Response surface optimised photocatalytic degradation and quantitation of repurposed COVID-19 antibiotic pollutants in wastewaters; towards greenness and whiteness perspectives
Heba T. Elbalkiny A , Ola M. El-Borady B , Sarah S. Saleh
A
B
Abstract
The consumption of repurposed antibiotics increased due to the management of COVID-19, which in turn led to their increased presence in wastewater and potential environmental effects. This change has created a greater need for their analysis and treatment in different environmental water. This work presents a safe, low-cost method for analysing and treating water samples to ensure their suitability for human and animal use.
Certain antibiotics have been repurposed for the management of infected COVID-19 cases, because of their possible effect against the virus, and treatment of co-existing bacterial infection. The consumption of these antibiotics leads to their access to sewage, industrial and hospital effluents, then to environmental waters. This creates a need for the routine analysis and treatment of water resources.
Detection and quantitation of three repurposed antibiotics: levofloxacin (LEVO), azithromycin (AZI) and ceftriaxone (CEF) were studied in different water samples using LC-MS/MS methods employing a C18 column and a mobile phase consisting of 80% acetonitrile/20% (0.1% formic acid in water) after solid phase extraction on Oasis HLB Prime cartridges. Real water samples were treated with synthesised graphitic carbon nitride (g-C3N4) to remove the three types of antibiotics from contaminated water under experimental conditions optimised by response surface methodology, using Box–Behnken experimental design.
The analytical method was validated in the concentration range of 10–5000 ng mL–1 for the three drugs. The removal percentages were found to be 92.55, 98.48 and 99.10% for LEVO, AZI and CEF, respectively, using synthesised g-C3N4.
The analytical method was used for the estimation of the three cited drugs before and after their removal. The method was assessed using ComplexGAPI as a greenness tool and the RGB 12 algorithm as a whiteness model. The method was applied for the analysis and treatment of real water samples before and after their treatment. It proved to be simple, low-cost and environmentally sustainable.
Keywords: azithromycin, Box–Behnken, ceftriaxone, ComplexGAPI, g-C3N4, levofloxacin, liquid chromatography, mass spectrometry.
Introduction
In the year 2019, the world was exposed to the first pandemic, COVID-19, and the number of infected cases exceeded 400 million by the end of 2022 (WHO Coronavirus (COVID-19) 2023). To face this plight, several approaches were adopted, such as preparing effective vaccines and inventing new molecules to treat the virus. Unfortunately, these approaches went on for some time with no immediate solution. Meanwhile, certain antibiotics were repurposed for the management of infected cases, because of their possible effect against the virus, and treatment of co-existing bacterial infection. These classes of antibiotics included fluoroquinolones, macrolides and cephalosporins (Yacouba et al. 2021; Thapa et al. 2022). Concerns about repurposed antibiotic consumption increased; accordingly, they found their way through sewage, industrial and hospital effluents into receiving environment waters (Hayden et al. 2022; Wang et al. 2022). There is an environmental risk of bioaccumulation of antibiotics to levels which would cause bacterial resistance, endocrine disturbance and genotoxic effects (Elbalkiny et al. 2020; Elbalkiny and Yehia 2022; Tantawy et al. 2023).
The selected antibiotics for this study were azithromycin (AZI), levofloxacin (LEVO) and ceftriaxone (CEF). AZI belongs to the checklist of substances to be monitored throughout the European Union in the field of water policy (Directive 2015/495/EC of 20 March 2015) as it poses a significant risk to the aquatic environment (Vella 2015). It was repurposed for patients with COVID-19 at the beginning of the pandemic due to its antiviral and immunomodulatory activity; however, AZI’s role in COVID-19 is still unclear (Parnham et al. 2014). LEVO is the first-line therapeutic agent for the management of respiratory tract infections due to its safety profile and high concentration in the lungs (Karampela and Dalamaga 2020). It continues to appear in the aquatic environment because of its insufficient metabolisation in human bodies (Felis et al. 2020). CEF is prescribed to treat COVID-19 patients because of the possible complications of bacterial superinfections which have been previously reported in the case of other viral respiratory infections (Giacomelli et al. 2021). It accumulates in water and causes ecological, environmental and health issues (Guo et al. 2015).
In the last few years, LEVO, AZI and CEF have been analysed either separately or with other antibiotics, in different types of wastewater samples by applying liquid chromatographic methods coupled with solid phase extraction (SPE) (Diwan et al. 2013; Opriş et al. 2013; Yu et al. 2016; Aydin et al. 2019; Younes et al. 2019; Serra-Compte et al. 2021; Sharma et al. 2022). AZI and LEVO were simultaneously detected in wastewater samples after SPE using liquid chromatography (LC) analysis (Yasojima et al. 2006; Golovko et al. 2014). The three drugs (LEVO, AZI and CEF) were quantified among other antimicrobials in water and livestock excreta using liquid chromatography with tandem mass spectrometry (LC-MS/MS) (Gao et al. 2016). Their presence in water could lead to the development of antibiotic resistance. As such there is great interest in their elimination. Unfortunately conventional methods are not successful for their removal in wastewater, therefore advanced methods are required, such as adsorption or an advanced oxidation process.
Different advanced methods have been reported for the removal of antibiotics from water by an adsorption process: for LEVO (Ullah et al. 2019; Li et al. 2020b; Gopal et al. 2022; El-Maraghy et al. 2023), AZI (Balarak et al. 2021; Upoma et al. 2022; Ameen et al. 2023) and CEF (Karungamye et al. 2022; Mohammadi Nezhad et al. 2023), and biological treatments (Tian et al. 2019; de Ilurdoz et al. 2022). The application of these methods is limited due to their high cost, low degradation efficiency, second-pollution and complicated technology (Jiang et al. 2012). Photocatalytic degradation is one of the most promising advanced oxidation processes (AOPs) that depends on the oxidation of the pollutants through the production of highly reactive radicals formed upon exposure of a photocatalyst to a UV or a solar/visible light source yielding, by mineralisation, less harmful products such as water, carbon dioxide and intermediates (Dewil et al. 2017). Photocatalytic degradation has the advantages of being eco-friendly, simple, economic, nontoxic, highly stabile and possesses excellent degradation efficiency (Jiang et al. 2012). Literature reviews have revealed the use of nanoparticles as photocatalysts for the removal of the three studied antibiotics using different metal nanocomposites such as silver, zinc, iron and titanium oxide nanoparticles (Shokri et al. 2016; Naraginti et al. 2019; Sayadi et al. 2019; El-Maraghy et al. 2020; Hashemi et al. 2020; Mehrdoost et al. 2021; Salesi and Nezamzadeh-Ejhieh 2022), graphene composites (Li et al. 2020a), and functionalised iron magnetic nanocatalysts (Shokri et al. 2016; Naraginti et al. 2019; Sayadi et al. 2019; El-Maraghy et al. 2020; Li et al. 2020a; Goulart et al. 2021; Gulen et al. 2021; Guo et al. 2021; Hu et al. 2021; Mehrdoost et al. 2021; Shen et al. 2021; Zhang et al. 2021; Salesi and Nezamzadeh-Ejhieh 2022; Abdullah et al. 2023), as shown in Supplementary Table S1.
One of the photocatalysts that has lately experienced a renaissance as a highly active metal-free photocatalyst is graphitic carbon nitride (g-C3N4), which renders materials nontoxic and biocompatible (Zhao et al. 2018; AttariKhasraghi et al. 2021; John and Thomas 2022). It displays significant blue photoluminescence between 430 and 550 nm at room temperature with onset of the bandgap adsorption at around 420 nm. Moreover, g-C3N4 has bandgap of 2.7 eV which make it suitable as a visible light-active photo-responsive photocatalyst with a conductive band of −1.1 eV and valence band of +1.6 eV, respectively (Ong et al. 2016). The OH· radical dot and holes are the two main reactive species in g-C3N4 that are responsible for the photocatalytic degradation of the studied drugs (Raizada et al. 2020). So, it is considered an economic, green and safe photocatalyst that requires only solar radiation as a driving force to conduct the catalytic reaction. Therefore, g-C3N4 has been used as a photocatalyst for the degradation of organic pollutants (Alias et al. 2020; Attarikhasraghi et al. 2021; John and Thomas 2022).
This study aimed to detect and quantify the repurposed antibiotics LEVO, AZI and CEF in different water samples coinciding with their reported treatment of COVID-19 infections using LC-MS/MS detection. The water samples were extracted using SPE and treated using the synthesised green and safe photocatalyst g-C3N4 under experimental conditions optimised by response surface methodology. The whole process is assessed in terms of Green Analytical Chemistry (GAC) and White Analytical Chemistry (WAC).
Experimental
Analysis methodology
The analysis was performed using a Shimadzu LC-MS/MS with a pump (model LC-20AD). The detection was performed using a triple quadrupole mass spectrometer (API 4000) equipped with an electrospray ionisation (ESI) source using multiple reaction monitoring (MRM) in positive ion mode. An autosampler was used with a sampling speed of 15 µL s–1 (Shimadzu SIL20A) and a pump (Shimadzu LC20AD). The data acquisitions were performed using Analyst 1.6.3 software. Oasis HLB Prime cartridges (500 mg/6 mL, Waters, MA, USA) were used for sample treatment.
For characterisation of g-C3N4, a JEOL JEM-2010 transmission electron microscope, a Shimadzu UV-2450 spectrophotometer, a Malvern Zetasizer Nano ZS90 analyser, a Shimadzu RF5301PC spectrofluorometer, an FTIR spectrophotometer (JASCO spectrometer), a Shimadzu XRD 6100 diffractometer, a Jeol model JSM-IT100 scanning electron microscope, and a Quantachrome TouchWin™ (ver. 1.2) surface analyser were used.
A reference standard of LEVO (99.54 ± 0.67) was kindly supplied by the Sanofi pharmaceutical company (Egypt). A reference standard of ceftriaxone sodium (99.58 ± 0.56) was kindly supplied by EIPICO pharmaceutical company (Egypt). Methanol, acetonitrile (HPLC grade), formic acid, melamine (≥ 99%), a reference standard of azithromycin dehydrate (99.78 ± 0.73), and erythromycin as an internal standard (IS) were purchased from Sigma–Aldrich, USA. Sodium hydroxide and hydrochloric acid (33%) were obtained from ADWIC (Egypt). The purity of the reference standards was checked using the official British Pharmacopoeia (BP) methods (British Pharmacopoeia Commision 2013).
The separation and identification were performed using an Agilent C18 column (4.6 mm × 50 mm, 4.6 µm particle size). The mobile phase consisted of 80% acetonitrile/20% (0.1% formic acid in water) in isocratic elution mode delivered at a flow rate of 0.8 mL min–1. The column temperature was set at 30°C and the amount of sample injected was 5 µL. A mass spectrometer (API 4000) equipped with a triple quadrupole was used and the detection was acheived using positive ion electrospray ionisation (ESI+), with air as a nebuliser gas and nitrogen as an auxiliary curtain and collision gas. The gas temperature was set at 300°C. The MRM transitions for LEVO, AZI, CEF and erythromycin (IS), and mass spectrometric parameters, are listed in Table 1.
Stock standard solutions of LEVO, AZI, CEF and erythromycin (IS) were prepared in methanol to a concentration of 100 μg mL–1. Freshly prepared working solutions for the three drugs were prepared by dilution of the stock solution using the same solvent. The calibration curves for the three drugs were prepared using concentrations of 10–500 ng mL–1 in triplicate for each drug. A calibration curve was plotted for each drug relating the prepared concentrations versus the peak area ratio of the corresponding drug to that of the IS. The three calibration curves were plotted, and regression equations were computed. Validation of the analytical method was performed as per ICH guidelines including the parameters of linearity, range, accuracy and precision, specificity, limit of quantification (LOQ) and limit of detection (LOD) (ICH Harmonisation Tripartite Guideline 2005).
In 250-mL amber glass bottles, 200 mL of water samples were collected from different locations and stored at −15°C for 10 days till extraction and analysis. These water samples were filtered through Whatman No. 41 and 0.7-µm filters prior to the preconcentration process, at the original pH (7–7.5) using an Oasis HLB Prime cartridge (500 mg/6 mL). Loading the cartridges was done at a flow rate of 1 mL min–1 using ultra-water samples for a sufficient contact time to allow targeted antibiotics to adsorb on the sorbent material. The sorbent was washed with 10 mL of water and then dried under vacuum and eluted with methanol (3 × 10 mL). The solvent was evaporated under nitrogen and the water samples were analysed according to the chromatographic conditions.
Synthesis and characterisation of graphitic carbon nitride (g-C3N4)
The pristine g-C3N4 powder was synthesised in a one-step process by thermally polymerising melamine (Svoboda et al. 2019) following the literature procedure with a simple modification: 5 g of melamine was weighed and placed in a porcelain crucible, which was then covered and heated in a muffle furnace for 4 h in an air atmosphere at 550°C with a heating rate of 2°C min. The resulting, yellow-coloured product was kept at room temperature for later investigations and use.
The morphological characteristics were analysed using a transmission electron microscope, operated at anaccelerating voltage of 200 kV, and included a Gatan digital camera (Model Erlangshen ES500). A Zeta sizer set to 25°C was used to determine the zeta-potential. The optical features of the solid sample were recorded using a spectrophotometer with 1 cm quartz cells. The g-C3N4 photoluminescence (PL) emission spectra were captured utilising a spectrofluorometer. The structural information and functional groups were identified using an FTIR spectrophotometer in the 4000–400 cm−1 range. X-Ray diffraction data were collected using a diffractometer with CuKa1 radiation (k = 1.54056 A), a 2θ range of 10–80, an operating data voltage of 40 kV, and a current of 30 mA. Energy-dispersive X-ray spectrometry (EDX) was utilised using a scanning electron microscope (SEM). The BET surface area of the sample was calculated employing a multipoint Brunauer–Emmett–Teller (BET) method using adsorption data relative pressure (P/P0) in the range of 0.05–0.9. The particle pore size distribution was measured by the Barrett–Joyner–Halenda (BJH) method using adsorption data, both methods were performed on a Quantachrome Nova instrument running TouchWin™ software (ver. 1.2).
Photocatalytic degradation experiment
The treatment of the studied antibiotics was undertaken using synthesised g-C3N4 nanosheets under a LED lamp (35 W) which produced visible light irradiation. Screening experiments were performed under variable conditions including pH ranges, initial concentrations of the three drugs, dose of g-C3N4, agitation rate, and time intervals in the dark to obtain an adsorption–desorption equilibrium, where one condition was changed while the others were kept constant. Two millilitres of each suspension was taken and centrifuged to remove the photocatalyst powders before measurement. The sample was analysed using the developed LC-MS/MS procedure. The screening experiments revealed the factors that will be included in the experimental design.
Box–Behnken experimental design (BBD), three-levels and three-factors, was employed for assigning the optimum conditions for maximising the removal of the three selected antibiotics, LEVO, AZI and CEF as dependent variables, against the dose of g-C3N4 (0.2–1.0 g L–1), pH (5–9) and time (30–90 min) as independent variables. Each variable was represented in three levels, high (+1), medium (0) and low (−1). Fifteen experimental points were performed including replications of the central points in randomised experimental order, as shown in Supplementary Table S2. ANOVA data analysis was performed to determine the lack of fit, and evaluate other parameters including the effects of quadratic, linear and variables’ interaction, where the model was verified by response surface methodology (RSM).
Optimum experimental conditions were applied to three types of water. The first type of water sample was a laboratory distilled water spiked with equal amounts of the three cited pharmaceuticals (500 ng mL–1). The second one was a real water sample free from pharmaceutical pollutants (clean real water) and spiked with equal amounts of the three cited pharmaceuticals (500 ng mL–1). The third were contaminated real water samples collected from the Nile River and industrial effluents collected from two cities named 6th of October and 10th of Ramadan. This approach allowed monitoring the degradation under realistic working conditions compared to the laboratory experiments.
Results and discussion
LC-MS/MS quantitation of water pollutants
Several mobile phase components with different ratios (methanol, acetonitrile, water and acidified water with formic acid) were trialled to elute symmetrical peaks with accepted tailing factors for the three drugs and IS. A C18 column and a mobile phase consisting of 80% acetonitrile/20% (0.1% formic acid in water) in isocratic elution mode at a flow rate of 0.8 mL min–1 gave optimum results as formic acid helped in the positive ionisation of the drugs. Regarding the mass spectrometric detector, the positive ion mode was selected for MRM analysis where the parent ions were selected at m/z 362.12 > 318.3 for LEVO, 555.2 > 396 for CEF, 749.61 > 83.0 for AZI and 734.55 > 158 for IS, as shown in Fig. 1a, b.
(a) Product ion spectra of [M + H]+ of the positive ion ESI-MS/MS spectra: LEVO 362.12 > 318.3 (m/z), AZI 749.61 > 83.0 (m/z), CEF 555.2 > 396 (m/z) and IS 734.55 > 158 (m/z); (b) multiple reaction monitoring (MRM) chromatograms of the three drugs and IS.
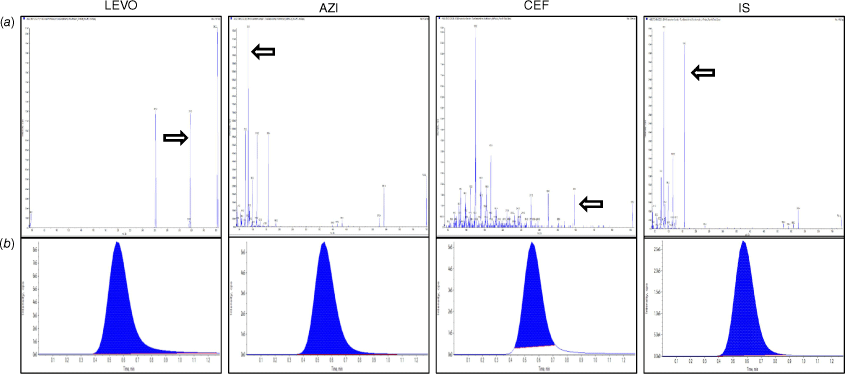
The validation parameters are presented in Table 2. The linearity range was 10–5000 ng mL–1 for AZI, CEF and LEVO as shown in Supplementary Fig. S1. The accuracy of the method was confirmed by calculating the recovery % of each drug using the corresponding regression equations. The inter- and intra-day precision scored %RSD <2 units. The method was specific as it could determine the three drugs simultaneously without interference in the laboratory prepared mixtures. The LOD of the drugs was ~3.5 ng mL–1 and LOQ was ~9 ng mL–1, which prove the high sensitivity of the method.
Parameter | LEVO | AZI | CEF | ||
---|---|---|---|---|---|
Linearity range (ng mL–1) | 10–5000 | ||||
Correlation coefficient (r) | 0.997 | 0.991 | 0.9996 | ||
Slope | 0.0009 | 0.0006 | 0.0004 | ||
Intercept | −0.0114 | 0.0676 | −0.01 | ||
s.d. of residuals | 0.099 | 0.110 | 0.018 | ||
LODA (ng mL–1) | 6.04 | 6.6 | 6.34 | ||
LOQA (ng mL–1) | 9.75 | 9.63 | 9.91 | ||
AccuracyB (Recovery % ± s.d.) | 99.83 ± 1.21 | 99.37 ± 1.25 | 99.0 ± 0.81 | ||
PrecisionC (%RSD) | Intra-day | 1.32 | 0.65 | 1.37 | |
Inter-day | 1.54 | 1.43 | 1.92 |
Oasis Prime HLB cartridges are preferred over HLB cartridges for SPE because they eliminate the conditioning step, and thus reduce solvent consumption and sample preparation time. They were also reported to have higher extraction recoveries (40%) than HLB cartridges that are reported to be commonly used for sample preconcentration of pharmaceuticals in complex environmental matrices (Elbalkiny et al. 2019; Yehia et al. 2019), even though the sorbent is the same in both cartridges (N-vinylpyrrolidone and divinylbenzene copolymer). As a result, Oasis Prime HLB cartridges at the original sample pH were chosen as they enabled a greener and better recovery of the selected drugs using a single solvent elution with pure methanol after spiking all water samples with 100 ng of each drug standard. The analysis of laboratory-spiked distilled water yielded the recovery of cartridges, and it was found to be 86.3, 83.8 and 89.1% for LEVO, AZI and CEF, respectively. The contaminated real water samples showed the presence of one or two of the cited drugs in each sample as shown in Table 3, while the clean real samples didn’t show any traces of the three drugs.
Characterisation analysis of g-C3N4
A typical transmission electron mciroscopy (TEM) image demonstrates the formation of mesoporous g-C3N4 as loose agglomerates with an irregular shape (Fig. 2a). The images also show a layered and plate-like and a worm-like porous structure (Fig. 2b) due to the substantial amounts of NH3 that may be released from the precursors which is attributed to an intrinsic catalytic property (Zhu et al. 2015). Furthermore, the selected area electron diffraction (SAED) pattern showed diffraction rings corresponding to 201 and 220 crystal planes (Fig. 2c) (Sun et al. 2022). Energy-dispersive X-ray spectroscopy (EDX) revealed the purity of the prepared sample and existence of all the anticipated elements in the photocatalyst, carbon (29.3%) and nitrogen (71.1%), as shown in Supplementary Fig. S2. The zeta (ζ) potential of g-C3N4 showed a value of −36.06 mV (Supplementary Fig. S3a), which reflects a high stability (El-Borady et al. 2021). The X-ray diffraction (XRD) pattern in Supplementary Fig. S3b displays a characteristic intense peak at a 2θ value of 27.7° (Zhu et al. 2015) which is characteristic of g-C3N4 (Liu et al. 2020). The surface features and adsorption–desorption isotherms were studied using BET and BJT as shown in Supplementary Table S3. As seen in Supplementary Fig. S3c, the adsorption–desorption isotherms were of type IV as a H3 hysteresis loop, implying the mesoporous nature of g-C3N4 which is consistent with its TEM images. The current BET surface area value is significantly greater than that previously prepared using melamine as a precursor (Yan 2012; Zhu et al. 2015). This increased surface area could be attributed to oxidation and exfoliation of g-C3N4, which are brought on by prolonged calcination and result in a more active catalyst surface, which is considered the key to the superior photocatalytic potential of the prepared g-C3N4. The structural and functional group information was demonstrated by FTIR analysis of both the melamine and the prepared g-C3N4, as in Supplementary Fig. S4, showing their characteristic spectra. The optical properties of the g-C3N4 were acquired by measuring its UV-Vis diffuse reflectance (DRS) (Zhang et al. 2016). Furthermore, the photoluminescence (PL) spectroscopy explained the photocatalytic potential as shown in Fig. 3. The PL spectrum (excitation at 325 nm) showed an emission peak around 443 nm that was ascribed to n–π∗ electronic transitions that included the lone pairs of the nitrogen atoms in g-C3N4 (Svoboda et al. 2019).
Photocatalytic degradation using g-C3N4
Based on the above characterisation results, the bandgap of g-C3N4 is relatively narrow, so it can generate electron–hole pairs under visible light irradiation. The photoexcited electrons in the conduction band (CB) of g-C3N4 can react with O2 in air to produce active superoxide radicals (·O2−), where this generated species can react with electrons to form H2O2. H2O2 then reacts with electrons to form hydroxyl radicals (OH). O2−, OH and H2O2 generated by g-C3N4 are involved in converting antibiotic pollutants into carbon dioxide and water (Dong et al. 2022), as shown in Supplementary Fig. S5.
The performance of the photocatalytic activity of g-C3N4 depends on different variables. Different pH ranges, initial concentrations of the three drugs, dose of g-C3N4, agitation rate and time intervals were studied. After the exploratory runs, it was found that the initial concentration of the studied antibiotics and the agitation rate had insignificant effects on the degradation rate. Meanwhile, the pH of the solution, dose of g-C3N4, and time intervals played a remarkable role in the photocatalytic reactions. As such, these three variables were included in the optimisation study that was designed via response surface methodology.
The quadratic statistical models were suggested with the highest order polynomial based on the experimental design results and statistical analysis performed on the three responses (highest F value and lowest P value). The model results obtained by experimental design are shown in Eqns 1–3:
where A is the pH, B is the dose of g-C3N4 in g L–1 and C is the time in minutes. The sign indicates the effect of the variable. A positive sign indicates a direct effect for B, C, AB and BC, while a negative sign indicates an inverse effect for A, AC, A2, B2 and C2.
According to the ANOVA of the model in Table 4, the F values were found to be 35.7, 26.4 and 30.5, and the P values were found to be 0.0005, 0.0011 and 0.0008 for the removal percentages of LEVO, AZI and CEF, respectively, which implies that the model is significant. The model coefficients (R2) were found to be 0.9847, 0.9794 and 0.9821, for the three responses, respectively. This indicates that the quadratic model explained 98.5, 97.9 and 98.2% of the total variances of the three responses. The R2 and adjusted R2 values were close to unity, which display the good agreement between experimental and predicted values. The values of the predicted R2 were found to be in reasonable agreement with the values of the adjusted R2, which supports the high significance of the model. High adequate precision values (>4) indicate that the model has a high signal-to-noise ratio as shown in Table 4.
Parameters | Removal % LEVO | Removal % AZI | Removal % CEF | |
---|---|---|---|---|
Model F value | 35.7 | 26.4 | 30.5 | |
Model P value | 0.0005 | 0.0011 | 0.0008 | |
R 2 | 0.9847 | 0.9794 | 0.9821 | |
R 2 (adjusted) | 0.9571 | 0.9422 | 0.9498 | |
R 2 (predicted) | 0.8805 | 0.8601 | 0.8994 | |
Adequate precision | 16.4936 | 14.0111 | 15.1717 |
The P and F values were calculated for each independent variable and its interaction. The calculated F value of the square term of pH had the largest effect on the removal percentages of the three drugs, followed by the effect of time. However, the dose of nanoparticles showed the minimum effect for the removal of the three drugs. Lack of fit was found to be insignificant for the three responses. The ANOVA of the quadratic model is shown in Supplementary Tables S4, S5 and S6.
The simultaneous effect of pH and dose of nanoparticles at constant time is shown in Fig. 4a. The pH of the reaction solution governs the surface charge on the photocatalyst. The surface of the prepared photocatalyst with pH values higher than 6.8 will be saturated by negative charges and at lower pH has a positive charge caused by H+ protons. Photodegradation efficiency was analysed at different pH values 5, 7 and 9 under visible light irradiation. LEVO and CEF contain a variety of functional groups with strongly acidic (carboxylic/sulfonic) or basic (amine/aminothiazole) properties, so they will be present in three different ionic forms, mainly depending on the pH. At acidic pH 5, they are positively charged, while AZI will exist as zwitterion in the pH range of 3.5−7.7, which explains the minimum interaction between the drug molecules and particles. The percentage removal of the three drugs LEVO, AZI and CEF increases until it reaches a maximum point at pH 7, where the molecules of the three drugs are uncharged. On this basis, it is preferable to keep the pH neutral to avoid repulsions that may cause lesser adsorption to the catalyst surface, which diminishes photodegradation efficiency (Shandilya et al. 2018). By increasing the pH of the solution to 9, AZI, CEF and LEVO molecules acquire a negative charge, which causes repulsion and a decrease in interaction with particles and thus their removal; another factor that can play a major role in low antibiotic degradation is the decomposition of hydroxyl radicals in alkaline media (Fauzi et al. 2018).
3D response surface contour plots showing the simultaneous effects on the removal % of LEVO, AZI and CEF. (a) Effects of pH and the dose of nanoparticles; (b) response surface contour plots showing the effects of pH and time; (c) response surface contour plots showing the effects of time and dose of nanoparticles.
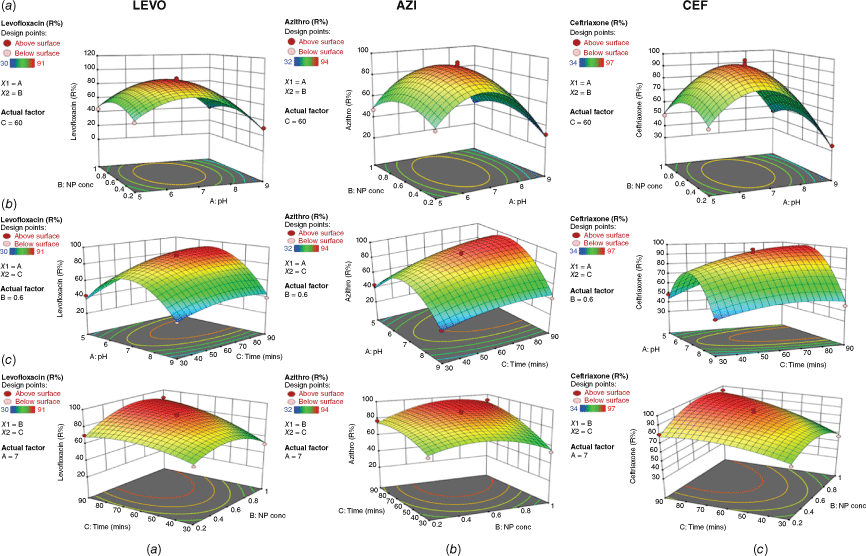
On the other hand, the removal of the three drugs is maximised at the middle level of the dose of nanoparticles (0.6 g L–1), whereas it slightly decreases at lower and higher doses as shown in Fig. 4a. Additionally, the mutual effect of pH and time at a constant dose of nanoparticles is shown in Fig. 4b, demonstrating that the removal of the three drugs increases with time at pH 7 as mentioned above. The removal % reaches its maximum at 60 min, but no further significant increase is observed against time. Fig. 4c shows the effect of time and dose of nanoparticles at constant pH which shows that the removal of the three drugs is greatly affected by increasing time (from 60 to 90 min) with a lower effect of nanoparticle dose.
Upon applying the optimum conditions, the removal percentage of the studied drugs was maximised as it reached ~97% in only 60 min, so no further modification such as element doping was required to accelerate photocatalytic performance. In addition, the higher surface area of the synthesised g-C3N4 provides more sites for interaction with the studied drugs (Zhu et al. 2015).
The validation of the RSM model was performed by measuring triplicate experiments in selected optimisation conditions as shown in Supplementary Fig. S6, where the optimised parameters obtained from the statistical analysis showing the highest desirability index (0.886) are listed in Table 5. By comparing actual percentage removal versus predicted percentage removal in the laboratory distilled water spiked with 500 ng mL–1 of each of LEVO, AZI and CEF, an error of 0.18, 1.2 and 0.46% was observed for the removal of each drug, respectively. Thus, the second-order polynomial regression equation was confirmed as an estimate of the accurate percentage of the removal from water samples.
Parameters | Optimised value | Predicted removal % | Observed removal %A | |||||
---|---|---|---|---|---|---|---|---|
LEVO | AZI | CEF | LEVO | AZI | CEF | |||
A pH | 6.66 | 94.3 | 97.3 | 99.6 | 92.6 | 98.5 | 99.1 | |
B Dose of nanoparticles (g L–1) | 0.71 | |||||||
C Time (min) | 86.40 | |||||||
Bias % | 0.18 | 1.20 | 0.46 |
The optimised conditions were applied to clean real water and compared to the results of the laboratory distilled water samples (both spiked with equal amounts of the three cited pharmaceuticals, 500 ng mL–1), and it was observed that the efficiency of photodegradation in real samples showed a slight decrease than that in distilled water, due to the matrix effect of environmental parameters such as the presence of pollutants other than our studied drug or microorganisms that might affect their removal, as shown in Fig. 5. The photodegradation of AZI showed the highest matrix effect of 6.20%, while LEVO and CEF showed similar matrix effects of 4.11 and 4.01%, respectively.
The calculated average photodegradation % of LEVO, AZI and CEF in spiked distilled water, spiked clean real water and contaminated real wastewater using g-C3N4.
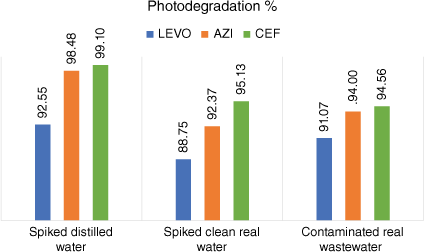
Due to the high efficiency of the process by g-C3N4 (>90%) and the low matrix effect for all drugs, the proposed photodegradation procedure was applied to real water samples containing the three cited drugs as shown in Fig. 5. The results of the analysis after photodegradation with g-C3N4 using the adopted chromatographic method are shown in Table 3, where the obtained results were found to be similar to the results of the clean real water samples.
Greenness and whiteness assessments
Complex green analytical procedure index (ComplexGAPI) was chosen for the assessment as it provides an evaluation of the entire methodology in addition to the synthesis of the proposed g-C3N4 particles. ComplexGAPI is considered a complementary tool to the previously developed GAPI metric (Płotka-Wasylka 2018; Płotka-Wasylka and Wojnowski 2021; Saleh et al. 2023). One hexagonal field was added to the original GAPI graph which evaluates several components of the pre-analysis processes and its conditions, the number of rules correlated to green economy, health and safety hazards of chemicals and instrumentation used in the synthesis process, purity of the end products, and calculation of its E factor. The original five pictograms of the GAPI tool are subdivided into smaller sub-pictograms to assess 15 aspects related to the analytical method including the type of method, the sample (collection – preservation – transport – storage – preparation), reagents, instrumentation and waste treatment (El-Naem and Saleh 2021; Kayali et al. 2023; Mannaa et al. 2023).
The ComplexGAPI showed a green profile as it scored 2 red sub-hexagons only related to the high temperature applied during synthesis of g-C3N4 which consumes a high amount energy. Only two red sub-pictograms were scored due to the high energy output of the analytical method (LC-MS/MS) and the non-treated waste of acetonitrile and formic acid with an NFPA score 2 or 3, produced from the method. Applying extraction using Oasis Prime HLB cartridges scored a yellow pictogram due to lower solvent consumption (10–100 mL) and miniaturised procedure. Moreover, the E factor was less than 0.1 because the analysis was conducted without any prior preparation, confirming the recommended method’s overall environmental friendliness as shown in Fig. 6a. The scores of the pictograms and hexagon are shown in Supplementary Table S7.
Analytical procedures are considered sustainable when they consider not just their environmental impact but also their economic cost, validity and efficiency. An effective tool for assessing the sustainability of analytical techniques is the RGB 12 algorithm (Morgan et al. 2023). According to White Analytical Chemistry (WAC), RGB 12 includes three principles (red, green and blue) of assessment. Each principle was subdivided into four parameters, for example red is subdivided into R1, R2, R3 and R4, where a score from 0–100 is assigned to each parameter, with 0 being the worst, and 100 means that the method is adapted to this parameter. The red principle (R1–R4), green principle (G1–G4) and blue principle (B1–B4) relate to analytical performance, environmental impact and economic criteria, respectively. The general accordance of the method with all three principles is expressed by an overall quantitative principle known as ‘whiteness’ (Nowak et al. 2021; Elbalkiny et al. 2022; Marzouk et al. 2023).
The proposed method showed an excellent balance between the three aspects of WAC, scoring an average white score of 80.1 as shown in Fig. 6b. It is worth mentioning that, through the performed WAC evaluation, all colours and principles were equally important, to maintain the idea of sustainability. This reflects the method’s high analytical efficiency, green profile and effective cost.
Conclusion
This study presented a simple, effective method to detect and quantify the repurposed antibiotics LEVO, AZI and CEF in different water samples coinciding with their reported treatment of COVID-19 infections which has led to their extensive use and disposal over the past few years. The quantitation was achieved using LC-MS/MS with positive ion mode for MRM detection. The water samples were extracted by SPE using Oasis HLB Prime cartridges which is considered a green option as it reduces sample preparation time and solvent consumption. This study also described the synthesis and characterisation of a modified green and safe photocatalyst g-C3N4 with increased surface area, and thus higher photocatalytic activity with no need for its doping. The g-C3N4 was used for the photocatalytic degradation of the three drugs of interest from spiked and real water samples under experimental conditions optimised by response surface methodology through Box–Behnken design. The whole procedure was assessed for its greenness and whiteness.
Data availability
The data that supports this study will be shared upon reasonable request to the corresponding author.
Conflicts of interest
The authors declare no potential conflicts of interest concerning the research, authorship and/or publication of this article.
Declaration of funding
The authors received no financial support for the research, authorship and/or publication of this article.
Author contributions
All authors contributed to the study conception and design. Material preparation, data collection and analysis were performed by Heba T. Elbalkiny, Ola M. El-Borady, Sarah S. Saleh and Christine M. El-Maraghy. The first draft of the manuscript was written equally by all authors. All authors read and approved the final manuscript.
References
Abdullah M, Iqbal J, Ur Rehman MS, Khalid U, Mateen F, Arshad SN, Al-Sehemi AG, Algarni H, Al-Hartomy OA, Fazal T (2023) Removal of ceftriaxone sodium antibiotic from pharmaceutical wastewater using an activated carbon based TiO2 composite: adsorption and photocatalytic degradation evaluation. Chemosphere 317, 137834.
| Crossref | Google Scholar | PubMed |
Alias NH, Jaafar J, Samitsu S, Ismail AF, Mohamed MA, Othman MHD, Rahman MA, Othman NH, Nor NAM, Yusof N, Aziz F (2020) Mechanistic insight of the formation of visible-light responsive nanosheet graphitic carbon nitride embedded polyacrylonitrile nanofibres for wastewater treatment. Journal of Water Process Engineering 33, 101015.
| Crossref | Google Scholar |
Ameen F, Mostafazadeh R, Hamidian Y, Erk N, Sanati AL, Karaman C, Ayati A (2023) Modeling of adsorptive removal of azithromycin from aquatic media by CoFe2O4/NiO anchored microalgae-derived nitrogen-doped porous activated carbon adsorbent and colorimetric quantifying of azithromycin in pharmaceutical products. Chemosphere 329, 138635.
| Crossref | Google Scholar | PubMed |
AttariKhasraghi N, Zare K, Mehrizad A, Modirshahla N, Behnajady MA (2021) Achieving the enhanced photocatalytic degradation of ceftriaxone sodium using CdS-g-C3N4 nanocomposite under visible light irradiation: RSM modeling and optimization. Journal of Inorganic and Organometallic Polymers and Materials 31, 3164-3174.
| Crossref | Google Scholar |
Aydin S, Aydin ME, Ulvi A, Kilic H (2019) Antibiotics in hospital effluents: occurrence, contribution to urban wastewater, removal in a wastewater treatment plant, and environmental risk assessment. Environmental Science and Pollution Research 26, 544-558.
| Crossref | Google Scholar | PubMed |
Balarak D, Mahvi AH, Shahbaksh S, Wahab MA, Abdala A (2021) Adsorptive Removal of Azithromycin Antibiotic from Aqueous Solution by Azolla Filiculoides-Based Activated Porous Carbon. Nanomaterials (Basel) 11, 3281.
| Crossref | Google Scholar | PubMed |
de Ilurdoz MS, Sadhwani JJ, Reboso JV (2022) Antibiotic removal processes from water & wastewater for the protection of the aquatic environment – a review. Journal of Water Process Engineering 45, 102474.
| Google Scholar |
Dewil R, Mantzavinos D, Poulios I, Rodrigo MA (2017) New perspectives for Advanced Oxidation Processes. Journal of Environmental Management 195, 93-99.
| Crossref | Google Scholar | PubMed |
Diwan V, Stålsby Lundborg C, Tamhankar AJ (2013) Seasonal and temporal variation in release of antibiotics in hospital wastewater: estimation using continuous and grab sampling. PLoS One 8, e68715.
| Crossref | Google Scholar | PubMed |
Dong J, Zhang Y, Hussain MI, Zhou W, Chen Y, Wang L-N (2022) g-C3N4: Properties, Pore Modifications, and Photocatalytic Applications. Nanomaterials 12, 121.
| Crossref | Google Scholar | PubMed |
Elbalkiny HT, El-Zeiny MB, Saleh SS (2022) Analysis of commonly prescribed analgesics using in-silico processing of spectroscopic signals: application to surface water and industrial effluents, and comparative study via green and white assessments. Environmental Chemistry 19, 446-459.
| Crossref | Google Scholar |
El-Borady OM, Fawzy M, Hosny M (2021) Antioxidant, anticancer and enhanced photocatalytic potentials of gold nanoparticles biosynthesized by common reed leaf extract. Applied Nanoscience 13, 3149-3160.
| Crossref | Google Scholar |
El-Maraghy CM, El-Borady OM, El-Naem OA (2020) Effective Removal of Levofloxacin from Pharmaceutical Wastewater Using Synthesized Zinc Oxid, Graphen Oxid Nanoparticles Compared with their Combination. Scientific Reports 10, 5914.
| Crossref | Google Scholar | PubMed |
El-Maraghy CM, Saleh SS, Ibrahim MS, El-Naem OA (2023) Green wastewater treatment of repurposed COVID-19 therapy (levofloxacin) using synthesized magnetite pectin nanoparticles, comparison with mesoporous silica nanoparticles. BMC Chemistry 17, 134.
| Crossref | Google Scholar | PubMed |
El-Naem OA, Saleh SS (2021) Eco-friendly UPLC-MS/MS analysis of possible add-on therapy for COVID-19 in human plasma: insights of greenness assessment. Microchemical Journal 166, 106234.
| Crossref | Google Scholar | PubMed |
Elbalkiny HT, Yehia AM (2022) Artificial networks for spectral resolution of antibiotic residues in bovine milk; solidification of floating organic droplet in dispersive liquid-liquid microextraction for sample treatment. Spectrochimica Acta Part A: Molecular and Biomolecular Spectroscopy 266, 120449.
| Crossref | Google Scholar | PubMed |
Elbalkiny HT, Yehia AM, Riad SM, Elsaharty YS (2019) Removal and tracing of cephalosporins in industrial wastewater by SPE-HPLC: optimization of adsorption kinetics on mesoporous silica nanoparticles. Journal of Analytical Science and Technology 10, 21.
| Crossref | Google Scholar |
Elbalkiny HT, Yehia AM, Riad SM, Elsaharty YS (2020) Derivative constant wavelength synchronous fluorescence spectrometry for the simultaneous detection of cefadrine and cefadroxil in water samples. Spectrochimica Acta Part A: Molecular and Biomolecular Spectroscopy 229, 117903.
| Crossref | Google Scholar | PubMed |
Fauzi AA, Jalil AA, Mohamed M, Triwahyono S, Jusoh NWC, Rahman AFA, Aziz FFA, Hassan NS, Khusnun NF, Tanaka H (2018) Altering fiber density of cockscomb-like fibrous silica–titania catalysts for enhanced photodegradation of ibuprofen. Journal of Environmental Management 227, 34-43.
| Crossref | Google Scholar | PubMed |
Felis E, Kalka J, Sochacki A, Kowalska K, Bajkacz S, Harnisz M, Korzeniewska E (2020) Antimicrobial pharmaceuticals in the aquatic environment-occurrence and environmental implications. European Journal of Pharmacology 866, 172813.
| Crossref | Google Scholar | PubMed |
Gao J, Cui Y, Tao Y, Huang L, Peng D, Xie S, Wang X, Liu Z, Chen D, Yuan Z (2016) Multiclass method for the quantification of 92 veterinary antimicrobial drugs in livestock excreta, wastewater, and surface water by liquid chromatography with tandem mass spectrometry. Journal of Separation Science 39, 4086-4095.
| Crossref | Google Scholar | PubMed |
Giacomelli A, Ridolfo AL, Oreni L, Vimercati S, Albrecht M, Cattaneo D, Rimoldi SG, Rizzardini G, Galli M, Antinori S (2021) Consumption of antibiotics at an Italian university hospital during the early months of the COVID-19 pandemic: were all antibiotic prescriptions appropriate? Pharmacological Research 164, 105403.
| Crossref | Google Scholar | PubMed |
Golovko O, Kumar V, Fedorova G, Randak T, Grabic R (2014) Seasonal changes in antibiotics, antidepressants/psychiatric drugs, antihistamines and lipid regulators in a wastewater treatment plant. Chemosphere 111, 418-426.
| Crossref | Google Scholar | PubMed |
Gopal G, Natarajan C, Mukherjee A (2022) Adsorptive removal of fluoroquinolone antibiotics using green synthesized and highly efficient Fe clay cellulose-acrylamide beads. Environmental Technology & Innovation 28, 102783.
| Crossref | Google Scholar |
Goulart LA, Moratalla A, Lanza MRV, Saez C, Rodrigo MA (2021) Photocatalytic performance of Ti/MMO/ZnO at degradation of levofloxacin: effect of pH and chloride anions. Journal of Electroanalytical Chemistry 880, 114894.
| Crossref | Google Scholar |
Gulen B, Demircivi P, Simsek EB (2021) UV-A light irradiated photocatalytic performance of hydrothermally obtained W doped BaZrO3 catalyst against the degradation of levofloxacin and tetracycline antibiotic. Journal of Photochemistry & Photobiology, A: Chemistry 404, 112869.
| Crossref | Google Scholar |
Guo X, Li D, Wan J, Yu X (2015) Preparation and electrochemical property of TiO2/Nano-graphite composite anode for electro-catalytic degradation of ceftriaxone sodium. Electrochimica Acta 180, 957-964.
| Crossref | Google Scholar |
Guo Y, Chen C, Ling L, Wang J, Qi H, Zhang B, Wu M (2021) Visible-light-driven photo-Fenton degradation of ceftriaxone sodium using SnS2/LaFeO3 composite photocatalysts. New Journal of Chemistry 45, 18933-18946.
| Crossref | Google Scholar |
Hashemi SY, Badi MY, Pasalari H, Azari A, Arfaeinia H, Kiani A (2020) Degradation of Ceftriaxone from aquatic solution using a heterogeneous and reusable O3/UV/Fe3O4@ TiO2 systems: operational factors, kinetics and mineralisation. International Journal of Environmental Analytical Chemistry 18, 6904-6920.
| Crossref | Google Scholar |
Hayden KR, Jones M, Elkin KR, Shreve MJ, Clees WI, Clark S, Mashtare ML, Veith TL, Elliott HA, Watson JE, Silverman J, Richard TL, Read AF, Preisendanz HE (2022) Impacts of the COVID‐19 pandemic on pharmaceuticals in wastewater treated for beneficial reuse: two case studies in central Pennsylvania. Journal of Environmental Quality 51, 1066-1082.
| Crossref | Google Scholar | PubMed |
Hu Z, Ge M, Guo C (2021) Efficient removal of levofloxacin from different water matrices via simultaneous adsorption and photocatalysis using a magnetic Ag3PO4/rGO/CoFe2O4 catalyst. Chemosphere 268, 128834.
| Crossref | Google Scholar | PubMed |
ICH Harmonised Tripartite Guideline (2005) Validation of Analytical Procedures: Text and Methodology Q2(R1). (International Conference of Harmonisation, Geneva, Switzerland). Available at https://database.ich.org/sites/default/files/Q2%28R1%29%20Guideline.pdf
Jiang L, Wang Y, Feng C (2012) Application of Photocatalytic Technology in Environmental Safety. Procedia Engineering 45, 993-997.
| Crossref | Google Scholar |
John A, Thomas J (2022) Enhancement of photocatalytic activity of g-C3N4 under solar light by Nd3+ doping and HPA incorporation and its application in the degradation of ceftriaxone sodium. International Journal of Environmental Analytical Chemistry 1-25.
| Crossref | Google Scholar |
Karampela I, Dalamaga M (2020) Could Respiratory Fluoroquinolones, Levofloxacin and Moxifloxacin, Prove to be Beneficial as an Adjunct Treatment in COVID-19? Archives of Medical Research 51, 741-742.
| Crossref | Google Scholar | PubMed |
Karungamye P, Rugaika A, Mtei K, Machunda R (2022) A Review of Methods for Removal of Ceftriaxone from Wastewater. Journal of Xenobiotics 12, 223-235.
| Crossref | Google Scholar | PubMed |
Kayali Z, Obaydo RH, Alhaj Sakur A (2023) Spider diagram and sustainability evaluation of UV-methods strategy for quantification of aspirin and sildenafil citrate in the presence of salicylic acid in their bulk and formulation. Heliyon 9, e15260.
| Crossref | Google Scholar |
Li C, Jin H, Hou Z, Guo Y (2020a) Study on Degradation of Azithromycin Antibiotics by Molybdenum Sulfide Graphene Oxide Composites under Visible Light. IOP Conference Series: Materials Science and Engineering 174, 012019.
| Crossref | Google Scholar |
Li J, Tao J, Ma C, Yang J, Gu T, Liu J (2020b) Carboxylated cellulose nanofiber/montmorillonite nanocomposite for the removal of levofloxacin hydrochloride antibiotic from aqueous solutions. RSC Advances 10, 42038-42053.
| Crossref | Google Scholar |
Liu R, Yang W, He G, Zheng W, Li M, Tao W, Tian M (2020) Ag-modified g-C3N4 prepared by a one-step calcination method for enhanced catalytic efficiency and stability. ACS Omega 5, 19615-19624.
| Crossref | Google Scholar | PubMed |
Mannaa IM, El Gazayerly ON, Abdelbary AA, Saleh SS, Mostafa DA (2023) Validated green spectroscopic manipulation of area under the curve (AUC) for estimation of Simvastatin: application to nano-structured lipid carriers and niosomal systems. Journal of Research in Pharmacy 27, 30-42.
| Crossref | Google Scholar |
Marzouk HM, El-Hanboushy S, Obaydo RH, Fayez YM, Abdelkawy M, Lotfy HM (2023) Sustainable chromatographic quantitation of multi-antihypertensive medications: application on diverse combinations containing hydrochlorothiazide along with LC–MS/MS profiling of potential impurities: greenness and whiteness evaluation. BMC Chemistry 17, 101.
| Crossref | Google Scholar | PubMed |
Mehrdoost A, Jalilzadeh Yengejeh R, Mohammadi MK, Babaei AA, Haghighatzadeh A (2021) Comparative Analysis of UV-assisted Removal of Azithromycin and Cefixime from Aqueous Solution Using PAC/Fe/Si/Zn Nanocomposite. Journal of Health Sciences & Surveillance System 9, 39-49.
| Crossref | Google Scholar |
Mohammadi Nezhad A, Talaiekhozani A, Mojiri A, Sonne C, Cho J, Rezania S, Vasseghian Y (2023) Photocatalytic removal of ceftriaxone from wastewater using TiO2/MgO under ultraviolet radiation. Environmental Research 229, 115915.
| Crossref | Google Scholar | PubMed |
Morgan EM, Lotfy HM, Obaydo RH, Fayez YM, Abdelkawy M, Boltia SA (2023) Whiteness and greenness assessment with efficacy evaluation of two UPLC systems applied for the quantification of cinnarizine and dimenhydrinate along with their toxic impurities. Sustainable Chemistry and Pharmacy 36, 101225.
| Crossref | Google Scholar |
Naraginti S, Yu Y-Y, Fang Z, Yong Y-C (2019) Visible light degradation of macrolide antibiotic azithromycin by novel ZrO2/Ag@ TiO2 nanorod composite: transformation pathways and toxicity evaluation. Process Safety and Environmental Protection 125, 39-49.
| Crossref | Google Scholar |
Nowak PM, Wietecha-Posłuszny R, Pawliszyn J (2021) White Analytical Chemistry: an approach to reconcile the principles of Green Analytical Chemistry and functionality. TrAC Trends in Analytical Chemistry 138, 116223.
| Crossref | Google Scholar |
Ong W-J, Tan L-L, Ng YH, Yong S-T, Chai S-P (2016) Graphitic carbon nitride (g-C3N4)-based photocatalysts for artificial photosynthesis and environmental remediation: are we a step closer to achieving sustainability? Chemical Reviews 116, 7159-7329.
| Crossref | Google Scholar | PubMed |
Opriş O, Soran M-L, Coman V, Copaciu F, Ristoiu D (2013) Determination of some frequently used antibiotics in waste waters using solid phase extraction followed by high performance liquid chromatography with diode array and mass spectrometry detection. Central European Journal of Chemistry 11, 1343-1351.
| Crossref | Google Scholar |
Parnham MJ, Erakovic Haber V, Giamarellos-Bourboulis EJ, Perletti G, Verleden GM, Vos R (2014) Azithromycin: mechanisms of action and their relevance for clinical applications. Pharmacology & Therapeutics 143, 225-245.
| Crossref | Google Scholar | PubMed |
Płotka-Wasylka J (2018) A new tool for the evaluation of the analytical procedure: Green Analytical Procedure Index. Talanta 181, 204-209.
| Crossref | Google Scholar | PubMed |
Płotka-Wasylka J, Wojnowski W (2021) Complementary green analytical procedure index (ComplexGAPI) and software. Green Chemistry 23, 8657-8665.
| Crossref | Google Scholar |
Raizada P, Sudhaik A, Singh P, Shandilya P, Thakur P, Jung H (2020) Visible light assisted photodegradation of 2, 4-dinitrophenol using Ag2CO3 loaded phosphorus and sulphur co-doped graphitic carbon nitride nanosheets in simulated wastewater. Arabian Journal of Chemistry 13, 3196-3209.
| Crossref | Google Scholar |
Saleh SS, Lotfy HM, Elbalkiny HT (2023) [In press] An integrated framework to develop an efficient valid green (EVG) HPLC method for the assessment of antimicrobial pollutants with potential threats to human health in aquatic systems. Environmental Science: Processes & Impacts
| Crossref | Google Scholar | PubMed |
Salesi S, Nezamzadeh-Ejhieh A (2022) Boosted photocatalytic effect of binary AgI/Ag2WO4 nanocatalyst: characterization and kinetics study towards ceftriaxone photodegradation. Environmental Science and Pollution Research 29, 90191-90206.
| Crossref | Google Scholar |
Sayadi MH, Sobhani S, Shekari H (2019) Photocatalytic degradation of azithromycin using GO@ Fe3O4/ZnO/SnO2 nanocomposites. Journal of Cleaner Production 232, 127-136.
| Crossref | Google Scholar |
Serra-Compte A, Pikkemaat MG, Elferink A, Almeida D, Diogène J, Campillo JA, Llorca M, Álvarez-Muñoz D, Barceló D, Rodríguez-Mozaz S (2021) Combining an effect-based methodology with chemical analysis for antibiotics determination in wastewater and receiving freshwater and marine environment. Environmental Pollution 271, 116313.
| Crossref | Google Scholar | PubMed |
Shandilya P, Mittal D, Soni M, Raizada P, Hosseini-Bandegharaei A, Saini AK, Singh P (2018) Fabrication of fluorine doped graphene and SmVO4 based dispersed and adsorptive photocatalyst for abatement of phenolic compounds from water and bacterial disinfection. Journal of Cleaner Production 203, 386-399.
| Crossref | Google Scholar |
Sharma G, Pahade P, Durgbanshi A, Carda-Broch S, Peris-Vicente J, Bose D (2022) Application of micellar liquid chromatographic method for rapid screening of ceftriaxone, metronidazole, amoxicillin, amikacin and ciprofloxacin in hospital wastewater from Sagar District, India. Total Environment Research Themes 1, 100003.
| Crossref | Google Scholar |
Shen J, Qian L, Huang J, Guo Y, Zhang Z (2021) Enhanced degradation toward Levofloxacin under visible light with S-scheme heterojunction In2O3/Ag2CO3: Internal electric field, DFT calculation and degradation mechanism. Separation and Purification Technology 275, 119239.
| Crossref | Google Scholar |
Shokri M, Isapour G, Shamsvand S, Kavousi B (2016) Photocatalytic degradation of ceftriaxone in aqueous solutions by immobilized TiO2 and ZnO nanoparticles: investigating operational parameters. Journal of Materials and Environmental Science 7, 2843-2851.
| Google Scholar |
Sun Z, Zhou Q, Yang Y, Li L, Yu M, Li H, Li A, Wang X, Jiang Y (2022) Identification and ultrasensitive photoelectrochemical detection of LncNR_040117: a biomarker of recurrent miscarriage and antiphospholipid antibody syndrome in platelet-derived microparticles. Journal of Nanobiotechnology 20, 396.
| Crossref | Google Scholar | PubMed |
Svoboda L, Škuta R, Matějka V, Dvorský R, Matýsek D, Henych J, Mančík P, Praus P (2019) Graphene oxide and graphitic carbon nitride nanocomposites assembled by electrostatic attraction forces: synthesis and characterization. Materials Chemistry and Physics 228, 228-236.
| Crossref | Google Scholar |
Tantawy MA, Yehia AM, Elbalkiny HT (2023) All-solid-state chip utilizing molecular imprinted polymer for erythromycin detection in milk samples: printed circuit board-based potentiometric system. Microchimica Acta 190, 408.
| Crossref | Google Scholar |
Thapa B, Pathak SB, Jha N, Sijapati MJ, Shankar PR (2022) Antibiotics Use in Hospitalised COVID-19 Patients in a Tertiary Care Centre: A Descriptive Cross-sectional Study. Journal of Nepal Medical Association 60, 625-630.
| Crossref | Google Scholar | PubMed |
Tian X, Shen Z, Han Z, Zhou Y (2019) The effect of extracellular polymeric substances on exogenous highly toxic compounds in biological wastewater treatment: an overview. Bioresource Technology Reports 5, 28-42.
| Crossref | Google Scholar |
Ullah A, Zahoor M, Alam S, Ullah R, Alqahtani AS, Mahmood HM (2019) Separation of Levofloxacin from Industry Effluents Using Novel Magnetic Nanocomposite and Membranes Hybrid Processes. BioMed Research International 2019, 5276841.
| Crossref | Google Scholar | PubMed |
Upoma BP, Yasmin S, Ali Shaikh MA, Jahan T, Haque MA, Moniruzzaman M, Kabir MH (2022) A Fast Adsorption of Azithromycin on Waste-Product-Derived Graphene Oxide Induced by H-Bonding and Electrostatic Interactions. ACS Omega 7, 29655-29665.
| Crossref | Google Scholar | PubMed |
Vella K (2015) Commission implementing decision (EU) 2015/495 of 20 March 2015 establishing a watch list of substances for Union-wide monitoring in the field of water policy pursuant to Directive 2008/105/EC of the European Parliament and of the Council (Position statement). Official Journal of the European Union.
Wang C, Mantilla-Calderon D, Xiong Y, Alkahtani M, Bashawri YM, Al Qarni H, Hong P-Y (2022) Investigation of Antibiotic Resistome in Hospital Wastewater during the COVID-19 Pandemic: Is the Initial Phase of the Pandemic Contributing to Antimicrobial Resistance? Environmental Science & Technology 56, 15007-15018.
| Crossref | Google Scholar | PubMed |
WHO Coronavirus (COVID-19) (2023) WHO Coronavirus (COVID-19) Dashboard. World Health Organization. Available at https://covid19.who.int/
Yacouba A, Olowo-okere A, Yunusa I (2021) Repurposing of antibiotics for clinical management of COVID-19: a narrative review. Annals of Clinical Microbiology and Antimicrobials 20, 37.
| Crossref | Google Scholar | PubMed |
Yan H (2012) Soft-templating synthesis of mesoporous graphitic carbon nitride with enhanced photocatalytic H2 evolution under visible light. Chemical Communications 48, 3430-3432.
| Crossref | Google Scholar | PubMed |
Yasojima M, Nakada N, Komori K, Suzuki Y, Tanaka H (2006) Occurrence of levofloxacin, clarithromycin and azithromycin in wastewater treatment plant in Japan. Water science and Technology 53, 227-233.
| Crossref | Google Scholar | PubMed |
Yehia AM, Elbalkiny HT, Riad SM, Elsaharty YS (2019) Chemometrics for resolving spectral data of cephalosporines and tracing their residue in waste water samples. Spectrochimica Acta Part A: Molecular and Biomolecular Spectroscopy 219, 436-443.
| Crossref | Google Scholar | PubMed |
Younes HA, Mahmoud HM, Abdelrahman MM, Nassar HF (2019) Seasonal occurrence, removal efficiency and associated ecological risk assessment of three antibiotics in a municipal wastewater treatment plant in Egypt. Environmental Nanotechnology, Monitoring & Management 12, 100239.
| Crossref | Google Scholar |
Yu X, Tang X, Zuo J, Zhang M, Chen L, Li Z (2016) Distribution and persistence of cephalosporins in cephalosporin producing wastewater using SPE and UPLC–MS/MS method. Science of the Total Environment 569–570, 23-30.
| Crossref | Google Scholar | PubMed |
Zhang W, Zhou L, Deng H (2016) Ag modified g-C3N4 composites with enhanced visible-light photocatalytic activity for diclofenac degradation. Journal of Molecular Catalysis A: Chemical 423, 270-276.
| Crossref | Google Scholar |
Zhang X, Zhang Y, Jia X, Zhang N, Xia R, Zhang X, Wang Z, Yu M (2021) In situ fabrication of a novel S-scheme heterojunction photocatalyts Bi2O3/P-C3N4 to enhance levofloxacin removal from water. Separation and Purification Technology 268, 118691.
| Crossref | Google Scholar |
Zhao Y, Liang X, Shi H, Wang Y, Ren Y, Liu E, Zhang X, Fan J, Hu X (2018) Photocatalytic activity enhanced by synergistic effects of nano-silver and ZnSe quantum dots co-loaded with bulk g-C3N4 for Ceftriaxone sodium degradation in aquatic environment. Chemical Engineering Journal 353, 56-68.
| Crossref | Google Scholar |
Zhu B, Xia P, Ho W, Yu J (2015) Isoelectric point and adsorption activity of porous g-C3N4. Applied Surface Science 344, 188-195.
| Crossref | Google Scholar |