E., Curtis, G. H., Cerling, T. E., Cerling, B. W., and Hampel, J. (1980) KBS Tuff dating and geochronology of tuffaceous sediments in the Koobi Fora and Shungura Formations, East Africa, Nature, 283, 368-372.
| Crossref | Google Scholar |
Duncan, R. A., and McDougall, I. (1976) Linear volcanism in French Polynesia, Journal of Volcanology and Geothermal Research, 1, 197-227.
| Crossref | Google Scholar |
Evernden, J. F., and Richards, J. R. (1962) Potassium‐argon ages in eastern Australia, Journal of the Geological Society of Australia, 9, 1-49.
| Crossref | Google Scholar |
Fitch, F. J., and Miller, J. A. (1970) New hominid remains and early artefacts from northern Kenya: Radioisotope age determinations of Lake Rudolf artefact site, Nature, 226, 226-228.
| Crossref | Google Scholar | PubMed |
Fujioka, T., Chappell, J., Honda, M., Yatsevich, I., Fifield, K., and Fabel, D. (2005) Global cooling initiated stony deserts in central Australia 2–4 Ma, dated by cosmogenic 21Ne-10Be, Geology, 33(12), 993-996.
| Crossref | Google Scholar |
Gleadow, A. J. W. (1980) Fission track age of the KBS Tuff and associated hominid remains in northern Kenya, Nature, 284, 225-230.
| Crossref | Google Scholar |
Gray, C. M., and McDougall, I. (2009) K-Ar geochronology of basalt petrogenesis, Newer Volcanic Province, Victoria, Australian Journal of Earth Sciences, 56(2), 245-258.
| Crossref | Google Scholar |
Honda, M., McDougall, I., Patterson, D. B., Doulgeris, A., and Clague, D. A. (1991) Possible solar noble-gas component in Hawaiian basalts, Nature, 349(6305), 149-151.
| Crossref | Google Scholar |
Honda, M., McDougall, I., Patterson, D. B., Doulgeris, A., and Clague, D. A. (1993) Noble gases in submarine pillow basalt glasses from Loihi and Kilauea, Hawaii: a solar component in the Earth, Geochimica et Cosmochimica Acta, 57(4), 859-874.
| Crossref | Google Scholar |
Matsumoto, T., Honda, M., McDougall, I., Yatsevich, I., and O’Reilly, S. Y. (1997) Plume-like neon in a metasomatic apatite from the Australian lithospheric mantle, Nature, 388(6638), 162-164.
| Crossref | Google Scholar |
McDougall, I. (1961) Determination of the age of a basic igneous intrusion by the potassium-argon method, Nature, 190, 1184-1186.
| Crossref | Google Scholar |
McDougall, I. (1962) Differentiation of the Tasmanian Dolerites: Red Hill dolerite-granophyre association, Geological Society of America Bulletin, 73, 279-316.
| Crossref | Google Scholar |
McDougall, I. (1963a) Potassium-argon age measurements on dolerites from Antarctica and South Africa, Journal of Geophysical Research, 68(5), 1535-1545.
| Crossref | Google Scholar |
McDougall, I. (1963b) Potassium-argon ages from western Oahu, Hawaii, Nature, 197(4865), 344-345.
| Crossref | Google Scholar |
McDougall, I. (1963c) Potassium-argon ages of some rocks from Viti Levu, Fiji, Nature, 198(4881), 677.
| Crossref | Google Scholar |
McDougall, I. (1964) Potassium-argon ages from lavas of the Hawaiian Islands, GSA Bulletin, 75(2), 107-128.
| Crossref | Google Scholar |
McDougall, I. (1979) Age of shield-building volcanism of Kauai and linear migration of volcanism in the Hawaiian Island Chain, Earth and Planetary Science Letters, 46, 31-42.
| Crossref | Google Scholar |
McDougall, I. (1981) 40Ar/39Ar age spectra from the KBS Tuff, Koobi Fora formation, Nature, 294(5837), 120-124.
| Crossref | Google Scholar | PubMed |
McDougall, I. (1985) K-Ar and 40Ar/39Ar dating of the hominid-bearing Pliocene-Pleistocene sequence at Koobi Fora, Lake Turkana, northern Kenya, Geological Society of America Bulletin, 96(2), 159-175.
| Crossref | Google Scholar |
McDougall, I. (2008) Brief history of isotope geology at the Australian National University, Australian Journal of Earth Sciences, 55(6–7), 727-736.
| Crossref | Google Scholar |
McDougall, I. (2013) Retrospective on the plate tectonic revolution focusing on K/Ar dating, linear volcanic chains and the geomagnetic polarity time scale, Earth Sciences History, 32(2), 313-331.
| Crossref | Google Scholar |
McDougall, I., and Brown, F. H. (2006) Precise 40Ar/39Ar geochronology for the upper Koobi Fora formation, Turkana Basin, northern Kenya, Journal of the Geological Society, 163(1), 205-220.
| Crossref | Google Scholar |
McDougall, I., and Brown, F. H. (2008) Geochronology of the pre-KBS Tuff sequence, Omo Group, Turkana Basin, Journal of the Geological Society, 165(2), 549-562.
| Crossref | Google Scholar |
McDougall, I., and Chamalaun, F. H. (1966) Geomagnetic polarity scale of time, Nature, 212(5069), 1415-1418.
| Crossref | Google Scholar |
McDougall, I., and Chamalaun, F. H. (1969) Isotopic dating and geomagnetic polarity studies on volcanic rocks from mauritius, Indian ocean, GSA Bulletin, 80(8), 1419-1442.
| Crossref | Google Scholar |
McDougall, I., and Duncan, R. A. (1980) Linear volcanic chains — recording plate motions? Tectonophysics, 63, 275-295.
| Crossref | Google Scholar |
McDougall, I., and Green, R. (1958) The use of magnetic measurements for the study of the structure of talus slopes, Geological Magazine, 95(3), 252-260.
| Crossref | Google Scholar |
McDougall, I., and Roksandic, Z. (1974) Total fusion 40Ar/39Ar ages using Hifar reactor, Journal of the Geological Society of Australia, 21(1), 81-89.
| Crossref | Google Scholar |
McDougall, I., and Tarling, D. H. (1963) Dating of polarity zones in the Hawaiian Islands, Nature, 200(4901), 54-56.
| Crossref | Google Scholar |
McDougall, I., and Tarling, D. H. (1964) Dating geomagnetic polarity zones, Nature, 202(4928), 171-172.
| Crossref | Google Scholar |
McDougall, I., and Watkins, N. D. (1973) Age and duration of the Réunion geomagnetic polarity event, Earth and Planetary Science Letters, 19(4), 443-452.
| Crossref | Google Scholar |
McDougall, I., and Wellman, P. (2011) Calibration of GA1550 biotite standard for K/Ar and 40Ar/39Ar dating, Chemical Geology, 280(1–2), 19-25.
| Crossref | Google Scholar |
McDougall, I., and Wensink, H. (1966) Paleomagnetism and geochronology of the Pliocene-Pleistocene lavas in Iceland, Earth and Planetary Science Letters, 1(4), 232-236.
| Crossref | Google Scholar |
McDougall, I., Maier, R., Sutherland-Hawkes, P., and Gleadow, A. J. W. (1980) K-Ar age estimate for the KBS Tuff, East Turkana, Kenya, Nature, 284(5753), 230-234.
| Crossref | Google Scholar |
McDougall, I., Brown, F. H., Vasconcelos, P. M., Cohen, B. E., Thiede, D. S., and Buchanan, M. J. (2012) New single crystal 40Ar/39Ar ages improve time scale for deposition of the Omo Group, Omo–Turkana Basin, East Africa, Journal of the Geological Society, 169, 213-226.
| Crossref | Google Scholar |
Merrihue, C., and Turner, G. (1966) Potassium-argon dating by activation with fast neutrons, Journal of Geophysical Research, 71(11), 2852-2857.
| Crossref | Google Scholar |
Patterson, D. B., Honda, M., and McDougall, I. (1990) Atmospheric contamination: A possible source for heavy noble gases in basalts from Loihi Seamount, Hawaii, Geophysical Research Letters, 17(6), 705-708.
| Crossref | Google Scholar |
Roche, A. (1951) Sur les inversions de l’aimentation remanente des roches volcaniques dans les monts d’Auvergne, Comptes Rendus Academic Science, 223, 1132-1134 [In French].
| Google Scholar |
Roche, A. (1956) Sur la date de la derniere inversion du champ magnetique Terrestre, Comptes Rendus Academic Science, 243, 812-814 [In French].
| Google Scholar |
Spell, T. L., and McDougall, I. (2003) Characterization and calibration of 40Ar/39Ar dating standards, Chemical Geology, 198, 189-211.
| Crossref | Google Scholar |
Tetley, N., McDougall, I., and Heydegger, H. R. (1980) Thermal neutron interferences in the 40Ar/39Ar dating technique, Journal of Geophysical Research: Solid Earth, 85(B12), 7201-7205.
| Crossref | Google Scholar |
Timmerman, S., Honda, M., Burnham, A. D., Amelin, Y., Woodland, S., Pearson, D. G., Jaques, A. L., Le Losq, C., Bennett, V. C., Bulanova, G. P., Smith, C. B., Harris, J. W., and Tohver, E. (2019) Primordial and recycled helium isotope signatures in the mantle transition zone, Science, 365(6454), 692-694.
| Crossref | Google Scholar | PubMed |
Vine, F. J., and Matthews, D. H. (1963) Magnetic anomalies over oceanic ridges, Nature, 199, 947-949.
| Crossref | Google Scholar |
Wellman, P., and McDougall, I. (1974) Potassium-argon ages on the Cainozoic volcanic rocks of New South Wales, Journal of the Geological Society of Australia, 21(3), 247-272.
| Crossref | Google Scholar |
Wilson, J. T. (1963a) Evidence from islands on the spreading of ocean floors, Nature, 197, 536-538.
| Crossref | Google Scholar |
Wilson, J. T. (1963b) Hypothesis of Earth’s behaviour, Nature, 198(4884), 925-929.
| Crossref | Google Scholar |
Wilson, J. T. (1963c) A possible origin of the Hawaiian islands, Canadian Journal of Physics, 41, 863-870.
| Crossref | Google Scholar |
Zeitler, P. K., Herczeg, A. L., McDougall, I., and Honda, M. (1987) U-Th-He dating of apatite: a potential thermochronometer, Geochimica et Cosmochimica Acta, 51(10), 2865-2868.
| Crossref | Google Scholar |
Zeitler, P., Harrison, M., Baldwin, S., Duncan, R., Spell, T., and Wijbrans, J. (2019) Ian McDougall (1935–2018), Eos, 100,.
| Crossref | Google Scholar |
Footnotes
3 McDougall (2008, 2013).
4 Glen (1982).
12 McDougall (2013).
13 McDougall (1964).
14 Wilson (1963a, 1963b, 1963c).
19 Frankel (2012).
20 Menard (1986).
21 Glen (1975).
31 McDougall (2008).
35 Lewin (1987).
38 Gleadow (1980).
45 Honda and others (1991, 1993).