Challenges to optimal macadamia (Macadamia spp.) kernel quality in a changing climate
Suzy Y. Rogiers
A
Abstract
Macadamias (Macadamia spp.) are highly desired for their flavour and nutritional characteristics. Despite cultivation in their native habitat, unpredictable and extreme weather events are applying pressure on the Australian macadamia industry to maintain the production of high-quality kernels. The industry has experienced losses in yield and quality due to shifts in the timing and volume of rain in recent years, and this has identified the requirement for transformational changes in orchard agronomic practices. Pre-harvest conditions that impinge on kernel oil content, kernel recovery, whole kernels and kernel appearance are discussed in this review. The impact of harvest and storage conditions on kernel quality are also reviewed. We propose opportunities for further research to optimise on-farm practices and to safeguard kernel quality post-harvest. This includes building on existing research to advance our understanding of the interaction of genetics with plant response to climate change. It also requires innovation to advance technologies that foster precision management, both spatially and temporally, in an environmentally sensitive manner.
Keywords: carbohydrate partitioning, climate change, drought, flooding, fruit abortion, irrigation, kernel discoloration, macadamia production, nut development, nut quality, pests and diseases, pollination, rancidity, review, waterlogging.
Introduction
The macadamia industry in Australia has experienced significant growth over the past few decades, driven by increasing global demand for macadamias due to their nutritional value and health benefits. This expansion has spurred investment in production, research, and processing technologies, positioning macadamias as a key global commodity. This literature review was written to address key challenges faced by the Australian macadamia industry, particularly in light of unpredictable and extreme weather events that have affected both yield and quality. The review is aimed at macadamia researchers, and provides valuable insights for growers, processors, industry associations, consultants, industry development and extension officers, and government agencies. By exploring the impact of pre-harvest conditions, harvest and storage practices on kernel quality, the review identifies critical areas for improvement and proposes opportunities for future research. It underscores the need for transformational changes in orchard agronomy and highlights the potential for precision management practices, alongside an increased understanding of genetics and climate change interactions, to safeguard macadamia production in an evolving climate.
The macadamia tree and nut
The macadamia tree belongs to the ancient Gondwanan Proteaceae family and is native to the rainforests of northern NSW and southern Queensland (25.5–29°S latitude) (Nock et al. 2019). The Macadamia genus is composed of Macadamia integrifolia, Macadamia tetraphylla, Macadamia ternifolia, and Macadamia jansenii; however, only the first two species and their hybrids are cultivated as the latter two produces small, bitter nuts high in cyanogenic compounds (Sedgley et al. 1990; Dahler et al. 1995). M. integrifolia produces smooth-shelled nuts, whereas M. tetraphylla are rough-shelled. These tall evergreen trees with a long juvenile period are now commercially grown in other countries between the latitudes of 32°S and 34°N. Flowers develop in 15–20 cm long raceme inflorescences (Trueman 2013). They are bee, wasp, beetle, microbat or butterfly pollinated (Howlett et al. 2015; Santos et al. 2020), and mostly outcrossing (Langdon et al. 2019; Richards et al. 2020). Under optimal conditions, kernel maturation requires 190 days from flowering, with the nuts commercially harvested at an oil content of 72–78 g per 100 g and specific gravity (SG) less than 1.00 (Hardner et al. 2001; Wall and Gentry 2007).
Macadamias are well known for their delicate flavour and nutritional value (Crain and Tang 1975; Shuai et al. 2023). The nuts are rich in bioactive macronutrients such as protein, dietary fibre, essential minerals (Mg, Ca, K, Fe), tocopherol, plant sterols and antioxidants (Rengel et al. 2015). Macadamia is a superior source of monounsaturated oil compared to other oils such as olive oil or rapeseed oil (Kaijser et al. 2000). Oleic (60%), arachidonic (16%) and palmitoleic acids (10%), all unsaturated fatty acids, are present in high quantities in macadamia oil relative to other nut oils such as walnut and cashew (Kaijser et al. 2000; Hu et al. 2019; Shuai et al. 2022). These fats confer a number of health benefits such as lowering blood cholesterol (Solà Marsiñach and Cuenca 2019) and lowering the risk of diabetes (Hu et al. 2019). In addition, macadamia oil is used in cosmetic and pharmaceutical products targeted at skin care, including moisturising and anti-aging products, and for the prevention and treatment of various health conditions (Insanu et al. 2021).
Nut development and kernel maturity
Macadamia fruit require around 7 months to reach maturity (Jones 1939; Jones and Shaw 1943) (see Fig. 1), but this may vary according to cultivar, orchard practices, and climate. Within a geographic location, climate related warming may result in an earlier spring and maturity as described in other perennial crops such as grapes (Vitis spp.) (Rogiers et al. 2022), peach (Prunus persica) (Vanalli et al. 2021), and apple (Malus spp.) (Kunz and Blanke 2022). Macadamia kernels are considered mature when the oil content is 60–75% of the nut’s weight and the moisture content is 20–30% (Quinlan et al. 2008). Flower initiation occurs in autumn and early winter, when minimum temperatures reach 11–15°C, with anthesis in late winter (Trueman 2013). The abscission of fertilised ovules shortly after anthesis is common. The self-management of crop load results in extensive nut abortion at 5–6 weeks after anthesis (Stephenson and Gallagher 1986a). However, both biotic and abiotic stresses can intensify spring ovule shedding. Water stress is known to result in significant abortion, thus irrigation may be beneficial during those stages (Stephenson et al. 2003). Physical forces, such as high wind, and temperatures above 30°C can also exacerbate abortion (Stephenson and Gallagher 1986a). Moreover, nuts may abscise prematurely because of pest and disease incursions.
Timeline of macadamia nut development illustrating accumulation of oil, alongside changes in kernel volume, water, sugars and protein. These changes coincide with fluxes in vegetative and root growth. The period of spring ovule shedding, premature and mature nut drop are also indicated.
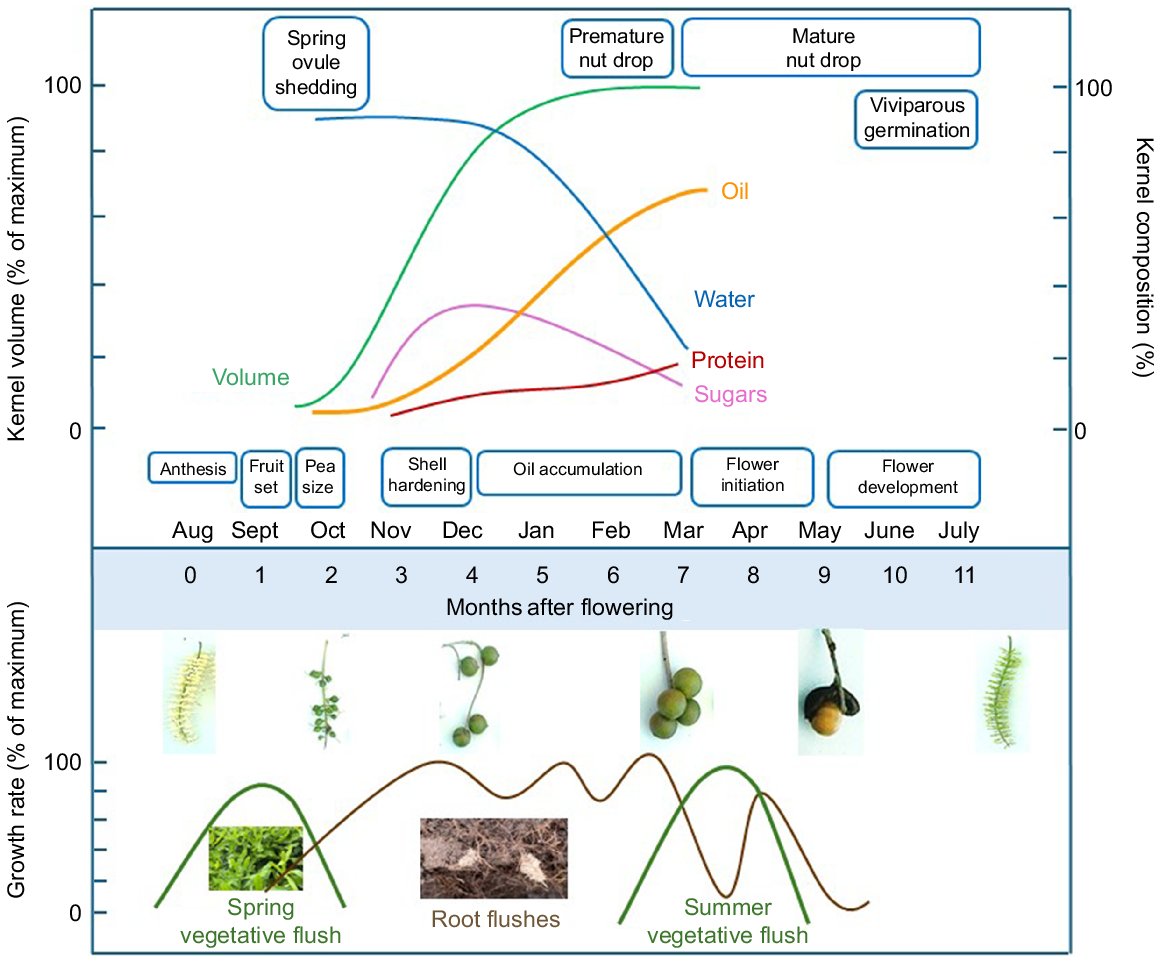
Having survived the spring ovule shedding phase, the retained fruit enlarge for 3 months after anthesis until the shell hardens. At this time, oil synthesis is initiated and continues rapidly for the following 2 months. Subsequently, the composition of polar lipids and fatty acids alters slowly from 5 months through to full maturity at 7 months after flowering (Koaze et al. 2002a). Hexose sugars are the precursors for short chain saturated fatty acids, which are subsequently converted into long chain unsaturated fatty acids (Jones and Shaw 1943). Accordingly, kernel sugar content declines during the oil accumulation phase. Protein levels remain low relative to the other compositional parameters (Fig. 1). Premature fruit drop may occur during these last phases of development. Immature kernels tend to have higher sucrose and reducing sugars, leading to more internal browning (Wall and Gentry 2007; Kader 2013).
Despite a short and defined flowering stage, the achievement of maximum oil content is not necessarily correlated with the onset of fruit abscission, and mature nut drop can extend over several months within a cultivar (Trueman et al. 2000). A delay in nut drop may have ramifications for the moisture and texture of the kernel. Oil, moisture and texture are relevant to both the raw kernel and nut-in-shell market. In one study, kernel shelf life was shorter for nuts that abscised 4 months later than those mature nuts that had abscised earlier (Gama et al. 2020).
Kernel size and shape
While oil content, flavour, and texture can serve as indicators of kernel quality, size and shape (including kernel integrity) are typically the primary characteristics monitored to assess economic value (Quinlan et al. 2008). The macadamia fruit is single seeded and composed of the kernel (embryo and endosperm, both progeny tissues), a particularly hard shell (testa), and husk (pericarp) (Hardner et al. 2001). Only one of two ovules develop within the globose follicle, and once mature, a single suture in the husk will release the nut, composed of the shell surrounding the kernel. High-quality macadamia kernels are uniform in size, shape, and colour. Macadamia kernels generally remain intact during nut development; however, smaller nuts tend to have lower kernel recovery (ratio of kernel to shell) and whole-kernel incidence than large nuts (Richards et al. 2020). Growers are paid a premium not only on oil content, but also for kernel recovery as a percentage of nut-in-shell. Kernels can be damaged and segmented during development, as well as after harvest when they are subjected to excessive force through dehusking and shell removal, or high-pressure during roasting. For instance, shoulder damage may occur as a result of dropping or dehusking (Walton and Wallace 2010). Moreover, the shell can crack due to changes in temperature or moisture levels, which can cause the kernel to break into smaller pieces. To maximise economic value, it is crucial for growers to carefully manage both the physical integrity of the kernels and the conditions under which they are processed, ensuring minimal damage and optimal recovery.
Kernel discolouration
Internal and external discolouration is a significant challenge affecting macadamia nut quality (Le Lagadec 2009). It can be present at the time of harvest, or may develop during handling, processing, roasting and storage. It has been established that internal discolouration, also referred to as kernel browning, may be the result of three different processes: (1) discolouration due to fungal or bacterial infection; (2) enzymatic browning; or (3) non-enzymatic browning (Penter et al. 2007; Quinlan et al. 2008; Martinez et al. 2024).
Discolouration due to infection can be caused by a variety of microorganisms, including Aspergillus spp., Penicillium spp., and Fusarium spp. Kernel browning can occur on the orchard floor and through to handling and storage stages. Infrequent harvesting and lengthy periods on the ground may exacerbate mould and this type of discolouration (Quinlan et al. 2008). Low temperature, low humidity, and low moisture content during storage will prevent and slow the likelihood of microbial degradation and improve the shelf life (Gama et al. 2018).
Enzymatic browning is a significant industry issue and can appear as a distinct line around the equator of the kernel, or progress to staining of the entire basal portion of the kernel to a depth of 5–10 cell layers (Penter et al. 2007). This stain is a water-soluble phenolic compound and is derived from the shell (Du Preez 2015). Polyphenol oxidase (PPO) and peroxidase (POD) are the primary enzymes responsible for enzymatic browning. These enzymes are located in the cell walls and are released when the cell walls are disrupted, leading to the oxidation of phenolic compounds and the formation of brown pigments. Enzymatic browning can occur during harvesting, processing, and storage of the nuts. For instance, post-harvest dropping of nuts can result in cellular damage, some of which is not revealed until roasting (Walton and Wallace 2010).
Non-enzymatic browning may also occur when the macadamia nuts are exposed to high temperatures and moisture during processing (Srichamnong and Srzednicki 2015). This type of browning is caused by the Maillard reaction, which is a chemical reaction between amino acids and reducing sugars. Maillard derived browning was more prevalent in immature fruits with high sugar and low oil content (Koaze et al. 2002b). Martinez et al. (2023) found that kernels with brown centres had a higher moisture content, and kernel browning could be induced in the lab by storage under high moisture and high temperatures (Martinez et al. 2024). The authors hypothesised that these conditions trigger the hydrolysis of sucrose into the reducing sugars, forming substrates for the Maillard reaction. Their studies also demonstrated that white kernels and brown centre kernels had similar fatty acid profiles and peroxide values, and that browning was not the result of non-enzymatic oil oxidation (Martinez et al. 2023). Confocal, X-ray tomography and MRI studies of cv. Daddow, a cultivar less prone to browning, revealed a smoother internal structure compared to cv. 842, which had more fractures (Srichamnong et al. 2013). The authors hypothesised that the structure of cv. Daddow would facilitate unrestricted diffusion and therefore high drying rates. Moreover, cv. Daddow exhibited lower shell and kernel density, also potentially accelerating drying rates, and therefore limiting chemical reactions related to browning. Non-enzymatic browning may alter the flavour and aroma of the nuts. Nonanoic acid, octanoic acid, and 2,3 butanediol, resulting from lipid oxidation, were associated with the brown centres (Martinez et al. 2024). It is evident that proper kernel drying to a moisture content of 1.5%, and subsequent storage at low humidity are prerequisite to warding off browning of macadamia kernels.
Calcium (Ca) is important to cell wall and membrane stabilisation; however, the low transpiration rates of structures such as seed kernels may result in deficiencies. Ca deficiency has been associated with browning in hazelnuts, and it was observed that the application of Ca to the soil resulted in a significant reduction in discolouration (Cacka and Sanguankeo 2014). Similarly, pecan opalescence, a discolouration ranging from white to brown, was found to be associated with high levels of oil and low levels of Ca (Wakeling et al. 2002). Unlike these two crops, this nutrient does not appear to be associated with kernel browning in macadamias. Martinez et al. (2024) found the brown regions had higher nutrient concentrations, including Ca. The authors suggested that the subcellular locations and concentrations may be critical, therefore the role of calcium and other nutrients requires further analysis.
Off-flavours and rancidity
Further quality defects in macadamia include off-flavour development caused by oxidation and rancidity (Wall 2013). Whilst the unsaturated fatty acids are nutritionally valuable, they are also prone to oxidation and result in the production of hydrogen peroxides. Peroxide value (PV) quantifies the amount of peroxides (primarily hydroperoxides) in the oil. Both the monounsaturated and polyunsaturated fatty acids are susceptible to oxidation when exposed to oxygen, moisture, heat and light (Borompichaichartkul et al. 2009). The oxidation process produces a variety of volatile compounds, including aldehydes, ketones, and alcohols, which can create off-flavours and aromas in the nuts. Compared with roasted kernels, raw kernels were found to have high levels of polyphenol oxidase, peroxide value and fatty acids with low concentrations of phenols, antioxidants and poor aroma (Buthelezi et al. 2021). In addition, the oxidation of unsaturated fatty acids can lead to the formation of free radicals, which can further accelerate the oxidation process and cause rancidity in the nuts (Shahidi and John 2013). Tocols are antioxidants that have the potential to kerb the onset of chronic diseases and have been shown to contribute to walnut (Juglans regia) and hazelnut (Corylus spp.) stability (Savage et al. 1997, 1999). Cultivar comparisons demonstrated that Hawaii-grown HAES 294 and HAES 835 had superior kernel oxidative stability, however this could not be attributed to the bioactive constituents tocopherols, tocotrienols or squalene (Wall 2010). Therefore, kernel stability may depend on interactions between specific fatty acids and other types of phytochemicals with antioxidant properties.
Pre-harvest challenges to optimal kernel quality
Cultivars, clones, and rootstocks
Cultivars, clones, and rootstocks have a significant impact on kernel weight and quality of macadamia nuts (Hardner et al. 2001; Penter et al. 2008; Topp et al. 2019). Macadamia has a short cultivation history, and most cultivars are only two to four generations from the wild (Lin et al. 2022). There are about 20 cultivars of macadamia planted globally (Trueman et al. 2024) Most of the breeding has focused on traits such as tree size, yield, and kernel recovery; however, little is known about differences in kernel quality parameters (Wall 2010). Further research into cultivar differences in propensity to insect damage, kernel discolouration and flavour is warranted.
Kernel recovery is the percentage of nut that is recovered as edible kernel and leads to better payment rates for growers. A negative relationship was evident between shell thickness and kernel recovery in wild macadamia germplasm (Mai et al. 2021). Cultivars also differ in shell hardness and thickness, and this impacts on the ease of cracking and drying rate. However, recent research has shown that pest control, particularly for Fruit Spotting Bug (FSB), is more effective with thicker shell and husk cultivars. If the combined shell and husk thickness exceeds 10 mm, FSB damage is significantly reduced, as the proboscis of the FSB is around 10 mm long. The cultivars A203, A268, A403 have a thick husk and shell and is reported to have less issues with ‘late insect’ damage (Bright and Alt 2024). Therefore, the trend towards higher kernel recovery may become less critical if the industry recognises the benefits of lower kernel recovery and reduced need for pest control measures.
Cultivar differences may play a significant role in resilience to climate change by providing various adaptations that help trees better cope with changing environmental conditions. The challenge lies in identifying the desirable traits and performance factors suited to the specific orchard situation and the potential climate influences on that particular site. Factors such tree shape, canopy density, length of flowering duration, compatibility with cross-pollinated cultivars, drop pattern of mature nut, and germination risk are some important considerations in the future that should be reviewed to ensure that the selected cultivar is well-suited to the specific orchard situation. For instance, dense canopies (344, 800 etc.) are difficult to penetrate with sprays and therefore may be more conducive to disease. Additionally dense canopies may lead to soil erosion due to low light levels on the orchard floor, especially in high rainfall areas. Cultivars that have a tendency for prolonged (849) or multiple nut drops may have reduced quality due to germination or kernel discolouration. In contrast, cultivars that have tendency for premature nut drop in hotter climates (741) will have lower oil content. Nuts of some cultivars (Beaumont) are prone to germination on the tree in wet conditions, reducing yield. Additionally, as described by Bright and Alt (2024), there are cultivars more susceptible to wind damage (A203, A268, 246 etc.), drier climates (cvs 660, A38 etc.), heat stress (cv. 344) abnormal vertical growth (cvs 344, 660, 741 etc.) and diseases such as Phytophthora (cvs H2, 816, 842, etc.), flower blight (849), Botryosphaeria (cvs 344, A268 etc.), and husk spot (Pseudocercospera macadamiae) (cvs 741, 816, A16, A4 etc.). This is useful information when planning to develop or maintain orchard into the future where climate variability is a certainty.
Climate-related stresses can lower productivity and rootstocks that provide better resistance to drought, heat, pests and disease will ensure farmers that their trees will remain productive. Beaumont is a common rootstock used in Australia but has a high-water requirement and suffers in drought (Bright and Alt 2024). H2 has a uniform and thick stem for grafting but is susceptible to phytophthora. Disease resilient rootstocks targeted for drought or flood prone regions will help macadamias thrive in these unpredictable climates. In addition, the development of vigour-reducing rootstocks to alleviate within tree competition for resources will provide new opportunities to improve orchard productivity and kernel quality. As evidenced in other crops such as apples, pears and stone fruits, high density orchards can increase yield per ha, are more efficient in water and nutrient use, and may result in improved quality due to better light penetration and pest and disease management with the proper plant architecture. Labour costs may also decline. Despite these potential benefits, research into specialised rootstocks is lacking.
Pollination
Macadamia trees can produce more than 3000 racemes, each bearing 100–300 flowers; however, like other Proteaceae species, fruit set is generally low (Trueman et al. 2022a). Once the flower has opened, the stigma is receptive for only 2 days (Sedgley 1983). Approximately 5% of flowers undergo fruit set, of which 1–2% become mature nuts (Trueman et al. 2022a, 2022b). The flowers are bisexual, but they are partially self-incompatible (Trueman 2013). Self-fertilisation is curtailed through impeded pollen tube growth (Sedgley 1983). Self-paternity was low at 0–3% in cvs Daddow, 816, and A4 (Richards et al. 2020). Similarly, in a varietal trial plot located in a large commercial orchard, self-pollination was not detected in cvs 246, 344, 800, A4, and A16, but ranged 20–40% in cvs 741, 791, 660, and 842 (Langdon et al. 2019). Cross pollination has positive effects on fruit set and nut retention (Wallace et al. 1996; Howlett et al. 2019) and may improve nut in shell (NIS) weight, kernel weight and kernel recovery (Trueman and Turnbull 1994; Wallace et al. 1996; Du Preez 2015; Trueman et al. 2024). A large study of 19 cultivars across 23 sites in Australia, Brazil, and South Africa revealed that 80–100% of fruit were cross pollinated, intermediate outcrossing (41–72%) was present in five sites, and low outcrossing (10%) was present in only one isolated cultivar at one site (Trueman et al. 2024). Self-pollinated fruitlets commonly abscise from the tree around 6 weeks after flowering (Trueman et al. 2022a). Macadamia’s pollen limitation was evidenced by a 63% increase in fruit set through supplemental pollen addition (Knight et al. 2006). Additionally, poor pollen quality (Van Etten 2022) and environmental conditions such as high or low temperature, low humidity and rain (Langdon et al. 2019) may play a role in abortion rates.
In Australia, the flowering racemes are pollinated by a wide variety of species including European (Apis mellifera) and native stingless bees (Tetragonula carbonaria) (Trueman et al. 2024). A study in Brazil found that butterflies were responsible for 50% of floral visits and that final fruit set was improved, but not kernel weight (Santos et al. 2020). In the three-country study (Trueman et al. 2024), outcrossed fruit were higher in oil concentration by 3.0 to 3.5% than fruit fertilised by pollen of the same cultivar. Additionally, a recent study found that both the seed parent and the pollen parent influence the fatty acid profile of the kernel (Hu et al. 2023). Therefore, outcrossing not only influences nut number, but also nut quality.
The extent of cross pollination in macadamia is important to orchard design (Anders et al. 2023) with the consideration of incorporating pollinating genotypes that bloom at the same phenological time in close proximity. With fruiting genotypes, stingless bee numbers within the orchard are dependent on the distance from the hive, and honeybee numbers are dependent on tree flower number (Evans et al. 2021). Therefore the physical distribution of hives, and the deployment of managed bee colonies at the correct phenological stage, can have a strong influence on pollination. Shifting climate patterns are putting pressure on particular pollinator species (Classen et al. 2015). For instance, honeybees are sensitive to extreme weather, resulting in reduced forage and increased disease, including parasites and pathogens (Ali et al. 2023). Pollinator species are not immune from the loss in biodiversity that is occurring at unprecedented rates because of habitat fragmentation. Considering that macadamia is pollinated by several species, these environmental shifts will have implications for future orchard design. Pollinator loss can be tackled, for instance, by incorporating diverse groundcovers, shrubs and trees in within or adjacent to the orchard to encourage, bees, flies and beetles. Reducing pesticide use, providing water sources and shelter for insects through deadwood and tree hollows will also encourage the presence of diverse pollinators.
Carbon allocation
Photosynthates are the primary source of energy for the tree, and their distribution and allocation to different carbon sinks can thus affect overall productivity (Spann et al. 2008). Temperature, light and water stress (too much or too little) inhibit photosynthesis, and consequently the accumulation and allocation of carbon (C). Notably, even under optimal conditions, macadamia is characterised by low rates of C assimilation relative to other perennial tree crops because of both stomatal and non-stomatal limitations (Smit et al. 2020). Light saturated net CO2 assimilation rate (Amax) was 8.3 μmol CO2 m−2 s−1 relative to other crops such as almond, pecan and pear which have values greater than 15 μmol CO2 m−2 s−1 (Smit et al. 2020). However, Amax was 56% higher during the fruit bearing stage of the season despite greater evaporative demands (Smit et al. 2020). This is likely because nut development requires a significant amount of energy (Stephenson et al. 1989a) for building complex energy compounds such as fatty acids and proteins. C movement is dynamic, with two-way interactions between storage in roots and stems, and mobilisation to service cell maintenance, growing points and reproductive development (Auzmendi and Hanan 2020). Tree non-structural carbohydrates are highest prior to flowering and then decline during this phenological phase (Scholefield et al. 1985). Likewise, fruit set is at least partially dependent on stored carbohydrates (Scholefield et al. 1985). Fruitlet abscission after mass flowering occurs early in fruit development, possibly because of a direct resource limitation, or as an adaptation to adjust crop load in line with tree resources (Trueman et al. 2022a).
Competition for C between fruit, vegetative development, and below ground biomass can have a significant influence on yield, even in evergreen canopies such as macadamia. Vegetative flushes can occur several times per year, with peaks in spring and later summer (Fig. 1). However, if new growth is excessive, energy stores for reproductive growth are compromised (McFadyen et al. 2011). For example, excessive nitrogen (N) application prolonged vegetative flushing and decreased first grade kernels by 8–10% (Stephenson et al. 2002). Likewise, new shoot growth, stimulated by pruning, increased fruit abscission and was associated with reduced stem carbohydrates (McFadyen et al. 2011). The timing of pruning can be critical to flowering and yield (Wilkie et al. 2009). However, if the plant’s carbohydrate status is adequate to sustain a high crop load, this may lead to higher kernel recovery (Wilkie 2009) and low kernel discolouration (Du Preez 2015). Herbert et al. (2019) found that branch girdling (cincturing), a technique to prevent carbohydrates from leaving or entering the branch through the removal of phloem, increased fruit set and yield. Similarly, assimilates were redirected from vegetative growth to priority sinks such as fruit development as evidenced by trunk girdling at anthesis, which reduced shoot growth without compromising yield (McFadyen et al. 2013a).
A better understanding of carbon allocation in response to environmental changes will facilitate the development of appropriate agronomic practices to maximise yield and quality (Fig. 2). The fluxes between above and belowground biomass pools, metabolic sinks such as respiration and root activity, as well as primary and secondary metabolites are complex and alter according to the stage of the reproductive cycle. The allocation of C for long-term storage in the woody tissues of large trees like macadamias that live for decades may allow them to withstand future stresses such as drought, when net C assimilation is low as a result of stomatal closure (Hartmann et al. 2020). The interactions between roots and the soil, from which they draw nutrients and water, are also likely to alter under various climate related stresses. For instance, rising temperature is decreasing the soil organic carbon stock through microbial stimulation and greater CO2 efflux, with ramifications for the chemical, physical and biological properties of the soil (Elbasiouny et al. 2022). Given the quantity and diversity of C molecules released from plant roots, in exchange for other resources such as nutrients (Selosse and Rousset 2011), fluxes in the population dynamics of root-associated microorganisms will likely have an impact on plant productivity. Therefore, the conservation of this C stock is an important component of sustainable land management.
Carbon competition between nut development and other sinks within the tree. Climate pressures such as cloud, heavy rain and the associated nutrient run-off, flooding, waterlogging, heat and water stress impact on how the non-structural carbohydrates (NSC) are allocated to the various growing and metabolically demanding sinks such as respiration, root nutrient and water uptake functions, and the formation of symbiotic relationships in the rhizosphere. NSCs may be stored and then mobilised to fuel areas of high demand when photosynthates are inadequate. Flower, ovule, and leaf abscission, as well as root senescence, represent a loss of plant C but can be incorporated into the soil as organic matter.
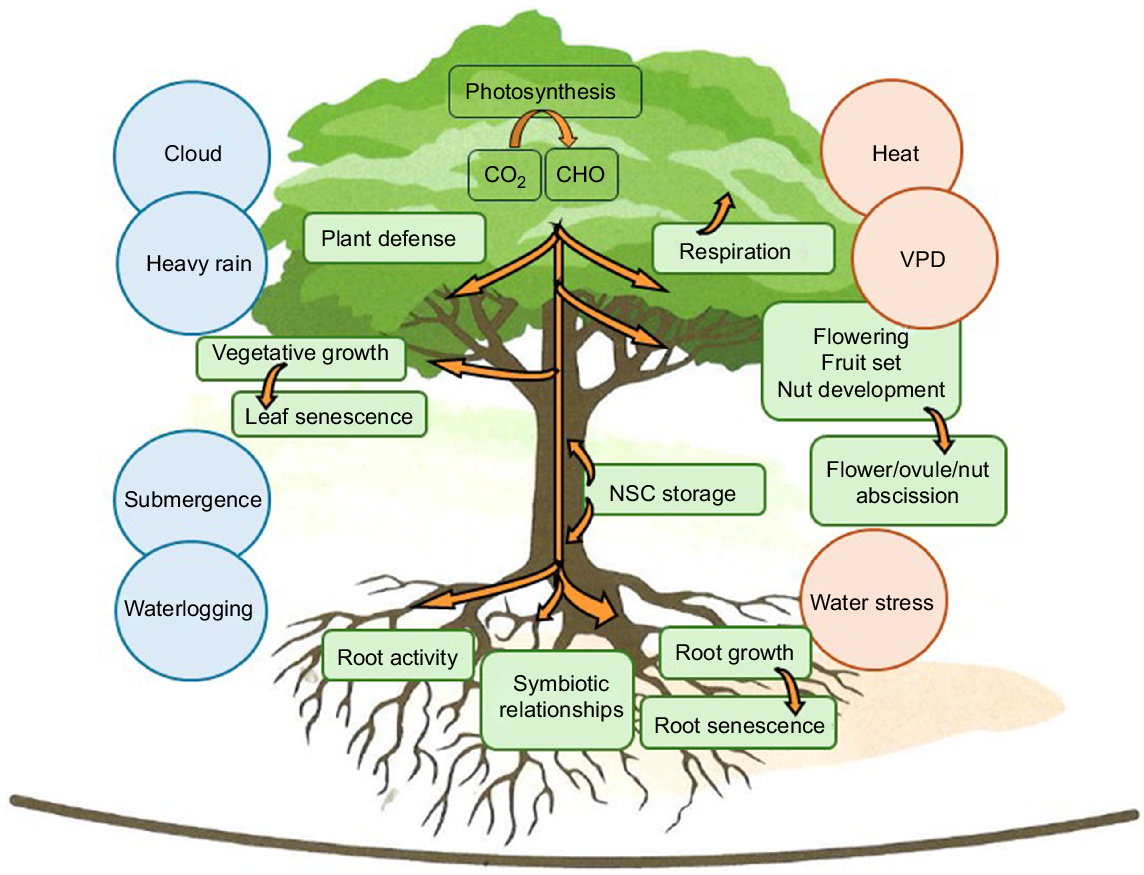
Solar radiation
Optimal light quality and duration are essential for producing high-quality nuts and maximising yield. This is partially because high light intensities are critical for optimal photosynthesis (Huett 2004), especially over the whole tree canopy. For example, cloudy conditions delay kernel filling and hence results in fruit quality concerns. Yield increases occur as canopy light interception increases (Huett 2004); notably higher yields occur in the upper part of the canopy. Low light incidence in the lower canopy generally supports new vegetative growth as opposed to reproductive growth (McFadyen et al. 2011), exacerbating reduced light penetration in the inner canopy. Ultimately yield per ha was compromised in orchard crowding (tree volumes >43,500 m3 ha−1 and light interception <94%); however, percentage kernel recovery, unsound kernel, or grade 1 kernel were not affected (McFadyen et al. 2004). Changes in light quality may be significant to changes in kernel development and yield. It has been demonstrated (Bastías and Corelli-Grappadelli 2012) that a low red-to-far-red (R/FR) ratio, such as occurs in the lowest canopy, can cause peach trees to elongate and become tall and spindly, resulting in reduced yield and quality.
The manipulation of light interception within the canopy, through various canopy management strategies can have an enormous effect on metabolic processes and whole plant physiology including water and carbon relations. The control of crop load and shading can influence kernel output, nut size, and quality. Hedging, limb removal, limb rejuvenation, skirting, row removal, stumping, topping or heading are practices that may be implemented at different stages of production to improve or stabilise yield and quality. These practices are labour and resource intensive and may result in temporary loss of production. An economic analysis is required to better understand the trade-offs between immediate costs and long-term gains in productivity. This analysis should also cover the trade-offs between intensive one-off practices such as row removal with slower incremental changes such as sequential limb removal over many seasons.
Heatwaves and drought
Climate change has resulted in temperature variability and the timing and quantity of precipitation (Hughes 2011). Macadamias originate from a sub-tropical climate, with mild winters and warm summers, and rainfall (1200–2000 mm) in distinct wet and dry seasons. High and low orchard temperatures are amongst the most significant environmental factors affecting macadamia kernel development. The minimum mean temperature for vegetative growth is approximately 12°C (Trochoulias and Lahav 1983), while temperatures above 30°C may be detrimental (Carr 2013; Shabalala et al. 2022). Macadamia’s native range extends south into the Northern Rivers of NSW, an important macadamia growing region. Here summer daytime temperatures typically reach 25–30°C, while winter night-time temperatures fall to 8–12°C. Maximum photosynthesis occurs at 16–25°C (Allan and de Jager 1979) and is severely curtailed at temperatures above 40°C (Allan and de Jager 1979). High temperatures during nut development can lead to reduced kernel weight, diminished kernel quality, and extensive kernel browning. A controlled-environment glasshouse study, exposing trees to a range of temperatures between 15 and 35°C, demonstrated that 25°C resulted in optimal oil content and kernel recovery, whereas these parameters were adversely affected at 35°C (Stephenson and Gallagher 1986b). Limited energy for nut development because of sub-optimal photosynthesis at this high temperature likely retarded oil accumulation. Moreover, the breakdown of respiratory substrates, important for oil accumulation, is increased during high temperatures (Stephenson and Gallagher 1986b).
Macadamias can withstand dry conditions to some extent (Smit et al. 2020). This tolerance can partially be attributed to their sclerophyllous leaves that are xeromorphic with sclerified bundle sheath tissues, and stomata that are limited to the abaxial surface (Stephenson et al. 1989b, 2003) restricting water loss from leaves. Stomatal conductance declines in response to dry soils and to increasing evaporative demand (Lloyd et al. 1991) so that the plant remains isohydric, at least during the non-fruiting stages (Smit et al. 2020). Because grafted macadamia trees have a relatively shallow root system with a short taproot and most fibrous roots in the upper 0.40 m of soil (Firth et al. 2003; Carr 2013), water stress can initiate quickly. However, macadamia’s unique cluster roots (formerly known as proteoid roots) may promote water uptake during dry periods (Stephenson and Trochoulias 1994), which typically occur after harvest. The cluster roots are formed near the soil surface and consist of many fine rootlets clustered together in a small area, making it highly efficient in absorbing pockets of water and nutrients. This root architecture is especially valuable to macadamias in their native environment which often experiences seasonal fluctuations in rainfall. However, water deficit during the early stage of nut development (predawn leaf water potential of −2.0 MPa) can lead to reduced fruit set and greater nut drop (Carr 2013), which ultimately decreases nut yield (Mayer et al. 2006). Stress during the kernel-filling stage can result in a lower kernel-to-nut ratio, smaller kernel size, more discoloured and shrivelled kernels and overall lower quality (Stephenson et al. 2003; Shabalala et al. 2022). Irrigation is thus an indispensable practice in water-deficient areas, as it maintains adequate water and nutrient availability, resulting in larger nuts with higher quality (Stephenson et al. 2003; Shabalala et al. 2022).
Disease and pests occurring under dry conditions may be alleviated by adequate irrigation, but saturated soil can exacerbate diseases such as Phytophthora (Dron et al. 2022) and promote insect damage (Bouarakia et al. 2023). There is a need for research on irrigation techniques to optimize water use efficiency, reduce water usage and minimise environmental impact. The development of accurate crop and plant sensors, smart irrigation controllers for automated irrigation schedules, as well as remote monitoring, will enhance efficiencies, along with micro-irrigation techniques and renewables-based irrigation systems to promote sustainability. Further research on cultivar differences in water-use efficiency is also required to improve macadamia sustainability. Crop coefficients to estimate water use, in combination with technology such as soil moisture probes, sap flow sensors and dendrometers, may offer cost effective grower solutions to ensure optimum plant water status is managed. Information on hydrological conditions such as infiltration and run-off are also important to achieving sustainable production.
To enhance plant resilience in challenging environmental conditions, various protective solutions are employed to mitigate water loss and stress. Antitranspirants, sunscreens, and film-forming barriers provide a thin protective coating over the canopy, preserving water and reducing evapotranspiration rate. These coatings can potentially decrease sunburn, a key driver of oxidative stress (Munné-Bosch and Vincent 2019). For example, kaolin clay had positive effects on kernel recovery in 6-year-old trees (Du Preez 2015); however, the impact of adverse environmental conditions must be considered and balanced with the potential negative impacts on photosynthesis. Sometimes these negative side effects are minimal or short lived (Rosati et al. 2007).
Waterlogging
Waterlogging, resulting from heavy rainfall or overirrigation, occurs when soils become saturated with water, which results in hypoxia in the soil restricting root respiration (Nguyen et al. 2018). Waterlogging is known to reduce the yield of macadamia nuts and diminishes the quality of the kernel. Trochoulias and Johns (1992) found a negative relationship between individual nut weight and irrigation amount. Reproductive development is hampered because waterlogging reduces nutrient uptake (Salvatierra et al. 2020), increases disease susceptibility, and constrains the growth and development of the tree, including the nuts from reduced carbon supply. Root growth may slow and there may even be root decay. In partial submergence the upper leaves of the plants may be retained so that photosynthesis is maintained. However, over the total canopy, decreased photosynthesis results in the reliance and depletion of starch reserves (Olesen et al. 2008) and contributes to plant death, when these stores are exhausted.
Given the general trend for changes in rainfall quantities and timing (Dey et al. 2019), site selection and preparation for flooding is central to the success of an orchard. The consideration of slope during site selection is critical to orchard productivity (Bright and Alt 2022). A mild slope can improve drainage but during excessive rainfall, soil erosion may occur. In contrast, low-lying flat land, without mounding or ridging and susceptible to flooding, will result in root disease and necrosis, and thus decreased overall productivity (Drenth et al. 2009). Hence, drainage management is critical after heavy rains because it helps maintain the topsoil whilst preventing waterlogging, and maintains machinery access such as mowers, slashers and harvesters (Bright and Alt 2022). These drainage considerations are best implemented prior to orchard planting.
Soil properties and nutrition
Macadamia prefers a loam or sandy-loam soil that is slightly acidic with a pHCa of 6.0 (Bright and Alt 2022). Zuza et al. (2023) found that if the soil pH in macadamia orchards is too low (<5.5), the availability of essential macronutrients (Ca2+, K+, P, and N) declines, leading to decreased kernel size, weight and sub-optimal quality (Bright and Alt 2022). Among the critical micronutrients for nut set and quality are boron (B) and zinc (Zn) (Stephenson et al. 1986; Nagao et al. 1992). A recent study by De Silva et al. (2022) showed that while B can improve initial fruit set in cv. 816, it did not significantly influence yield or nut quality. Other cultivars were not tested. There is a need for research on critical nutrient thresholds, timing, form, movement and methods of nutrient application to optimize tree growth and yield while maintaining soil health and reducing nutrient loss from the orchard. Information on the interactions between the nutrients (e.g. cation antagonism) and nutrient dynamics in different soil types is limited. Once inside the plant, the specific macro or micronutrient will have an important influence on whole plant and nut physiology. In many agricultural industries, rapid, non-destructive tools for assessing plant nutrient status are available. For example, hyperspectral imaging of leaves has already shown potential for rapid nutrient assessment and requires further development for better accuracy and for a greater range of nutrients (De Silva et al. 2023).
Macadamia trees form cluster roots, which are particularly efficient in phosphorus (P) uptake, especially when the plant is P-deficient (Aitken et al. 1992; Hue 2009; Zhao et al. 2019, 2021). The role of cluster roots in the uptake of other nutrients, including N, requires investigation. Excessive N during oil production may lead to excessive vegetative growth, which competes with nut development (Stephenson et al. 2002). All four macadamia species are also high manganese (Mn) accumulators (Abubakari et al. 2022; Zhao et al. 2023), likely due to the exudates from cluster roots that not only solubilise P but also several micronutrients (Lambers et al. 2015). Conversely, Moodley et al. (2007) found that the uptake of metals and their distribution to the nuts were largely controlled by the plant’s inherent mechanisms and were low for toxic metals. Further investigation into how the presence of cluster roots alters soil chemistry and microbial activity will enhance the ability of macadamias to thrive in nutrient-poor soils, reducing the need for synthetic fertilisers.
A well-structured soil with good porosity is vital for proper root development and nutrient uptake, both of which are essential for healthy nut growth. Van Zwieten et al. (2003) observed that older macadamia orchards in NSW exhibited signs of soil compaction, erosion, and reduced organic carbon, especially in conventional farms, whereas these issues were less prominent in organic orchards. Practices like mulching and incorporating compost can enhance soil porosity and fertility. For instance, the application of poultry litter biochar in macadamia orchards has been shown to increase soil fertility, likely due to its slow-release fertiliser properties (Hosseini Bai et al. 2015). Assessments of different mulch and compost types, including the effects of temperature and moisture on decomposition rates and nutrient release rates, will help optimise their use. Likewise, carbon sequestration through mulching and composting requires further exploration. Moreover, research on the cost-effectiveness and scalability of specific soil amendments are required, especially in established orchards as previously alluded to.
Effective orchard floor management plays a key role in preventing soil erosion. One particular challenge in macadamia orchards is stem flow, which is the movement of water along the outside of the tree trunk that accumulates and contributes significantly to soil erosion at the tree base (Levia and Germer 2015). This is particularly challening during rainfall. To mitigate erosion, light hedging can promote light penetration to the orchard floor, encouraging the growth of ground cover and reducing soil loss (McFadyen et al. 2013b). In older orchards with low light conditions (Firth et al. 2002), pasture species are suitable for ground cover. However, from a farmer’s perspective, the orchard floor must also support efficient nut harvesting. Large mechanical harvesters rely on sweeping nuts into bins, a process that can be hindered by excessive vegetation and debris. Finding the right balance between orchard floor conditions and mechanical harvesting may be site specific and require experimentation and adjustments through trial and error.
Pest and disease pressure
Macadamia nut pests and diseases can significantly diminish the quality of the kernel. Ellis et al. (2023) have emphasised increased interest in integrated pest and disease control in the macadamia industry. However, shifts in frequency and intensity of precipitation, as well as temperature, may alter the dynamics between pests and their natural predators, compromising integrated pest management. Furthermore, the direct and indirect effects of climate change drive changes in the temporal and spatial distribution of insects and microbes (Skendžić et al. 2021). Temperature is especially important to population dynamics (Zvereva and Kozlov 2006) because it affects the metabolic rates, development, and reproduction of both insects and microbes. For instance, many insect species have specific temperature thresholds for development, and as temperatures rise, these species may experience accelerated growth rates, shorter life cycles, and increased reproductive output. Additionally, other climatic effects such as water and heat stress reduce the plant’s resilience so that they are more susceptible to pests and diseases. Examples include the fruit spotting bug and the banana-spotting bug (Amblypelta spp.), the foremost cause of nut rejection at the factory (Bright 2019). They feed on the kernel tissue from pea size until harvest, causing it to become discoloured, misshapen and translucent. The fruit spotting bug and the macadamia nut borer (Cryptophlebia spp.), another pest of great concern in the industry (Smith et al. 2022), can cause nuts to fall prematurely. Amblypelta spp. pierce young nuts and these drop readily, whereas older pierced nuts tend to stay on the tree (Ellis et al. 2023). The green vegetable bug (Nezara viridula) causes similar damage to the kernel but there are no external symptoms (Bright 2019). Adults inject digestive fluids into the kernel, causing entry points for pathogens and contributing to rancidity (Jamieson et al. 2004). Diversifying understory plants in macadamia orchards provides habitats for beneficial insects, which can help control pests like the felted coccid (Eriococcus ironsidei) (Gutierrez-Coarite et al. 2018). Reduced mowing, combined with the removal of unwanted weeds, has also been shown to increase non-pest arthropod numbers in orchards (Bright 2019), thereby promoting natural pest control.
Fungal diseases are common following wet weather and are more likely in dense, shaded canopies, as well as the upper zone of tall trees outside of spray coverage. Disease incidence can lead to kernel necrosis or discolouration, diminishing both kernel weight and quality (Doster and Michailides 1999). Husk spot can cause a significant increase in immature rejects (Nunn et al. 2023). Phytophthora root rot can reduce kernel quality by causing the tree to produce smaller, lower-quality nuts (Hunter et al. 1971). Husk spot results in immature abscission and therefore reduces yields (Akinsanmi et al. 2008). Frequent monitoring of weather, orchard conditions and pest presence will be required, alongside predictive modelling to prepare for incursions. An environmentally conscious integrated pest and disease management program that is responsive to weather variability will reduce the risk of significant economic losses, when the necessary research is completed to facilitate such a program.
Kernel harvest and storage conditions
Effects of harvest delays
Aside from the phenology of crop growth, climate change has an impact on the entire food system, including harvesting, post-harvest handling, and storage. If not managed appropriately, these factors contribute to wastage through losses in quality and spoilage. For instance, unseasonal heavy rains can hamper orchard accessibility by farm equipment such as harvesters and can contribute to a delayed harvest. Prolonged exposure on the orchard floor will result in sun damage, fungal diseases, viviparous germination, or other physiological changes within the nut. Viviparous germination is more likely to occur during sunlight exposure and warm temperatures (Quinlan et al. 2008). Enzymatic browning, leading to higher levels of discolouration, is the result of movement of tannin from the shell (Penter et al. 2007; Walton and Wallace 2009). Apparently, the more water is available in the nut, the higher the rate of this enzymatic browning (Borompichaichartkul et al. 2009). It is unclear if this a direct effect of rain or relative humidity, or how wet the nuts are before going into storage. It was noted that frequent harvesting (less than every 4 weeks) improves sound kernel recovery (Liang et al. 1996; Quinlan et al. 2008). Once harvested, it is critical that the husk is removed from the rest of the nut within 24 h of harvest. Its presence initiates the translocation of sugars in preparation for germination and will result in the formation of a dark halo around the circumference of the nut (Mereles et al. 2022).
Moisture content and storage conditions
During storage, nuts with high moisture content are more likely to undergo kernel browning, possibly because of higher metabolic activity (Walton et al. 2013). Silo size and shape can have an influence on rates of drying. For example, nuts stored in smaller silos (less than 20 tonnes) had reduced unsound kernel recovery relative to larger silos (Quinlan et al. 2008). Depth of nuts within the silo as well as efficiency of air flow through the bed of nuts is also important. Nuts are typically harvested with a 20–25% moisture content, and rapid drying to 10% moisture is recommended prior to transport for processing to alleviate enzyme activity, chemical reactions or microbial activity (Wall and Gentry 2007; Walton and Wallace 2010). Nuts are further dried down to 3.5% moisture content before the kernel is removed from the shell (Duduzile Buthelezi et al. 2019). By contrast, nuts that are stored in high humidity or moisture conditions can cause the nut to stick to the shell, resulting in a lower kernel recovery rate. High temperature storage can lead to an increase in free fatty acids, resulting in the loss of vitamin E and other antioxidants that are important for retaining the quality of macadamia nuts. Vitamin E is known for its antioxidant properties and plays a crucial role in inhibiting lipid oxidation in nuts. Some research has found that storage temperatures higher than 25°C can lead to a reduction in vitamin E content in macadamia nuts (Cavaletto et al. 1966). Temperature fluctuations during storage can also affect the quality of macadamia kernels. Exposure to fluctuating temperatures can cause moisture migration within the nut, which can lead to quality deterioration such as rancidity and discolouration. Therefore, macadamia processors should store kernels at a constant low temperature (around 4°C) to preserve their quality and freshness.
Roasting and its impact on nut quality
Despite disagreement by processors on the shelf life of raw versus roasted nuts, roasting of kernels results in higher flavonoid, phenols and antioxidant activity and can improve the sensory quality and shelf life of macadamia (Duduzile Buthelezi et al. 2019). Roasting results in crisper kernels (Tu et al. 2021); however, over-roasting can cause the kernels to become dry and brittle, whereas under-roasting can result in a softer, chewier texture (Aruwajoye et al. 2023). Browning during roasting may be a consequence of the Maillard reaction resulting from high reducing sugar and amino acid content of the kernel (Srichamnong and Srzednicki 2015). Roasting may thus result in brown centres if the nuts are not fully mature and still high in sugar content (Wall and Gentry 2007). The high sugar content, combined with differing temperature and moisture between inner and outer parts of the kernel, may result in brown centres (Wall and Gentry 2007). After-roast darkening may occur because of enzymatic changes (cell wall invertase) in sugar composition driven by a loss in membrane integrity through high incubation temperatures (Albertson et al. 2006). Kernels susceptible to after-roast darkening were found to contain higher glucose and fructose concentrations relative to cream-coloured kernels. The processing of immature kernels with high sugar levels should thus be avoided, and sugar assessments at harvest may be warranted.
Advanced methods for assessing nut quality
Traditional wet chemistry methods to assess nut quality can be costly and time consuming and may require expensive laboratory equipment and the associated expertise. Accurate, simple and cost-effective technologies targeted at kernel discolouration, lipid and moisture content aids in the detection of sub-standard nuts. The Australian industry predominantly uses colour (RGB) sorters to assess nut defects but is currently also accepting X-ray technology for sorting nuts in shell at both farm and processor levels. The development of AI in sorting and defect identification has progressed rapidly and is often used in conjunction with a range of spectroscopic methods. For instance, nut internal defects can be detected with Near InfraRed (NIR) spectroscopy (Guthrie et al. 2004) and chemometric modelling (Rahman et al. 2021). Furthermore, nuclear magnetic resonance (NMR) was also useful for detecting external defects in unshelled macadamia kernels (Carvalho et al. 2019); however, high cost and complexity of data interpretation are major drawbacks. Meanwhile, hyperspectral imaging and machine learning was used to detect kernel moisture content, with the advantages in that it is simpler, non-destructive, and faster than the oven-drying technique during post-harvest processing (Farrar et al. 2024). However, some of these promising techniques require further development before they can be used at the commercial scale.
Summary
The consumption of macadamia nuts has increased considerably because of greater awareness of their nutritional and flavour value. Pre-harvest stress resulting from extreme weather can significantly influence macadamia nut and kernel development and quality by altering various physiological and metabolic processes both in the tree and nut itself. These stresses can ultimately lead to reduced nut yield, changes in kernel composition, and altered shelf life (Fig. 3). Along with appropriate genetic material, it is essential for macadamia growers to carefully manage orchard conditions to ensure optimal nut production and quality. New approaches in orchard management, nut processing and storage will improve overall quality in a more environmentally friendly manner. Directing low-quality kernels and shells to other industries such as cosmetics, heating or mulching will increase their utility and contribute to the circular economy. Prioritising research and development, increasing grower awareness through information exchange, combined with the formulation of appropriate polices, will contribute to productivity at the orchard level.
References
Abubakari F, Nkrumah PN, Fernando DR, Erskine PD, van der Ent A (2022) Manganese accumulation and tissue-level distribution in Australian Macadamia (Proteaceae) species. Environmental and Experimental Botany 193, 104668.
| Crossref | Google Scholar |
Aitken RL, Moody PW, Compton BL, Gallagher EC (1992) Plant and soil diagnostic tests for assessing the phosphorus status of seedling Macadamia integrifolia. Australian Journal of Agricultural Research 43(1), 191-201.
| Crossref | Google Scholar |
Akinsanmi OA, Miles AK, Drenth A (2008) Alternative fungicides for controlling husk spot caused by Pseudocercospora macadamiae in macadamia. Australasian Plant Pathology 37, 141-147.
| Crossref | Google Scholar |
Albertson PL, Bursle JK, Forrester RI, McConchie CA (2006) Hexose synthesis by cell wall invertase activity and its effects on the roasting behaviour of macadamia kernel. Australian Journal of Agricultural Research 57(1), 47-54.
| Crossref | Google Scholar |
Ali MA, Abdellah IM, Eletmany MR (2023) Climate change impacts on honeybee spread and activity: a scientific review. Chelonian Research Foundation 18(2), 531-554.
| Google Scholar |
Allan P, de Jager J (1979) Net photosynthesis in macadamia and papaw and the possible alleviation of heat stress. Acta Horticulturae 102, 23-30.
| Crossref | Google Scholar |
Anders M, Grass I, Linden VMG, Taylor PJ, Westphal C (2023) Smart orchard design improves crop pollination. Journal of Applied Ecology 60(4), 624-637.
| Crossref | Google Scholar |
Aruwajoye NN, Buthelezi NMD, Mditshwa A, Tesfay SZ, Magwaza LS (2023) Assessing the impact of roasting temperatures on biochemical and sensory quality of macadamia nuts (Macadamia integrifolia). Foods 12(11), 2116.
| Crossref | Google Scholar |
Auzmendi I, Hanan JS (2020) Investigating tree and fruit growth through functional–structural modelling: implications of carbon autonomy at different scales. Annals of Botany 126(4), 775-788.
| Crossref | Google Scholar |
Bastías RM, Corelli-Grappadelli L (2012) Light quality management in fruit orchards: physiological and technological aspects. Chilean Journal of Agricultural Research 72(4), 574-581.
| Crossref | Google Scholar |
Borompichaichartkul C, Luengsode K, Chinprahast N, Devahastin S (2009) Improving quality of macadamia nut (Macadamia integrifolia) through the use of hybrid drying process. Journal of Food Engineering 93(3), 348-353.
| Crossref | Google Scholar |
Bouarakia O, Anders M, Linden VMG, Grass I, Westphal C, Taylor PJ, Foord SH (2023) Reduced macadamia nut quality is linked to wetter growing seasons but mitigated at higher elevations. Journal of Agriculture and Food Research 12, 100569.
| Crossref | Google Scholar |
Buthelezi NMD, Tesfay SZ, Magwaza LS (2021) Influence of roasting on antioxidants, fatty acids, sensory properties and oxidative stability of macadamia nuts. Scientia Horticulturae 278, 109850.
| Crossref | Google Scholar |
Cacka J, Sanguankeo P (2014) Calcium influence on hazelnut quality and yields in Oregon. Acta Horticulturae 1052, 187-193.
| Crossref | Google Scholar |
Carr MKV (2013) The water relations and irrigation requirements of macadamia (Macadamia spp.): a review. Experimental Agriculture 49(1), 74-90.
| Crossref | Google Scholar |
Carvalho LCd, Verbi Pereira FM, Morais CdLMd, Lima KMGd, Teixeira GHdA (2019) Assessment of macadamia kernel quality defects by means of near infrared spectroscopy (NIRS) and nuclear magnetic resonance (NMR). Food Control 106, 106695.
| Crossref | Google Scholar |
Cavaletto C, Cruz AD, Ross E, Yamamoto HY (1966) Factors affecting macadamia nut stability. I. Raw kernel. Food Technology 20, 108-111.
| Google Scholar |
Classen A, Peters MK, Kindeketa WJ, Appelhans T, Eardley CD, Gikungu MW, Hemp A, Nauss T, Steffan-Dewenter I (2015) Temperature versus resource constraints: which factors determine bee diversity on Mount Kilimanjaro, Tanzania? Global Ecology and Biogeography 24(6), 642-652.
| Crossref | Google Scholar |
Crain WO, Jr, Tang CS (1975) Volatile components of roasted macadamia nuts. Journal of Food Science 40(1), 207-208.
| Crossref | Google Scholar |
Dahler JM, Mcconchie C, Turnbull CGN (1995) Quantification of cyanogenic glycosides in seedlings of three Macadamia (Proteaceae) species. Australian Journal of Botany 43(6), 619-628.
| Crossref | Google Scholar |
De Silva AL, Kämper W, Wallace HM, Ogbourne SM, Hosseini Bai S, Nichols J, Trueman SJ (2022) Boron effects on fruit set, yield, quality and paternity of macadamia. Agronomy 12(3), 684.
| Crossref | Google Scholar |
De Silva AL, Trueman SJ, Kämper W, Wallace HM, Nichols J, Hosseini Bai S (2023) Hyperspectral imaging of adaxial and abaxial leaf surfaces as a predictor of macadamia crop nutrition. Plants 12(3), 558.
| Crossref | Google Scholar |
Dey R, Lewis SC, Arblaster JM, Abram NJ (2019) A review of past and projected changes in Australia’s rainfall. WIREs Climate Change 10(3), e577.
| Crossref | Google Scholar |
Doster MA, Michailides TJ (1999) Relationship between shell discoloration of pistachio nuts and incidence of fungal decay and insect infestation. Plant Disease 83(3), 259-264.
| Crossref | Google Scholar | PubMed |
Drenth A, Akinsanmi OA, Miles A (2009) Macadamia diseases in Australia. Southern African Macadamia Growers’ Association Yearbook 17, 48-52.
| Google Scholar |
Dron N, Simpfendorfer S, Sutton T, Pengilley G, Hobson K (2022) Cause of death: phytophthora or flood? Effects of waterlogging on Phytophthora medicaginis and resistance of chickpea (Cicer arietinum). Agronomy 12(1), 89.
| Crossref | Google Scholar |
Duduzile Buthelezi NM, Samukelo Magwaza L, Zeray Tesfay S (2019) Postharvest pre-storage processing improves antioxidants, nutritional and sensory quality of macadamia nuts. Scientia Horticulturae 251, 197-208.
| Crossref | Google Scholar |
Elbasiouny H, El-Ramady H, Elbehiry F, Rajput VD, Minkina T, Mandzhieva S (2022) Plant nutrition under climate change and soil carbon sequestration. Sustainability 14(2), 914.
| Crossref | Google Scholar |
Ellis KL, Anderson JM, Yonow T, Kriticos DJ, Andrew NR (2023) Biology and ecology of insect pests in macadamia: a review of the current status of IPM strategies in Australia. Journal of Integrated Pest Management 14(1), 26.
| Crossref | Google Scholar |
Evans LJ, Jesson L, Read SFJ, Jochym M, Cutting BT, Gayrard T, Jammes MAS, Roumier R, Howlett BG (2021) Key factors influencing forager distribution across macadamia orchards differ among species of managed bees. Basic and Applied Ecology 53, 74-85.
| Crossref | Google Scholar |
Farrar MB, Omidvar R, Nichols J, Pelliccia D, Lateef Al-Khafaji S, Tahmasbian I, Hapuarachchi N, Hosseini Bai S (2024) Hyperspectral imaging predicts macadamia nut-in-shell and kernel moisture using machine vision and learning tools. Computers and Electronics in Agriculture 224, 109209.
| Crossref | Google Scholar |
Firth DJ, Jones RM, McFadyen LM, Cook BG, Whalley RBD (2002) Selection of pasture species for groundcover suited to shade in mature macadamia orchards in sub-tropical Australia. Tropical Grasslands 36(1), 1-12.
| Google Scholar |
Firth DJ, Whalley RDB, Johns GG (2003) Distribution and density of the root system of macadamia on krasnozem soil and some effects of legume groundcovers on fibrous root density. Australian Journal of Experimental Agriculture 43(5), 503-514.
| Crossref | Google Scholar |
Gama T, Wallace HM, Trueman SJ, Hosseini-Bai S (2018) Quality and shelf life of tree nuts: a review. Scientia Horticulturae 242, 116-126.
| Crossref | Google Scholar |
Gama T, Wallace HM, Trueman SJ, Jones K, Hosseini-Bai S (2020) Late-dropping macadamia nuts have reduced shelf life. Scientia Horticulturae 268, 109378.
| Crossref | Google Scholar |
Guthrie J, Greensill C, Bowden R, Walsh K (2004) Assessment of quality defects in macadamia kernels using NIR spectroscopy. Australian Journal of Agricultural Research 55(4), 471-476.
| Crossref | Google Scholar |
Gutierrez-Coarite R, Mollinedo J, Cho A, Wright MG (2018) Canopy management of macadamia trees and understory plant diversification to reduce macadamia felted coccid (Eriococcus ironsidei) populations. Crop Protection 113, 75-83.
| Crossref | Google Scholar |
Hardner C, Winks C, Stephenson R, Gallagher E (2001) Genetic parameters for nut and kernel traits in macadamia. Euphytica 117, 151-161.
| Crossref | Google Scholar |
Hartmann H, Bahn M, Carbone M, Richardson AD (2020) Plant carbon allocation in a changing world–challenges and progress: introduction to a Virtual Issue on carbon allocation. New Phytologist 227(4), 981-988.
| Crossref | Google Scholar | PubMed |
Herbert SW, Walton DA, Wallace HM (2019) The influence of pollen-parent and carbohydrate availability on macadamia yield and nut size. Scientia Horticulturae 251, 241-246.
| Crossref | Google Scholar |
Hosseini Bai S, Xu C-Y, Xu Z, Blumfield TJ, Zhao H, Wallace H, Reverchon F, Van Zwieten L (2015) Soil and foliar nutrient and nitrogen isotope composition (δ15N) at 5 years after poultry litter and green waste biochar amendment in a macadamia orchard. Environmental Science and Pollution Research 22, 3803-3809.
| Crossref | Google Scholar |
Howlett BG, Nelson WR, Pattemore DE, Gee M (2015) Pollination of macadamia: review and opportunities for improving yields. Scientia Horticulturae 197, 411-419.
| Crossref | Google Scholar |
Howlett BG, Read SFJ, Alavi M, Cutting BT, Nelson WR, Goodwin RM, Cross S, Thorp TG, Pattemore DE (2019) Cross-pollination enhances macadamia yields, even with branch-level resource limitation. HortScience 54(4), 609-615.
| Crossref | Google Scholar |
Hu W, Fitzgerald M, Topp B, Alam M, O’Hare TJ (2019) A review of biological functions, health benefits, and possible de novo biosynthetic pathway of palmitoleic acid in macadamia nuts. Journal of Functional Foods 62, 103520.
| Crossref | Google Scholar |
Hu W, Fitzgerald M, Topp B, Alam M, Pun S, Liu D, Torrisi C, O’Hare TJ (2023) The effects of pollen source on the fatty acid profile of Macadamia kernels. Journal of Food Composition and Analysis 115, 104943.
| Crossref | Google Scholar |
Hue NV (2009) Iron and phosphorus fertilizations and the development of proteoid roots in macadamia (Macadamia integrifolia). Plant and Soil 318, 93-100.
| Crossref | Google Scholar |
Huett DO (2004) Macadamia physiology review: a canopy light response study and literature review. Australian Journal of Agricultural Research 55(6), 609-624.
| Crossref | Google Scholar |
Hughes L (2011) Climate change and Australia: key vulnerable regions. Regional Environmental Change 11, 189-195.
| Crossref | Google Scholar |
Hunter JE, Kunimoto RK, Rohrbach KG (1971) Phytophthora blight, a new disease of macadamia. Phytopathology 61, 1130-1134.
| Crossref | Google Scholar |
Insanu M, Hartati R, Bajri F, Fidrianny I (2021) Macadamia genus: an updated review of phytochemical compounds and pharmacological activities. Biointerface Research in Applied Chemistry 11, 14898-14905.
| Crossref | Google Scholar |
Jamieson LE, Dawson T, Seldon D, Froud KJ (2004) Green vegetable bug on macadamia nuts – a sustainable pest management system. The Tree Cropper 38, 13-16.
| Google Scholar |
Jones WW (1939) A study of developmental changes in composition of the macadamia. Plant Physiology 14(4), 755-768.
| Crossref | Google Scholar | PubMed |
Jones WW, Shaw L (1943) The process of oil formation and accumulation in the macadamia. Plant Physiology 18(1), 1-7.
| Crossref | Google Scholar | PubMed |
Kaijser A, Dutta P, Savage G (2000) Oxidative stability and lipid composition of macadamia nuts grown in New Zealand. Food Chemistry 71(1), 67-70.
| Crossref | Google Scholar |
Knight TM, Steets JA, Ashman T-L (2006) A quantitative synthesis of pollen supplementation experiments highlights the contribution of resource reallocation to estimates of pollen limitation. American Journal of Botany 93(2), 271-277.
| Crossref | Google Scholar | PubMed |
Koaze H, Karanja PN, Kojima M, Baba N, Ishibashi K-I (2002a) Lipid accumulation of macadamia nuts during kernel development. Food Preservation Science 28(2), 67-73.
| Crossref | Google Scholar |
Koaze H, Ndaka DS, Karanja PN, Ishibashi K-I, Baba N (2002b) Changes in quality of dried macadamia nuts during a peak harvest season in Kenya. Food Science and Technology Research 8, 32-35.
| Crossref | Google Scholar |
Kunz A, Blanke M (2022) “60 years on” – effects of climatic change on tree phenology – a case study using pome fruit. Horticulturae 8(2), 110.
| Crossref | Google Scholar |
Lambers H, Hayes PE, Laliberté E, Oliveira RS, Turner BL (2015) Leaf manganese accumulation and phosphorus-acquisition efficiency. Trends in Plant Science 20(2), 83-90.
| Crossref | Google Scholar | PubMed |
Langdon KS, King GJ, Nock CJ (2019) DNA paternity testing indicates unexpectedly high levels of self-fertilisation in macadamia. Tree Genetics & Genomes 15, 29.
| Crossref | Google Scholar |
Le Lagadec MD (2009) Kernel brown centres in macadamia: a review. Crop & Pasture Science 60(12), 1117-1123.
| Crossref | Google Scholar |
Levia DF, Germer S (2015) A review of stemflow generation dynamics and stemflow-environment interactions in forests and shrublands. Reviews of Geophysics 53(3), 673-714.
| Crossref | Google Scholar |
Liang T, Meng Q, Ji F (1996) Prediction of macadamia nut spoilage for harvest decision making. Journal of Agricultural Engineering Research 63(3), 237-242.
| Crossref | Google Scholar |
Lin J, Zhang W, Zhang X, Ma X, Zhang S, Chen S, Wang Y, Jia H, Liao Z, Lin J, Zhu M, Xu X, Cai M, Zeng H, Wan J, Yang W, Matsumoto T, Hardner C, Nock CJ, Ming R (2022) Signatures of selection in recently domesticated macadamia. Nature Communications 13(1), 242.
| Crossref | Google Scholar |
Lloyd J, Trochoulias T, Ensbey R (1991) Stomatal responses and whole-tree hydraulic conductivity of orchard Macadamia integrifolia under irrigated and non-irrigated conditions. Functional Plant Biology 18(6), 661-671.
| Crossref | Google Scholar |
Mai TTP, Hardner CM, Alam MM, Henry RJ, Topp BL (2021) Phenotypic characterisation for growth and nut characteristics revealed the extent of genetic diversity in wild macadamia germplasm. Agriculture 11(7), 680.
| Crossref | Google Scholar |
Martinez M, Wallace HM, Searle C, Elliott B, Hosseini Bai S (2023) Chemical differences between brown centre and white macadamia kernels. Journal of Agriculture and Food Research 14, 100878.
| Crossref | Google Scholar |
Martinez M, Wallace HM, Searle C, Elliott B, Hosseini Bai S (2024) Understanding chemical pathways of brown centre formation in laboratory induced and conventionally dried nut-in-shell macadamia kernels. Heliyon 10(3), e25221.
| Crossref | Google Scholar |
Mayer DG, Stephenson RA, Jones KH, Wilson KJ, Bell DJD, Wilkie J, Lovatt JL, Delaney KE (2006) Annual forecasting of the Australian macadamia crop–integrating tree census data with statistical climate-adjustment models. Agricultural Systems 91(3), 159-170.
| Crossref | Google Scholar |
McFadyen LM, Morris SG, Oldham MA, Huett DO, Meyers NM, Wood J, McConchie CA (2004) The relationship between orchard crowding, light interception, and productivity in macadamia. Australian Journal of Agricultural Research 55(10), 1029-1038.
| Crossref | Google Scholar |
McFadyen LM, Robertson D, Sedgley M, Kristiansen P, Olesen T (2011) Post-pruning shoot growth increases fruit abscission and reduces stem carbohydrates and yield in macadamia. Annals of Botany 107(6), 993-1001.
| Crossref | Google Scholar | PubMed |
McFadyen L, Robertson D, Sedgley M, Kristiansen P, Olesen T (2013a) Effects of girdling on fruit abscission, yield and shoot growth in macadamia. Scientia Horticulturae 164, 172-177.
| Crossref | Google Scholar |
McFadyen L, Robertson D, Sedgley M, Kristiansen P, Olesen T (2013b) Production trends in mature macadamia orchards and the effects of selective limb removal, side-hedging, and topping on yield, nut characteristics, tree size, and economics. HortTechnology 23(1), 64-73.
| Crossref | Google Scholar |
Moodley R, Kindness A, Jonnalagadda SB (2007) Chemical composition of edible Macadamia nuts (Macadamia integrifolia) and impact of soil quality. Journal of Environmental Science and Health, Part A 42(14), 2097-2104.
| Crossref | Google Scholar |
Munné-Bosch S, Vincent C (2019) Physiological mechanisms underlying fruit sunburn. Critical Reviews in Plant Sciences 38(2), 140-157.
| Crossref | Google Scholar |
Nagao MA, Hirae HH, Stephenson RA (1992) Macadamia: cultivation and physiology. Critical Reviews in Plant Sciences 10(5), 441-470.
| Crossref | Google Scholar |
Nguyen LTT, Osanai Y, Anderson IC, Bange MP, Braunack M, Tissue DT, Singh BK (2018) Impacts of waterlogging on soil nitrification and ammonia-oxidizing communities in farming system. Plant and Soil 426, 299-311.
| Crossref | Google Scholar |
Nock CJ, Hardner CM, Montenegro JD, Ahmad Termizi AA, Hayashi S, Playford J, Edwards D, Batley J (2019) Wild origins of macadamia domestication identified through intraspecific chloroplast genome sequencing. Frontiers in Plant Science 10, 334.
| Crossref | Google Scholar |
Nunn J, Hardner C, De Faveri J, Akinsanmi OA, O’Connor K, Alam M, Topp B (2023) Genetic parameters of husk spot resistance in macadamia breeding families. Euphytica 219(5), 54.
| Crossref | Google Scholar |
Olesen T, Robertson D, Muldoon S, Meyer R (2008) The role of carbohydrate reserves in evergreen tree development, with particular reference to macadamia. Scientia Horticulturae 117(1), 73-77.
| Crossref | Google Scholar |
Penter M, Nkwana E, Nxundu Y, Kruger FJ (2007) An investigation into the occurrence of kernel discolouration in the ‘Beaumont’ cultivar. South African Macadamia Growers Association Yearbook 15, 9-12.
| Google Scholar |
Penter MG, Nkwana E, Nxundu Y (2008) Factors influencing kernel breakage in the South African macadamia industry. South African Macadamia Growers Association Yearbook 16, 6-10.
| Google Scholar |
Quinlan K, Treverrow N, O’Hare P, Slaughter G, Mason R, Wallace HM (2008) Adoption of quality management systems in macadamia. Horticulture Australia Final report, MC03008. Available at https://www.horticulture.com.au/globalassets/hort-innovation/historic-reports/adoption-of-quality-management-systems-in-macadamia-mc03008.pdf
Rahman A, Wang S, Yan J, Xu H (2021) Intact macadamia nut quality assessment using near-infrared spectroscopy and multivariate analysis. Journal of Food Composition and Analysis 102, 104033.
| Crossref | Google Scholar |
Rengel A, Pérez E, Piombo G, Ricci J, Servent A, Tapia MS, Gibert O, Montet D (2015) Lipid profile and antioxidant activity of macadamia nuts (Macadamia integrifolia) cultivated in Venezuela. Natural Science 7, 535-547.
| Crossref | Google Scholar |
Richards TE, Kämper W, Trueman SJ, Wallace HM, Ogbourne SM, Brooks PR, Nichols J, Hosseini Bai S (2020) Relationships between nut size, kernel quality, nutritional composition and levels of outcrossing in three macadamia cultivars. Plants 9(2), 228.
| Crossref | Google Scholar |
Rogiers SY, Greer DH, Liu Y, Baby T, Xiao Z (2022) Impact of climate change on grape berry ripening: an assessment of adaptation strategies for the Australian vineyard. Frontiers in Plant Science 13, 1094633.
| Crossref | Google Scholar |
Rosati A, Metcalf SG, Buchner RP, Fulton AE, Lampinen BD (2007) Effects of kaolin application on light absorption and distribution, radiation use efficiency and photosynthesis of almond and walnut canopies. Annals of Botany 99(2), 255-263.
| Crossref | Google Scholar | PubMed |
Salvatierra A, Toro G, Mateluna P, Opazo I, Ortiz M, Pimentel P (2020) Keep calm and survive: adaptation strategies to energy crisis in fruit trees under root hypoxia. Plants 9(9), 1108.
| Crossref | Google Scholar |
Santos RdS, Milfont MdO, Silva MM, Carneiro LT, Castro CC (2020) Butterflies provide pollination services to macadamia in northeastern Brazil. Scientia Horticulturae 259, 108818.
| Crossref | Google Scholar |
Savage GP, McNeil DL, Dutta PC (1997) Lipid composition and oxidative stability of oils in hazelnuts (Corylus avellana L.) grown in New Zealand. Journal of the American Oil Chemists’ Society 74(6), 755-759.
| Crossref | Google Scholar |
Savage GP, Dutta PC, McNeil DL (1999) Fatty acid and tocopherol contents and oxidative stability of walnut oils. Journal of the American Oil Chemists’ Society 76(9), 1059-1063.
| Crossref | Google Scholar |
Scholefield PB, Sedgley M, Alexander DME (1985) Carbohydrate cycling in relation to shoot growth, floral initiation and development and yield in the avocado. Scientia Horticulturae 25(2), 99-110.
| Crossref | Google Scholar |
Sedgley M (1983) Pollen tube growth in macadamia. Scientia Horticulturae 18(4), 333-341.
| Crossref | Google Scholar |
Sedgley M, Bell FDH, Bell D, Winks CW, Pattison SJ, Hancock TW (1990) Self- and cross-compatibility of macadamia cultivars. Journal of Horticultural Science 65(2), 205-213.
| Crossref | Google Scholar |
Selosse M-A, Rousset F (2011) The plant-fungal marketplace. Science 333(6044), 828-829.
| Crossref | Google Scholar | PubMed |
Shabalala M, Toucher M, Clulow A (2022) The Macadamia bloom–What are the hydrological implications? Scientia Horticulturae 292, 110628.
| Crossref | Google Scholar |
Shuai X, Dai T, Chen M, Liu C-M, Ruan R, Liu Y, Chen J (2022) Characterization of lipid compositions, minor components and antioxidant capacities in macadamia (Macadamia integrifolia) oil from four major areas in China. Food Bioscience 50, 102009.
| Crossref | Google Scholar |
Shuai X, Dai T, McClements DJ, Ruan R, Du L, Liu Y, Chen J (2023) Hypolipidemic effects of macadamia oil are related to AMPK activation and oxidative stress relief: in vitro and in vivo studies. Food Research International 168, 112772.
| Crossref | Google Scholar |
Skendžić S, Zovko M, Živković IP, Lešić V, Lemić D (2021) The impact of climate change on agricultural insect pests. Insects 12(5), 440.
| Crossref | Google Scholar |
Smit TG, Taylor NJ, Midgley SJE (2020) The seasonal regulation of gas exchange and water relations of field grown macadamia. Scientia Horticulturae 267, 109346.
| Crossref | Google Scholar |
Smith AK, Slippers B, Hurley BP, Fourie G (2022) Diversity of Lepidoptera associated with macadamia nut damage in South Africa and development of molecular tools to monitor pest populations. Agricultural and Forest Entomology 24(3), 332-343.
| Crossref | Google Scholar |
Solà Marsiñach M, Cuenca AP (2019) The impact of sea buckthorn oil fatty acids on human health. Lipids in Health and Disease 18(1), 145.
| Crossref | Google Scholar |
Spann TM, Beede RH, DeJong TM (2008) Seasonal carbohydrate storage and mobilization in bearing and non-bearing pistachio (Pistacia vera) trees. Tree Physiology 28(2), 207-213.
| Crossref | Google Scholar | PubMed |
Srichamnong W, Srzednicki G (2015) Internal discoloration of various varieties of Macadamia nuts as influenced by enzymatic browning and Maillard reaction. Scientia Horticulturae 192, 180-186.
| Crossref | Google Scholar |
Srichamnong W, Price B, Gardner T, Dean R, Plougonven E, Leonard A, Srzednicki G (2013) Studies of microstructure of kernels of Macadamia integrifolia and its hybrids through MRI, X-ray tomography and confocal microscopy. Journal of Food Science and Engineering 3(9), 503-516.
| Google Scholar |
Stephenson RA, Gallagher EC (1986a) Effects of temperature on premature nut drop in macadamia. Queensland Journal of Agricultural and Animal Sciences 43(2), 97-100.
| Google Scholar |
Stephenson RA, Gallagher EC (1986b) Effects of temperature during latter stages of nut development on growth and quality of macadamia nuts. Scientia Horticulturae 30, 219-225.
| Crossref | Google Scholar |
Stephenson RA, Cull BW, Mayer DG, Price G, Stock J (1986) Seasonal patterns of macadamia leaf nutrient levels in south east Queensland. Scientia Horticulturae 30(1-2), 63-71.
| Crossref | Google Scholar |
Stephenson RA, Gallagher EC, Rasmussen TS (1989a) Effects of growth manipulation on carbohydrate reserves of macadamia trees. Scientia Horticulturae 40(3), 227-235.
| Crossref | Google Scholar |
Stephenson RA, Ko HL, Gallagher EC (1989b) Plant-water relations of stressed, non-bearing macadamia trees. Scientia Horticulturae 39(1), 41-53.
| Crossref | Google Scholar |
Stephenson RA, Gallagher EC, Gogel BJ (2002) Macadamia nut size and maturity influenced by lime and nitrogen applications. Australian Journal of Agricultural Research 53(6), 677-680.
| Crossref | Google Scholar |
Stephenson RA, Gallagher EC, Doogan VJ (2003) Macadamia responses to mild water stress at different phenological stages. Australian Journal of Agricultural Research 54(1), 67-75.
| Crossref | Google Scholar |
Trochoulias T, Johns GG (1992) Poor response of macadamia (Macadamia integrifolia Maiden and Betche) to irrigation in a high rainfall area of subtropical Australia. Australian Journal of Experimental Agriculture 32(4), 507-512.
| Crossref | Google Scholar |
Trochoulias T, Lahav E (1983) The effect of temperature on growth and dry-matter production of macadamia. Scientia Horticulturae 19, 167-176.
| Crossref | Google Scholar |
Trueman SJ (2013) The reproductive biology of macadamia. Scientia Horticulturae 150, 354-359.
| Crossref | Google Scholar |
Trueman SJ, Turnbull CGN (1994) Effects of cross-pollination and flower removal on fruit set in Macadamia. Annals of Botany 73(1), 23-32.
| Crossref | Google Scholar |
Trueman SJ, Richards S, McConchie CA, Turnbull CGN (2000) Relationships between kernel oil content, fruit removal force and abscission in Macadamia. Australian Journal of Experimental Agriculture 40(6), 859-866.
| Crossref | Google Scholar |
Trueman SJ, Kämper W, Nichols J, Ogbourne SM, Hawkes D, Peters T, Hosseini Bai S, Wallace HM (2022a) Pollen limitation and xenia effects in a cultivated mass-flowering tree, Macadamia integrifolia (Proteaceae). Annals of Botany 129(2), 135-146.
| Crossref | Google Scholar | PubMed |
Trueman S, De Silva A, Kämper W, Nichols J, Hosseini Bai S, Wallace H, Royle J, Peters T, Ogbourne S (2022b) Pollen parentage of nuts during premature nut drop: do self-pollinated nuts drop and cross-pollinated nuts remain. Australian Macadamia Society News Bulletin 50, 19-20.
| Google Scholar |
Trueman SJ, Penter MG, Malagodi-Braga KS, Nichols J, De Silva AL, Ramos ATM, Moriya LM, Ogbourne SM, Hawkes D, Peters T, Kasinadhuni N, Hosseini Bai S, Wallace HM, Kämper W (2024) High outcrossing levels among global macadamia cultivars: implications for nut quality, orchard designs and pollinator management. Horticulturae 10(3), 203.
| Crossref | Google Scholar |
Tu X-H, Wu B-F, Xie Y, Xu S-L, Wu Z-Y, Lv X, Wei F, Du L-Q, Chen H (2021) A comprehensive study of raw and roasted macadamia nuts: lipid profile, physicochemical, nutritional, and sensory properties. Food Science & Nutrition 9(3), 1688-1697.
| Crossref | Google Scholar | PubMed |
Vanalli C, Casagrandi R, Gatto M, Bevacqua D (2021) Shifts in the thermal niche of fruit trees under climate change: the case of peach cultivation in France. Agricultural and Forest Meteorology 300, 108327.
| Crossref | Google Scholar |
Van Etten ML (2022) Quality matters. A commentary on: pollen limitation and xenia effects in a cultivated mass-flowering tree, Macadamia integrifolia (Proteaceae). Annals of Botany 129(2), iii-iv.
| Crossref | Google Scholar | PubMed |
Van Zwieten L, Kingston T, Cox J, Ayres M, Walker B, Hotson I, Morris S (2003) Healthy soils in macadamia orchards. Australian Nutgrower 17(2), 18-24.
| Google Scholar |
Wakeling LT, Mason RL, D’arcy BR, Caffin NA (2002) Opalescence in Australian-grown pecan kernels: occurrence and causes. Journal of Food Science 67(8), 2873-2880.
| Crossref | Google Scholar |
Wall MM (2010) Functional lipid characteristics, oxidative stability, and antioxidant activity of macadamia nut (Macadamia integrifolia) cultivars. Food Chemistry 121(4), 1103-1108.
| Crossref | Google Scholar |
Wall MM, Gentry TS (2007) Carbohydrate composition and color development during drying and roasting of macadamia nuts (Macadamia integrifolia). LWT - Food Science and Technology 40(4), 587-593.
| Crossref | Google Scholar |
Wallace HM, Vithanage V, Exley EM (1996) The effect of supplementary pollination on nut set of macadamia. Annals of Botany 78, 765-773.
| Crossref | Google Scholar |
Walton DA, Wallace HM (2009) Delayed harvest reduces quality of raw and roasted macadamia kernels. Journal of the Science of Food and Agriculture 89(2), 221-226.
| Crossref | Google Scholar |
Walton DA, Wallace HM (2010) Dropping macadamia nuts-in-shell reduces kernel roasting quality. Journal of the Science of Food and Agriculture 90(13), 2163-2167.
| Crossref | Google Scholar | PubMed |
Walton DA, Randall BW, Le Lagadec MD, Wallace HM (2013) Maintaining high moisture content of macadamia nuts-in-shell during storage induces brown centres in raw kernels. Journal of the Science of Food and Agriculture 93(12), 2953-2958.
| Crossref | Google Scholar | PubMed |
Wilkie JD, Sedgley M, Olesen T (2009) A model of vegetative flush development and its potential use managing macadamia (Macadamia integrifolia) tree canopies. Crop & Pasture Science 60(5), 420-426.
| Crossref | Google Scholar |
Zhao X, Dong Q, Ni S, He X, Yue H, Tao L, Nie Y, Tang C, Zhang F, Shen J (2019) Rhizosphere processes and nutrient management for improving nutrient-use efficiency in macadamia production. HortScience 54(4), 603-608.
| Crossref | Google Scholar |
Zhao X, Lyu Y, Jin K, Lambers H, Shen J (2021) Leaf phosphorus concentration regulates the development of cluster roots and exudation of carboxylates in Macadamia integrifolia. Frontiers in Plant Science 11, 610591.
| Crossref | Google Scholar |
Zhao X, Lyu Y, Dong Q, He X, Yue H, Yang L, Tao L, Gong L, Zheng H, Wen S, Lambers H, Shen J, Cavagnaro T (2023) Biomass partitioning and ionomics of Macadamia with high manganese and low phosphorus concentrations. Functional Plant Biology 50(7), 559-570.
| Crossref | Google Scholar | PubMed |
Zuza EJ, Maseyk K, Bhagwat SA, Chemura A, Brandenburg RL, Emmott A, Rawes W, Hancock W, Mnthambala F, Araya YN (2023) Factors affecting soil quality among smallholder macadamia farms in Malawi. Agriculture & Food Security 12(1), 17.
| Crossref | Google Scholar |
Zvereva EL, Kozlov MV (2006) Consequences of simultaneous elevation of carbon dioxide and temperature for plant–herbivore interactions: a metaanalysis. Global Change Biology 12(1), 27-41.
| Crossref | Google Scholar |