Interference of sterile oat (Avena ludoviciana) and Mexican pricklepoppy (Argemone mexicana) in chickpea
Gulshan Mahajan
A The Centre for Crop Science, Queensland Alliance for Agriculture and Food Innovation (QAAFI), The University of Queensland, Gatton, Qld 4343, Australia.
B Punjab Agricultural University, Ludhiana, Punjab 141004, India.
C The Centre for Crop Science, Queensland Alliance for Agriculture and Food Innovation (QAAFI) and School of Agriculture and Food Sciences (SAFS), The University of Queensland, Gatton, Qld 4343, Australia.
D Department of Agronomy, Chaudhary Charan Singh Haryana Agricultural University, Hisar, Haryana 125004, India.
Abstract
Knowledge gaps exist for the interference of Avena ludoviciana and Argemone mexicana in chickpea grown in eastern Australia.
This research aimed to examine the effect of different interference levels of A. ludoviciana and A. mexicana for their potential to cause yield loss in chickpea.
Experiments were conducted in a randomised complete block design with five weed infestation levels of both weeds (none, low, medium, high, and very high) in three replications.
Infestations of A. ludoviciana and A. mexicana at 15 and 17 plants m−2 caused an 83 and 48% reduction in chickpea yield, respectively, compared with weed-free situations. Based on the regression model, a 50% yield reduction of chickpea occurred at 10 and 17 plants m−2 of A. ludoviciana and A. mexicana, respectively. Based on the modified hyperbolic model, maximum seed production of A. ludoviciana and A. mexicana in chickpea at an infestation level of 1 plant m−2 was estimated at 366 and 7800 seeds m−2, respectively. At crop harvest, seed retention of A. ludoviciana and A. mexicana was greater than 50 and 90%, respectively.
A. ludoviciana and A. mexicana infestation in chickpea caused a substantial reduction in crop yield. High seed retention in A. mexicana and A. ludoviciana in chickpea suggests the possibility to manage these two weeds through harvest weed seed control.
The information generated from this study could help in strengthening integrated weed management in chickpea.
Keywords: biology, seed production, seed retention, weed, weed biomass, weed competition, weed density, yield.
Introduction
Chickpea (Cicer arietinum L.) belongs to the Fabaceae family and is responsible for about 16% of global pulse production (Merga and Haji 2019). In eastern Australia, it is an important export-oriented crop grown in the winter season. Chickpea helps in improving system-based crop productivity as two crops (summer and winter) are grown in this region and the cultivation of chickpea in the cereal system helps in breaking the disease cycle. In addition, chickpea cultivation improves soil fertility by contributing to atmospheric nitrogen fixation (by the bacteria) in the soil (Berger et al. 2004). In Australia, the chickpea crop occupied an area of 0.5 m ha during 2020–2021 with seed production of 0.7 m t (ABARES 2021). Yield gaps for chickpea in Australia are larger compared with cereal and canola crops, and it was estimated that the average yield gap for chickpea in South Australia was as high as 50% (Mawalagedera and Brand 2022).
Weeds are a limiting factor in chickpea productivity as they utilise significant moisture in the soil profile and compete with the crop, particularly when the crop is rainfed (Whish et al. 2002; Mahajan et al. 2019). The chickpea crop, being slow-growing, short-statured, and forming an open canopy at an early stage of development, is a poor competitor with weeds (Solh and Pala 1990; Boydston et al. 2018). Yield losses in chickpea may vary from 24 to 85%, depending upon the extent of weed competition imposed by different levels of infestation and types of weed species (Tiwari et al. 2001; Tanveer et al. 2015).
In Australia, wide row spacing (50–100 cm) in chickpea is popular to utilise the soil profile moisture efficiently (Reid et al. 2004). It is opined that a wide-row planting system conserves moisture in the soil profile and provides an opportunity for cropping in dry regions (Whish et al. 2002). Therefore, weed management in chickpea is essential for the success of wide-row systems, to conserve moisture in the soil profile and for better crop growth.
Weed infestation levels in fields play an important role in determining critical crop–weed competition and weed threshold levels (Martin et al. 2014). Economic weed threshold levels in the field and the high shattering ability of weeds justify the use of weed control measures at the right time for high economic returns and for restricting further weed reinfestation in the field (Cousens 1985; Walsh et al. 2012; Mahajan and Chauhan 2021a). Growth behaviours of crops may differ with different weed species and levels of weed infestation (Manalil and Chauhan 2019; Mahajan and Chauhan 2021b); therefore, studies on weed interference in different crops are important.
Sterile oats [Avena sterilis ssp. ludoviciana (Durieu)] is an important winter season weed of eastern Australia owing to its highly competitive ability (Nugent et al. 1999). A recent study in Australia reported that 16 plants m−2 of Avena ludoviciana caused a 50% reduction in wheat (Triticum aestivum L.) yield (Mahajan and Chauhan 2021b). High infestations of A. ludoviciana in winter season crops could be due to its favourable biological attributes (Mahajan and Chauhan 2021a). For example, high seed-shattering ability, adaptation to a wide range of temperatures and water stress for germination and growth, and innate dormancy in seeds are some of the attributes that make A. ludoviciana emerge at different periods of crop seasons (Sahil et al. 2020; Mahajan and Chauhan 2021c). Emergence pattern studies indicate the potential of A. ludoviciana to emerge from March to October (autumn, winter, and spring) in eastern Australia (Mahajan and Chauhan 2021b).
Under lack of competition, A. ludoviciana could produce 2660 seeds plant−1 if it emerges at the beginning of the winter season (May) (Mahajan and Chauhan 2021a). Even late (July) cohorts of A. ludoviciana could produce 430 seeds plant−1. In wheat, it has been observed that the shattering tendency of A. ludoviciana varied from 64 to 80%, suggesting that it could cause a reinfestation in the fields (Mahajan and Chauhan 2021a, 2021b). In Australia, A. ludoviciana has evolved resistance to Group 1 and 2 herbicides (Storrie 2007). The threat of herbicide resistance appears to be greater in chickpea crops as it is mainly Group 1 and 2 herbicides that are available for post-emergent control. Therefore, assessing chickpea yield at different densities of A. ludoviciana is important for guiding suitable weed control measures at the right time.
Mexican pricklepoppy (Argemone mexicana L.), which belongs to the Papaveraceae family, is a rapidly emerging weed in eastern Australia (Manalil et al. 2017). It grows well in winter crops sown at wide spacing and its infestation in chickpea crops and fallow fields is observed in eastern Australia (GRDC 2017; Gill et al. 2021). This weed is poisonous to humans and livestock (CottonInfo 2014) and one single plant can produce more than 10 000 seeds plant−1 (CottonInfo 2014).
Knowledge of interference and the competitive behaviour of weeds in crops could strengthen integrated weed management programs (IWM) by having an understanding of their weed competitive ability (Eslami et al. 2006; Lemerle et al. 2014; Reiss et al. 2018). The competitive ability of different weeds varies with different crops (Soltani et al. 2018; Korres et al. 2019). Information on the growth behaviour, maturity, and dispersal of weeds in different crops provides an opportunity for harvest weed seed control (HWSC) and can help in strengthening IWM (Mahajan and Chauhan 2021a; Squires et al. 2021). The maturity, seed production, and seed retention behaviour of A. ludoviciana and A. mexicana may vary in chickpea at different levels of infestation (Mahajan and Chauhan 2021a). Taller weed plants relative to crops at crop harvest may provide an opportunity for HWSC through the Harrington seed destructor (Walsh et al. 2012, 2013).
Knowledge gaps exist for the interference of A.ludoviciana and A. mexicana in chickpea grown in eastern Australia. Information is needed for the effect of interference of A. ludoviciana and A. mexicana on chickpea yield under Australian conditions. Therefore, field studies were conducted in the winter seasons of 2020 and 2021 to examine the effect of interference of A. ludoviciana and A. mexicana in chickpea.
Materials and methods
Field trial and observations
A field experiment was conducted in 2020 and repeated in 2021 at the research facility of the University of Queensland, Gatton (27.5514°S and 152.3428°E). The Gatton site is situated in the subtropical climatic region of Australia and has an average annual rainfall of 728 mm (30-year climatic normal; http://www.bom.gov.au). The soil type of the experimental site was medium clay (typic chromstert, quaternary alluvium) with a pH (1:2 H2O) of 6.9 and 1.4% organic carbon (Walkley Black). Both experiments were repeated in the same field. No fertiliser was applied to the crop in both seasons. For sowing, a fine seed bed was prepared with a disc-harrow (two times) followed by a rotovator. The field remained fallow after the first chickpea crop. During fallow conditions, weeds were not allowed to produce seeds and the field was ploughed one week before the planting of the second-year chickpea crop. Chickpea (variety PBA Seamer) was planted on 7 May each year using 30 seeds m−2 at a row spacing of 35 cm with the help of a cone planter in both years. The size of each plot was 7 m2 (5.0 m × 1.4 m).
Each experiment was conducted in a randomised complete block design with five weed infestation levels (none, low, medium, high, and very high) in three replications. The corresponding densities at different A. ludoviciana infestations levels were 0, 12, 15, 23, and 27 plants m−2. These densities for A. mexicana were 0, 3, 7, 12, and 17 plants m−2. Weed density in medium and high-level plots was fixed on the basis of minimum weed density observed in plots marked with low and very high-density levels and to keep the minimum labour for weeding. Weed density for respective plots was fixed by hand-weeding. Other weeds were also removed by hand-weeding after emergence.
To ensure a uniform A. ludoviciana and A. mexicana infestation across the experimental site, seeds were mixed with sand and broadcasted before chickpea planting (6 May). A shallow tillage operation was performed to bury A. ludoviciana and A. mexicana seeds in the soil at a depth of 2–3 cm. Weed seeding rates for each plot were decided based on a germination test performed in the laboratory so that at a high weed infestation level, about 25 plants m−2 could emerge in each plot. Weed density was fixed after the emergence of weeds in the field. In the laboratory, seeds of A. mexicana exhibited dormancy, while seeds of A. ludoviciana did not exhibit dormancy. The criteria for adjusting the weed density of two species in different infestation levels were decided on the basis of the highest weed emergence in the plots that were marked with very high infestation levels. Due to the high dormancy of A. mexicana seeds, we could not achieve 25 weeds m−2 in the plots designated to the very high infestation level; therefore, weed density in different infestation levels of A. mexicana was maintained lower than A. ludoviciana. In the second year, attempts were made to create a similar weed density to the first year in each infestation level.
The crop was sprinkler-irrigated (two times; first just after sowing and second 7 days after sowing) to ensure uniform crop and weed emergence. Manual weeding was done regularly (two times per week) for the initial 45 days to remove other weeds and for maintaining the required weed density. At crop harvest (5 October), weed density and biomass were recorded by placing a quadrat (50 cm × 50 cm) at two random places in each plot. For weed biomass, plants from the quadrat area were harvested at the base level, placed in paper bags, and dried in an oven at 70°C for 72 h.
Before removing weeds from the quadrat area, the total number of weed seeds in the quadrat area was also counted and then converted into seeds m−2. For A. ludoviciana, florets (empty and full) on each panicle in the quadrat area were counted. For A. mexicana, seed capsules (empty and full) in the quadrat area were counted. From those samples, about 20 filled seed capsules were chosen for seed count and then averaged to get seeds per capsule.
Before crop harvesting, five plants of chickpea were chosen randomly from each plot (at the centre of each plot) for calculating the total number of pods and then averaged into pods per plant. For calculating seed per pod, 10 pods were chosen from each plot and then averaged to get seed per pod. One-hundred seeds were counted from the harvested samples and weighed. Crop harvesting was done with a plot harvester, and seed yield was adjusted to 12% moisture content. The crop was harvested from 5.6 m2 (4.0 m × 1.4 m).
Emergence, flowering, and maturity of chickpea, A. ludoviciana, and A. mexicana were related to growing degree-days base 5ºC {GDD5 = [(maximum daily temperature + minimum daily temperature)/2) – 5]} (Martin et al. 2014). Weather data of the Gatton location were obtained from the Bureau of Meteorology, Australia (http://www.bom.gov.au/climate/) from a station situated within 500 m of the experimental field.
The crop received a total rainfall of 115 and 213 mm in 2020 and 2021, respectively. In 2020, rainfall was relatively higher from August to September; while in 2021, crops received higher rainfall in May and July (Fig. 1). The mean monthly maximum temperature during the crop season ranged between 21.7–30.9°C and 20.5–25.9°C in 2020 and 2021, respectively (Fig. 1). In both years, the mean monthly maximum temperature was lowest in July and highest in October. The mean monthly minimum temperature during the crop season ranged between 6.8–11.6°C and 7.1–10.1°C in 2020 and 2021, respectively (Fig. 1). The mean monthly minimum temperature in September 2020 (10.4°C) was relatively higher than in September 2021 (7.4°C). Similarly, the mean monthly maximum temperature in September 2020 (27.8°C) was relatively higher than in September 2021 (23.8°C).
Statistical analyses
The data for both years in each experiment were subjected to an analysis of variance (ANOVA) using the software CPCS1, verified with GENSTAT 19th edition (VSN International, Hemel Hempstead, UK). Year × treatment interactions were modest (lowest P = 0.8) for each parameter; therefore, data were pooled over the years (a total of six replications) for further analysis (Supplementary Tables S1 and S2). Treatment means were compared at the 5% level of significance using Fisher’s protected least significant difference (l.s.d.). Data were also validated to meet the assumptions of normality and variance before analysis.
The relationship between chickpea yield (% of weed-free control) and weed density/weed biomass was estimated using a three-parameter logistic model in SigmaPlot 14.5 (Systat Software, San Jose, CA, USA):
where y is the chickpea yield as a percentage of weed-free control at weed density/weed biomass x, a is the maximum yield, x0 is the weed density/biomass required for a 50% reduction in yield and b is the slope (SigmaPlot 14.5; Systat Software, San Jose, CA, USA).
The relationship between weed density and weed biomass; and, weed density and weed seed production was estimated using a modified hyperbola model:
where y is the weed seed production at weed density x, a is the maximum weed seed production estimated at weed infestation of 1 plant m−2, and b is the slope. The fit of the selected models was determined using R2 values.
Results and discussion
The chickpea crop emerged 10 (GDD5 = 141) and 11 (GDD5 = 140) days after seeding (DAS) in 2020 and 2021, respectively. In both years, A. ludoviciana and A. mexicana emerged at 8 and 14 DAS, respectively. Flower initiation in chickpea started at 84 (GDD5 = 914) and 93 (GDD5 = 913) DAS in 2020 and 2021, respectively. A. ludoviciana flowered at 65 (GDD5 = 716) and 69 (GDD5 = 685) DAS in 2020 and 2021, respectively. A. mexicana flowered at 110 (GDD5 = 1181) and 118 (GDD5 = 1183) DAS in 2020 and 2021, respectively. The chickpea crop attained maturity at 155 (GDD5 = 1802) and 157 (GDD5 = 1604) DAS in 2020 and 2021, respectively.
At the A. ludoviciana infestation level of 12 plants m−2, weed biomass was 347 g m−2, which further increased to 478, 644, and 834 g m−2 at the infestation levels of 15, 23, and 27 plants m−2, respectively (Table 1). A. ludoviciana produced 4753 seeds m−2 at the infestation level of 12 plants m−2 (Table 1). Seed production of A. ludoviciana at the weed infestation level of 23 and 27 plants m−2 increased by 104 and 166%, respectively, when compared with the infestation level of 12 plants m−2. At chickpea maturity, seed retention of A. ludoviciana at the infestation level of 12 plants m−2 was 59% and it reduced to 45% at the infestation level of 27 plants m−2 (Table 1).
Weed infestation levels (plants m−2) | Avena ludoviciana | Chickpea | ||||||
---|---|---|---|---|---|---|---|---|
Biomass (g m−2) | Seed production (no. m−2) | Seed retention (%) | Pods (no. plant−1) | Seeds (no. pod−1) | 100-seed weight (g) | Seed yield (kg ha−1) | ||
0 | – | – | – | 29.6c | 1.8c | 21.9a | 3221c | |
12 | 347.5a | 4753a | 59b | 17.2b | 1.4b | 18.3a | 1112b | |
15 | 477.9b | 6549a | 49a | 7.7a | 1.0a | 21.2a | 538ab | |
23 | 643.8c | 9721b | 45a | 6.2a | 1.0a | 21.0a | 310a | |
27 | 833.8d | 12 660c | 49a | 5.0a | 0.9a | 14.1a | 116a | |
l.s.d. (0.05) | 97.3 | 2890 | 5 | 3.3 | 0.1 | n.s. | 619 |
Year × treatment interactions were modest (smallest P = 0.08 or >0.08); therefore, data were pooled.
n.s., non-significant.
Lowercase letters indicate differences (P ≤ 0.05) between treatments.
The seed yield of chickpea in the weed-free environment (A. ludoviciana interference trial) was 3221 kg ha−1, and it was reduced by 65, 83, 90, and 96% at the weed infestation levels of 12, 15, 23, and 27 plants m−2, respectively (Table 1). About 30 pods per plant were produced by chickpea in the weed-free environment, and the pod number per plant decreased by 42, 74, and 83% at the weed infestation levels of 12, 15, and 27 plants m−2, respectively (Table 1). Seed numbers per pod were highest (1.8 seeds pod−1) in the weed-free environment and it reduced with increased levels of weed infestation (P ≤ 0.001; Table 1). At the A. ludoviciana infestation levels of 15, 23, and 27 plants m−2, chickpea plants produced only one seed per pod. The 100-seed weight of chickpea in the weed-free environment was 22 g and it did not reduce with increased levels of weed infestation (Table 1).
The biomass of A. mexicana at the infestation level of 3 plants m−2 was 190 g m−2 and it was similar at infestation levels of 7 and 12 plants m−2 (Table 2). Biomass of A. mexicana at the infestation level of 17 plants m−2 increased by 120% (419 g m−2) compared with the density of 3 plants m−2. Seed production of A. mexicana at the infestation level of 3 plants m−2 was 22 250 seeds m−2 (Table 2), and it increased by 313 and 512% at infestation levels of 12 and 17 plants m−2, respectively. At chickpea maturity, seed retention of A. mexicana at the infestation level of 3 plants m−2 was 93% and it reduced to 91 and 90%, respectively, at weed infestation levels of 12 and 17 plants m−2 (Table 2).
Weed infestation levels (plants m−2) | Argemone mexicana | Chickpea | ||||||
---|---|---|---|---|---|---|---|---|
Biomass (g m−2) | Seed production (no. m−2) | Seed retention (%) | Pods (no. plant−1) | Seeds (no. pod−1) | 100-seed weight (g) | Seed yield (kg ha−1) | ||
0 | – | – | – | 30.2d | 1.7e | 22.0bc | 3126e | |
3 | 190.5a | 22 250a | 93c | 25.8c | 1.6 d | 21.8ab | 2851d | |
7 | 212.9ab | 58 980ab | 91b | 22.7b | 1.3c | 21.9b | 2211c | |
12 | 302.8b | 91 952b | 91b | 20.3b | 1.2b | 21.6ab | 1908b | |
17 | 419.4c | 136 090c | 90a | 16.8a | 1.0a | 21.5a | 1611a | |
l.s.d. (0.05) | 122.3 | 42 274 | 0.8 | 2.4 | 0.07 | 0.3 | 271 |
Year × treatment interactions were modest (lowest P ≥ 0.8); therefore, data were pooled.
Lowercase letters indicate differences (P≤ 0.05) between treatments.
The seed yield of chickpea in the weed-free environment (A. mexicana interference trial) was 3126 kg ha−1, and it was reduced by 9, 29, 39, and 48% at the weed infestation levels of 3, 7, 12, and 17 plants m−2, respectively (Table 2). About 30 pods per plant were found in chickpea in the weed-free plot, and the pod number per plant decreased by 14, 25, 32, and 44% at the weed (A. mexicana) infestation levels of 3, 7, 12, and 17 plants m−2, respectively (Table 2). Seed numbers per pod were the highest (1.7 seeds pod−1) in the weed-free environment and were reduced by 41% (1.0 seed pod−1) at the infestation level of 17 plants m−2. The 100-seed weight of chickpea in the weed-free environment was 22 g and it decreased by 2 and 2.3%, respectively, at infestation levels of 12 and 17 plants m−2 (Table 2).
This study reports the interference of A. ludoviciana and A. mexicana in chickpea at various infestation levels. Results demonstrated that A. ludoviciana was more competitive than A. mexicana (on the basis of regression analysis) in chickpea; as the extent of the yield reduction in relation to weed biomass was higher for A. ludoviciana compared with A. mexicana infestation (Figs 2 and 3). The faster growth of A. ludoviciana compared with A. mexicana (as evidenced by the emergence and flowering data) made A. ludoviciana more competitive against chickpea. Based on the three-parameter regression model, A.ludoviciana and A. mexicana densities corresponding to the 50% yield reduction were 10 and 17 plants m−2, respectively (Figs 2 and 3). Based on the three-parameter logistic model, A. ludoviciana and A. mexicana biomass corresponding to the 50% yield reduction were 283 and 413 g m−2, respectively (Figs 2 and 3). Further, the modified hyperbola model predicts that A. ludoviciana and A. mexicana could produce biomass of 29 and 58 g m−2, respectively, at weed density of 1 plant m−2 with chickpea (Figs 2 and 3). Similarly, 1 plant m−2 of A. ludoviciana and A. mexicana in chickpea could produce 366 and 7887 seeds m−2, respectively (Figs 2 and 3).
Chickpea yield as percent of weed-free control in response to (a) weed (Avena ludoviciana) density; and (b) weed biomass. The lines in (a) and (b) represent a logistic model fit to the data. (c) Weed biomass in response to weed density; (d) weed seed production in response to weed density. The lines in (c) and (d) represent a hyperbolic model fit to the data. Error bars represent standard error (±).
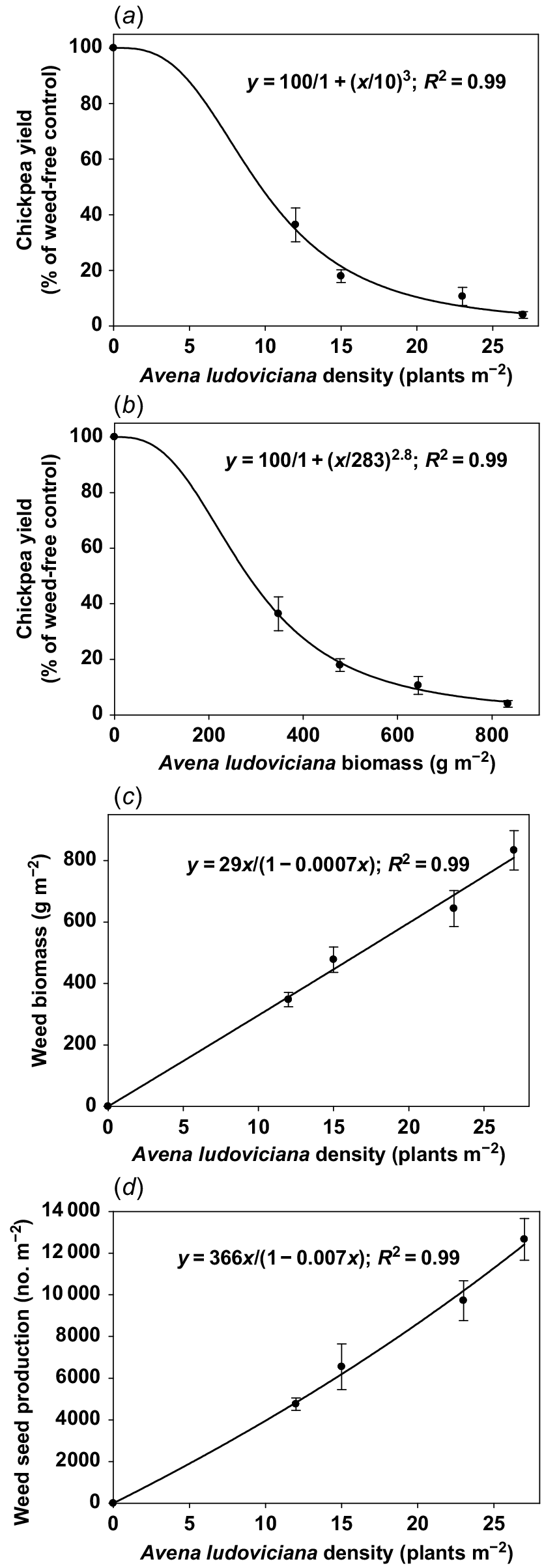
Chickpea yield as percent of weed-free control in response to (a) weed (Argemone mexicana) density; and (b) weed biomass. The lines in (a) and (b) represent a logistic model fit to the data. (c) Weed biomass in response to weed density; (d) weed seed production in response to weed density. The line in (c) represents a hyperbolic model fit to the data. Error bars represent standard error (±).
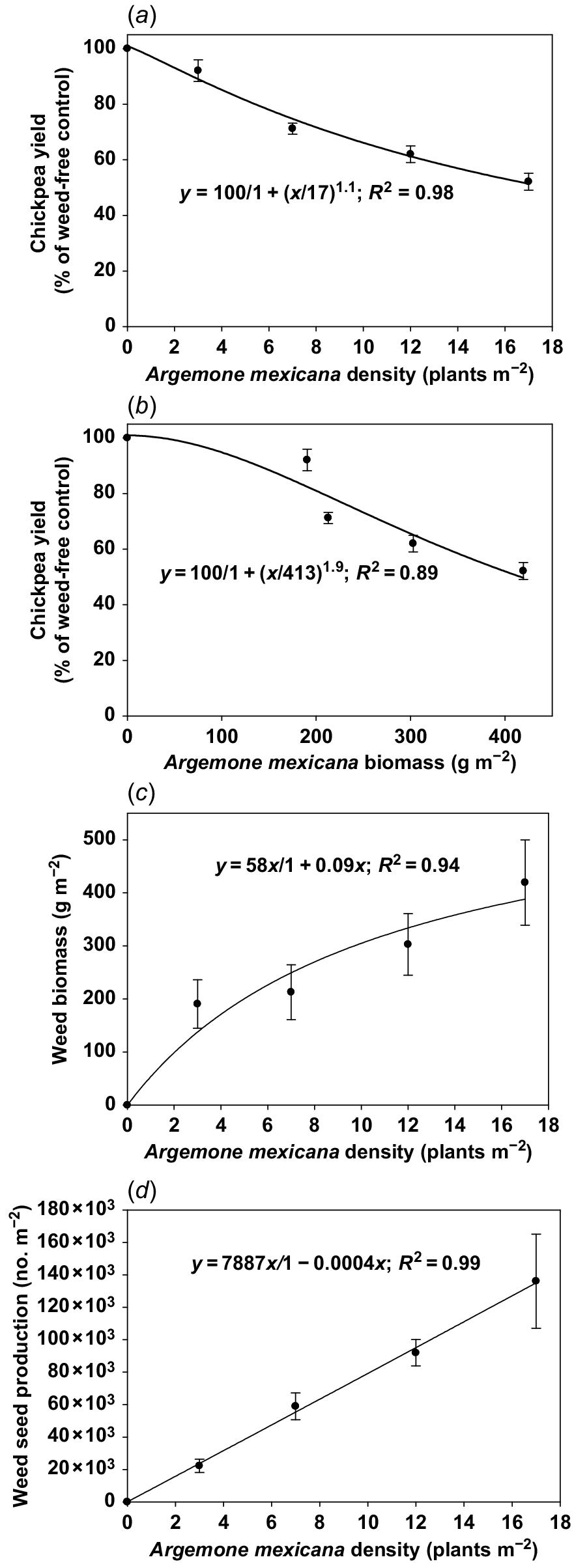
The yield reduction in chickpea due to the increased infestation of A. ludoviciana and A. mexicana could be related to high weed–crop competition, which resulted in a lower number of pods per plant, and number of seeds per pod. This suggests that early competition by A. ludoviciana and A. mexicana in chickpea may reduce pod number and late competition reduce the seeds per pod. This study also revealed that the seed retention of A. mexicana (~90%) was higher than A. ludoviciana (45–59%), suggesting a greater opportunity for HWSC for A. mexicana than A. ludoviciana.
The high level of seed production in A. ludoviciana and A. mexicana indicates the potential of these weeds to become dominant weeds. However, the greater height (Fig. 4) of these weeds (~145 cm for A. ludoviciana and ~98 cm for A. mexicana) compared with chickpea (~65 cm), and high seed retention of both weeds at chickpea harvest provides an opportunity to control these weeds through tactics such as HWSC. Almost half the seeds of A. ludoviciana were shattered on the ground at chickpea maturity. These results suggest that a short-duration variety of chickpea may provide a greater opportunity for controlling A. ludoviciana through HWSC.
Chickpea is a slow-growing crop, and if weeds are allowed to grow, they could cause a substantial reduction in yield. Various researchers have reported a reduction in chickpea yield due to the interference of weeds (Lyon and Wilson 2005; Mishra et al. 2006; Frenda et al. 2013). A previous study in Iran revealed that weed infestation in chickpea resulted in reduced dry weight of crops ranging from 50 to 60% compared with weed-free environments (Mohammadi et al. 2005). Further, these authors found that weed infestation in chickpea could cause a reduction in seed yield ranging from 44 to 66%, depending upon the level of weed infestation (Mohammadi et al. 2005). Most information on yield losses due to interference of weeds is generalised and not weed-specific. The current study filled the research gaps for assessing chickpea yield losses due to the interference of two specific weeds (A. ludoviciana and A. mexicana). Similarly, for specific weed interference in chickpea, a recent study in Australia revealed that 16 plants m−2 of A. ludoviciana in wheat could cause a 50% reduction in yield (Mahajan and Chauhan 2021b).
The results of the current study suggest that A. ludoviciana and A. mexicana tend to produce enough seeds in chickpea to allow replenishment of seedbanks in the soil, and persistence in the fields. The seeds of A. mexicana could persist in the soil for a longer period if fresh seed production, reinfestation, and dispersal are not controlled. In Australia, it was observed that at 2 and 10-cm soil depths, >30% of seeds of A. mexicana were viable after 42 months of seed burial (Manalil and Chauhan 2021). In another study, it was observed that plants of A. mexicana were unable to produce seeds when infested in wheat crop due to high competition offered by the crop (Manalil and Chauhan 2019). These results implied that the competitiveness of A. mexicana may differ with solid drilled and wide-row crops. These results suggest that crop rotation with wheat crops could be used as a weed control tactic in fields having a history of high infestation of A. mexicana. Our previous study revealed that seeds of A. ludoviciana are unable to emerge after 2 years if fresh seed production is not allowed in the field (Mahajan and Chauhan 2021b). These observations suggest that timely weed control is important to reduce A. ludoviciana reinfestation in the field.
A. ludoviciana and A. mexicana can produce multiple flushes under a wide range of climatic conditions. It was observed that multiple flushes of A. ludoviciana in eastern Australia could occur from March to October (Mahajan and Chauhan 2021b). Similarly, the infestation of A. mexicana in Australian fields was observed in summer (cotton, Gossypium hirsutum L.) as well as in winter seasons (chickpea) (Werth et al. 2013; Manalil et al. 2017). Therefore, the results of these studies suggest that A. ludoviciana and A. mexicana can infest chickpea crops under varied planting times and cause substantial yield losses, if not managed in a timely fashion. The sole reliance on chemical weed control may not provide valuable control of A. ludoviciana and A. mexicana for a long time due to the high selection pressure imposed by chemicals (Duke and Heap 2017). Therefore, timely weed control using IWM practices, such as improved crop competition, HWSC tactics, and judicious herbicide use could provide effective control of A. ludoviciana and A. mexicana in chickpeas.
In conclusion, a high infestation of A. ludoviciana and A. mexicana (Fig. 4) in chickpea fields could cause a substantial reduction in yield. Therefore, timely control of these weeds is warranted to improve chickpea yield. At chickpea maturity, seed retention of A. ludoviciana and A. mexicana was ~50 and 90%, respectively, indicating the opportunity for HWSC for these weeds in chickpea. The information generated from this study could help in guiding farmers to take suitable weed control measures at the right time and aid in strengthening the IWM program by utilising the opportunity of HWSC using the Harrington seed destructor, narrow-windrow burning, etc. The data generated from this study could also help in assessing yield losses in chickpea due to the infestation of these weeds through modelling and remote sensing.
References
ABARES (2021) Australian crop report. December. CC BY 4.0. (Australian Bureau of Agricultural and Resource Economics and Sciences: Canberra, ACT, Australia) Available at http://data.daff.gov.au/data/warehouse/aucrpd9abcc003/aucrpd9aba [Accessed 25 October 2021]
Berger JD, Turner NC, Siddique KHM, Knights EJ, Brinsmead RB, Mock I, Edmondson C, Khan TN (2004) Genotype by environment studies across Australia reveal the importance of phenology for chickpea (Cicer arietinum L.) improvement. Australian Journal of Agricultural Research 55, 1071-1084.
| Crossref | Google Scholar |
Boydston RA, Nelson H, Chaves-Cordoba B (2018) Tolerance of chickpeas to postemergence broadleaf herbicides. Weed Technology 32, 190-194.
| Crossref | Google Scholar |
Cousens R (1985) A simple model relating yield loss to weed density. Annals of Applied Biology 107, 239-252.
| Crossref | Google Scholar |
Eslami SV, Gill GS, Bellotti B, McDonald G (2006) Wild radish (Raphanus raphanistrum) interference in wheat. Weed Science 54, 749-756.
| Crossref | Google Scholar |
Frenda AS, Ruisi P, Saia S, Frangipane B, Di Miceli G, Amato G, Giambalvo D (2013) The critical period of weed control in faba bean and chickpea in Mediterranean areas. Weed Science 61, 452-459.
| Crossref | Google Scholar |
Gill G, Borger C, Chauhan B (2021) Ecology of major emerging weeds. Available at https://grdc.com.au/resources-and-publications/all-publications/publications/2021 [Accessed 9 July 2022]
GRDC (2017) Weed management in chickpeas. Grains Research and Development Corporation. Available at https://grdc.com.au/resources-and-publications/grdc-update-papers/tab-content/grdcupdate-papers/2017/03/weed-management-in-chickpeas [Accessed 20 June 2022]
Korres NE, Norsworthy JK, Mauromoustakos A (2019) Effects of Palmer amaranth (Amaranthus palmeri) establishment time and distance from the crop row on biological and phenological characteristics of the weed: implications on soybean yield. Weed Science 67, 126-135.
| Crossref | Google Scholar |
Lemerle D, Luckett DJ, Lockley P, Koetz E, Wu H (2014) Competitive ability of Australian canola (Brassica napus) genotypes for weed management. Crop & Pasture Science 65, 1300-1310.
| Crossref | Google Scholar |
Lyon DJ, Wilson RG (2005) Chemical weed control in dryland and irrigated chickpea. Weed Technology 19, 959-965.
| Crossref | Google Scholar |
Mahajan G, Chauhan BS (2021a) Biological traits of six sterile oat biotypes in response to planting time. Agronomy Journal 113, 42-51.
| Crossref | Google Scholar |
Mahajan G, Chauhan BS (2021b) Interference of wild oat (Avena fatua) and sterile oat (Avena sterilis ssp. ludoviciana) in wheat. Weed Science 69, 485-491.
| Crossref | Google Scholar |
Mahajan G, Chauhan BS (2021c) Seed longevity and seedling emergence behavior of wild oat (Avena fatua) and sterile oat (Avena sterilis ssp. ludoviciana) in response to burial depth in eastern Australia. Weed Science 69, 362-371.
| Crossref | Google Scholar |
Mahajan G, McKenzie K, Chauhan BS (2019) Influence of row spacing and cultivar selection on annual ryegrass (Lolium rigidum) control and grain yield in chickpea (Cicer arietinum). Crop & Pasture Science 70, 140-146.
| Crossref | Google Scholar |
Manalil S, Chauhan BS (2019) Interference of turnipweed (Rapistrum rugosum) and Mexican pricklepoppy (Argemone mexicana) in wheat. Weed Science 67, 666-672.
| Crossref | Google Scholar |
Manalil S, Chauhan BS (2021) Seedbank persistence and emergence pattern of Argemone mexicana, Rapistrum rugosum and Sonchus oleraceus in the eastern grain region of Australia. Scientific Reports 11, 18095.
| Crossref | Google Scholar |
Manalil S, Werth J, Jackson R, Chauhan BS, Preston C (2017) An assessment of weed flora 14 years after the introduction of glyphosate-tolerant cotton in Australia. Crop & Pasture Science 68, 773-780.
| Crossref | Google Scholar |
Mawalagedera SM, Brand JD (2022) Yield gap of winter pulses in South Eastern Australia. In ‘Proceedings of the 20th agronomy Australia conference’. Toowoomba Qld. Available at www.agronomyaustraliaproceedings.org
Merga B, Haji J (2019) Economic importance of chickpea: production, value, and world trade. Cogent Food & Agriculture 5, 1615718.
| Crossref | Google Scholar |
Mishra JS, Singh VP, Yaduraju NT (2006) Wild onion (Asphodelus tenuifolius Cav.) interference in lentil and chickpea crops and its management through competitive cropping. Weed Biology and Management 6, 151-156.
| Crossref | Google Scholar |
Mohammadi G, Javanshir A, Khooie FR, Mohammadi SA, Zehtab Salmasi S (2005) Critical period of weed interference in chickpea. Weed Research 45, 57-63.
| Crossref | Google Scholar |
Nugent T, Storrie A, Medd R (1999) Managing wild oats. (CRC for Weed Management Systems and Grains Research and Development Corporation). Available at https://archive.lls.nsw.gov.au/__data/assets/pdf_file/0004/495346/archive-wild_oats.pdf
Reiss A, Fomsgaard IS, Mathiassen SK, Stuart RM, Kudsk P (2018) Weed suppression by winter cereals: relative contribution of competition for resources and allelopathy. Chemoecology 28, 109-121.
| Crossref | Google Scholar |
Sahil , Mahajan G, Loura D, Raymont K, Chauhan BS (2020) Influence of soil moisture levels on the growth and reproductive behaviour of Avena fatua and Avena ludoviciana. PLoS ONE 15, e0234648.
| Crossref | Google Scholar |
Soltani N, Dille JA, Robinson DE, Sprague CL, Morishita DW, Lawrence NC, Kniss AR, Jha P, Felix J, Nurse RE, Sikkema PH (2018) Potential yield loss in sugar beet due to weed interference in the United States and Canada. Weed Technology 32, 749-753.
| Crossref | Google Scholar |
Squires C, Mahajan G, Walsh M, Chauhan BS (2021) Effect of planting time and row spacing on growth and seed production of junglerice (Echinochloa colona) and feather fingergrass (Chloris virgata) in sorghum. Weed Technology 35, 974-979.
| Crossref | Google Scholar |
Storrie A (2007) ‘Wild oat resistance options.’ Grains Research Update: Northern region. (Grains Research & Development Corporation) Available at http://users.tpg.com.au/icanadsl/newsletters/NL37V4.pdf
Tanveer A, Javaid MM, Irfan M, Khaliq A, Yaseen M (2015) Yield losses in chickpea with varying densities of dragon spurge (Euphorbia dracunculoides). Weed Science 63, 522-528.
| Crossref | Google Scholar |
Tiwari AN, Tiwari SN, Rathi JPS, Verma RN, Tripathi AK (2001) Crop–weed competition studies in chickpea having Asphodelus tenuifolius dominated weed community under rain fed condition. Indian Journal of Weed Science 33, 198-199.
| Google Scholar |
Walsh MJ, Harrington RB, Powles SB (2012) Harrington seed destructor: a new nonchemical weed control tool for global grain crops. Crop Science 52, 1343-1347.
| Crossref | Google Scholar |
Walsh M, Newman P, Powles S (2013) Targeting weed seeds in-crop: a new weed control paradigm for global agriculture. Weed Technology 27, 431-436.
| Crossref | Google Scholar |
Werth J, Boucher L, Thornby D, Walker S, Charles G (2013) Changes in weed species since the introduction of glyphosate-resistant cotton. Crop & Pasture Science 64, 791-798.
| Crossref | Google Scholar |
Whish JPM, Sindel BM, Jessop RS, Felton WL (2002) The effect of row spacing and weed density on yield loss of chickpea. Australian Journal of Agricultural Research 53, 1335-1340.
| Crossref | Google Scholar |