A speculative ridge within the Kidson Sub-basin – integrated interpretation from geophysical data
Y. Zhan A *A
![]() Yijie (Alex) Zhan graduated from China University of Geoscience (Beijing) with a BSc in 2005. He has worked on structural interpretation, fracture detection and reservoir modelling in various basins. He has published in English and Chinese on seismic interpretation, airborne electromagnetic data, azimuthal anisotropy, forward modelling and characterisation of gas-bearing volcanoclastic and conventional reservoirs. He joined the Geological Survey of Western Australia’s Basin and Energy Geoscience group as Senior Geophysicist in 2011, and is a member of PESA. |
Abstract
This paper presents an analysis of the basement geometry and its implications on the Kidson Sub-basin in the southern Canning Basin. Integration of seismic data primarily near the rim of the sub-basin with airborne electromagnetic data across its central portion reveals an east-northeast oriented ridge at Permian level, suggesting a possible elevated structure in the Ordovician and basement. The proposed ridge effectively separates the Kidson Sub-basin into two distinct parts, providing an explanation for facies and thickness variations observed in the Nambeet and Goldwyer formations, as well as the absence of the Willara Formation in the southeastern portion of the sub-basin. The presence of shallowing trends observed in seismic profiles along the southern flank of the ridge suggests the proximity of the basement high. The ridge is speculative and requires further work to verify its existence, potentially including passive and reflection seismic surveys across the structure. If confirmed, it might be a significant feature with far-reaching implications for the prospectivity of resources in the Kidson Sub-basin. The hydrocarbon sourced from the Nambeet, Goldwyer and Bongabinni formations in the NNW depocentre may have migrated via the extension of the Parallel Range Fault and be trapped in the footwall block over the ridge. Compared to the structures in the Fitzroy Trough, the traps within the Kidson Sub-basin are expected to maintain reasonable integrity and have good potential for petroleum accumulation and carbon sequestration in the thick Paleozoic succession.
Keywords: AEM, airborne electromagnetic data, basement, Canning Basin, gravity, Kidson Sub-basin, prospectivity, seismic, structure.
Introduction
The Kidson Sub-basin is the largest tectonic component in the Canning Basin, encompassing an area of over 90,000 km2 with potential for even greater extent given its arbitrary boundaries (Fig. 1). However, despite its significant size, details of its geology have long remained enigmatic, hampered by limited coverage of seismic data and inherent complexities. In an effort to overcome these challenges, the Geological Survey of Western Australia (GSWA) undertook Falcon airborne gravity gradiometry surveys over the Kidson area and its northwestern extension during 2017–2018 (Fig. 2a) and conducted the Kidson Sub-basin seismic survey in collaboration with Geoscience Australia (GA) in 2018. This seismic survey extended west from 30 km west of the Kiwirrkurra community near the WA/NT border to near the Marble Bar township in the eastern Pilbara region. Finally, GA led a continental-scale airborne electromagnetic (AEM) data acquisition, the AusAEM 2019–2020 survey, which spanned from 25°S to 16°S covering the Kidson Sub-basin with east-oriented lines (Fig. 1b). Although significant progress has been made in terms of data collection, there remains a substantial area where seismic data are still either unavailable or of inadequate quality to illuminate the deep realms of the sub-basin.
Maps of the Kidson Sub-basin in the southern Canning Basin: (a) SEEBASE depth to basement (Frogtech Geoscience 2017), tectonic units (GSWA 2017) and seismic coverage; (b) bedrock geology (GSWA 2016) and AEM data, with sections from a to i shown in Fig. 5.
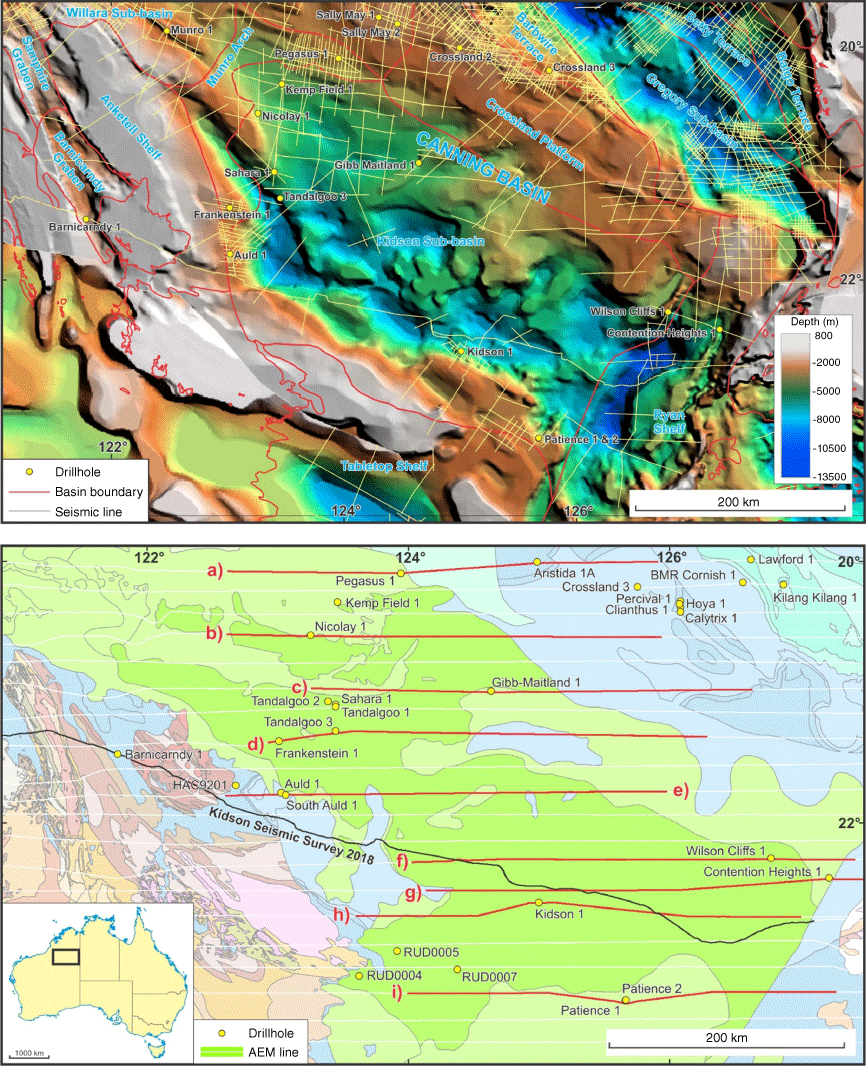
Gravity and aeromagnetic anomalies within the Kidson Sub-basin: (a) Bouguer gravity (GSWA 2020); (b) first vertical derivative of magnetic data (GSWA 2023).
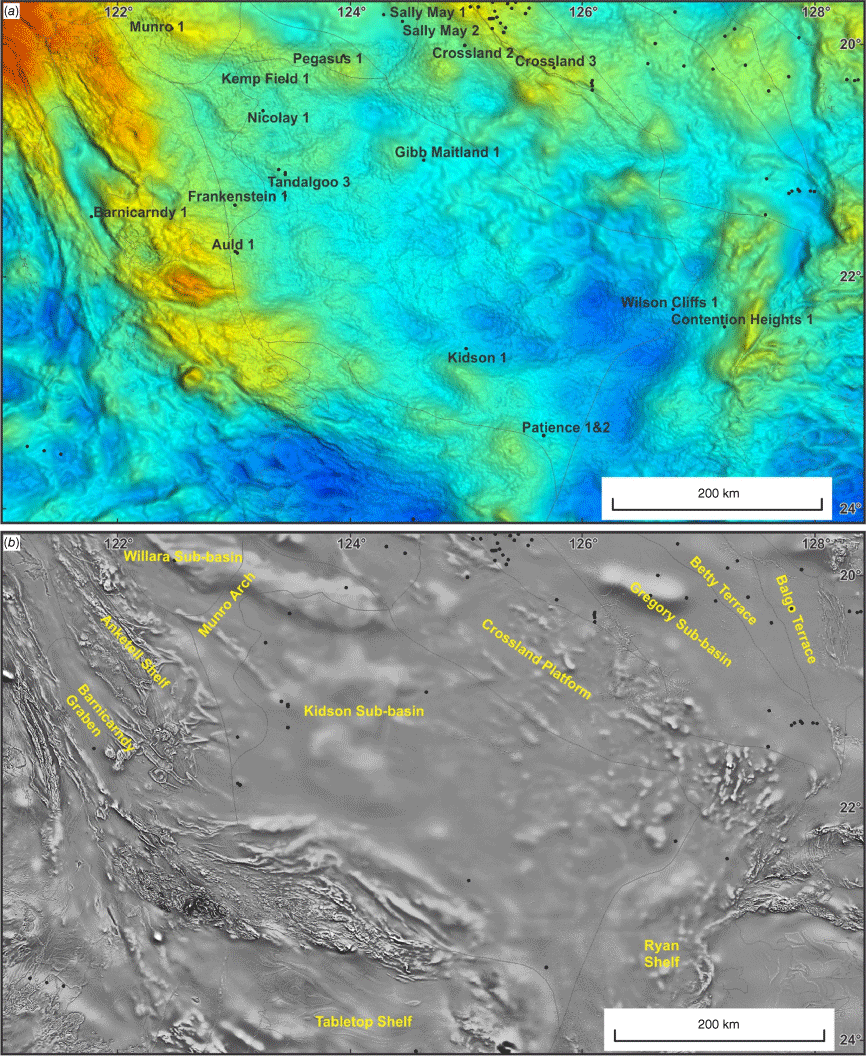
To fill these knowledge gaps and determine the sub-basin’s deepest point, this study focuses on assessing the basement configuration and analysing the potential and uncertainties for both petroleum and mineral resources. A multi-disciplinary approach is adopted with integration of seismic, AEM, gravity and aeromagnetic data (Fig. 2). By leveraging these diverse datasets, this paper presents an alternative model for the sub-basin’s geometry, with implications for resource propectivity within the Paleozoic succession.
Previous understanding
The Kidson Sub-basin has long been considered as a relatively simple sag deepening into its depositional axis, inferred to be in the central part of the sub-basin. The Canning SEEBASE© interpretations (Frogtech Geoscience 2017; Fig. 1a), which were largely based on gravity and magnetic data, have provided valuable insights, suggesting that the sub-basin deepens towards its southwestern and southeastern regions. This deepening trend was interpreted by Frogtech Geoscience (2017) as the result of overthrusting of the ‘Kidson Craton’ from the southwest by the Rudall Province and from the east by the ‘Gillespie Terrane’. The thickest part of the sedimentary successions was mapped in the southeast region near the Ryan Shelf (Frogtech Geoscience 2017; Fig. 1), predominantly aligned with Bouguer gravity anomalies observed in the area (GSWA 2020; Fig. 2a).
Subsequent studies, including the analysis of gravity gradiometry and the Kidson seismic survey, have further shed light on the structural characteristics of the Kidson Sub-basin. Interpretations (e.g. Southby et al. 2019; Zhan and Haines 2021; Fig. 3a) indicate that the sub-basin forms a broad sag depression, alongside the Ryan and Anketell shelves. The Kidson seismic survey shows several apparent folds, but these are caused by acquisition along bends in the road, and the sag remains relatively undeformed. Notably, high-angle faults are present in the Lower–Middle Ordovician strata along the eastern and western margins. Moreover, the configuration of the lower succession along these margins suggests that the Kidson Sub-basin originally extended beyond its current boundaries to the west and east. However, uplift and erosion processes have subsequently removed part of the sedimentary sections from these areas, leaving remnants along the sub-basin’s edges or possible outliers, such as the Cobb Embayment, beyond the main part of the Kidson Sub-basin.
Regional seismic sections in the Kidson Sub-basin: (a) Kidson seismic survey across the southern flank of the sub-basin; (b) composite line across the northwestern part of the sub-basin and Crossland Platform.
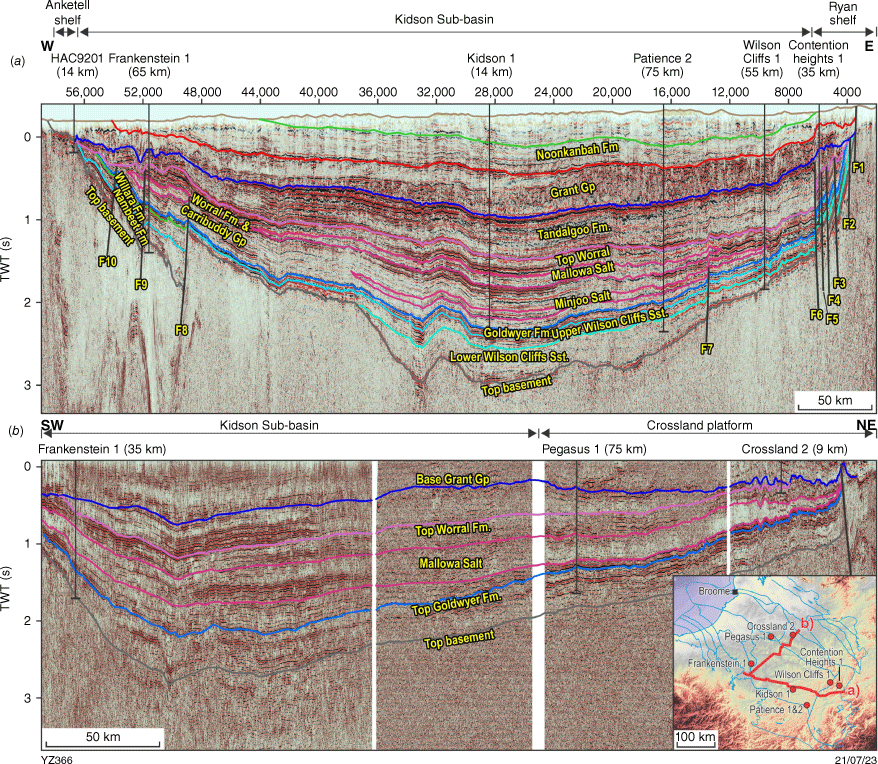
Although the combination of limited seismic data and well data from the sub-basin’s periphery allows for extrapolation of the basement structure, it becomes apparent that the Lower–Middle Ordovician strata should deepen towards the central part of the sub-basin, creating a deep depocentre in the southern region of the Canning Basin (Figs 1 and 2). However, it is important to note that this understanding of the sub-basin as a simple structural entity is solely based on mathematical extrapolation, and the correctness of this concept remains uncertain due to the significant data gap to the north of the Kidson seismic survey.
Integrated interpretation
The Kidson seismic survey has addressed some key questions regarding the depth and scale of the depocentre within the southern flank of the sub-basin. However, despite these advancements, challenges and uncertainties regarding basin structure still persist in the region. For instance, the regional seismic survey was oriented in a west–east direction nearly parallel to the strike of the Kidson Sub-basin, posing difficulties in determining the structure away from the survey. Additionally, one of the biggest faults (F8 in Fig. 3a; Zhan and Haines 2021) swings back and re-intersects the seismic profile in the Barnicarndy Graben as the Parallel Range Fault, leading to uncertainty as to where and how much it extends into the Kidson Sub-basin. The directional disparity complicates the interpretation of the sub-basin’s structural framework and necessitates a comprehensive approach to achieve a better structural understanding. This study integrates all available seismic profiles with subsurface geology, AEM, gravity and magnetic data to delineate an alternative framework of the Kidson Sub-basin.
Geomorphology comparison between basement and Permian
Well correlations from west to east across the Kidson Sub-basin (Fig. 4; after Haines 2011; Zhan and Haines 2021) have revealed the presence of several unconformities, including the base of Cenozoic sediments, base of the Permo–Carboniferous Grant Group and top of the upper Silurian–Lower Devonian Worral Formation. These unconformities, along with the Permian Noonkanbah Formation, exhibit a deepening trend from the sub-basin’s margin towards its central region. The deepening becomes more accentuated in the Lower–Middle Ordovician strata, leaving basement un-intersected before total depth at 4184 m in Patience 2 and 4435 m in Kidson 1 in the central area.
The Kidson seismic survey (Fig. 3a; Zhan and Haines 2021) has imaged the structural trend identified from depth variation of formations in well intersections. The structural trend is parallel within the Paleozoic strata, including the tops of the Noonkanbah Formation, Grant Group, Worral Formation, Carribuddy Group, Goldwyer and Nambeet formations above the basement. The structural similarity in the Paleozoic can also be observed in a northeast–southwest composition seismic profile (Fig. 3b), indicating that the entire sedimentary fill inherited the fundamental geometry of the basement despite several episodes of uplifting and erosion in the Kidson Sub-basin.
The structural inheritance observed in the Paleozoic succession holds significant implications, as it provides the possibility of projecting the unknown basement geometry from shallow horizons. Based on the structural pattern observed in the Permian, the overall basement configuration can be extrapolated from the known area near the margins of the Kidson Sub-basin to the central part of Kidson Sub-basin where the seismic data is not available.
Permian interpretation of AEM and wells
In contrast to the basement and Lower Ordovician horizons, the Permian sequence is better defined by the abundant well intersections and AEM data (Fig. 1a, b). The AusAEM dataset covered an area from the northwest part of the NT to the western coast of WA at a nominal line spacing of 20 km in two tranches. The lines in the study area were west–east-oriented and strategically positioned to fly across existing wells, thereby providing data calibrations for the unexplored area.
The AEM conductivity depth images (CDI) provide lithological information down to 600 m after data inversion by GA. Based on calibration with wells, it is evident that a relatively conductive zone corresponds to the Noonkanbah Formation, reflecting its mudstone and siltstone content (Fig. 5). A high level of consistency exists between the conductivity zone and the penetrated formation in most of the wells, including Frankenstein 1 (Fig. 5d), Wilson Cliffs 1 (Fig. 5f), Contention Heights 1 (Fig. 5g), Kidson 1 (Fig. 5h) and Patience 2 (Fig. 5i). Some exceptions exist in Auld 1 and South Auld 1, which exhibit mismatches in conductivity due to salt water in nearby lakes (Fig. 5e).
West–east AEM section across the Kidson Sub-basin, see Fig. 1b for profile locations of parts (a)–(i).
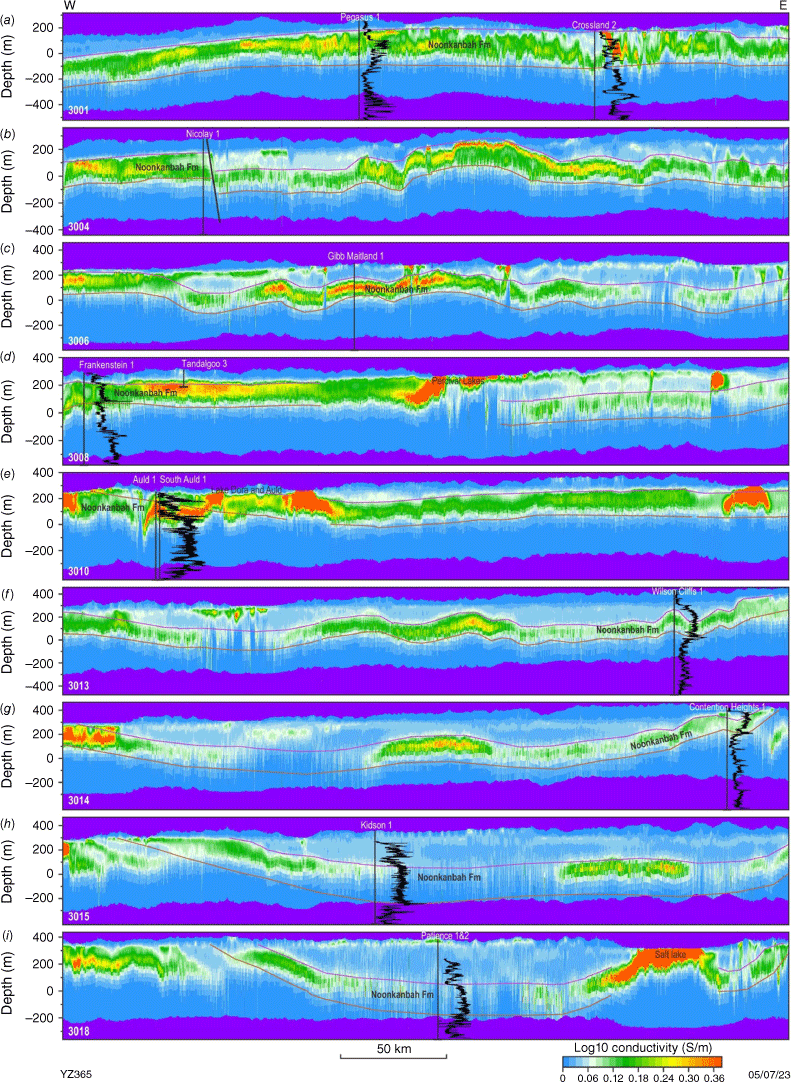
The significant consistency between the intersected geology and AEM data provides reasonable confidence to trace the top and base of the Permian Noonkanbah Formation on the conductivity profiles. However, due to some conductive overburden from salt lakes and paleochannels, for example, Lake Dora and Percival Lakes (Fig. 5d, e, i), these ultra-high conductive bodies and their underlying resistive zones need to be discounted when interpretating the Noonkanbah Formation. Nevertheless, the lateral intrusion of the saltwater into the formation is notably less pronounced than seawater in the Broome Sandstone near the coastal area (Zhan 2022), suggesting a much lower porosity and permeability in the Permian siltstone and shale units. The AEM data also indicate the possible presence of faults displacing the shallow formations (Fig. 5b). These are likely oriented in a north–south direction but are difficult to correlate across the AEM profiles. Any faults oriented in a west–east direction, if they exist, would be difficult to observe from the current AEM data as they will be parallel to the survey direction.
The AEM CDI sections show that the top of the Noonkanbah Formation generally dips from ~300 m above mean sea level (AMSL) in the periphery of the sub-basin to ~30 m AMSL in the central area (Fig. 5). However, three profiles (line 3010 in Fig. 5e and lines 3011, 3012 in Fig. 6a) indicate a relatively shallow top (~250 m AMSL) to the Noonkanbah Formation in the previously perceived depocentre. The shallow position of the Noonkanbah Formation (Fig. 6a) in the central part of the Kidson Sub-basin might explain the partial alignment with water wells sunk along the Canning Stock Route, where water exists near surface and required minimal digging when constructing a series of wells in the Great Sandy Desert during the Canning 1906 survey (Fig. 6b, c). The less permeable bed at the structural high (Fig. 6a) could act as a lower confining bed, preventing water from leaking through the sand dunes and sandstones, providing containment of the aquifer at shallow depths.
AEM interpretation and mapping: (a) line 3012 across Well 36 and 37 along the Canning Stock Route; (b) depth to top of Noonkanbah Formation; (c) depth to base of Noonkanbah Formation.
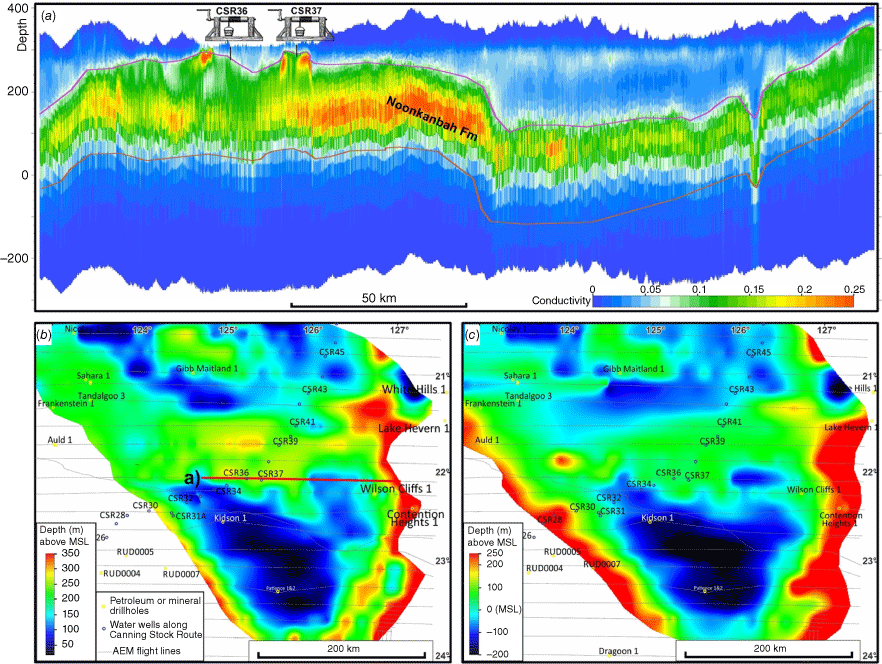
The depth maps (Fig. 6b, c) for the top and base of the Noonkanbah Formation are gridded from the depth interpretation of AEM sections, highlighting a near west–east trending structural high that divides the sub-basin into north and south structural components. Within the two components, the top of the Noonkanbah Formation lies at ~80 m and ~30 m AMSL respectively, compared to ~250 m AMSL along the structural high in the central area.
Basement mapping with constraints
Seismic data in the area of interest is mostly unavailable beyond the area of the current Kidson seismic survey, particularly to the north of the survey, where the data gap is approximately 100 km wide and 300 km long. On the other hand, the AEM data has a limited depth of investigation and does not provide any direct information for the deep basement. The lateral gap of seismic data and depth limitation of the AEM data pose significant challenges to the interpretation of the basement structure, if relying on them separately. However, the geomorphology comparison exhibits an inheritance of structural geometry from the basement to Permian strata based on well correlation and existing seismic lines (Fig. 3a, b). It is possible, therefore, to project a basement ridge within the sub-basin on the basis of the structural high seen in the Permian Noonkanbah Formation. The depth difference from the ridge to the southern depocentre should be greater at basement level than at the Permian and likely exceeds 500 m or more.
This extrapolated basement ridge is approximately aligned with the elevated basement shown on the Kidson seismic profile (common depth point, CDP 38,000–49,000 in Fig. 3a), prompting the possibility that its boundary is a fault that extends into the Kidson Sub-basin as a splay of the Parallel Range Fault. Here, the basement ridge is mapped with the north-dipping boundary fault along the trend of the Noonkanbah Formation (Fig. 7a). Based on the interpretation of the Kidson seismic survey, the fault (F8 in Fig. 3a) was likely initiated before the development of the Canning Basin, with a component of syn-depositional movement during the Early Ordovician, as shown by the thickening trend within the hanging-wall towards the boundary fault.
Supporting evidence
The mapping of the basement ridge, based on its assumed structural manifestation in the Permian strata, offers a reasonable explanation for the depositional compartmentalisation between the southeast and the northwest of the Kidson Sub-basin. It also accounts for the differences in seismic signatures near the flanks, as well as the gravity and magnetic responses.
Depositional variation
The Lower–Middle Ordovician succession comprises the Nambeet, Willara and Goldwyer formations, which were commonly intersected in the Willara Sub-basin and Broome Platform. In the reference section of the Nambeet Formation in Olympic 1 (Dent et al. 2021), its upper Samphire Marsh Member is predominately composed of mudstone and carbonate, whereas the underlying Fly Flat Member is sandstone-dominated. The Nambeet Formation is widespread in the Willara Sub-basin and Broome Platform, but it is absent in Wilson Cliffs 1, Contention Heights 1 and Patience 2 in the southeast of the Kidson Sub-basin. Instead, those wells intersected the Wilson Cliffs Sandstone, which was considered an age-equivalent to the Nambeet Formation based on conodont data from Wilson Cliff 1 (Nicoll 1993).
The Willara Formation contains predominantly limestone, accompanied by lesser amounts of dolostone, mudstone, and sandstone, all of which were deposited in shallow marine environments in the Willara Sub-basin and Broome Platform (Haines 2004). A significant portion of the Willara Formation, if not all, appears to be missing in the southeast of the Kidson Sub-basin, based on the absence of four conodont zones in Wilson Cliffs 1 (Nicoll 1993). The intersections in Wilson Cliffs 1, Contention Heights 1, Kidson 1 and Patience 2 show that the Goldwyer Formation directly overlies the Wilson Cliffs Sandstone, suggesting a possible depositional hiatus after the deposition of the Wilson Cliffs Sandstone in the southeast (Figs 4, 7b).
The Goldwyer Formation consists of predominately mudstone with intraformational carbonate build-ups in the Willara Sub-basin and Broome Platform area. In the northwestern part of the Kidson Sub-basin, Nicolay 1 intersected a similar package of lithologies within the 293 m thick Goldwyer Formation (New Standard Onshore Pty Ltd 2013). Haines (2004) indicates a broad pattern for the ratio of mudstone to carbonate in which the formation is more mudstone-dominated in deeper basinal regions, whereas the carbonate percentage generally increases on adjacent platforms and terraces. This lithology combination can be visualised in the coastal area on seismic profiles, where high-amplitude reflectors correspond to the carbonate member and are enclosed by thick, low-amplitude mudstone packages (Zhan 2017). However, these seismic characteristics are not seen when interpreting the Kidson seismic survey and nearby lines due to the significant increase of sandstone to the southeast: abundant siltstone and fine-grained sandstone can be found in Wilson Cliffs 1, Patience 2, Kidson 1, and Contention Heights 1 with much less carbonate compared to the wells in the Willara Sub-basin and Broome Platform. The difference in the lithofacies of the Goldwyer Formation suggests a source of fine sand into the southeastern Kidson Sub-basin (Haines 2011) that was not transported to the area of open marine conditions in the northwest. In addition to the lithofacies, the thickness of the formation also varies significantly, ranging from 300 to 900 m from the Willara Sub-basin to Munro Arch in the northwest (map 19 in Zhan 2019) to 100–250 m in the southeast (Haines 2011; Zhan and Haines 2021).
The variations in the Nambeet and Willara formations from the northwest to southeast posed challenges in interpreting the Kidson seismic survey and led to the interpretation of a stratigraphic boundary along the splay of the Parallel Range Fault (F8 in Fig. 3a; Zhan and Haines 2021), effectively dividing the Lower Ordovician sequence into the Nambeet and Willara formations on the western flank of the sub-basin from the Wilson Cliffs Sandstone in the majority of the sub-basin. The basement high and associated fault probably played a similar role during the deposition of the Goldwyer Formation, separating a relatively high-energy environment with more sand sources in the southeast from the quiet low-energy marine environment in the northwest.
The sub-division of the Kidson Sub-basin by the proposed ridge suggests that the northwestern part of the sub-basin and the Willara Sub-basin may have shared a similar depositional environment during the Lower–Middle Ordovician. This is consistent with well intersections in the Willara Sub-basin (e.g. Willara 1), Broome Platform (e.g. Olympic 1, Theia 1), and northwestern part of Kidson Sub-basin (e.g. Nicolay 1, Frankenstein 1), all of which exhibit significant differences in facies from the wells in the southeast (e.g. Kidson 1, Patience 2, Wilson Cliffs 1 and Contention Heights 1).
The potential ridge mapped from AEM data is connected with the elevated basement shown on the Kidson seismic survey (CDP 38,000–49,000 in Fig. 3a; plate 1 of Zhan and Haines 2021). Regardless of whether the boundary fault (F8) extends farther into the sub-basin, the near west–east-oriented basement high might be the explanation for the differences in the Lower–Middle Ordovician succession in terms of the depositional environment and thickness variation of the Nambeet and Goldwyer formations, as well as the absence of the Willara Formation.
Seismic profiles near the southern flank of the ridge
Apart from the Kidson seismic survey, which potentially crosses the western end of the proposed basement ridge (Fig. 3a), no seismic data are available to resolve the structure in the middle part of the Kidson Sub-basin. However, a few seismic profiles lie near the southern flank of the ridge and shed some light on the regional structure (Fig. 8).
Seismic profiles and maps near the possible ridge: (a) line T73-Helena-E to the east; (b) line U-K81-003 in the southern margin; (c) line U-K81-004 in the southern margin; (d) map of Kidson Southeast Reconnaissance A1 and B1 in 1965 (section lost); (e) enlarged map showing original basement interpretation by Young (1965); (f) location map of the seismic lines.
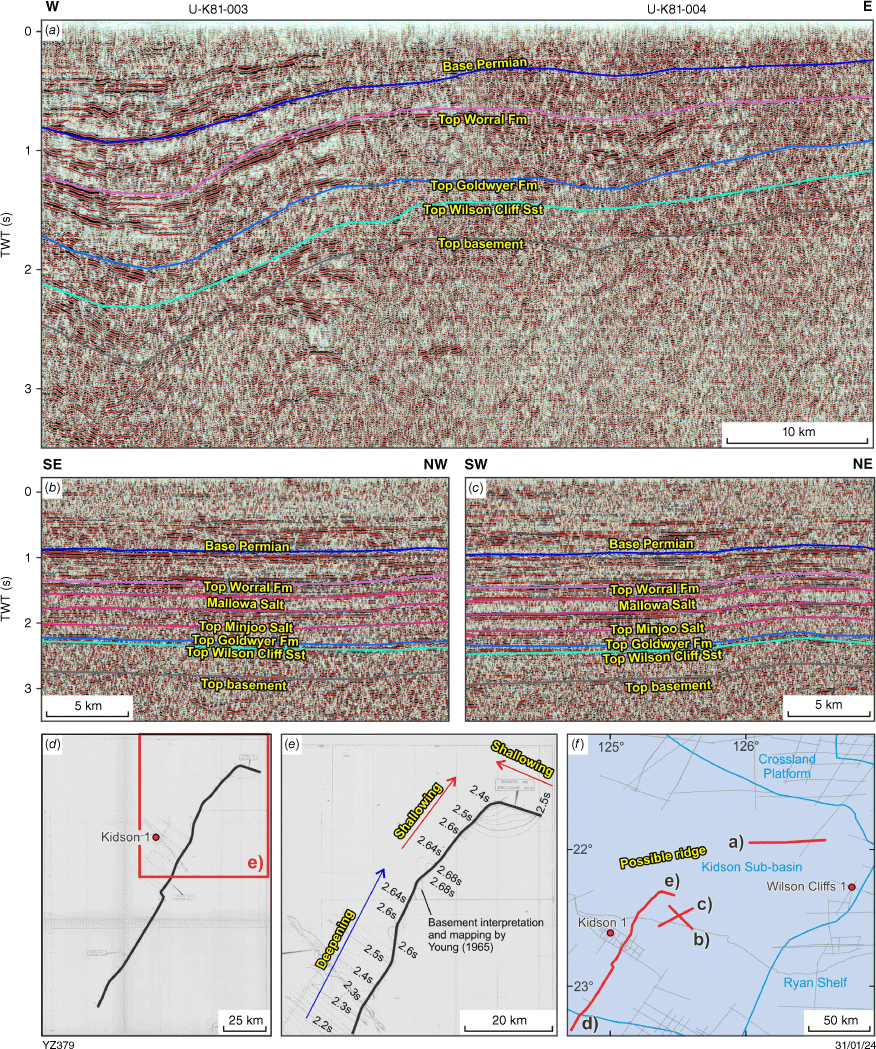
Thornton-Helena-E line (Fig. 8a) shows that the Paleozoic succession is generally dipping from the east to the central part of the Kidson Sub-basin. However, the profile shows that the basement shallows from ~2.8 to ~2.5 s over a distance of 8 km near its western end. On the southern margin of the ridge, two seismic lines from the Ural survey show a similar but more subtle shallowing towards the central part of the sub-basin near the northern end of the profiles (Fig. 8b, c). The Kidson Reconnaissance survey (Fig. 8d) was acquired about 30 km west of these Ural lines in 1965, but the data was lost for the northwest portion of the seismic profile. According to the original interpretation of the profile by Young (1965), the basement depth decreases from approximately 2.68 s in the southeast to about 2.4 s in the northwest (Fig. 8e), similar to the shallowing trend observed from other nearby lines.
Nevertheless, without a seismic line covering the ridge, the structural changes are only shown as a small-scale basement rise near the ends of the seismic profiles. Those local small-scale variations would be masked during the contouring process by the broad deepening trend around the periphery of the Kidson Sub-basin. Thus, the Permian constraints provided by the AEM data become important for mapping the area where seismic data are not available. The trend of rising basement observed from the seismic lines in the Kidson Sub-basin (Fig. 8) is consistent with their proximities to a ridge, with the structural crest likely farther north (Fig. 7).
Signatures on gravity and magnetic data
Gravity and magnetic data have been extensively utilised in the SEEBASE study (Frogtech Geoscience 2017), revealing the deepest basement about 40 km south of Wilson Cliffs 1 in the southeast. Within the interpreted depocentre (Frogtech Geoscience 2017), the north–south strike correlates with the Bouguer gravity in the region (Fig. 2a; GSWA 2020). The gravity data was upgraded by a Falcon gradiometry survey, which increased the resolution and showed similar gravity lows in the southeast margin of the Kidson Sub-basin. The new gravity image shows moderate highs along the proposed ridge in the central part of the Kidson Sub-basin, compared to the depocentres on either side, although this is not a prominent anomaly.
Magnetic responses along the ridge are much more subtle than the gravity response, possibly due to the depth and magnetic susceptibility of the basement (Fig. 2b; GSWA 2023). The first vertical derivative of the magnetic grid shows discrete anomalies south of the boundary fault, providing possible evidence for the presence of the elevated basement in the central part of the Kidson Sub-basin. However, those magnetic anomalies are more isolated and difficult to interpolate about 50 km to the north of Kidson 1 (Fig. 2b).
These subdued gravity and magnetic signatures, especially the feature about 60 km to the northwest of Kidson 1, corroborate the concept of the basement crest inferred from the AEM data and the ends of seismic lines (Fig. 7). The gravity high to the northwest is likely connected to the elevated basement observed on the Kidson seismic profile (Fig. 3a), forming an elongated west–east structure along the fault splays.
Prospectivity discussion
The interpretation of the basement ridge carries a high level of uncertainty, as discussed above. Nonetheless, it is worthwhile investigating the implications for resource prospectivity should the interpreted structure be correct. This section discusses the exploration potential for hydrocarbon resources and carbon dioxide geosequestration, as well as carbon-free, basement-sourced hydrogen, helium and minerals.
The Nambeet, Goldwyer and Bongabinni formations have long been identified as possible hydrocarbon source rocks in the Willara Sub-basin and Broome Platform (Taylor 1992; Kennard et al. 1994; Edwards et al. 1997; Haines 2010; Ghori 2013). Recent data from Olympic 1 have provided further evidence for organic-rich source rocks within the Nambeet Formation (Normore and Dent 2017; Dent et al. 2021). After extensive analyses of total organic carbon (TOC) and Rock-Eval pyrolysis for Olympic 1, the latter two studies suggested that the Nambeet Formation consists of two source intervals that are laterally continuous, based on well correlations and seismic interpretation, and hold high potential for hydrocarbon generation.
The main hydrocarbon source sequence in the Ordovician section is probably the Goldwyer Formation, which contains rocks with high concentrations of oil-prone Gloeocapsomorpha prisca-bearing rocks, known as ‘kukersite’ for oil shale of Ordovician age (Foster et al. 1986; Edwards et al. 1997; Haines 2004). Theia 1 drilled on the Broome Platform in 2015 intersected organic-rich shales within the Goldwyer Formation that exhibit good to very good potential for unconventional resources. Finder Shale Pty Ltd (2016) concluded that the production index at 1554 m reached a maximum of approximately 0.38, and thermal maturity equivalent to 1.1% vitrinite reflectance, placing its lower member within the peak to late oil generative window.
In addition to the Nambeet and Goldwyer formations, the Middle–Upper Ordovician Bongabinni Formation is locally dominated by dark green to dark grey and occasionally black calcareous mudstone varying to argillaceous limestone in mineral exploration drill cores along the Admiral Bay Fault Zone (Haines 2010). The formation has black coal-like interbeds up to about 1 min thickness, which have been referred to as algal coal (McCracken 1994) or oil shale (Edwards et al. 1995; McCracken 1997). Such beds were analysed by Haines (2010) and shown to contain the richest source potential of any rocks reported from the Canning Basin, with TOC values ranging from 3.9 to 62.2% and potential yield (S1 + S2) varying from 4.9 to 208.2 mg/g. The organic-rich rocks may extend into the Willara and Kidson sub-basins, improving the possibility of the source reaching sufficient maturity to generate significant hydrocarbons under the deep burial in the depocentre.
The proposed basement ridge separates the southeastern portion of the Kidson Sub-basin from the northwestern portion, the latter connected to the Willara Sub-basin. Hydrocarbons sourced from the Bongabinni, Goldwyer and Nambeet formations could have migrated from that northwest depocentre into overlying reservoirs above the ridge. The dolomitised Nita Formation above the Goldwyer Formation has been ranked as the most promising potential reservoir because of repeated oil and gas shows (Karajas and Kernick 1984; Jackson et al. 1994; McCracken 1997; Haines 2004), with the Gloeocapsomorpha prisca biomarker indicating source from the Goldwyer Formation (Edwards et al. 1997). In addition to the Nita Formation, the Wilson Cliffs Sandstone is believed to extend from the southeast to the inferred ridge (plate 1 of Zhan and Haines 2021) and can potentially serve as an additional reservoir unit. Above the reservoir, the Upper Ordovician–Silurian Carribuddy Group and Worral Formation contain thick and extensive evaporite facies that could form an effective regional seal over underlying petroleum systems (Haines 2010).
The shallower Ordovician section above the inferred basement ridge would offer advantages, such as reduced drilling cost and favourable traps, for hydrocarbon accumulation within the footwall block or anticlines without fault splays. Based on the Kidson seismic survey, the geometry in the sub-basin appears to be less complex compared to the Fitzroy Trough (Zhan and Mory 2013), with fewer faults or inversion features. It seems that the younger movement in the Fitzroy Trough did not propagate into the Kidson Sub-basin. If this is the case, it is conceivable that the traps would remain unbreached within the west–east-oriented ridge.
Unbreached structural closures along the ridge may be suitable for carbon sequestration purposes within the Lower–Middle Ordovician Wilson Cliffs Sandstone and Nita Formation and potentially the Devonian Tandalgoo Formation above. However, the structural integrity and reservoir quality in this part of the basin remain untested, and any potential is highly conceptual until proven by drilling.
The proposed ridge structure is likely connected through a series of fault splays with the basement high observed on the Kidson seismic survey (Zhan and Haines 2021). If these faults are the eastern extension of the Parallel Range Fault in the middle part of the Kidson Sub-basin, it is likely that the basement below the Paleozoic succession belongs to the Neoproterozoic Yeneena Basin. With this configuration, the ridge structure could also be favourable for exploration for other resources, such as hydrogen, helium and minerals. Based on geochemical and geophysical data, Lefeuvre et al. (2022) proposed four factors that constitute a promising geological setting for natural hydrogen exploration: (1) shallow basement under the right pressure–temperature conditions for serpentinisation, (2) major faults for large-scale fluid flow convergence and drainage, (3) sharp relief and temperature pressure gradients to trigger fluid migration, and (4) impermeable caprocks above porous reservoir to trap hydrogen. All could be present within the speculative ridge structure and applicable in the exploration of basement-sourced natural hydrogen and helium in the Kidson Sub-basin.
In terms of mineral exploration, the movement of the Parallel Range Fault and its splays beneath the Kidson Sub-basin might have had a similar structural history to those in the west, where gold and copper deposits have been discovered at Telfer, Nifty and Winu. Therefore, the Neoproterozoic strata underneath the ridge could be favourable to similar mineralisation. However, the depth of the inferred Neoproterozoic would limit exploration on economic grounds.
Uncertainty and de-risking analysis
The existence of a basement ridge in the Kidson Sub-basin is uncertain due to limited data. Despite supportive evidence from regional depositional facies, marginal seismic data, and gravity and magnetic maps, the lack of a seismic profile across the ridge or drilling leaves its presence unconfirmed. The current AEM data, limited in coverage and orientation, do not provide definitive proof of a shallow conductive zone for the Noonkanbah Formation (Fig. 1b). Even if future AEM data suggests a high conductivity ridge, this electrical response would only indicate changes in subsurface lithostratigraphy, rather than the strata age. In other words, the high conductivity zone might be younger than the Noonkanbah Formation, such as the Liveringa Group, in which case the Noonkanbah Formation would be still deeper and not indicative of the basement shape.
The inference of a ridge in the basement and Paleozoic section is based on the assumption that the structural geometry of the Permian section was inherited from the basement topography observed from the Kidson seismic survey. However, this assumption may not necessarily be applicable to the central part of the sub-basin, even if the Permian Noonkanbah Formation does possess two depocentres. For example, these two depocentres could be caused by deeper salt dissolution or withdrawal, forming two synclines on either side of the ridge. In this case, the formations underlying the salt would be less affected, and the basement geometry would not necessarily resemble the geometry of the Permian strata. Due to the high level of uncertainties and limited data, further work will be required to confirm the presence of the inferred ridge in the middle of the Kidson Sub-basin (Fig. 9). Such de-risking investigations should be considered based on cost, data availability and logistical feasibility:
Reprocessing of the AEM data is recommended to avoid any erroneous conductivity profiles. Currently, the AEM data were inverted on a line-by-line basis, rather than being processed in a 3D conductivity model. This has led to uncertainty about the existence of a conductive zone at the shallow depth shown on three critical profiles, despite it appearing on two independent models from CGG and GA.
An AEM survey could be acquired in a north–south direction to complement the AusAEM02 data (Fig. 9). This will form a grid of AEM profiles and provide improved spatial information over the conductive zone, specifically its shallowing trend towards the inferred ridge.
Shallow drilling would be valuable in the uppermost 200 m section to test whether the Noonkanbah Formation exists at shallow levels over the inferred ridge (Fig. 9). Currently, there is lack of petroleum wells, mineral drillholes or waterbores within the area of the data gap. The shallow drillings could be designed along AEM line 3012 to calibrate the AEM and perpendicular to the line to assess if an anticlinal structure exists within the Noonkanbah Formation.
With confirmation from shallow AEM data, a passive seismic survey could be prioritised to investigate any velocity variations in the deep Paleozoic section and the basement within a depth range of 2000–7000 m across the ridge (Fig. 9).
Once a ridge signature is observed from AEM and passive seismic surveys, an ideal next step would be a reflection seismic survey across the proposed ridge, with an aim to intersect the Kidson seismic survey and tie to Kidson 1 and Gibb Maitland 1. However, logistical challenges are anticipated, such as operating across sand dunes.
Deep drilling could be conducted on the inferred ridge to obtain the information about the sedimentary succession, as well as the petroleum and mineral potential within the Paleozoic and Precambrian basement.
Conclusion
The Kidson Sub-basin in the southern Canning Basin has long been considered as a simple structural depression with a general deepening of basement towards its central region. The reliability of this interpretation is limited by poor data coverage, particularly in the central part of the sub-basin, and has here been tentatively revised after integrated mapping of the area. Well correlations and seismic profiles, where available, suggest a structural resemblance between the deep basement and shallow Permian section. This relationship can be used to map the basement structure in areas where there is a lack of seismic data.
Using downward extrapolation of the structural pattern in the shallow Permian strata, a basement ridge can be tentatively delineated in the central part of the Kidson Sub-basin, potentially rising 500 m or more above the basement depth in the southern depocentre. If this elevated basement structure exists, it probably connects with the basement high shown on the Kidson seismic survey, with the boundary fault initiated before the development of the Canning Basin.
The proposed ridge explains the variations in thickness and depositional facies in Lower–Middle Ordovician section from the southeast to northwest. Wells in the northwest commonly encountered thick mudstone and carbonate in the Nambeet, Willara and Goldwyer formations, whereas across the ridge, the southeast sub-basin contains Nambeet-equivalent Wilson Cliffs Sandstone, sandy and thin Goldwyer Formation, with the Willara Formation missing. The ridge structure is further supported by subtle shallowing trends observed on the ends of seismic profiles near the southern flank of the proposed ridge. The gravity and magnetic data also suggest different responses in the two depocentres and exhibit some isolated anomalies along the inferred ridge.
The existence of this basement ridge remains highly uncertain, given the lack of a seismic profile across the ridge or drilling to confirm basement at shallower depths than expected from the previous basin models. To verify the presence of this ridge, further work would be required, potentially including reprocessing of the AEM data, acquisition of north–south-oriented AEM profiles to form a grid, shallow drillings to test the depth of the Noonkanbah Formation, passive and reflection seismic surveys across the ridge, and deep drilling.
The potential ridge could enhance the prospectivity of the area for petroleum, natural hydrogen and helium, as well as mineral resources, compared to the currently perceived simple structural entity for the Kidson Sub-basin. Organic-rich source rocks of the Nambeet and Goldwyer formations are widespread to the northwest of the ridge and have been substantiated through recent drilling in the Willara Sub-basin and Broome Platform. Hydrocarbons sourced from these two formations could have migrated towards the footwall block on the ridge via the east extension of the Parallel Range Fault. Reservoir units are probably present on the ridge, including the dolomitised vuggy Nita Formation and the Nambeet-equivalent Wilson Cliffs Sandstone. The thick and extensive evaporite facies in the Carribuddy Group and shale in the Worral Formation can provide an effective regional seal.
The traps within the west–east-oriented ridge inferred within the Kidson Sub-basin are believed to be less affected by younger movements and remain unbreached, compared to the Fitzroy Trough. These structural closures also have potential as sites for carbon sequestration within the thick Paleozoic succession. The basement within the proposed ridge is possibly related to the Neoproterozoic Yeneena Basin that is exposed to the west and has potential for mineral deposits along the extension of the Parallel Range Fault, although the greater depth compared to existing deposits may limit their economic potential.
Data availability
The data that support this study are available in Western Australian Petroleum and Geothermal Information Management System (WAPIMS) at https://wapims.dmp.wa.gov.au/wapims, GSWA's Geophysical survey index and data repository (MAGIX) at https://magix.dmirs.wa.gov.au/, and Geoscience Australia data portal for AusAEM 02 WA/NT 2019–20 Airborne Electromagnetic Survey at https://ecat.ga.gov.au/geonetwork/srv/eng/catalog.search#/metadata/140156.
Declaration of funding
This research has been supported by the Government of Western Australia through GSWA operational funding.
Acknowledgements
The author acknowledges the constructive comments and suggestions from Peter Haines, Deidre Brooks and two anonymous reviewers. Adam Symonds is also thanked for drafting the figures. This paper is published with the permission of the Executive Director of the Geological Survey of Western Australia.
References
Edwards DS, Summons RE, Kennard JM, Nicoll RS, Bradshaw J, Bradshaw M, Foster CB, O’Brien GW, Zumberge JE (1997) Geochemical characteristics of Palaeozoic petroleum systems in northwestern Australia. The APPEA Journal 37, 351-377.
| Crossref | Google Scholar |
Foster CB, O’Brien GW, Watson ST (1986) Hydrocarbon source potential of the Goldwyer Formation, Barbwire Terrace, Canning Basin, Western Australia. The APPEA Journal 26, 142-155.
| Crossref | Google Scholar |
Haines P (2010) The Carribuddy Group and Worral Formation, Canning Basin, Western Australia: Reassessment of stratigraphy and petroleum potential. The APPEA Journal 50, 425-444.
| Crossref | Google Scholar |
Karajas J, Kernick CN (1984) A prospective Nita Formation reservoir trend on the Broome Platform, in the Canning Basin. In ‘Geological Society of Australia and Petroleum Exploration Society of Australia, Canning Basin Symposium’, 1984, Perth, WA. (Ed. PG Purcell) Proceedings. pp. 169–177. (GSA, Western Australian Branch)
Lefeuvre N, Truche L, Donzé F-V, Gal F, Tremosa J, Fakoury R-A, Calassou S, Gaucher EC (2022) Natural hydrogen migration along thrust faults in foothill basins: The North Pyrenean Frontal Thrust case study. Applied Geochemistry 145, 105396.
| Crossref | Google Scholar |
McCracken SR (1994) Timing of hydrocarbon migration into the Admiral Bay Fault Zone, Canning Basin. In ‘The Sedimentary Basins of Western Australia. Proceedings of the PESA West Australian Basins Symposium’, Perth, WA. (Eds PG Purcell, RR Purcell) pp. 739–751. (Petroleum Exploration Society of Australia)
Southby C, Carr L, Henson P, Haines P, Zhan A, Anderson J, MacFarlane S, Formin T, Costelloe R (2019) Exploring for the future: Kidson Sub-basin seismic interpretation. ASEG Extended Abstracts 2019(1), 1-3.
| Crossref | Google Scholar |
![]() Yijie (Alex) Zhan graduated from China University of Geoscience (Beijing) with a BSc in 2005. He has worked on structural interpretation, fracture detection and reservoir modelling in various basins. He has published in English and Chinese on seismic interpretation, airborne electromagnetic data, azimuthal anisotropy, forward modelling and characterisation of gas-bearing volcanoclastic and conventional reservoirs. He joined the Geological Survey of Western Australia’s Basin and Energy Geoscience group as Senior Geophysicist in 2011, and is a member of PESA. |