Securing the Australian CCS project rollout by improving aspects of the GHG storage legislation: a discussion paper
Geoffrey O’Brien A , Simone de Morton A * and David Bason AA
![]() Geoff O’Brien is the Chief Scientist for CO2CRC. Geoff has over 35 years of experience in the marine research, petroleum, and CCS sectors and has worked in and for the petroleum industry as a petroleum systems and CCS technical specialist. He has also worked for Geoscience Australia, where he was the Research Group Leader for the Marine Environment & Offshore Petroleum Group, as a research consultant at the University of Adelaide, as the Energy Geoscience Manager at Geoscience Victoria and as the Chief Geoscientist for NOPTA. Dr O’Brien has a comprehensive understanding knowledge of Australia’s sedimentary basin systems and their petroleum potential, and over the past 15 years has become increasingly involved in the CCS sector. A passion is the development of tailored and targeted CCS work programs that relate specifically to the ultimate regulatory requirements, thereby facilitating the Pathway to Storage and providing greater efficiencies for CCS project proponents. |
![]() Simone de Morton is a Techno-Regulatory Advisor at CO2CRC with 8 years of experience in the industry, holding a PhD in Geology along with a Bachelor of Science (Honours) degree. Simone has worked as a Production Geologist and Exploration Geologist at ExxonMobil Australia and as a Geologist at the National Offshore Petroleum Titles Administrator (NOPTA). At CO2CRC, Simone has combined her extensive regulatory and geological expertise to provide techno-regulatory guidance to CCS project proponents and has delivered CCS regulatory educational courses to non-technical professionals. |
![]() David Bason is a Senior Reservoir Engineer with CO2CRC in Melbourne, Victoria with 15 years of experience in the Energy industry. David is currently developing the latest research program for Stage 4 CO2 experiments at the Otway International Test Centre. David also consults to CO2Tech on various CCUS-focused projects. He holds an MPhys from the University of Manchester, UK, and an MSc in Petroleum Engineering from Heriot-Watt University in Aberdeen, UK. David has previously worked as a reservoir engineer for Woodside Energy and as a consultant with EY. |
Abstract
The respective Federal petroleum and greenhouse-gas (GHG) storage legislation was examined in detail to understand the interactions between the technical requirements in the legislation, the overarching emissions-policy framework and the existing and likely future technical capabilities of the carbon capture and storage (CCS) industry. Key elements of the GHG legislation were then stress-tested using CCS project examples to determine applicability. The number of CCS projects required to meet the nation’s emission reduction targets over the next 5–25 years is high (probably between 20–40 projects of 4 million tonnes per annum (Mtpa)), but the existing 8–10 year approvals timeline provides only a maximum of 2.5 full project cycles to undertake 20–40 projects, a goal not considered possible without substantial regulatory reform. Opportunities for improvement should focus on facilitating a robust and rapid CCS project rollout and could include the following: changing the legislation to facilitate the accelerated utilisation of depleted petroleum fields to storage facilities, including allowing the progressive expansion of the awarded injection licence (IL); changing the concept of containment to emphasise carbon dioxide (CO2) plume management via assessing risk and potential impacts, not by artificial constraints into GHG permits and licences; including unitisation and combination pathways in the GHG legislation; ‘CO2 plume’ is not defined in legislation, even though the spatial location of a plume is central to numerous approvals, such as declaration of storage, demonstrating containment and all measurement, monitoring and verification (MMV) programs; developing a framework to manage future CCS project interactions at a basin scale, as is undertaken in groundwater management onshore.
Keywords: Australia, Australian GHG legislation, carbon capture and storage, CCS, CCS projects through time, CO2 plume definition, emission policies, GHG unitisation and combination certificates, historical basis of GHG legislation, informed pore space management, regulatory reform.
Introduction
Over the past 20 years, there has been a rapid evolution in the carbon capture and storage (CCS) landscape within Australia, across the policy, legislative–regulatory and project areas. Policy changes, such as the 43% emissions reduction by 2030 and Net Zero 2050 targets and the Safeguard Mechanism have driven a rapid uptick in the rollout of planned CCS projects in offshore Australian waters, which are now mostly in the early stages of the regulatory approvals cycle. These projects need to be approved efficiently and quickly if the nation is to meet its agreed targets in the required timeframes. If Australia’s booming liquified natural gas industry, a key part of the Asia Pacific energy security equation, is to have a secure future within a low-emissions framework, CCS is a necessity.
In late 2022, CO2CRC recognised that several key inefficiencies and inconsistencies were present within Australia’s otherwise comprehensive and well-constructed Australian Offshore Petroleum and Greenhouse Gas Storage Act (2006) (OPGGSA) and Offshore Petroleum and Greenhouse Gas Storage (Greenhouse Gas Injection and Storage) Regulations (2011) (GHG Regulations). These issues in the GHG legislation have probably stemmed largely from the influence of the petroleum framework within the OPGGSA, the age of the OPGGSA (~20 years) and, critically, the rapid and recent changes in the overarching policy area, which have produced a disconnect between the newer policies and the legacy regulatory regime. These issues had the collective potential to delay the nation’s CCS project rollout, resulting in higher than desired emissions, increased project costs, decreased economic activity, increased energy insecurity within the Asia Pacific and, in the worst case, cancelled or delayed LNG, gas and CCS investments.
We propose that some elements of the current regulatory regime are not fit for purpose due to three primary reasons, namely,
Their legacy nature and petroleum-based heritage.
A misalignment between the nature of the current and likely future CCS project rollout and the regulatory regime.
The fact that legislation is now somewhat asynchronous with overarching and major policy changes, such as the 2030 43% emissions reduction target, the Safeguard Mechanism and Net Zero 2050.
Discussion is restricted to the Australian offshore Federal jurisdiction and our focus is on larger (>2 million tonnes per annum, Mtpa) CCS-storage projects, mostly LNG developments offshore. A key objective has been to understand the high-level interplays between the developing CCS project mix, overarching emissions policy and the history of the legislation (i.e. its fundamental origins). The paper will investigate the key differences between petroleum projects and CCS projects, examine permitting processes and provide worked CCS project examples.
A number of options for GHG regulatory streamlining will be proposed that could increase regulatory efficiencies and eliminate artificial CCS project bottlenecks and obstructions.
Projects: an evolving GHG landscape
The dramatic changes in the CCS project landscape over the past 20–25 years are summarised in Fig. 1 for the periods 2006–2007, 2011, 2020 and 2023. Also shown are the planned sources of the CO2, operational status and key regulatory or policy developments that were introduced within those time intervals. The changes in the nature of the planned projects over time can provide insights into the factors that shaped investment.
In the early–mid 2000s, the four proposed CCS projects were largely situated onshore in eastern Australia and were focused on sequestering CO2 generated from power generation (Fig. 1).
By 2011, when the GHG Regulations were first introduced, six proposed CCS projects were under consideration. Focus had shifted principally to Western Australia (an exception was Victoria’s CarbonNet Project) and involved both CO2 sequestration from power generation and industrial production and CO2 capture from LNG.
Between 2011 and 2020, the number of potential projects decreased, with only Gorgon (now operational), CarbonNet and the Otway International Test Centre (OITC) remaining active (Fig. 1). However, Moomba CCS had appeared as a major potential project. Importantly, despite the introduction of offshore regulations in 2011, no new offshore projects were proposed or initiated.
In October 2021, the Federal government announced a net-zero emissions target by 2050 and released ‘Australia’s Long‑term Emissions Reduction Plan’ (APH 2023). Shortly after the 2022 Federal election, the new government legislated ‘Net Zero by 2050’. Subsequently, in 2023, the revised Safeguard Mechanism (SGM) was introduced. The goal of the SGM was to channel a reduction in industrial emissions to support achieving Australia’s 43% emission reduction targets and Net Zero by 2050 (DCCEEW 2023).
Alongside these policy changes, there was a surge in the number of proposed CCS projects to 17, with one operational (Gorgon) and one in the final development stage (Moomba CCS).
Policy: dramatically changing settings
Planned CCS projects in Australia have reached record numbers and are now dominantly emission mitigation strategies for LNG projects; these are situated principally offshore and are located mostly in Western Australia. This is a vastly different environment from when the supporting OPGGSA (2006) and the GHG Regulations (2011) were released.
LNG projects are large, multi-decadal operations and will hence produce significant CO2 from both the reservoir1 and from LNG production itself over their lifespan. Mitigation of these high volumes of CO2, potentially at storage rates of 4–20 Mtpa for hub-centric storage facilities, was not widely anticipated in either 2006 or 2011.
A generally accepted concept is that the regulatory environment significantly influences the progress of projects (OECD 2023). However, the life cycle of the Australian CCS projects over the past 20–25 years has been driven principally by recent policy shifts (43% 2030, SGM, Net Zero) rather than the pre-existing regulatory frameworks. Ideally, new regulations would have specific objectives (such as to reduce emissions) and would directly support the recently introduced policies, rather than the new policies being supported by a legacy regulatory suite, a suite that was developed well before the current CCS project and policy realities developed.
According to the IEA (2023), and Net Zero Australia (2023), CCS must play a crucial role in reaching 43% emissions reduction and Net Zero targets. In the IEA Net Zero Scenario,2 6.2 Gt of CO2 storage will be required globally by 2050. In the Net Zero Australia scenarios, Australia will require anywhere from 85 to 150 Mtpa storage capacity by 2050. This equates to between 20 and 40 new ‘major projects’,3 each of 4 Mtpa, being required by 2045 (Fig. 2).
Australia is committed to fulfilling its obligations to our Asia–Pacific energy partners, as is evident in the latest Production Gap Report (PGR) (SEI et al. 2023). The PGR noted that Australia projects a small increase in LNG production to 2035, 70% of which will be exported (SEI et al. 2023). Australian energy exports play a dual role by not only ensuring energy security for our Asia–Pacific trading partners (Bethune 2023), but also contributing significantly to their own emission reduction goals.
Our work indicates that individual project-approval time for a major CCS project in offshore Australia is currently in the range of 8–10 years (Fig. 2). This means that the nation must deliver perhaps 40 or more CCS projects in what is ~2.5 project-approval lifespans. Moreover, there is a substantial increase in the number of required operating projects from ~2030 to 2032, meaning that the existing challenge will become progressively much more difficult from that date.
In summary, CCS presents a wonderful opportunity for Australia to meet its 43% 2030, SGM and Net Zero commitments. Regulatory reform can genuinely help reduce costs, increase jobs, encourage investment and improve energy security in the Asia–Pacific.
Regulation: historical roots of the OPGGSA
Work on the OPGGSA began over 20 years ago, with the OPGGSA released in 2006; the GHG Regulations followed 5 years later, in 2011.
Policy makers were very familiar with the existing petroleum legislative framework, and used this experience in crafting the GHG legislation. There are also many commonalities in both technology and infrastructure between CCS and petroleum, and the few existing operational CCS projects at the time, such as Sleipner in offshore Norway, were developed by the parent petroleum company. As a result, a permitting system for CCS was developed in Australia that closely mirrored the offshore petroleum approach; this included the gazetting of, and competitive bidding for, acreage and the concept that the broad permitting steps are indeed equivalent between petroleum and GHG storage. For example, a petroleum exploration permit is considered similar to a GHG assessment permit, a petroleum discovery was, and still is, seen as equivalent to an eligible storage formation, a declaration of location equates directly to an identified storage formation and petroleum production licence is equivalent to a GHG injection licence (NOPTA 2023b).
However, the fundamental assumption of the equivalence between the petroleum and GHG regimes is somewhat flawed and has led to a number of potentially significant inefficiencies that could affect CCS projects in offshore Australian waters, now and in the future.
Our purpose is to identify these opportunities for improvement now, early in the current CCS project cycle, to promote discussion and provide time for appropriate action.
Comparison of the petroleum- and GHG-storage technical determinants
Table 1 presents a comparison between key technical and regulatory elements that pertain to petroleum and CCS projects. Its purpose is to break down these elements into their most fundamental components or determinants and, thereby, to identify the similarities and differences.
Petroleum project | GHG/CCS project | |
---|---|---|
Hydrocarbon ‘pools’ are where you find them; pools can be mapped out relatively accurately by using ‘real’ data, often using large numbers of appraisal and production wells, 3D seismic etc. | Storage formations are where you say they are, before, and to some extent after, CO2 injection begins; the location of a CO2 plume within the storage formation is the result of complex migration processes and its location is almost exclusively model-based, often with more limited data | |
Hydrocarbon pools are well defined (Lahee 1944; API 1969) in legislation and spatially, with their distribution able to be characterised objectively | CO2 plumes are not defined in Australian legislation and the definitions available in other jurisdictions overseas may be worse than having none (via unintended consequences) | |
Sealing: do the seals work or work well enough to trap commercial quantities of hydrocarbons? Understanding the exact trapping mechanism is not essential and, in some cases (Rankin Trend, Jablonski et al. 2013), could be unknown throughout part of or the entire life of the development project. | Containment: must demonstrate the nature of the trapping and sealing mechanisms; must demonstrate formation and well integrity; must demonstrate that the CO2 plume does not migrate out of the permit, leak or migrate out of the storage formation vertically or laterally | |
Reservoir: reservoir quality affects project economics, not project approval by regulator | Injectivity–capacity: must fully demonstrate the nature of the storage system itself, for the planned duration, rates and locations of CO2 injection | |
Impacts are typically much more local as the producing petroleum pools typically get smaller (their spatial footprint decreases) with time | Impacts: must demonstrate no deleterious impacts on other resources (discovered and undiscovered) and on the natural (especially groundwater) and man-made environments (plume gets bigger with time) |
Table 2 presents a comparison of petroleum locations and GHG storage formation, which display the primary constriction placed on CCS projects when applying equivalence to the petroleum regime.
Petroleum location | GHG storage formation | |
---|---|---|
Petroleum discovery – a notification is required if a petroleum discovery is made in a well. Notification must be given within 30 days after the completion of the well. A location can be declared if a petroleum pool/s has been identified within an exploration permit, petroleum has been recovered from a pool/s, and the identified pool/s is within the nominated block/s. | Eligible storage formation – the titleholder must inform within 30 days when there are reasonable grounds to suspect that a portion of a geological formation constitutes an ‘eligible GHG storage formation’. The GHG formation must be wholly situated in the GHG assessment permit/holding lease or injection licence area. | |
Declaration of location – a means to identify and set aside specific blocks within an exploration permit to cover a discovery. The permittee can then choose these blocks for a retention lease or production licence. | Identified storage formation – provides the mechanism to transition from a GHG assessment permit to a GHG holding lease or GHG injection licence following the identification of an eligible storage formation. | |
Timeframes – declared location comes into force for a 2-year period from the date on which it is declared, during which time a permittee may apply for a retention lease (RL) or a production licence (PL). | Timeframes – a declaration of an identified GHG storage formation retains its significance over the life of the GHG storage project, even if an injection licence is granted. | |
Variations – a declared location can be varied to add or remove blocks | Variations – a declaration of an identified GHG storage formation must be varied if any of the fundamental suitability determinants, or the spatial extent estimated in the storage formation, change at any time during the life of the GHG storage project. The declaration of GHG storage formation will form the basis of the information of Part 3 and 4 of Part B of the site plan |
Petroleum pools versus CO2 plumes
In petroleum, a petroleum pool is well defined in law (Lahee 1944; API 1969) and is categorised according to its individual structural or stratigraphic trapping condition and its pressure regime. The definition of the extent of a pool is critical, because the Australian petroleum legislation specifically uses the exact spatial extent of a pool, and its spatial relationship to other pools, to determine whether two or more pools belong to the one petroleum field. For example, if two separate pools are situated within 1000 m of each other, or they partially or completely overlie each other, then they are categorised as constituting part of the one field (Fig. 3). The spatial location and characteristics of a pool are determined at the time of discovery (Table 2), are further refined under appraisal, and are constrained by a large amount of data. The spatial context is critical because, under the OPGGSA, a petroleum location (within an exploration permit) is awarded on the basis of the intersection of the spatial location of a pool with the relevant graticular blocks; hydrocarbons must also have been recovered from the pool and characterised (Table 2).
A schematic example of the distinction between (a) ‘pools’ and (b) ‘fields’ in relation to hydrocarbon accumulations.
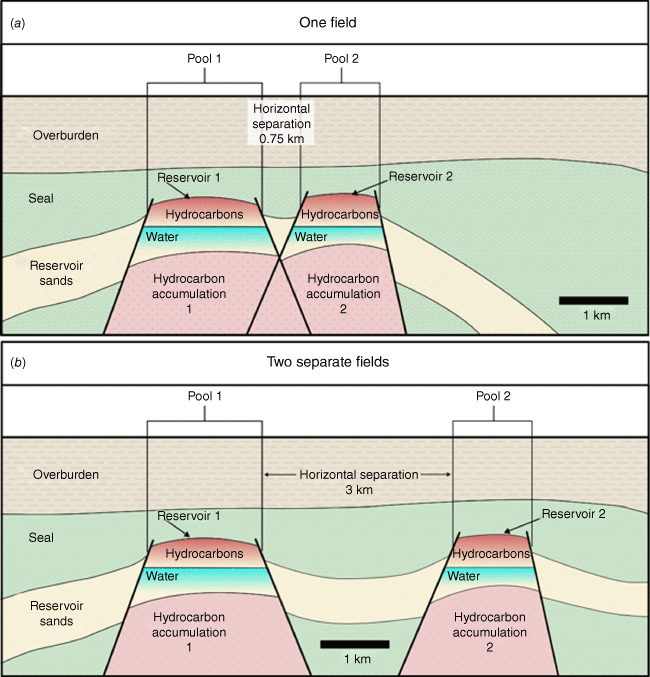
With a hydrocarbon pool, the pool location is strictly defined in law and is technically well constrained (by seismic and drilling) from the beginning of a project. In contrast, the CO2 ‘plume’ in a CCS project is, using the definitions from the Australian GHG legislation (OPGGSA 2006), effectively situated where the proponent says it is and it goes where the proponent says it goes. This is mostly based on modelling through time, complemented by measurement, monitoring and verification (MMV) activities. Moreover, there is no definition of a CO2 ‘plume’ in the Australian legislation, in spite of the fact that the location of the plume, and demonstrating that the operator can fully constrain the location of the plume through time, is critical. Because the CO2 plume is not defined, the spatial relationships of an individual plume, or indeed the relationship between adjacent, abutting, or overlying plumes (using the petroleum analogy above), cannot be established. In our opinion, definitions of what defines a plume, and especially the absolute outer edges of a CO2 plume, need to be developed within legislation to ensure that project proponents can develop unambiguous MMV programs.
The ambiguity surrounding the definition of a plume is not limited to Australian legislation. Article 3, paragraph 15 of the European CCS Directive characterises a ‘CO2 plume’ as the ‘dispersing volume of CO2 in the geological formation’ (European Commission 2009). Title 40, part 146.81(d) of the Code of Federal Regulations, which governs Class VI wells in the United States, describes a CO2 plume as the ‘extent underground, in three dimensions, of an injected carbon dioxide stream’ (EPA 2023). Additionally, the Alberta Energy Regulator outlines two distinct types of CO2 plumes; the ‘fluid plume’ (p. 23) is ‘defined as the ‘free phase CO2 directed into the aquifer’, whereas the ‘pressure plume’ (p. 12) is defined as the ‘spatial footprint of the maximum connected pore volume in the sequestration complex affected by an increase in pore pressure’ (Alberta Energy Regulator 2023). In the SPE CO2 Storage Resources Management System (SRMS) guidelines, the concept of a ‘containment area’ (p. 43) is discussed (SPE 2022). This includes the extent of free-phase CO2, the pressure effect resulting from injection and the area of formation water displaced by the injection activities.
In summary, a specific, fit-for-purpose definition of what constitutes a CO2 plume, including the edge of a plume, is required and the existing overseas definitions do not seem either fully appropriate or comprehensive. Clarification around the concept of a ‘field of plumes’, especially where several CO2 plumes might be adjacent, abut or overlap, may be helpful.
Sealing versus containment
In petroleum exploration and development, an understanding of the nature of the sealing mechanism is desirable for the operator but is not required by the regulator; the sealing mechanism in many fields is poorly or not understood but the project operates successfully. In contrast, GHG proponents must demonstrate that they understand the sealing and trapping mechanism for their proposed CCS play, that the migrating CO2 plume does not migrate out of the identified storage formation (either laterally, vertically or into deeper formations), and that the CO2 plume does not leave the permit. Should the plume migrate outside the permit, the project could be potentially effectively shut down, even in the case where it stays within a geological formation equivalent to the identified storage formation, has no leakage risk and will cause no negative impacts. This narrow interpretation of containment imposes limitations on CCS projects that simply do not exist within petroleum development activities. For example, should a petroleum pool be found to be larger than first thought, and perhaps extend into an adjacent licence, opportunities exist for the respective parties to share this joint resource via processes such as the addition of graticular blocks to a declared location, or through unitisation and combination (certificates); an example of this is the Perseus Field on the Rankin Trend, Carnarvon Basin, which was shown to be in communication with the adjacent Athena Field. By analogy to the CCS arena, the capacity to expand GHG permits, and to enter into arrangements, including cross-permit plume migration with flanking storage holders, and project/plume unitisation and combination, and allow plume interactions from separate permit holders within basins, would increase project flexibility.
Reservoir versus injectivity–capacity
In petroleum, reservoir quality is seen as more of a commercial rather than regulatory risk. However, within the GHG regulations, the project proponent must know, prior to submitting an application for a Declaration of an Identified Storage Formation (DoISF), how much CO2 will be injected, at what rate, where and for how long (injectivity–capacity; Table 1). Critically, it is expected that these factors will be largely reflected in the ultimate application for an injection licence (IL) and a site plan; this requires a very high level of understanding of future plume-migration behaviour, even though the project, especially in the case of a saline reservoir trapping play, may, as-yet, have limited data compared with an equivalent petroleum project. This is in sharp contrast to, and sets much higher requirements than in, the petroleum case, wherein to gain a petroleum location, the proponent has to demonstrate only that petroleum has been recovered from the pool and that the distribution of the pool is known. No development planning is required; this comes at the subsequent retention lease (RL) and production licence (PL)/field development plan stages (NOPTA 2023a). These requirements could have the effect of delaying the application by a proponent for a DoISF until effectively all of the data supporting the IL and site plan are available.
Impacts on petroleum and CCS
Given that the spatial extent of an originally identified hydrocarbon pool typically gets smaller as it is produced, the direct impacts that the pool has on its surroundings usually decrease progressively from the start of production onward. A GHG project must be able to demonstrate that it has no impacts on the natural and man-made environment through time and that it does not sterilise other resources, such as petroleum and potable groundwater. This is also within the context of a migrating CO2 plume the extent of which increases through time. While the requirement to minimise impacts is entirely reasonable, it is quite a different situation to petroleum.
In summary, fundamental differences in definition and application exist between the essential technical determinants that apply in petroleum and CCS projects. We consider that the strict spatial and other definitions that apply to petroleum pools are missing for CO2 plumes in the GHG legislation and that this may contribute to confusion in the future.
Worked examples
The following discussion explores how the ambiguity around technical determinants, and the issues arising from permit equivalence, affect real-world CCS projects in Australia. Fig. 4 provides examples of different storage-project scenarios, each of which are derived from potential projects around Australia.
Schematic examples of different types of storage-project scenarios around Australia. (a) An existing PL utilises a depleted field for CO2 storage. (b) A potential injection licence could simply add graticular blocks (blue polygon) immediately adjacent to the existing PL to expand the spatial extent of the permit and therefore the potential storage formation. This would ensure better utilisation of available storage space and better configuration of injection and monitoring wells. (c) A large GHG assessment permit encompassing a large proportion of the basin, as well as the existing petroleum PL. (d) The map view of the cross section shown in Fig. 5 displays the spatial extent of a potential storage formation across the boundary of an existing petroleum PL.
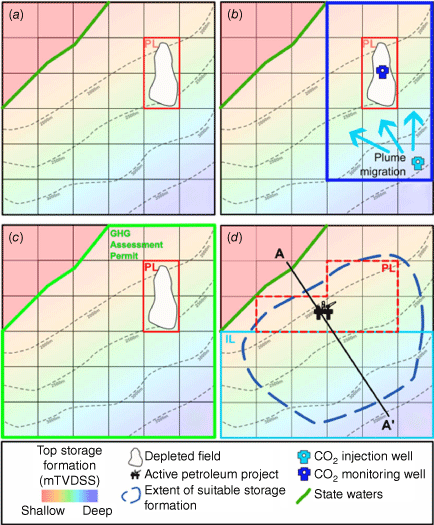
Depleted fields
An existing late-life PL holder must decide whether to convert their existing permit to an IL (Fig. 4a). This step will drastically limit the potential storage volumes and the ultimate extent of the CO2 plume (effectively to the existing PL’s extent, at a maximum if there is no mechanism to expand). The operator already has a vast amount of knowledge derived from years of petroleum production and so is extremely well- and best-placed to apply immediately for an identified storage formation and then move rapidly to the injection phase. However, because PLs are small, relative to an ideal IL, the identified storage formation must also be geographically small and lie wholly within the permit. In this scenario, an operator would use the existing facilities for both injection and monitoring. As such, injection from the top of the structure limits storage capacity from both a seal integrity-pressure and pore-space utilisation standpoint.
Saline storage formation
Another limitation of converting a PL to an IL is that it does not allow for expansion, particularly into saline formations. At some potential storage sites, there may be opportunities to utilise off-structure saline formation storage (Fig. 4b). Saline formations offer large storage capacities, making them attractive for large-scale, long-term storage of CO2. Utilising saline formations allows for the efficient use of existing infrastructure for monitoring purposes, while leveraging the larger capacities of saline formations for future expansion. This is not possible if converting an existing petroleum licence, because the title area would be too small to encompass a large saline formation. A simple solution would be to allow the addition of graticular blocks around a depleted field (blue polygon in Fig. 4b), maximising storage efficiency, and transforming previously unviable storage projects into viable ones.
Hybrid storage – depleted fields and saline formation storage
Recognising the limitations imposed in converting a petroleum title (as per Fig. 4a), and being unable to simply add graticular blocks around an existing PL (as per Fig. 4b), a project proponent may instead decide to apply for a GHG assessment permit that encompasses an area wider than the existing petroleum licence/s (Fig. 4c). The process of nominating acreage, gazettal, bidding, and assessment all prolong time to injection. Although there may be some recognition that the existing petroleum licensee (and future GHG operator) has extensive experience and a wealth of data in the immediate geological area, this does not guarantee that they will be awarded the assessment permit. As a result, they must bid on an overly bold work program to assess the GHG assessment permit or risk losing out in the bidding process. The award of the GHG acreage entails a 3-year primary work program that must be honoured, despite significant data being available to move directly to the declaration of storage and injection phases. The primary work program on the GHG assessment permit may prove to be unnecessary and could only serve to further delay the operator moving to the injection phase.
Project and permit unitisation
Another potential limitation of the existing regulations is the inability to unitise projects/permits. Under the petroleum regime, project unitisation can be agreed on between titleholders of a shared petroleum pool that extends across two (or more) title boundaries (Griffiths 2001). Similarly, in CCS operations, if a basin has multiple CCS and petroleum operators, there is potential for projects to cross permit boundaries (Fig. 4d, map view, Fig. 5, cross-section). The complexity is heightened by the lack of a clear definition for a plume in the OPGGSA, as discussed earlier. Additionally, it’s unclear how the process would unfold if a potential storage formation spanned multiple titles. Such a scenario could occur between adjacent ILs or between an IL and a PL (Fig. 5). In both scenarios, the CO2 plume could cross multiple separate licences.
A schematic representation of a geological basin with both petroleum and CCS activities. In this example, a CCS project injects CO2 into a deep saline storage formation. The plume migrates up-dip through time and eventually migrates across the permit boundary into an adjacent petroleum PL. The large regional seal isolates the CO2 plume from the overlying hydrocarbon reservoir and would therefore have no deleterious impacts on petroleum production activities. From a geological perspective, the maximum extent of the CO2 plume is limited by the edge of the regional seal (highlighted by the black arrow). However, the OPGGSA limits the maximum extent of the plume to the edge of the permit (red dashed line).
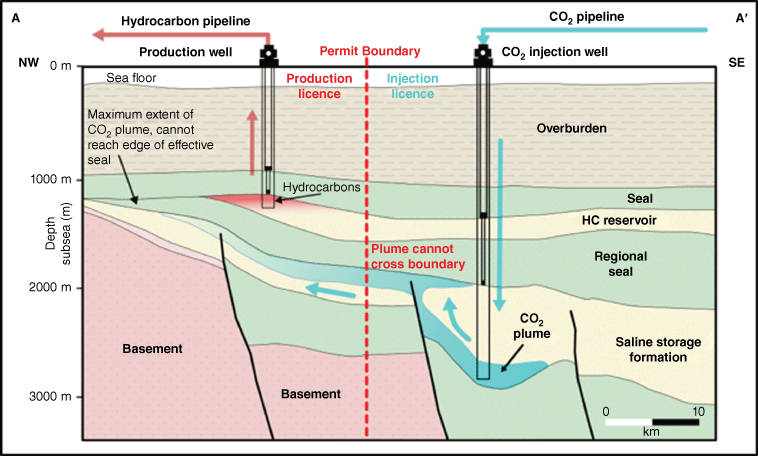
Integration and proposed actions
We have reviewed the current status of the GHG legislation in Australia and consider that there is a partial disconnect between recently developed emission policies, the legacy, near-20-year-old regulations being asked to deliver that policy and the nature, needs and timeframes of the CCS projects which are being rolled out to reduce the nation’s emissions. In an ideal world, new fit-for-purpose GHG storage regulations would have been developed and rolled-out to support major new policy initiatives such as 43% emissions reduction and Net Zero 2050. Nevertheless, improvements can be made to the existing regulatory framework, which will result in improved efficiencies, lower costs and project risks and lesser emissions, while facilitating the growth of Australia’s LNG industry within a low-emissions setting. Addressing the following items and actions will assist the delivery of the CCS project rollout within the required timeframes.
A definition for a subsurface CO2 plume needs to be developed, which is clear and unambiguous; this will make MMV compliance more transparent and increase investment certainty.
Change the definition of containment to allow CO2-plume migration outside of the existing permit, provided that no deleterious impact results. Move the focus for containment to a risk-based assessment focussed on a combination of achieving permanent CO2 removal from the atmosphere, with CCS resulting in minimal to no deleterious impact.
The ability to add graticular blocks to permits (outside of the existing permit) and to combine plumes is required, because this will remove barriers to the ongoing expansion of individual projects as storage volumes grow. The concepts of expanding petroleum locations, unitisation and combination certificates could all be applied to CCS/GHG regulations, with beneficial results.
Depleted fields are the proverbial ‘low-hanging fruit’ of the available storage options and have a wealth of existing data and technical understanding, which should favour a rapid transition to CCS. However, this option is currently limited by the inherent small size of the pre-existing petroleum licence (and location/RL/PL), which may impair their use in large-scale CCS projects. Removal of this barrier would allow the use of the surrounding CO2 migration catchments to be utilised for storage, dramatically increasing the potential storage volumes. In addition, many of the near- or now-depleted petroleum assets are facing decommissioning if a viable and rapid transition to CCS activities is not possible, which imposes a severe time constraint on the potential time windows.
Move, via the suggestions outlined above, to having smaller GHG assessment permits and injection licences, by allowing controlled migration of the subsurface CO2 plumes from these permits into the wider basin pore and pressure space.
Position the nation for a CCS success case in 2035, wherein several, large CCS projects can and will operate (and inter-operate) effectively within the same basin or sub-basin. This will require the regulator to have an informed overview of how the pore and pressure can be most efficiently used over time.
Conclusions
The Federal GHG storage legislation was examined to understand the potential interactions between the technical requirements in the legislation, the overarching emissions-policy framework and the existing and likely future technical capabilities of the CCS industry. Overwhelmingly, the GHG regulations are well-thought through and well-constructed and provide a robust roadmap for project proponents and operators. However, recent changes to overarching emissions policy, and the rapid response (and altered project mix) from CCS proponents to these changes, have highlighted a number of bottlenecks and unintended consequences within the legislation that have the potential to mute the industry’s response, and delay projects, thereby producing suboptimal outcomes for emission reductions, energy security and jobs. In particular, there are several key inefficiencies and chokepoints within the GHG legislation that offer opportunities for substantial improvements and decreased attendant project-approval timeframes, which would significantly assist Australia in rolling out the required large number of CCS projects within the timeframe needed.
The provision of more certainty around definitions, such as what constitutes a ‘CO2 plume’, would potentially make regulatory compliance simpler, especially for MMV activities. A regulatory focus on managing CCS projects in terms of risk and impacts, by removing artificial constraints on plume migration, such as permit boundaries, could lead to greatly improved utilisation of the nation’s CO2 storage resources, both within and between projects operating within the same sub-basin or basin. Similarly, the ability to easily unitise or combine plumes and adjacent projects, to allow cross-permit migration of CO2 plumes, and to expand the areal size of a CCS permit that is operating, would all increase project and storage efficiency, especially for depleted field-based projects. Finally, a success case for the nation’s CCS project rollout (in 10–15 years) will require the regulators to manage the interactions between multiple CCS projects at a basin scale, as is done in groundwater management onshore; priority consideration should be given to the implications of this now, so that the optimal use of the nation’s pore and pressure space can be achieved.
Data availability
The data that support this study are publicly available at the sources listed in the reference list.
Conflicts of interest
The authors declare that they have no conflicts of interest. This research was conducted in the absence of any commercial or financial relationships that could be construed as a potential conflict of interest. The authors have adhered to ethical standards in the preparation of this paper, ensuring objectivity and transparency in all aspects of the research.
References
Alberta Energy Regulator (2023) Directive 065: Resources Applications for Oil and Gas Reservoirs. Available at https://static.aer.ca/prd/documents/directives/Directive065.pdf [27 July 2023]
APH (2023) ‘Australia’s climate change policy to 2021: a chronology.’ (Parliament of Australia) Available at https://parlinfo.aph.gov.au/parlInfo/download/library/prspub/9168138/upload_binary/9168138.pdf [15 May 2023]
Bethune G (2023) ‘Is Australia quietly quitting the LNG business?’ (The Oxford Institute for Energy Studies) Available at https://www.oxfordenergy.org/wpcms/wp-content/uploads/2023/06/Insight-130-Is-Australia-quietly-quitting-the-LNG-business.pdf [June 2023]
DCCEEW (2023) ‘Safeguard Mechanism.’ (Department of Climate Change, Energy the Environment and Water) Available at https://www.dcceew.gov.au/climate-change/emissions-reporting/national-greenhouse-energy-reporting-scheme/safeguard-mechanism [November 2023]
DCCEEW (2024) Safeguard Mechanism. Guidelines for setting international best practice benchmarks, January 2024. Available at https://www.dcceew.gov.au/sites/default/files/documents/international-best-practice-benchmark-guidelines.pdf
EPA (2023) Underground Injection Control Program: Criteria and Standards, Title 40 CFR 146.81 (d). Code of Federal Regulations. Available at https://www.ecfr.gov/current/title-40/part-146/section-146.81
Griffiths J (2001) Unitisation of Petroleum Fields Under the PSLA. The APPEA Journal 41, 90-94.
| Crossref | Google Scholar |
IEA (2023) ‘Energy Technologies Perspectives 2023.’ (International Energy Agency) Available at https://www.iea.org/reports/energy-technology-perspectives-2023 [January 2023]
Lahee F (1944) Classification of Exploratory Drilling and Statistics for 1943. Bulletin of the American Association of Petroleum Geologists 28, 701-721.
| Crossref | Google Scholar |
Net Zero Australia (2023) Net Zero Australia Modelling Summary Report. Available at https://www.netzeroaustralia.net.au/final-modelling-results/ [19 April 2023]
NOPTA (2023a) Field Development Plan. Available at https://www.nopta.gov.au/application-processes/development/field-development-plan.html
NOPTA (2023b) Offshore Petroleum and Greenhouse Gas Storage Titles: an introduction to the titles framework. Available at https://www.nopta.gov.au/_documents/guidelines/Introduction-to-the-offshore-petroleum-and-GHG-titles-framework.pdf [November 2023]
OECD (2023) ‘Better Regulation of the Green Transition.’ OECD Public Governance Policy Papers 40. (OECD Publishing: Paris, France) 10.1787/c91a04bc-en
SEI Climate Analytics, E3G, IISD, UNEP (2023) ‘The Production Gap: Phasing down or phasing up? Top fossil fuel producers plan even more extraction despite climate promises.’ (Stockholm Environment Institute, Climate Analytics, E3G, International Institute for Sustainable Development and United Nations Environment Programme)
Footnotes
1 The amended Safeguard Rules stipulate that the best-practice benchmark for new gas fields is zero reservoir CO2 emissions (DCCEEW 2024).
2 The IEA Net Zero scenario sets out a pathway to stabilise global average temperatures at 1.5°C above pre-industrial levels by 2050 (IEA 2023).
![]() Geoff O’Brien is the Chief Scientist for CO2CRC. Geoff has over 35 years of experience in the marine research, petroleum, and CCS sectors and has worked in and for the petroleum industry as a petroleum systems and CCS technical specialist. He has also worked for Geoscience Australia, where he was the Research Group Leader for the Marine Environment & Offshore Petroleum Group, as a research consultant at the University of Adelaide, as the Energy Geoscience Manager at Geoscience Victoria and as the Chief Geoscientist for NOPTA. Dr O’Brien has a comprehensive understanding knowledge of Australia’s sedimentary basin systems and their petroleum potential, and over the past 15 years has become increasingly involved in the CCS sector. A passion is the development of tailored and targeted CCS work programs that relate specifically to the ultimate regulatory requirements, thereby facilitating the Pathway to Storage and providing greater efficiencies for CCS project proponents. |
![]() Simone de Morton is a Techno-Regulatory Advisor at CO2CRC with 8 years of experience in the industry, holding a PhD in Geology along with a Bachelor of Science (Honours) degree. Simone has worked as a Production Geologist and Exploration Geologist at ExxonMobil Australia and as a Geologist at the National Offshore Petroleum Titles Administrator (NOPTA). At CO2CRC, Simone has combined her extensive regulatory and geological expertise to provide techno-regulatory guidance to CCS project proponents and has delivered CCS regulatory educational courses to non-technical professionals. |
![]() David Bason is a Senior Reservoir Engineer with CO2CRC in Melbourne, Victoria with 15 years of experience in the Energy industry. David is currently developing the latest research program for Stage 4 CO2 experiments at the Otway International Test Centre. David also consults to CO2Tech on various CCUS-focused projects. He holds an MPhys from the University of Manchester, UK, and an MSc in Petroleum Engineering from Heriot-Watt University in Aberdeen, UK. David has previously worked as a reservoir engineer for Woodside Energy and as a consultant with EY. |