Molecular cloning and characterisation of root-specific SlREO promoter of the Indian tomato (Solanum lycopersicum L.) cultivar
Penki Jenny A , Amar A. Sakure A , Ankit Yadav A and Sushil Kumar
A
Abstract
Genetic transformation is helpful in enhancing crops, utilising promoters that can be constitutive, inducible, or tissue-specific. However, the use of constitutive promoters may hinder plant growth due to energy consumption during cellular processes. To optimise transgene effects, tissue-specific promoters like root-specific ones prove valuable in addressing root-related issues and enhancing productivity. Yet, identified root-specific promoters in crop are limited. To address this gap, the expression pattern of the root-specific SlREO promoter was examined across various crops. Sequencing confirmed its identity and high homology (99%) with the NCBI database, distinct from other plants tested. Using the PLACE database, six motifs associated with root expression were identified, along with several other important elements. The 2.4 kb SlREO promoter was linked to a ß-glucuronidase (GUS) reporter gene alongside the CaMV35S promoter in pRI 201-AN-GUS vectors to study its expression. Histochemistry revealed strong root-specific expression in tomato (Solanum lycopersicum) root tissues and limited expression in stems. However, the SlREO promoter did not consistently maintain its root-specific expression in other plants. Conversely, the CaMV35S promoter exhibited constitutive expression across all tissues in various plants. This study underscores the potential of the SlREO promoter as a root-specific regulatory element, offering avenues for improving crops, particularly against environmental stresses.
Keywords: Agrobacterium, CaMV35S promoter, cloning, constitutive promoters, GUS, root specific, SlREO promoter.
Introduction
By 2050, the global population is projected to reach 9 billion, with climate change exacerbating abiotic stresses such as high temperatures and drought, leading to substantial yield reductions in crop plants (Kumar et al. 2022). To meet the needs of such a vast population and to overcome the effects of a harsher climate, scientists must increase food production at the same rate by manipulating the genomic makeup of crop species. Generally, changes in the genetic makeup of a crop occur through conventional or molecular breeding to increase food production, but this is a labour-intensive and time-consuming process. Moreover, breeding will be futile where variability in the gene pool is very limited and hybridisation is not fruitful. In such cases, to improve production and productivity, genetic engineering can be exploited to produce transgenic plants with improved resistance to diseases and insects, fruits and seeds with enhanced nutritional qualities, shelf life and higher yield that are also tolerant to adverse environmental conditions or abiotic stresses (Jewell et al. 2010). Historically, transgenic plants were first reported in tobacco (Nicotiana tabacum) in 1983 (Jhansi Rani and Usha 2013). Transgenics are also used for studying the expression and function of genes. The success of transgenic plants depends on the expression of foreign genes in the host cell. The expression of any gene in the recipient host depends on promoter activity. Therefore, the identification, isolation and characterisation of novel promoters are necessary for successful and viable plant genetic engineering.
Promoters are specific DNA sequences located upstream of the gene’s coding region, initiating transcription. However, the success of gene transfer technologies largely depends on the type of promoter selected during the experiment (Potenza et al. 2004). There are different kinds of promoters, such as constitutive, inducible, cell- or tissue-specific promoters. Constitutive promoters direct gene expression in many plants, and inducible promoters expressed only in the presence of certain factors or cues may be physical or chemical and often directly expressed in certain tissues (Tyagi 2001); however, tissue-specific promoters direct gene expression only in a particular tissue of the plant so that transgenes can be expressed in their respective tissues wherever they are effective, which also minimises the cost of transgenics. By using different tissue-specific promoters, gene expression is controlled both spatially and temporally (Xiao et al. 2005; Saranya and Kanchana 2016).
Roots are the most important plant organs and are vulnerable to various stresses, such as osmotic and ionic stresses. In response to these stresses, root tissues undergo alterations in gene expression via physical and metabolic changes. The root-specific promoter is a type of tissue-specific promoter that has been used for developing resistance to root pathogens and tolerance to environmental stresses (e.g. drought, salinity, heavy metals), capturing micro- and macronutrients; and improving beneficial plant‒microbe interactions (Yoshimoto et al. 2002; Grunennvaldt et al. 2015). Hence, it is necessary to control transgene expression only in roots for the identification or manipulation of root-specific functions. However, constitutive promoters compete for energy that are mainly required for growth and development. By addressing such root-related issues, improved crops with sustainable and significant yields can be obtained.
To date, many root-specific promoters have been identified, but only a few root promoters have retained their root-specific expression, such as the phosphate transporter genes PHT1 promoter from Arabidopsis thaliana, which shows root-specific expression in rice (Oryza sativa) and is localised to the root hairs and outer layers of the main roots (Koyama et al. 2005). The root specific promoter AtNRT2.1 (nitrate transporter 2) derived from A. thaliana was shown root-specific expression in tomato (Solanum lycopersicum) (Kong et al. 2014). The extensor gene promoter has been shown to exhibit root-specific expression in tomato (Showalter et al. 1992) and tobacco (Zhang et al. 2016). The ToRB7 root-specific promoter was isolated from tobacco and is localised to central cylinder regions and the root meristem (Conkling et al. 1990). The LAC1 gene promoter is expressed in the root apex as a result of salt stress in maize (Zea mays) (Liang et al. 2006). The PsPR10 gene promoter has shown more root-specific expression under abiotic stress in tobacco in lateral roots (Xu et al. 2010). The ribonuclease LX gene promoter has shown activity in root tips of tomato as a result of phosphate starvation (Köck et al. 2006). However, the deployment of these promoters is limited because they are strongly induced by certain nutrients, their localisation to the outermost layers of roots, environmental conditions or their expression only in some specific plants. One of the root-specific promoters is SlREO.
The SlREO promoter was isolated from tomato, which is a highly expressible root-specific promoter in this species. The size of this promoter is 2.4 kb. This gene is a member of the superfamily of Fe(II)-dependent dioxygenases and 2-oxoglutarate. This promoter has a very good ability to express a specific gene in tomato root tissue, especially in the root cortex, and has very low expression in aerial plant organs (Jones et al. 2008). Dehydration and wounding stress had little effect on promoter activity, whereas exposure to NaCl, salicylic acid and jasmonic acid had a slight suppressive effect, and exposure to gibberellic acid and abscisic acid had a strong effect on promoter activity.
For the functional analysis of genes, stable transformation is the most important, but it is a lengthy process. Therefore, transient transient assays mediated by Agrobacterium-mediated, was used as alternatives for identifying the function of genes, protein production and promoter analysis. Due to its preference for integration into actively transcribed areas, high expression of introduced genes and low copy number, Agrobacterium-mediated transformation outperforms all other transformation techniques in terms of effectiveness, convenience, and cost. Precise gene fusions or reporter assays are commonly used to evaluate transient and stable transformation in plants to simplify analysis. There are many reporter genes in which the GUS reporter gene system (Jefferson et al. 1987) has been extensively applied in plant gene expression studies because of its high sensitivity and enzyme stability, and it can be detected by fluorometric, histochemical and spectrophotometric techniques (Fior and Gerola 2009). Therefore, the uidA (encoding the enzyme β-glucuronidase GUS) GUS reporter gene was placed under the control of the SlREO promoter sequence to determine the expression pattern of the promoter via histochemical techniques.
The main aim of this study was to determine the root-specific expression of the SlREO promoter via histochemical analysis of the GUS reporter gene in major plants of different families, such as Solanaceae (tomato, tobacco), Poaceae (rice, maize), Euphorbiaceae (castor, Ricinus communis) and Malvaceae (cotton, Gossypium hirsutum).
Materials and methods
Collection of experimental material and growth conditions
For this study, the donor organism tomato (Solanum lycopersicum L.) variety GAT-5 was collected from a distant hybridisation farm at the Department of Agricultural Biotechnology, Anand Agricultural University, Anand, India. The seeds of the recipient crops (tomato; tobacco, Nicotiana tabacum L.; rice, Oryza sativa L.; maize, Zea mays L.; cotton, Gossypium hirsutum L.; and castor, Ricinus communis L.) were procured from the respective research stations of Anand Agricultural University, Anand, India. Seeds of the respective plants were germinated and grown in vitro by placing them on ½ strength MS media (Murashige and Skoog 1962) salts and vitamins at pH 5.8 in a growth room at 25°C with 16 h light and 8 h dark periods and 70% humidity.
Isolation of the promoter sequence
After the isolation of genomic DNA from the tomato leaves using cetyl-trimethyl ammonium bromide (CTAB) buffer using a modified method described by Doyle and Doyle (1990), full-length promoter sequence of 2.4 kb was isolated using promoter-specific infusion primers containing an XbaI restriction site (forward primer) and a PstI site adjacent to the site of translation initiation (reverse primer, Table 1), and both primers also had 15 bp homologous sequences similar to the vector synthesised using Snapgene software (http://www.snapgene.com) by retrieving the SlREO promoter sequence from the NCBI database deposited by Jones et al. (2008) with the gene IDEU59149. The PCR cocktail containing high fiedelity 2× CloneAmp PCR master mix (Takara, Japan) was used for amplification of SlREO promoter following the manufacturer’s protocol. The PCR reaction was carried out in a 200-μL tube with an initial denuration at 95°C for 5 min, and 35 cycles of denaturation at 95°C for 35 s, annealing at 58°C for 30 s, extension at 72°C for 1 min and final extension at 72°C for 10 min followed by 4°C.
Primers | Sequences (5′−3′) A | Temp. (°C) | Product size (bp) | |
---|---|---|---|---|
Promoter specific amplification | ||||
SlREO – I F (PstI) | CCAAGCTTGCATGCCTGCAGCACAAGGCAACGGATGG | 66.81 | ~2.4 kb | |
SlREO – I R (XbaI) | TTGTGATGTATCTAGAAATTAATGGGTTTTTACTTTGAACCTTAAAG | 76.11 | ||
Colony PCR | ||||
GUS F | GATCGCGAAAACTGTGGAAT | 56 | ~800 bp | |
GUS R | TGAGCGTCGCAGAACATTAC |
Construction of promoter-uidA constructs by infusion cloning
The binary vector pRI 201-AN-GUS containing the CaMV35S promoter, which controls the expression of the uidA gene, and the nptII selectable marker were used as reference vectors for cloning. The amplified SlREO promoter sequence was flanked by 15 bp homologous sequences, allowing the promoter sequence to be conveniently exchanged in place of the CaMV35S promoter (infusion cloning). The pRI 201-AN-GUS vector containing the CaMV35S promoter upstream of GUS was used as a positive control (35S-GUS). After infusion cloning, the pRI 201-AN-GUS vector was recognised as pRI201-SlREO-GUS. Therefore, the vectors containing both the SlREO and CaMV35S constructs (Fig. 1) were independently transformed into Escherichia coli DH5α cells via the heat shock method to increase the number of copies of the constructs. The colonies tested for positive by colony PCR were used for plasmid isolation by culturing overnight at 28°C with rotation at 250 rpm in 50 mL of LB media supplemented with 50 mg/L kanamycin and were screened by restriction enzyme digestion of both constructs. A BigDye Terminator ver. 3.1 Cycle Sequencing Kit was used to sequence the SlREO construct, followed by sequence processing in the ABI PRISM 3500 Genetic Analyser Module, Basecaller, Dye set/Primer and Matrix files to confirm the presence, orientation and integrity of the respective promoter fragment.
In silico analysis
The sequences obtained after processing were subjected to the online BLAST tool of the NCBI gene bank and the PLACE database (http://www.dna.affrc.go.jp/htdocs/PLACE/) for understanding homology among and among different species and key characteristics of the promoter, such as GATA, CAAT, TATA boxes and root-specific motifs.
Agrobacterium-mediated transformation of various plant species
The promoter-uidA constructs, i.e. the SlREO and CaMV35S constructs, were introduced into Agrobacterium tumefaciens strain EHA 105 through electroporation (TAKARA cat: 1652089) for transformation into the tissues of the different plants used in this study. Single colonies were identified and tested for both the SlREO and CaMV35S promoters by colony PCR using vector-specific (GUS) primers (Table 1). The positive colonies were cultured overnight at 28°C with rotation at 250 rpm in 50 mL of Luria broth (LB) media supplemented with 50 mg/L streptomycin, 50 mg/L kanamycin and 50 mg/L rifampicin. Each culture was used for plasmid isolation, and the plasmids were subsequently screened by restriction enzyme digestion for the presence of both promoters.
Overnight cultivation of Agrobacterium cultures was carried out at 27°C in yeast extract broth (YEB) liquid media, and the pH was maintained at 7.0. This growth medium was supplemented with 50 mg/L kanamycin and 100 mg/L streptomycin. Bacterial growth to the log phase was achieved with an optical density at 600 nm (A600) ranging from 0.4 to 0.6. Subsequently, the bacterial cells were harvested through centrifugation at 1500g for 2 min and subsequently reconstituted in fresh MS liquid supplemented with acetosyringone (200 μM) to achieve a final OD of 0.5 at A600 (Clough and Bent 1998).
Different explants (roots, stems and leaves) of 1 cm in size were prepared from 14 days-old seedlings raised in vitro and were co-cultivated with Agrobacterium solution for 20 min, followed by blotting dry on sterile Whatman filter paper to remove excess Agrobacterium cells. Further, tissues were cocultivated on cocultivation media (CCM) for 3 days in the dark at 24°C; subsequently, these tissues were subjected to histochemical GUS stain as described by Jefferson et al. (1987).
Histochemical localisation of GUS
Histochemical GUS staining was carried out on the transformed tissues of different plants by incubating the tissues in 10 mL of GUS buffer consisting of 1 mL of sodium phosphate buffer (50 mM, pH 7.0), 20 μL of Na-EDTA (0.5 M, pH 8.0), 1 mL of both 50 mM potassium ferricyanide and potassium ferrocyanide, 500 μL of X-Gluc (25 mg/mL) and 1 mL of Triton X-100 (10% (v/v)) at 37°C for 12–24 h. After staining, the tissues were destained with 70% ethanol several times. GUS expression was detected by the blue colour, which results from the enzymatic cleavage of X-GLUC, and photos at 4× resolution were captured by Stemi 2000-C Stereo Microscope (Zeiss, Switzerland).
Statistical analysis
The GUS assay was performed in accordance with a completely randomised design with five repetitions using MS-DOS ver. 5.1.2600. In each repetition, 20 leaf, stem and root tissues were collected in 5-mL microtubes for the control and transformed tissues of both CaMV35S and SlREO plants. The data were collected on a visual basis for the number of tissues showing GUS activity (blue stain), and the mean data were used for statistical analysis as described by Snedecor and Cochran (1967).
Results and discussion
Amplification and cloning of the SlREO promoter
For amplification of the SlREO promoter from the DNA of the tomato variety GAT-5, promoter-specific infusion primers were designed from published sequences to specifically amplify the promoter from the host plant DNA. An expected SlREO promoter size of 2.4 kb was obtained (Fig. 2). The amplified promoter fragment was connected to the plant expression vector (pRI 201-AN-GUS) by the infusion cloning method. The amplified PCR product was cloned and inserted into the pRI 201-AN-GUS vector via an in-fusion cloning reaction. After transformation into stable competent cells, approximately 37 colonies were obtained. Sixteen colonies were randomly selected for PCR to confirm that the clones were correct. All 16 colonies were positive according to the 2.4 kb PCR amplicon. Restriction digestion was performed to investigate the orientation of SlREO in the cloned plasmid. Two bands of 11.4 kb and 2.4 kb were observed after restriction digestion, suggesting that the promoter was successfully cloned (Fig. 3). The cloned plasmid was further confirmed by Sanger DNA sequencing using vector-specific primers.
Bioinformatics analysis of the SlREO promoter sequence
The 2.4 kb SlREO promoter sequence was subsequently amplified, cloned and sequenced, which displayed a high nucleotide similarity of 99.34% with the SlREO sequence (EU591493.1) previously submitted by Jones et al. (2008) in S. lycopersicum cv. I-3 on chromosome 1 (CP023757.1). Additionally, BLAST searches revealed that the SlREO promoter exhibited maximum similarity with that of Solanum tuberosum (potato), while no similarity was found with those of the cotton, castor, rice, maize and tobacco genome databases.
To determine the presence and location of cis-acting regulatory elements in the SlREO promoter, the PLACE database was utilised (Higo et al. 1999). The PLACE database, which has also been referenced by Kumar et al. (2016), Xiao et al. (2006), Chen et al. (2015) and Koehorst-van Putten et al. (2012), provides information on these regulatory elements and serves as a valuable resource for studying the transcriptional regulation of genes.
Based on the PLACE database results, the SlREO promoter sequence contained 39 TATA boxes, 20 GATA boxes and 45 CAAT boxes. Additionally, six motifs associated with root expression were found: (1) OSE1; (2) OSE2ROOTNODULE; (3) ROOTMOTIFTAPOX1; (4) RAV1AAT; (5) P1BS; and (6) ASF1MOTIFCAMV (Fig. 4). The number of repetitions of these root-specific motifs in the SlREO promoter sequence is in Table 2. These findings suggest that the promoter possesses the necessary elements for root-specific expression of transgenes.
Cis-Acting regulatory elements in the SlREO promoter. Red boxes, CAAT box motif; yellow boxes, TATA box motif; green boxes, GATA box motif; pink boxes, OSE2ROOTNODULE root motif; sky blue boxes, OSE1ROOTNODULE root motif; dark green boxes, ROOTMOTIFTAPOX1 root motif; grey boxes, RAV1AAT root motif; grey/green boxes, ASF1MOTIFCAMV root motif; teal boxes, P1BS root motif.
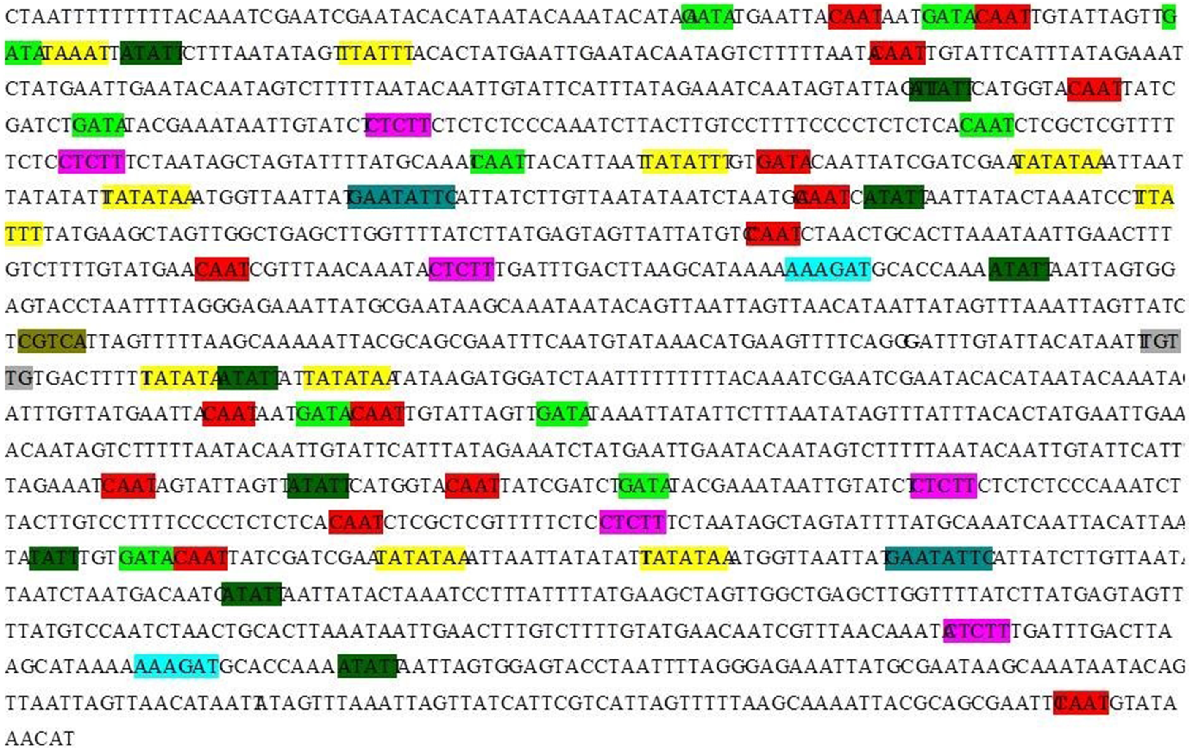
Cis-element | Sequence | Strand and location | Description | |
---|---|---|---|---|
OSE1ROOTNO DULE | AAAGAT | +674, +1592 | One of the organ-specific elements (OSE) consensus sequence motifs characteristic of the promoters activated in infected cells of root nodules (Fehlberg et al. 2005). | |
OSE2ROOTNODULE | CTCTT | +292, +356, +648, +1210, +1274, +1566 | One of the organ-specific elements (OSE) consensus sequence motifs characteristic of the promoters activated in infected cells of root nodules (Fehlberg et al. 2005). | |
ROOTMOTIFTAPOX1 | ATATT | +97, −106, +245, +401, −426, +444, +507, −469, +470, −487, −687, +688, −890, +891, −903, +1015, −1024, +1163, −1344, +1362, +1387, +1388, −1405, +1425, −1605, +1606 | Motif was found both in promoters of rolD and had a distinctive pattern of expression in roots (Elmayan and Tepfer 1995). | |
RAV1AAT | CAACA | −872 | Motif found in Arabidopsis and shown relatively high expression in rosette leaves and roots (Kagaya et al. 1999). | |
ASF1MOTIFCAMV | TGACG | −790 | Motif was found in CaMV35S promoter and are involved in transcriptional activation of several genes by auxin and/or salicylic acid (Krawczyk et al. 2002). | |
PIBS | GNATATNC | −468, +468, −1386, +1386 | The motif was found in upstream regions of phosphate-starvation responsive genes in various plant species, with the highest expression in trichoblast (root hair) cells (Schünmann et al. 2004). |
−, coding strands; +, non-coding strands.
Similar studies have been conducted by Xiao et al. (2005), Chen et al. (2015), Zhang et al. (2016) and Mohan et al. (2017) in other plant systems. Xiao et al. (2005) identified the root-specific motif ROOTMOTIFTAPOX1 (ATATT) in the promoters of MtPT1 and MtPT2 in Medicago truncatula Using the PLACE and PlantCARE databases, Chen et al. (2015) identified the root-specific motifs ROOTMOTIFTAPOX1 (ATATT) and OSE2ROOTNODULE (CTCTT), as well as ABA-responsive and cytokinin-responsive elements in the GmTIP root-specific promoter in soybean (Glycine max). Zhang et al. (2016) identified four root-specific motifs in the root-specific promoter (NtREL1) of tobacco using the PLACE database. Using the PLACE database, Mohan et al. (2017) identified the root-specific motifs ROOTMOTIFTAPOX1 (ATATT) and LEAFYATAG (CCAATGT) in the synthetic root-specific module (SynR2) promoter in transgenic tobacco plants.
The SlREO promoter sequence also contains the auxin-responsive element (D2GMAUX28) motif ATTTATATAAA (Nagao et al. 1993) and the ABA-responsive element (MYB1AT, MYB2CONSENSUSAT) motifs WAACCA and YAACKG, respectively (Abe et al. 2003), whereas the TGAC motif (WRKY71OS) functions as a transcriptional activator in abscisic acid signalling by transcriptional repression of the gibberellin signalling pathway (Eulgem et al. 1999), the cytokinin responsive elements (ARR1AT and CPBCSPOR) motifs NGATT and TATTAG (Ross et al. 2004; Fusada et al. 2005), and the gibberellin responsive element (CARGNCAT) motif CC[A/T]8GG (Wang et al. 2004).
Histochemical GUS activity of the SlREO and CaMV35S promoters
After confirmation of the plasmids isolated from the Agrobacterium by restriction enzyme digestion (Fig. 3), the cultures that were positive for transforming A. tumefaciens containing both the SlREO and CaMV35S constructs were separately transformed into plant tissues (roots, stems and leaves) of different plants. These transformed tissues were subsequently utilised for histochemical GUS staining analysis. The GUS gene driven by the constitutively active promoter was positive.
In the case of SlREO-transformed tomato tissues, GUS expression analysis revealed significant differences between the roots (93.89%) and stem tissues (69.68%). However, little to no significant GUS expression was detected in the leaf tissues, indicating that the SlREO promoter exhibited stronger expression in the roots than in the stems and leaves. Notably, the optical density of the Agrobacterium was maintained at 1.0 at 600 nm to ensure consistent results. Similarly, Jones et al. (2008) reported GUS expression patterns similar to those of SlREO in roots and stems, but they observed little expression in leaf tissues. In contrast, in other plant species, such as tobacco, rice, maize, cotton and castor, the SlREO promoter did not exhibit root-specific expression in any of these ’plant tissues. In contrast, the SlREO promoter was expressed in cotton leaves (Fig. 5, Table 3).
Histochemical staining for GUS activity in different tissues of different plants under a trilocular stereozoom microscope (4×). (a) Tomato, (b) tobacco, (c) rice, (d) maize, (e) cotton, and (f) castor.
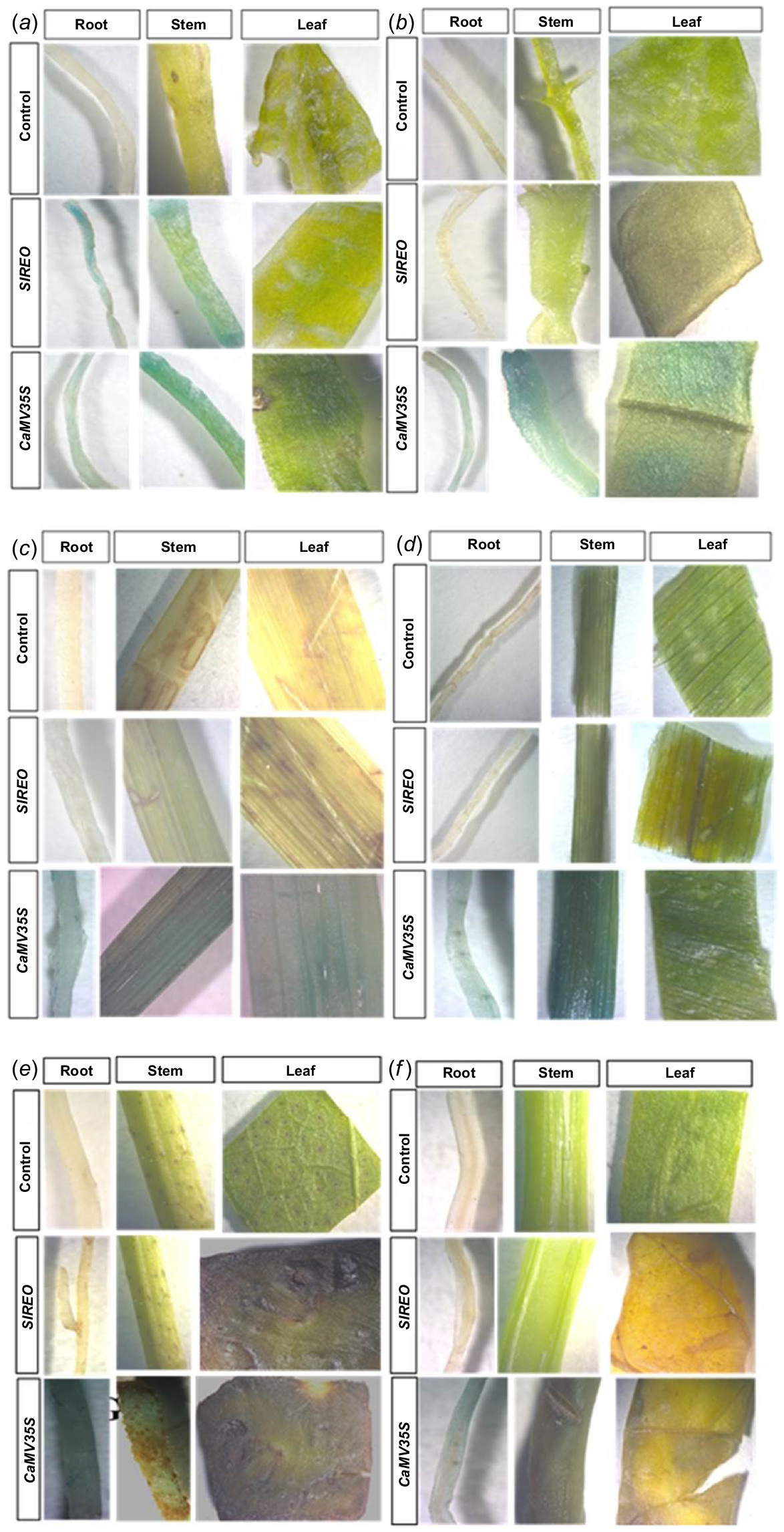
Crop | Tissue | Explants transformed | Explant GUS positive | Treatment efficiency (%) | ±s.e.m. | CD (P < 0.05) | CV (%) | |||||
---|---|---|---|---|---|---|---|---|---|---|---|---|
T1 | T2 | T3 | ||||||||||
SlREO | CaMV35S | SlREO | CaMV35S | Positive control (CaMV35S) | Negative control | SlREO | ||||||
Count | Mean | |||||||||||
Tomato | Root | 19.6 | 20 | 18.4 | 14.4 | 72.00** | 0 | 93.89** | 0.905 | 2.789 | 3.66 | |
Stem | 19.8 | 20 | 13.8 | 20 | 100.00** | 0 | 69.68** | 0.93 | 2.86 | 3.68 | ||
Leaf | 20 | 20 | 0 | 20 | 100.00** | 0 | 0 | 0 | 0 | 0 | ||
Tobacco | Root | 20 | 20 | 0 | 15.6 | 78.00** | 0 | 0 | 0.7 | 2.18 | 6.08 | |
Stem | 20 | 20 | 0 | 16 | 79.98** | 0 | 0 | 0.18 | 0.56 | 1.54 | ||
Leaf | 20 | 20 | 0 | 20 | 100.00** | 0 | 0 | 0 | 0 | 0 | ||
Rice | Root | 20 | 19.8 | 0 | 13.6 | 68.68** | 0 | 0 | 0.56 | 5.47 | 1.72 | |
Stem | 20 | 20 | 0 | 14.2 | 71.00** | 0 | 0 | 0.57 | 5.45 | 1.78 | ||
Leaf | 20 | 20.4 | 0 | 17.6 | 88.00** | 0 | 0 | 0.7 | 5.39 | 2.18 | ||
Maize | Root | 20 | 20 | 0 | 12 | 60.00** | 0 | 0 | 0 | 3.88 | 0 | |
Stem | 20 | 20 | 0 | 19.2 | 96.00** | 0 | 0 | 0.57 | 4.03 | 1.78 | ||
Leaf | 20 | 19.4 | 0 | 16.6 | 83.00** | 0 | 0 | 0.7 | 5.71 | 2.18 | ||
Cotton | Root | 20 | 20 | 0 | 14.6 | 75.29** | 0 | 0 | 0.38 | 3.45 | 1.19 | |
Stem | 20 | 20 | 0 | 15.8 | 79.00** | 0 | 0 | 0.58 | 4.9 | 1.79 | ||
Leaf | 20 | 20 | 0 | 20 | 100.00** | 0 | 0 | 0.58 | 2.4 | 1.78 | ||
Castor | Root | 20 | 20 | 0 | 12 | 60.00** | 0 | 0 | 3.88 | 0 | 0 | |
Stem | 20 | 19.8 | 0 | 19.4 | 97.94** | 0 | 0 | 0.726 | 4.97 | 2.238 | ||
Leaf | 20 | 20 | 0 | 15.2 | 76.00** | 0 | 61.00** | 0.577 | 5.1 | 1.78 |
CD, critical difference; CV, coefficient of variation.
**P < 0.01.
The results of this study suggest that the root-specific expression of the SlREO promoter may not be guaranteed in heterologous hosts. The lack of GUS expression in heterologous hosts could be due to the absence of SlREO sequence similarity in those plants, indicating the potential absence of active transcription factors to stimulate the SlREO promoter sequence. Interestingly, significant expression of this gene was detected in cotton leaves, suggesting that the SlREO promoter may have lost its root specificity in cotton during evolution. Gittins et al. (2001), Chan et al. (2005) and Vaughan et al. (2006) reported similar observations regarding the lack of SlREO promoter expression in heterologous hosts and the loss of root specificity in certain plants.
In contrast, the CaMV35S promoter (positive control) was significantly more highly expressed in all tissues of the various plants than was the negative control. The CaMV35S promoter is known for its constitutive expression properties, as reported by Odell et al. (1985), Jefferson et al. (1987), Medberry et al. (1992) and Potenza et al. (2004). The results of the present study regarding the CaMV35S promoter indicated its ability to drive high levels of transgenic expression in both dicots and monocots, in line with previous studies by Battraw and Hall (1990) and Benfey and Chua (1990).
Conclusion
This study conclude that the SlREO promoter shows a root-specific nature exclusively in the homologous host tomato plant. Therefore, it can be used in transgenic tomato to express genes in overcoming specific root related stresses/issues in water and nutrient uptake, root growth, drought tolerance and resistance to multiple pathogens. This reduces the undesired impact of transgenes on edible plant parts and provides a selection tool for transgenic tomato breeding. Furthermore, the identification of root-specific promoters is necessary to enhance the expression of target genes in a wide range of plants.
Author contributions
SK and AAS conceptualised the experiment and interpreted the data; PJ performed the experiment and wrote the first draft of the paper; AY helped in cloning and data interpretation.
Acknowledgements
The authors thank Anand Agricultural University, Anand, Gujarat, India for providing the necessary facilities to conduct the experiment.
References
Abe H, Urao T, Ito T, Seki M, Shinozaki K, Yamaguchi-Shinozaki K (2003) Arabidopsis AtMYC2 (bHLH) and AtMYB2 (MYB) function as transcriptional activators in abscisic acid signaling. The Plant Cell 15(1), 63-78.
| Crossref | Google Scholar | PubMed |
Battraw MJ, Hall TC (1990) Histochemical analysis of CaMV35S promoter-β-glucuronidase gene expression in transgenic rice plants. Plant Molecular Biology 15, 527-538.
| Crossref | Google Scholar | PubMed |
Benfey PN, Chua N-H (1990) The cauliflower mosaic virus 35S promoter: combinatorial regulation of transcription in plants. Science 250(4983), 959-966.
| Crossref | Google Scholar | PubMed |
Chan Y-L, Prasad V, Sanjaya , Chen KH, Liu PC, Chan M-T, Cheng C-P (2005) Transgenic tomato plants expressing an Arabidopsis thionin (Thi2.1) driven by fruit-inactive promoter battle against phytopathogenic attack. Planta 221, 386-393.
| Crossref | Google Scholar | PubMed |
Chen L, Jiang B, Wu C, Sun S, Hou W, Han T (2015) The characterization of GmTIP, a root-specific gene from soybean, and the expression analysis of its promoter. Plant Cell, Tissue and Organ Culture (PCTOC) 121, 259-274.
| Crossref | Google Scholar |
Clough SJ, Bent AF (1998) Floral Dip: a simplified method for Agrobacterium-mediated transformation of Arabidopsis thaliana. The Plant Journal 16(6), 735-743.
| Crossref | Google Scholar | PubMed |
Conkling MA, Cheng C-L, Yamamoto YT, Goodman HM (1990) Isolation of transcriptionally regulated root-specific genes from tobacco. Plant Physiology 93(3), 1203-1211.
| Crossref | Google Scholar | PubMed |
Doyle JJ, Doyle JL (1990) Isolation of plant DNA from fresh tissue. Focus 12, 13-15.
| Google Scholar |
Elmayan T, Tepfer M (1995) Evaluation in tobacco of the organ specificity and strength of therolD promoter, domain A of the 35S promoter and the 35S2 promoter. Transgenic Research 4(6), 388-396.
| Crossref | Google Scholar | PubMed |
Eulgem T, Rushton PJ, Schmelzer E, Hahlbrock K, Somssich IE (1999) Early nuclear events in plant defence signalling: rapid gene activation by WRKY transcription factors. The EMBO Journal 18(17), 4689-4699.
| Crossref | Google Scholar | PubMed |
Fehlberg V, Vieweg MF, Dohmann EMN, Hohnjec N, Pühler A, Perlick AM, Küster H (2005) The promoter of the leghaemoglobin gene VfLb29: functional analysis and identification of modules necessary for its activation in the infected cells of root nodules and in the arbuscule-containing cells of mycorrhizal roots. Journal of Experimental Botany 56(413), 799-806.
| Crossref | Google Scholar | PubMed |
Fior S, Gerola PD (2009) Impact of ubiquitous inhibitors on the GUS gene reporter system: evidence from the model plants Arabidopsis, tobacco and rice and correction methods for quantitative assays of transgenic and endogenous GUS. Plant Methods 5(1), 19.
| Crossref | Google Scholar |
Fusada N, Masuda T, Kuroda H, Shimada H, Ohta H, Takamiya K-I (2005) Identification of a novel cis-element exhibiting cytokinin-dependent protein binding in vitro in the 5′-region of NADPH-protochlorophyllide oxidoreductase gene in cucumber. Plant Molecular Biology 59, 631-645.
| Crossref | Google Scholar | PubMed |
Gittins JR, Hiles ER, Pellny TK, Biricolti S, James DJ (2001) The Brassica napus extA promoter: a novel alternative promoter to CaMV 35S for directing transgene expression to young stem tissues and load bearing regions of transgenic apple trees (Malus pumila Mill.). Molecular Breeding 7, 51-62.
| Crossref | Google Scholar |
Grunennvaldt RL, Degenhardt GJ, Gerhardt IR, Quoirin M (2015) Promoters used in genetic transformation of plants. Research Journal of Biological Sciences 10(2), 1-9.
| Google Scholar |
Higo K, Ugawa Y, Iwamoto M, Korenaga T (1999) Plant cis-acting regulatory DNA elements (PLACE) database: 1999. Nucleic Acids Research 27(1), 297-300.
| Crossref | Google Scholar | PubMed |
Jefferson RA, Kavanagh TA, Bevan MW (1987) GUS fusions: beta-glucuronidase as a sensitive and versatile gene fusion marker in higher plants. The EMBO Journal 6(13), 3901-3907.
| Crossref | Google Scholar | PubMed |
Jhansi Rani S, Usha R (2013) Transgenic plants: types, benefits, public concerns and future. Journal of Pharmacy Research 6(8), 879-883.
| Crossref | Google Scholar |
Jones MO, Manning K, Andrews J, Wright C, Taylor IB, Thompson AJ (2008) The promoter from SlREO, a highly-expressed, root-specific Solanum lycopersicum gene, directs expression to cortex of mature roots. Functional Plant Biology 35(12), 1224-1233.
| Crossref | Google Scholar | PubMed |
Kagaya Y, Ohmiya K, Hattori T (1999) RAV1, a novel DNA-binding protein, binds to bipartite recognition sequence through two distinct DNA-binding domains uniquely found in higher plants. Nucleic Acids Research 27(2), 470-478.
| Crossref | Google Scholar | PubMed |
Koehorst-van Putten HJJ, Wolters A-MA, Pereira-Bertram IM, van den Berg HHJ, Van der krol AR, Visser RGF (2012) Cloning and characterization of a tuberous root-specific promoter from cassava (Manihot esculenta Crantz). Planta 236(6), 1955-1965.
| Crossref | Google Scholar | PubMed |
Kong K, Makabe S, Ntui VO, Khan RS, Nakamura I (2014) Synthetic chitinase gene driven by root-specific LjNRT2 and AtNRT2.1 promoters confers resistance to Fusarium oxysporum in transgenic tobacco and tomato. Plant Biotechnology Reports 8, 151-159.
| Crossref | Google Scholar |
Koyama T, Ono T, Shimizu M, Jinbo T, Mizuno R, Tomita K, Sakka K (2005) Promoter of Arabidopsis thaliana phosphate transporter gene drives root-specific expression of transgene in rice. Journal of Bioscience and Bioengineering 99(1), 38-42.
| Crossref | Google Scholar | PubMed |
Krawczyk S, Thurow C, Niggeweg R, Gatz C (2002) Analysis of the spacing between the two palindromes of activation sequence-1 with respect to binding to different TGA factors and transcriptional activation potential. Nucleic Acids Research 30(3), 775-781.
| Crossref | Google Scholar | PubMed |
Kumar A, Joshi I, Kohli D, Satheesh V, Abdin MZ, Sirohi A, Srinivasan R, Jain PK (2016) Characterization of root-knot nematode responsive and root-specific promoter containing PIN domain from Arabidopsis thaliana (L.) Heynh. Indian Journal of Genetics and Plant Breeding 76, 75-83.
| Crossref | Google Scholar |
Köck M, Stenzel I, Zimmer A (2006) Tissue-specific expression of tomato Ribonuclease LX during phosphate starvation-induced root growth. Journal of Experimental Botany 57(14), 3717-3726.
| Crossref | Google Scholar | PubMed |
Liang M, Haroldsen V, Cai X, Wu Y (2006) Expression of a putative laccase gene, ZmLAC1, in maize primary roots under stress. Plant, Cell & Environment 29(5), 746-753.
| Crossref | Google Scholar | PubMed |
Medberry SL, Lockhart BE, Olszewski NE (1992) The commelina yellow mottle virus promoter is a strong promoter in vascular and reproductive tissues. Plant Cell 4(2), 185-192.
| Crossref | Google Scholar | PubMed |
Mohan C, Jayanarayanan AN, Narayanan S (2017) Construction of a novel synthetic root-specific promoter and its characterization in transgenic tobacco plants. 3 Biotech 7, 234.
| Crossref | Google Scholar |
Murashige T, Skoog F (1962) A revised medium for rapid growth and bio assays with tobacco tissue cultures. Physiologia Plantarum 15(3), 473-497.
| Crossref | Google Scholar |
Nagao RT, Goekjian VH, Hong JC, Key JL (1993) Identification of protein-binding DNA sequences in an auxin-regulated gene of soybean. Plant Molecular Biology 21, 1147-1162.
| Crossref | Google Scholar | PubMed |
Odell JT, Nagy F, Chua N-H (1985) Identification of DNA sequences required for activity of the cauliflower mosaic virus 35S promoter. Nature 313(6005), 810-812.
| Crossref | Google Scholar | PubMed |
Potenza C, Aleman L, Sengupta-Gopalan C (2004) Targeting transgene expression in research, agricultural, and environmental applications: promoters used in plant transformation. In vitro Cellular & Developmental Biology - Plant 40(1), 1-22.
| Crossref | Google Scholar |
Ross EJH, Stone JM, Elowsky CG, Arredondo-Peter R, Klucas RV, Sarath G (2004) Activation of the Oryza sativa non-symbiotic haemoglobin-2 promoter by the cytokinin-regulated transcription factor, ARR1. Journal of Experimental Botany 55(403), 1721-1731.
| Crossref | Google Scholar | PubMed |
Saranya M, Kanchana M (2016) Promoter diversity in plants – a review. International Journal of Applied and Advanced Scientific Research 1(1), 209-217.
| Google Scholar |
Schünmann PH, Richardson AE, Vickers CE, Delhaize E (2004) Promoter analysis of the barley Pht1;1 phosphate transporter gene identifies regions controlling root expression and responsiveness to phosphate deprivation. Plant Physiology 136(4), 4205-4214.
| Crossref | Google Scholar |
Showalter AM, Butt AD, Kim S (1992) Molecular details of tomato extensin and glycine-rich protein gene expression. Plant Molecular Biology 19, 205-215.
| Crossref | Google Scholar | PubMed |
Tyagi AK (2001) Plant genes and their expression. Current Science 80(2), 161-169.
| Google Scholar |
Vaughan SP, James DJ, Lindsey K, Massiah AJ (2006) Characterization of FaRB7, a near root-specific gene from strawberry (Fragaria × ananassa Duch.) and promoter activity analysis in homologous and heterologous hosts. Journal of Experimental Botany 57(14), 3901-3910.
| Crossref | Google Scholar | PubMed |
Wang H, Caruso LV, Downie AB, Perry SE (2004) The embryo MADS domain protein AGAMOUS-Like 15 directly regulates expression of a gene encoding an enzyme involved in gibberellin metabolism. The Plant Cell 16(5), 1206-1219.
| Crossref | Google Scholar | PubMed |
Xiao K, Zhang C, Harrison M, Wang Z-Y (2005) Isolation and characterization of a novel plant promoter that directs strong constitutive expression of transgenes in plants. Molecular Breeding 15, 221-231.
| Crossref | Google Scholar |
Xiao K, Liu J, Dewbre G, Harrison M, Wang ZY (2006) Isolation and characterization of root-specific phosphate transporter promoters from Medicago truncatula. Plant Biology 8(4), 439-449.
| Crossref | Google Scholar | PubMed |
Xu X, Guo S, Chen K, Song H, Liu J, Guo L, Qian Q, Wang H (2010) A 796 bp PsPR10 gene promoter fragment increased root-specific expression of the GUS reporter gene under the abiotic stresses and signal molecules in tobacco. Biotechnology Letters 32, 1533-1539.
| Crossref | Google Scholar | PubMed |
Yoshimoto N, Takahashi H, Smith FW, Yamaya T, Saito K (2002) Two distinct high-affinity sulfate transporters with different inducibilities mediate uptake of sulfate in Arabidopsis roots. The Plant Journal 29(4), 465-473.
| Crossref | Google Scholar | PubMed |
Zhang C, Pan S, Chen H, Cai T, Zhuang C, Deng Y, Zhuang W (2016) Characterization of NtREL1, a novel root-specific gene from tobacco, and upstream promoter activity analysis in homologous and heterologous hosts. Plant Cell Reports 35(4), 757-769.
| Crossref | Google Scholar | PubMed |