Profiling the omega-3 content of annual and perennial forages during growth and development and the relationship with other quality parameters
E. H. Clayton
A
B
C
Abstract
Omega-3 in pasture-fed beef and lamb is related to its availability in the forage species grazed. The variation in omega-3 content of several forages has been examined in the USA and UK, but not in south-eastern Australia across different stages of maturity.
This study aimed to determine the change in omega-3, at different stages of plant development, in four species of forage commonly grazed by ruminants in south-eastern Australia.
Four species, oats (Avena sativa L. cv. Eurabbie), annual ryegrass (Lolium perenne L. cv. Rambo), phalaris (Phalaris aquatica L. cv HoldfastGT) and subterranean clover (Trifolium subterrraneum L. cv. Coolamon) were grown in a replicated pot trial and harvested at seven stages of maturity corresponding to early vegetative, late vegetative, stem elongation, boot, anthesis, soft dough and ripening for oats, phalaris and ryegrass, or relative to day of flowering in clover. Proximate analysis and the concentration of fatty acids including C18:3n-3 and C18:2n-6 as well as the ratio of n-6:n-3 was determined.
The mean (±s.e.) proportion of C18:3n-3 was highest in clover (50.6 ± 0.8), and higher in ryegrass (45.1 ± 0.5) and phalaris (44.0 ± 0.6) compared with oats (39.4 ± 0.5). Omega-3 proportion decreased with increasing maturity for oats (13.9 ± 0.86 vs 64.3 ± 0.68), ryegrass (17.3 ± 0.86 vs 68.2 ± 0.65) and phalaris (21.6 ± 1.63 vs 65.1 ± 0.79) but not to the same extent for sub clover (41.5 ± 1.38 vs 57.4 ± 0.79). The proportion of omega-3 was positively correlated with crude protein content.
Omega-3 levels decreased in forages during development and was positively related to crude protein content, which is likely associated with total leaf chloroplasts. Although crude protein content remained higher for clover compared with other species, this did not translate to a higher proportion of omega-3 for any crude protein level.
Grazing forages at earlier stages of maturity and maximising the amount of crude protein available for growth will increase the amount of omega-3 in plant material. The availability of this omega-3 for incorporation into meat and milk should be examined.
Keywords: beef meat, crude protein, fatty acid, grazing management, health benefits, lamb meat, omega-3, pasture quality.
Introduction
Consumer demand for pasture-fed red meat is increasing due to a growing preference for sustainably produced, ‘clean, green’ product with greater health benefits. Red meat contains long-chain omega-3 polyunsaturated fatty acids (LCn-3PUFA, Meyer et al. 2003; Howe et al. 2007) and the consumption of red meat can contribute significantly to the overall intake of LCn-3PUFA in the Australian diet (Howe et al. 2006). Increasing the level of LCn-3PUFA in red meat may provide improved human health benefits through reducing the risk of cardiovascular disease (CVD) and other inflammatory disorders (Simopoulos 1999; Djuricic and Calder 2021).
The major factor affecting the concentration of LCn-3PUFA in ruminant animal products is the diet consumed (Scollan et al. 2001; Clayton 2014; Nogoy et al. 2022). Omega-3 is higher when sheep and cattle are fed fresh forage (Dewhurst et al. 2003, 2006; Wathes et al. 2007) or linseed oil (Kitessa et al. 2012) and lower when fed C18:2n-6 linoleic acid (LA), found predominantly in grain (for review, see Gulliver et al. 2012). The amount of LCn-3PUFA in lamb was also lower when the diet offered to animals consisted of grain compared with pasture or green feed (Ponnampalam et al. 2014).
An understanding of the level of omega-3 in different plant species and the development of pasture and grazing management practices to increase the amount of omega-3 may increase the health benefits of red meat for consumers. If the concentration of omega-3 fatty acids can be increased to levels considered a ‘source’ or ‘good source’ (FSANZ 2012), this may attract a price premium in some markets (Stampa et al. 2020; Condon 2021). This improved quality may allow producers to target specific production systems and maximise profit.
The omega-3 concentration in forages is affected by a number of factors including species, stage of growth and fertiliser application (Khan et al. 2012; Glasser et al. 2013; Clayton 2014). Omega-3 is predominantly found in leaf material (Harwood 1980) and is generally higher in young, rapidly-growing plant material (Bauchart et al. 1984; Boufaïed et al. 2003) and with higher nitrogen application (Mayland et al. 1976; Witkowska et al. 2008).
The variation in omega-3 content of several forage species have been examined in the UK (Dewhurst et al. 2006), USA (Clapham et al. 2005; Ribeiro et al. 2005) and Canada (Boufaïed et al. 2003), however, the change in omega-3 in common forage species grown in south eastern Australia across different stages of maturity has not previously been examined. Therefore, the aim of the current study was to determine the change in omega-3 concentration in four species of forages commonly grazed by ruminants in south eastern Australia, including oats (Avena sativa L. cv. Eurabbie), annual ryegrass (Lolium perenne L. cv. Rambo), phalaris (Phalaris aquatica L. cv. Holdfast GT) and subterranean clover (Trifolium subterrraneum L. cv. Coolamon) at different stages of plant development. The hypothesis of the study was that omega-3 concentration would be higher in early stages of development and lower at later stages of development and would be related to the crude protein (CP) concentration of the forage.
Materials and methods
Soil
The soil used for the study was a Red Kandosol (Isbell 1996) with moderate phosphorus concentration (Colwell P 64 mg kg−1, (Colwell 1963); Method 9b, (Rayment and Lyons 2011), and moderate cation exchange capacity (CEC, Gillman and Sumpter 1986), see Table 1) collected from the Wagga Wagga Agricultural Institute (WWAI, 35°02′51″S 147°20′17″E). Prior to commencement of the study, the field capacity (FC) of the soil was determined according to the method of Veihmeyer and Hendrickson (1931) as described previously (Twarakavi et al. 2009).
EC (dS cm−1) | pH (CaCl2) | CEC (cmol kg−1) | Exchangeable Cations (cmol(+) kg−1) | |||||||
---|---|---|---|---|---|---|---|---|---|---|
Al | Mn | Na | Ca | Mg | K | |||||
Analysis | 0.085 | 4.7 | 5.9 | 0.10 | 0.06 | 0.01 | 4.0 | 0.76 | 1.0 |
EC, electrical conductivity; CEC, cation exchange capacity.
Treatments and allocation
The study design was a 4 × 2 factorial, with four species viz; oats, (Avena sativa L. cv. Eurabbie, Nutrien Ag, Holbrook), annual ryegrass (Lolium multiflorum L. cv. Rambo, Nutrien Ag, Holbrook), phalaris (Phalaris aquatica L. cv. Holdfast GT, Barenberg) and subterranean clover (Trifolium subterraneum L. cv. Coolamon, Nutrien Ag, Holbrook) and two fertiliser treatments (mono-ammonium phosphate (MAP) only or MAP plus urea, see Table 2) with four replicates per treatment combination. The allocation of species, fertiliser and replicates to pots was achieved using the ‘R’ statistical program (ver. 4.2.0) with the DiGGer package (Coombes 2002) as described previously (Cullis et al. 2006) and employed in similar pot trials (Li et al. 2008, 2010). Four blocks within the glass house were chosen for the four replicates.
Cut | Oats | Ryegrass | Phalaris | Subterranean Clover | |
---|---|---|---|---|---|
1 | Early vegetative (Before Zadoks 20) | Early vegetative (one leaf, two tillers) | Early vegetative (two leaves, three tillers) | Day 66 | |
2 | Late vegetative (Before Zadoks 30) | Late vegetative (three leaves, four tillers) | Late vegetative (four leaves, five tillers) | Day 84 | |
3 | Stem Elongation (Approx Zadoks 32) | Early stem elongation (five leaves, seven tillers) | Early stem elongation (five leaves, six tillers) | Day 102 | |
4 | Boot (Zadoks 47) (Flag leaf sheath opening) | Late stem elongation (six leaves, 10 tillers) | Late stem elongation (six leaves, eight tillers) | Day 115 | |
5 | Anthesis (Approx Zadoks 65) (Mid Flowering) | Ear emergence (six leaves, 13 tillers) | Ear emergence (six leaves, 12 tillers) | Day 126 (Flowering) | |
6 | Soft Dough (Zadoks 85) | Anthesis | Anthesis | Day 142 (Seed set) | |
7 | Ripening (Zadoks 92) | Mature | Mature | Day 162 |
Plant material and growth conditions
Dry soil was brought to 70% FC and mixed thoroughly with MAP (10% nitrogen, 21.9% phosphorus, applied at 100 mg kg−1). Polyvinyl chloride pots (100 mm internal diameter, 300 mm height) were filled with the equivalent of 3118 g of oven-dried soil and additional water added to bring the soil to the mass calculated to be 80% of FC. The four forage species were sown at a rate of 15 seeds per pot. Clover was inoculated with an appropriate rhizobium strain (Group F, New Edge Microbial, Albury, NSW) prior to sowing. Due to the very low amount of biomass available for early harvests, the number of plants per pot was modified with stage of maturity by hand thinning, so that pots harvested at Growth Stages 1–3 had 12 plants pot−1, those harvested at Growth Stages 4–5 had 6 plants pot−1 and those harvested at Growth Stages 6–7 had 4 plants pot−1. Pots were placed in a glasshouse (maximum 33°C, minimum 9.2°C) under natural light during July–December at the WWAI. Prilled urea (50 kg ha−1) was added to pots by top-dressing 42 days after sowing when all seeds had germinated. Pots were maintained at approximately 80% field capacity by watering to weight three times per week.
Plant harvesting and preparation
Plants were harvested at seven stages of maturity according to the Zadoks scale (Zadoks et al. 1974) for oats, and stage of development in relation to vegetative or reproductive growth for phalaris and ryegrass (corresponding to early vegetative, late vegetative, stem elongation, boot, anthesis, soft dough and ripening); or relative to day of flowering in clover (Table 2). These stages of development were expected to reflect changes in quality and yield, particularly relating to potential grazing (Kaiser et al. 2007). The sub clover had not set seed at the time of the last harvest. All material from each pot was dried in an oven at 80°C for 24 h for proximate analysis or frozen and lyophilised in a freeze dryer prior to being ground through a 1 mm screen for fatty acid analysis. The total material in each pot, dry matter and water use (g water g DM−1 produced) was recorded.
Proximate analysis
Oven-dried samples were analysed for quality parameters including acid detergent fibre (ADF), neutral detergent fibre (NDF), nitrogen (N), acid insoluble ash (Ash), dry matter digestibility (DMD), digestible organic matter in the dry matter (DOMD), total lipid (ether extract, EE) and water soluble carbohydrates (WSC) by Near Infrared Reflectance (NIR) Spectroscopy with a Bruker multi-purpose analyser (MPA, Bruker Optik GmbH, Ettlingen, Germany) and OPUS software (ver. 5.1) with calibrations developed by the NSW Department of Primary Industries Feed Quality Service (NSW DPI FQS) as described previously (Packer et al. 2011). Crude protein (CP, % DM) and metabolisable energy (M/D) content (MJ kg DM−1) was calculated using the following equations (AFIA 2006):
Fatty acid analysis
Total fatty acids in freeze-dried samples were extracted and methylated using the one-step procedure of Lepage and Roy (1986) with modifications as described previously (Clayton et al. 2012). In brief, a 20 mg freeze-dried sample was added to a glass culture tube followed by 2.0 mL of methanol:toluene (4:1) containing C13:0 (10 μg mL−1) and C19:0 (10 μg mL−1) as internal standards. Methylation of the fatty acids was achieved by slowly adding 200 μL acetyl chloride while vortexing and heating at 100°C for 1 h. After methylation, samples were rapidly cooled and 5 mL of 6% K2CO3 added slowly while vortexting prior to centrifugation for 10 min at 1500g. A sub-sample of the toluene layer (approximately 250 μL) which contains the fatty acid methyl esters (FAME) was transferred into glass gas chromatography (GC) vials sealed with Teflon-lined screw-cap for subsequent analysis by GC.
Gas chromatography
Individual FAME were identified using an Agilent 7890A gas chromatograph with dual auto-injectors and dual flame ionisation detectors (FIDs). FAME were separated using two fused carbon–silica columns, coated with cyanopropylphenyl (BPX70, 30 m x 0.25 mm i.d. and 0.25 μm film thickness, SGE Analytical Science, Ringwood, Victoria, Australia, P/N 054622). The carrier gas was helium with a total flow rate of 12.4 mL min−1, a split ratio of 10:1 and a column flow of 0.9 mL min−1. The inlet temperature was 250°C, the inlet pressure was 107.8 kPa and the injection volume was 2 μL into a focus inlet liner (4 mm i.d. SGE Analytical Science, P/N 092002) that was changed at least every 150 injections.
The oven temperature was set at 150°C and held for 0.5 min, increased at 10°C min−1 to 180°C, increased at 1.5°C min−1 to 220°C and then increased at 30°C min−1 up to 260°C and maintained for 5 min to give a total run time of 36.5 min. The FID temperature was set at 280°C with the following gas flow rates; hydrogen = 35 mL min−1, instrument air = 350 mL min−1 and nitrogen make-up gas = 30 mL min−1. Sample FAME peaks were identified by comparing their retention times with those of a standard mixture of FAME (NuChek Prep, Elysian, MN USA; Sigma, St Louis, MO and Supelco, Bellefonte, PA, USA) as described previously (Clayton et al. 2012) and quantitated using Agilent Chemstation Ver. C.01 and Microsoft Excel using a three point standard curve for each FAME with C19:0 as the internal standard. Unknown fatty acids were also quantified and all results were calculated as g kg DM−1. Total fatty acid concentration was also converted to an equivalent lipid content using a lipid conversion factor (LCF) of 0.883 (Weihrauch et al. 1977). The lower limit of detection (LoD) was less than 0.02 g kg DM−1 for all FAME analysed and the intra and inter-assay coefficient of variation was 9.7 and 11.2%, respectively, for all FAME between 0.2 and 5.0% of total FAME; and 1.7 and 1.6%, respectively, for all FAME greater than 5% of total FAME.
Statistical analyses
Prior to analysis, data were assessed for assumptions of normal distribution by the evaluation of frequency histogram and quantile–quantile plots and the Kolmogorov–Smirnov goodness of fit statistic using the Univariate procedure in the SAS statistical program (SAS Institute Inc. 1997). Differences in the concentration of fatty acids between species over time were determined by repeated measures analysis using the Mixed Model procedure in SAS (SAS Institute Inc. 1997). The restricted maximum likelihood (REML) estimation used ‘Pot’ as the individual experimental unit (Littell et al. 1998). The most appropriate covariance structure for each analysis was determined by reference to the Schwarz’s Bayesian Information Criterion (BIC, Wang and Goonewardene 2004). The analysis examined the fixed effects of ‘Species’, ‘Fertiliser’ and ‘Stage of Growth’ as well as the interaction between fixed effects with ‘Range’ and ‘Row’ examined as random effects. As the fixed effect of ‘Fertiliser’ and the interaction between ‘Fertiliser’ with other fixed effects was not significant (P > 0.05) for any parameter analysed, results are presented for the fixed effects of ‘Species’ and ‘Stage of Growth’ only. The relationships between the analysis of proximate components of forage and the proportion of individual fatty acids or total fatty acid concentration were analysed by Pearson correlation also using SAS. Data are presented as least squares means ± s.e. of least squares means. An alpha of 0.05 was used for all statistical tests.
Results
Plant growth, water use and quality
The amount of dry matter harvested (g dry matter plant−1) differed between species (P < 0.001) and was higher for oats (10.35 ± 0.35 g dry matter [DM]) and ryegrass (9.68 ± 0.35 g DM) compared with phalaris (4.52 ± 0.45 g DM) and clover (3.21 ± 0.50 g DM) at the final harvest. Water use (g g DM−1) was lowest for oats compared with any other species (Table 3) and generally decreased with increasing stage of maturity for ryegrass, phalaris and clover (Fig. 1b). The water use for phalaris declined initially and then increased towards later stages of maturity.
Analysis | Mean water use and proximate analysis A | P-values | ||||||
---|---|---|---|---|---|---|---|---|
Oats | Ryegrass | Phalaris | Clover | Species | Harvest | Species × Harvest | ||
Water | ||||||||
DM (% as-is) | 21.5b (±0.40) | 21.7b (±0.40) | 26.9a (±0.42) | 16.9c (±0.80) | <0.001 | <0.001 | <0.001 | |
Water (g g DM−1) | 267.7b (±13.53) | 320.2a (±13.73) | 387.5a (±14.72) | 337.7a (±29.83) | <0.001 | <0.001 | <0.001 | |
Proximate | ||||||||
NDF (%DM) | 48.2a (±0.48) | 43.3c (±0.44) | 45.8b (±0.47) | 36.7d (±0.88) | <0.001 | <0.001 | <0.001 | |
ADF (%DM) | 26.8a (±0.20) | 24.7b (±0.18) | 23.7b (±0.20) | 21.7c (±0.39) | <0.001 | <0.001 | <0.001 | |
CP (%DM) | 11.56d (±0.41) | 15.26c (±0.40) | 16.44b (±0.42) | 23.25a (±0.94) | <0.001 | <0.001 | <0.001 | |
M/D (MJ kg DM−1) | 11.31c (±0.08) | 12.61a (±0.07) | 12.19b (±0.08) | 11.44c (±0.15) | <0.001 | <0.001 | <0.001 | |
EE (%DM) | 3.14c (±0.03) | 3.31b (±0.03) | 3.56a (±0.03) | 3.47a (±0.07) | <0.001 | <0.001 | <0.001 | |
WSC (%DM) | 19.6b (±0.77) | 23.1a (±0.75) | 21.3ab (±0.77) | 10.3c (±1.51) | <0.001 | <0.001 | <0.001 |
Values are least squares means ± s.e. of the least squares means.
NDF, neutral detergent fibre; ADF, acid detergent fibre; CP, crude protein; M/D, metabolisable energy; EE, ether extract; WSC, water soluble carbohydrates.
Mean change in (a) dry matter (DM, % as-is) and (b) water use (g g DM−1) for oats (), ryegrass (
), phalaris (
) and clover (
) over different stages of development. Values are least squares means ± s.e. of the least squares means.
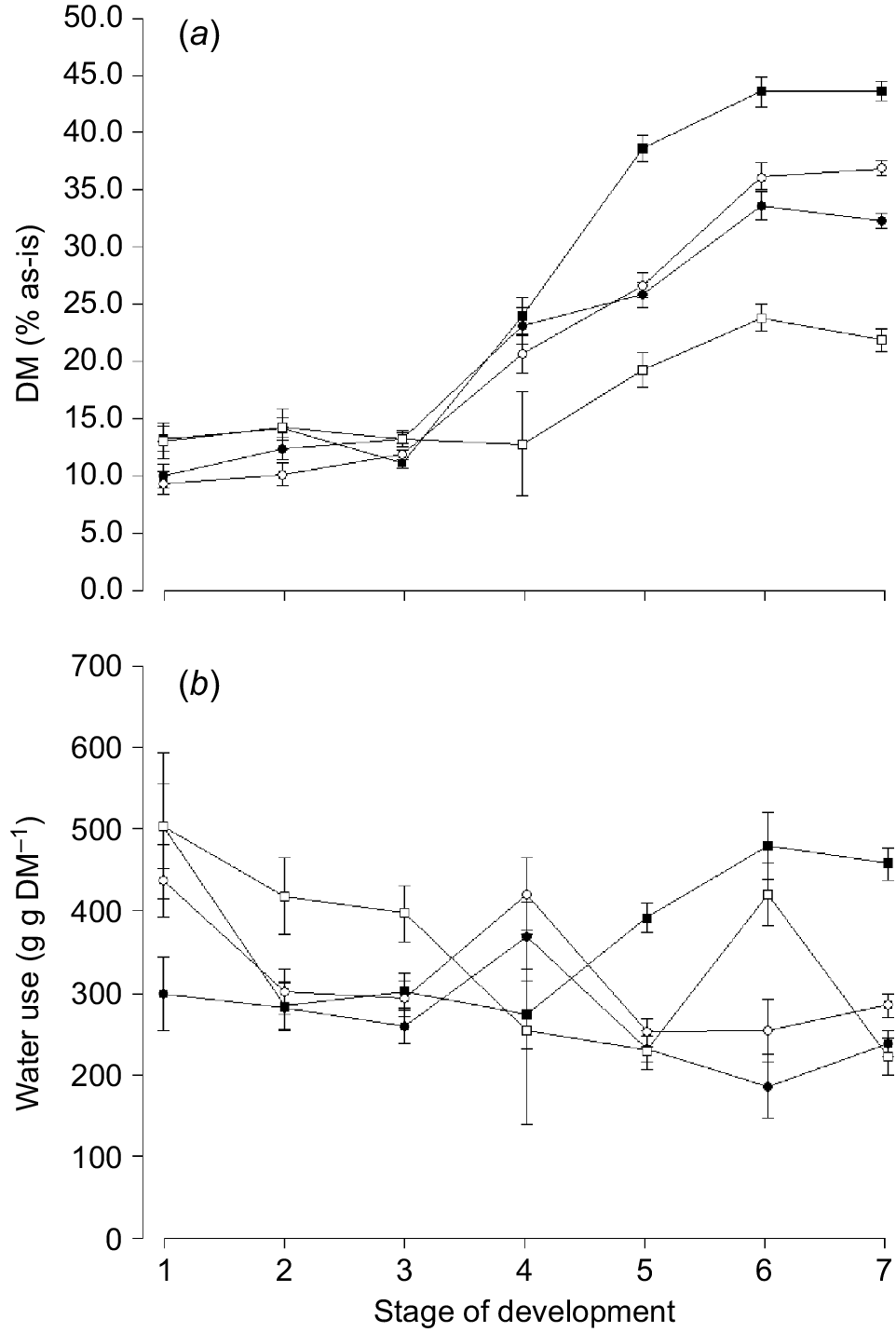
The NDF content was highest for oats compared with any other species and lowest for clover (Table 3). The CP (Fig. 2a) and EE (Fig. 2b) content declined with increasing stage of maturity for all species and CP was highest for clover compared with any other species and lowest for oats (Table 3). The EE content of clover increased from Harvest 2 to 4, however, declined again with later maturity (Fig. 2b). The M/D content of oats increased at the ‘boot stage’ (Harvest 4), however, declined again with increasing stage of maturity (Fig. 2c). The M/D content of clover remained relatively constant with increasing stages of maturity (Fig. 2c).
Mean concentration of (a) crude protein, (b) ether extract, and (c) metabolisable energy (M/D) for oats (), ryegrass (
), phalaris (
) and clover (
) over different stages of development. Values are least squares means ± s.e. of the least squares means.
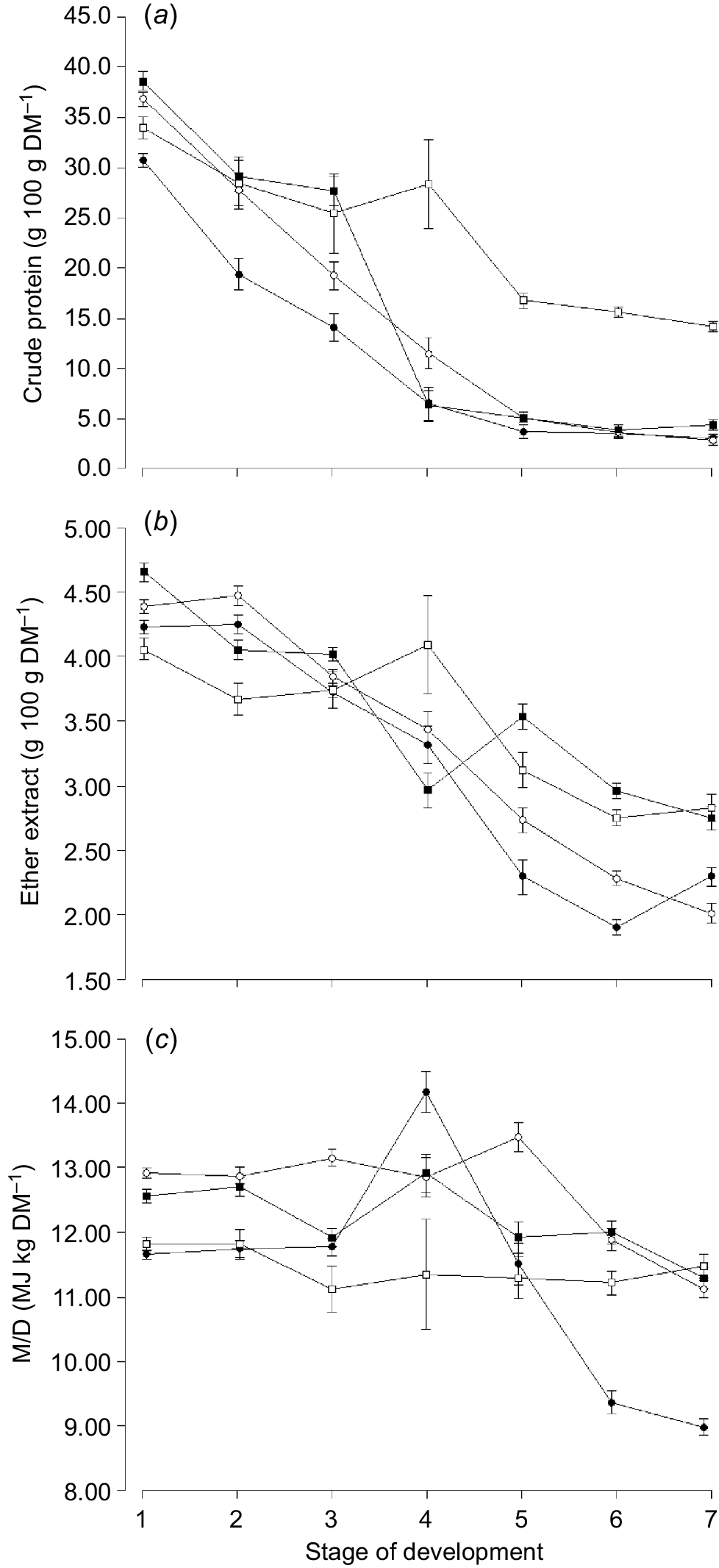
The M/D of oats, ryegrass and phalaris (M/D = −0.18 × NDF + 20.3, r2 = 0.83, P < 0.001) but not clover (M/D = −0.05 × NDF + 13.4, r2 = 0.08, P = 0.854) was negatively correlated with the NDF content of forage at any stage of development. The EE content of oats, ryegrass and phalaris (EE = 0.85 × Ln(CP) + 1.42, r2 = 0.84, P < 0.001) and clover (EE = 1.42 × Ln(CP) − 0.97, r2 = 0.84, P < 0.001) was positively correlated with the CP content of forage across different stages of development.
Fatty acid changes over time
The proportion of C18:3n-3 (i.e. cis-9, cis-12, cis-15 octadecatrienoic acid, α-linolenic acid) and total omega-3 was highest in clover compared with any other species and higher in ryegrass and phalaris compared with oats (Table 4). Similarly, the proportion of the omega-6 fatty acid C18:2n-6 (i.e. cis-9, cis-12 octadecadienoic acid, linoleic acid) was also higher in clover compared with any other species (Table 4). The proportion of omega-3 decreased with increasing stage of maturity for oats (13.9 ± 0.86 vs 64.3 ± 0.68), ryegrass (17.3 ± 0.86 vs 68.2 ± 0.65) and phalaris (21.6 ± 1.63 vs 65.1 ± 0.79) but not to the same extent for sub clover (41.5 ± 1.38 vs 57.4 ± 0.79, Fig. 3a). The omega-6:omega-3 (n-6:n-3) ratio was highest for clover compared with any other species at early stages of growth, but similar to ryegrass and phalaris at later stages of growth (Fig. 3c).
FAME | FAME Proportion (g 100 g Total FAME−1) A | P-values | ||||||
---|---|---|---|---|---|---|---|---|
Oats | Ryegrass | Phalaris | Clover | Species | Harvest | Species × Harvest | ||
SFA | ||||||||
C8:0 | 0.04c (±0.00) | 0.03c (±0.00) | 0.13a (±0.01) | 0.08b (±0.01) | <0.001 | <0.001 | <0.001 | |
C10:0 | 0.76c (±0.03) | 0.96b (±0.03) | 0.80c (±0.04) | 3.14a (±0.04) | <0.001 | <0.001 | <0.001 | |
C12:0 | 1.32b (±0.03) | 1.41ab (±0.03) | 1.52a (±0.05) | 0.75c (±0.05) | <0.001 | <0.001 | <0.001 | |
C14:0 | 0.81c (±0.04) | 1.28a (±0.04) | 1.01b (±0.06) | 0.36d (±0.06) | <0.001 | <0.001 | <0.001 | |
C16:0 | 16.3c (±0.09) | 17.1a (±0.09) | 16.7b (±0.12) | 14.3d (±0.14) | <0.001 | <0.001 | <0.001 | |
C18:0 | 1.88b (±0.04) | 1.65c (±0.04) | 2.90a (±0.05) | 2.76a (±0.06) | <0.001 | <0.001 | <0.001 | |
C20:0 | 0.98b (±0.02) | 0.63c (±0.02) | 1.06a (±0.03) | 0.53d (±0.04) | <0.001 | <0.001 | <0.001 | |
C22:0 | 1.28a (±0.02) | 0.98b (±0.02) | 0.82c (±0.03) | 0.72d (±0.03) | <0.001 | <0.001 | <0.001 | |
C24:0 | 0.74c (±0.02) | 0.75c (±0.02) | 1.00b (±0.03) | 1.18a (±0.03) | <0.001 | <0.001 | <0.001 | |
Total SFA | 23.7c (±0.20) | 24.3b (±0.20) | 25.3a (±0.26) | 23.2c (±0.31) | <0.001 | <0.001 | <0.001 | |
MUFA | ||||||||
C14:1n-7 | 0.15 (±0.02) | 0.18 (±0.02) | 0.15 (±0.03) | 0.09 (±0.03) | 0.091 | 0.002 | 0.129 | |
C14:1n-5 | 0.08a (±0.01) | 0.10a (±0.01) | 0.08a (±0.01) | 0.02b (±0.01) | <0.001 | <0.001 | <0.001 | |
C15:1n-5 | 0.68c (±0.02) | 0.87a (±0.02) | 0.80b (±0.03) | 0.70c (±0.03) | <0.001 | <0.001 | <0.001 | |
C16:1n-9 | 1.48b (±0.04) | 1.92a (±0.05) | 1.94a (±0.08) | 1.86a (±0.07) | <0.001 | <0.001 | <0.001 | |
C16:1n-7 | 0.16b (±0.00) | 0.18a (±0.00) | 0.13c (±0.00) | 0.14c (±0.00) | <0.001 | <0.001 | <0.001 | |
C16:1n-2 | 0.53ab (±0.02) | 0.56a (±0.02) | 0.46b (±0.03) | 0.49b (±0.03) | 0.019 | <0.001 | 0.002 | |
C17:1n-7 | 0.27b (±0.01) | 0.32a (±0.01) | 0.18c (±0.02) | 0.12d (±0.02) | <0.001 | <0.001 | <0.001 | |
C18:1n-9 | 7.62a (±0.20) | 2.95bc (±0.21) | 3.63b (±0.38) | 2.17c (±0.34) | <0.001 | <0.001 | <0.001 | |
C18:1n-7 | 0.41a (±0.01) | 0.40a (±0.01) | 0.28b (±0.01) | 0.27b (±0.01) | <0.001 | <0.001 | <0.001 | |
C20:1n-9 | 0.22a (±0.01) | 0.10c (±0.01) | 0.14b (±0.01) | 0.09c (±0.01) | <0.001 | <0.001 | <0.001 | |
C22:1n-9 | 0.06a (±0.00) | 0.03c (±0.00) | 0.04b (±0.00) | 0.02d (±0.00) | <0.001 | <0.001 | <0.001 | |
C24:1n-9 | 0.14a (±0.00) | 0.15a (±0.00) | 0.09b (±0.01) | 0.03c (±0.01) | <0.001 | <0.001 | <0.001 | |
Total MUFA | 11.8a (±0.19) | 7.77b (±0.20) | 7.90b (±0.37) | 5.99c (±0.33) | <0.001 | <0.001 | <0.001 | |
n-3 PUFA | ||||||||
C18:3n-3 | 39.4c (±0.48) | 45.1b (±0.49) | 44.0b (±0.58) | 50.6a (±0.78) | <0.001 | <0.001 | <0.001 | |
C18:4n-3 | 0.08c (±0.00) | 0.12b (±0.00) | 0.07d (±0.00) | 0.26a (±0.00) | <0.001 | <0.001 | <0.001 | |
Total n-3 PUFA | 39.4c (±0.48) | 45.3b (±0.49) | 44.1b (±0.58) | 50.9a (±0.78) | <0.001 | <0.001 | <0.001 | |
n-6 PUFA | ||||||||
C18:2n-6 | 17.0b (±0.15) | 13.5d (±0.16) | 14.1c (±0.23) | 18.3a (±0.25) | <0.001 | <0.001 | <0.001 | |
C18:3n-6 | 0.10b (±0.01) | 0.18a (±0.01) | 0.04c (±0.02) | 0.10b (±0.02) | <0.001 | <0.001 | <0.001 | |
Total n-6 PUFA | 17.1b (±0.17) | 13.7c (±0.17) | 14.2c (±0.24) | 18.4a (±0.27) | <0.001 | <0.001 | <0.001 | |
Total FAME | 27.5c (±0.52) | 29.7b (±0.52) | 33.1a (±0.68) | 31.2ab (±0.79) | 0.002 | <0.001 | <0.001 | |
n-6:n-3 Ratio | 0.57a (±0.01) | 0.31b (±0.02) | 0.34b (±0.03) | 0.37b (±0.03) | <0.001 | <0.001 | <0.001 |
Values are least squares means ± s.e. of the least squares means.
FAME, fatty acid methyl ester; SFA, saturated fatty acids; MUFA, monounsaturated fatty acids; n-3 PUFA, omega-3 polyunsaturated fatty acids; n-6 PUFA, omega-6 polyunsaturated fatty acids; n-6:n-3, n-6 PUFA:n-3 PUFA ratio; C17:1n-7, (C17:1n-8 + C17:1n-7); Other, other minor fatty acids including C15:0, C17:0, C21:0 + unidentified fatty acids; Total FAME, total fatty acid concentration (g kg DM−1).
Mean proportion of (a) C18:3n-3 (α-linolenic acid), (b) C18:2n-6 (linoleic acid), and (c) n-6:n-3 ratio for oats (), ryegrass (
), phalaris (
), and clover (
) over different stages of development. Values are least squares means ± s.e. of the least squares means.
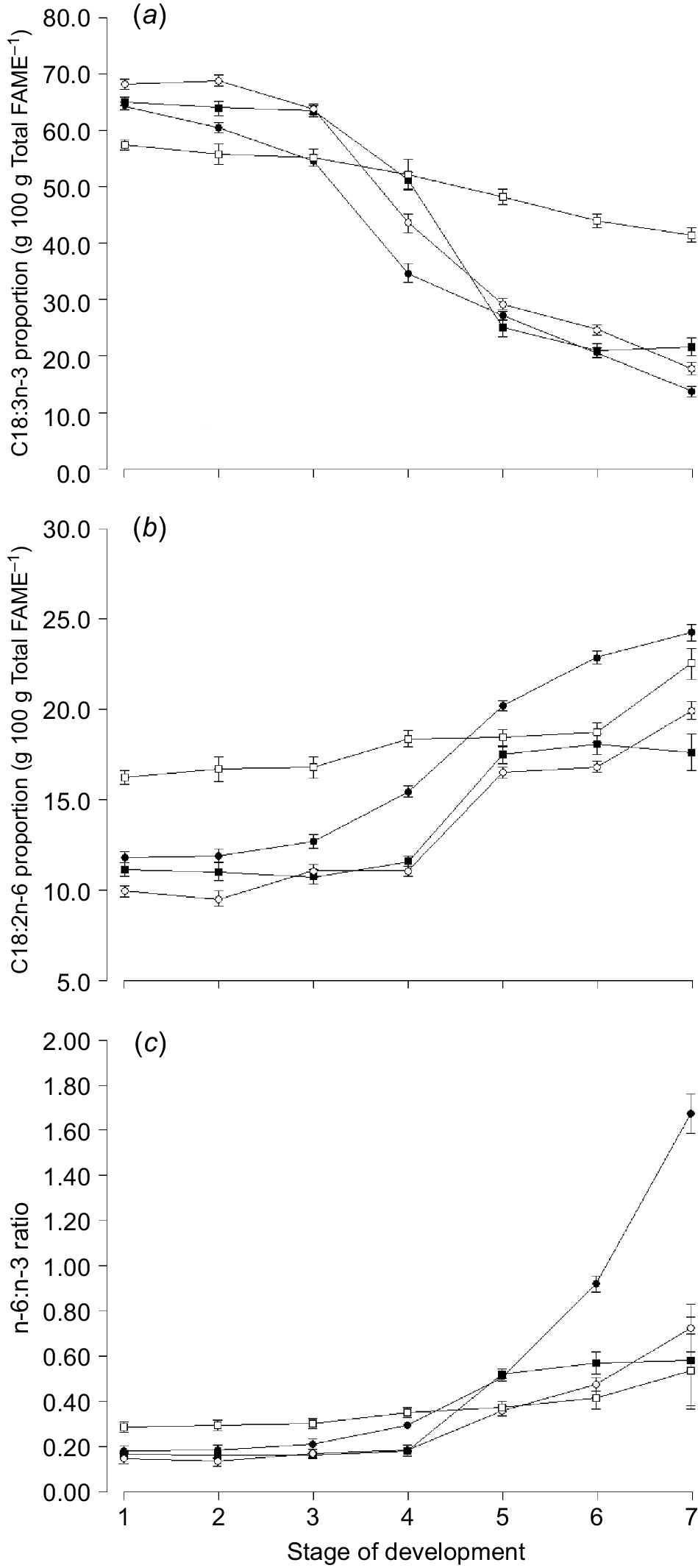
The n-6:n-3 ratio increased markedly in oats after Zadocks 47 (Harvest 5) and continued to increase towards maturity (Fig. 3c). The proportion of C16:0 increased with increasing stage of maturity for all species, but declined again towards senescence for oats (Fig. 4a). The proportion of C18:1n-9 increased over time and was higher for oats than any other species in the ‘ripening’ stage of development (Fig. 4c).
Mean proportion of (a) C16:0 (palmitic acid), (b) C18:0 (stearic acid), and (c) C18:1n-9 (oleic acid) for oats (), ryegrass (
), phalaris (
), and clover (
) over different stages of development. Values are least squares means ± s.e. of the least squares means.
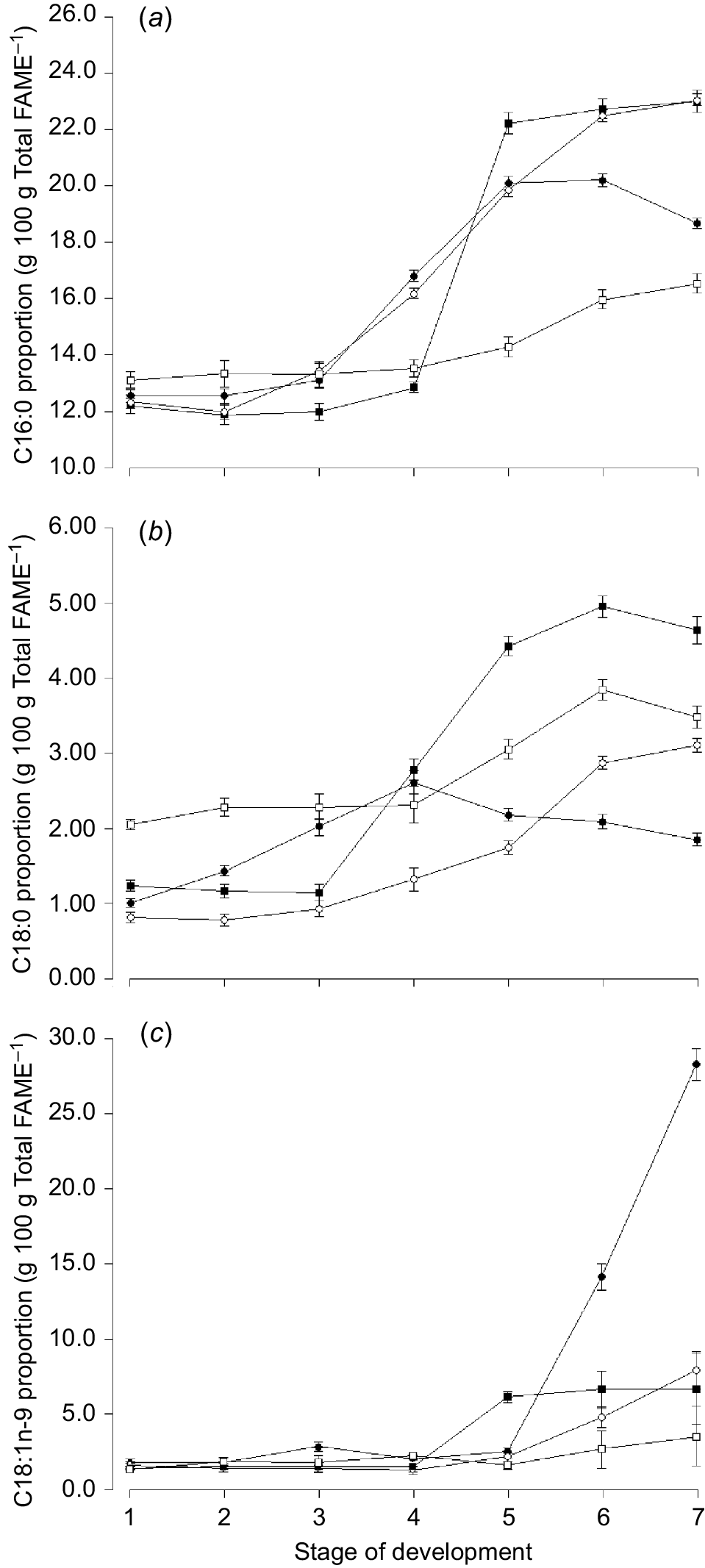
The proportion of total monounsaturated fatty acids (MUFA) was highest for oats compared with any other species (Table 4), which was mainly related to a higher proportion of C18:1n-9, particularly at later stages of development (Fig. 4c). The proportion of total saturated fatty acids (SFA) was highest for phalaris compared with any other species, however, the concentration of the major SFA C16:0 was highest for ryegrass (Table 4).
Correlation between forage quality and fatty acids
The proportion of omega-3 (g 100 g total fatty acids−1) was positively correlated with the CP content of plant material (Fig. 5). The omega-3 content was lower for clover compared with oats, ryegrass and phalaris at similar CP concentrations (Fig. 5). For phalaris, the omega-3 content appeared to remain above 50% total fatty acids until the CP content decreased below approximately 6% DM and then declined markedly. The proportion of C18:3n-3 was also positively related to EE (r2 = 0.77, 0.89, 0.43, 0.62 for oats, ryegrass, phalaris and clover, respectively) and M/D (r2 = 0.89, 0.24, 0.18, 0.28 for oats, ryegrass, phalaris and clover, respectively). The total analysed concentration of FAME was significantly positively correlated with the total lipid concentration determined by EE (Fig. 6).
Relationship between crude protein (g 100 g DM−1) and the proportion of C18:3n-3 (α-linolenic acid, g 100 g Total FAME−1) in oats ( C18:3n-3 = 21.6 × Ln (CP) − 5.62, r2 = 0.97, P = 0.000516), ryegrass (
C18:3n-3 = 21.0 × Ln (CP) − 3.57, r2 = 0.97, P = 0.0000344), phalaris (
C18:3n-3 = 19.2 × Ln (CP) − 1.20, r2 = 0.86, P = 0.0027) and clover (
C18:3n-3 = 16.8 × Ln (CP) − 0.90, r2 = 0.90, P = 0.001) over different stages of development.
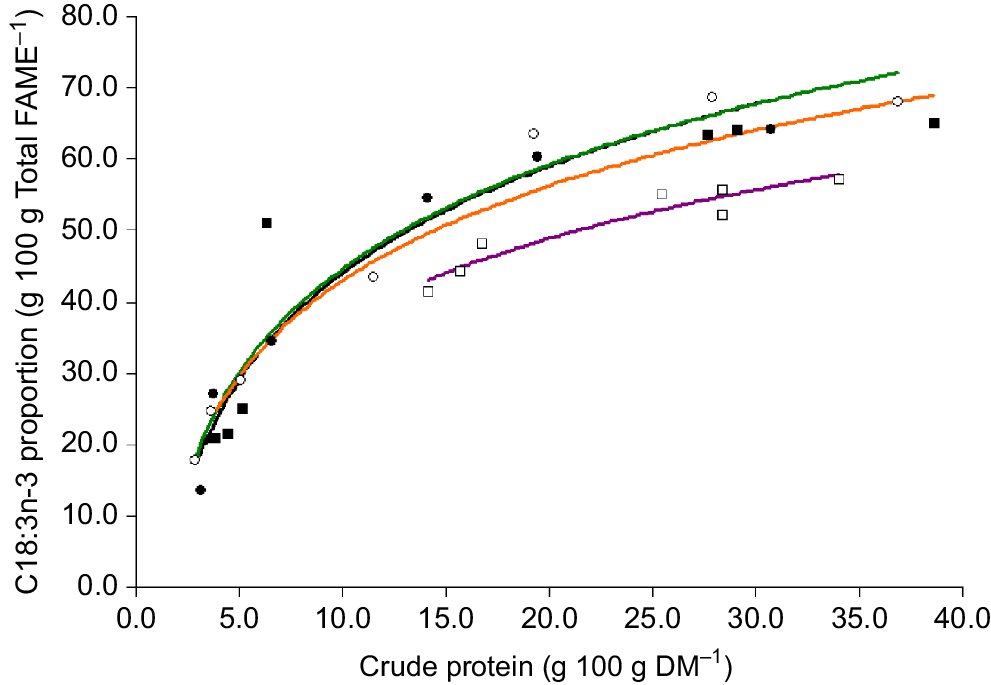
Relationship between total lipid determined from ether extract and total lipid calculated from total fatty acid concentration (total lipid = total FAME/0.883 g 100 g DM−1) in oats (), ryegrass (
), phalaris (
), and clover (
) over different stages of development. Total fatty acid concentration = 1.88 × EE − 2.89, r2 = 0.78, P = 0.0000000004165. Dashed line (– – –) denotes y = x.
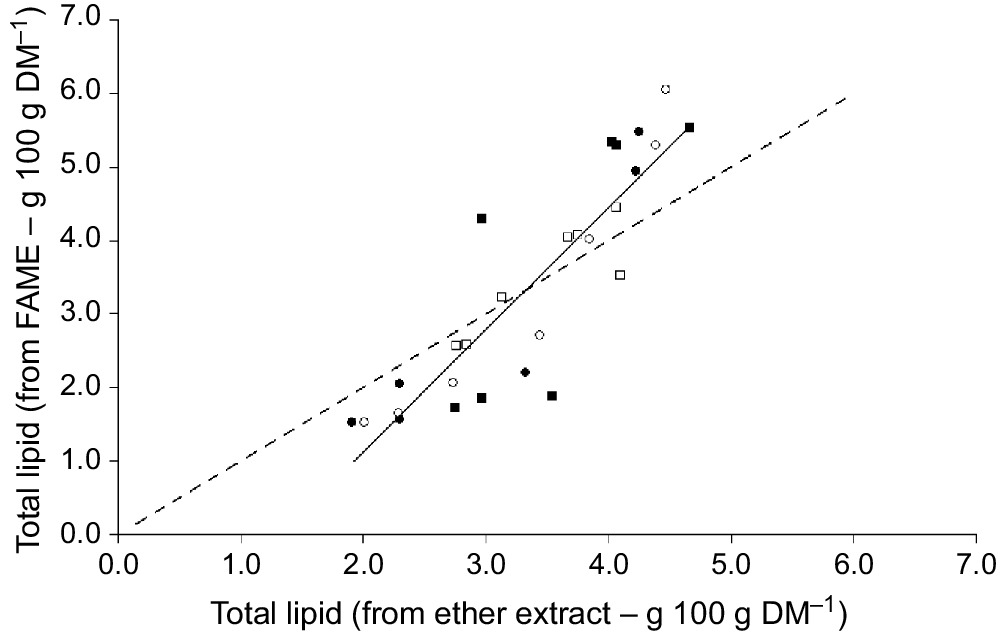
Discussion
Plant growth, water use and quality
The higher dry matter yield of oats and ryegrass compared with phalaris and clover was expected given previous assessments of the growth of annual and perennial forages (Muldoon 1986). The lower water use for phalaris compared with clover at early stages of growth, but higher water use at later stages, reflected the overall pattern of plant growth observed and previously established differences in water use efficiency between species (Johns and Lazenby 1973; Guobin et al. 1992).
The decrease in protein and energy content of all forages over time was typical of maturing forages (Cherney et al. 1993; Chaves et al. 2006). The negative relationship between NDF and M/D was expected and reflects the relatively low energy content of the fibre component of plant cell walls and the increase in structural carbohydrates at later stages of maturity (Cherney et al. 1993). The higher NDF content of oats compared with clover may indicate that the dry matter intake (DMI) of oats by livestock would be lower due to the negative relationship observed between NDF and potential intake, where DMI (% liveweight) = 120/NDF (% DM) (Van Soest 1965). The higher CP content of clover compared with other species at later stages of maturity was also expected given previous comparisons of forage species (Rattray and Joyce 1974). The higher M/D of oats approaching the ‘boot’ stage of development (Zadoks 47) and subsequent decline towards maturity was similar to previous results with cereal crops (Kaiser et al. 2007; Coblentz et al. 2018; Piltz et al. 2021) and corresponded to changes in water use observed in the current study.
Fatty acid changes over time
Although the omega-3 content was lower in phalaris than annual ryegrass at early stages of development, the consistent level of omega-3 over the first three stages of development in phalaris occurred despite the concentration of CP decreasing. The omega-3 content of phalaris remained higher than 50% of total fatty acids when CP was above 5% DM, indicating a preferential conservation of omega-3. The higher omega-6 content of oats at the ‘soft dough’ (Zadoks 85) and ‘ripening’ (Zadoks 92) stages was also expected given the development of seed material (Heppard et al. 1996). Desaturation of C18:1 fatty acids to C18:2 or C18:3 occurs after incorporation of C18:1 into glycerolipids following the action of acyl-CoA synthase on C18:0 (Browse and Somerville 1991). The desaturation of C18:1 to C18:2n-6 is facilitated by Δ−12 desaturase (FAD-2) found in higher abundance in seed compared with leaf material; and further desaturation to C18:3n-3 is facilitated by Δ−15 desaturase (FAD-3) found in higher abundance in leaf (Browse et al. 1986; Harwood 1996). The preferential action of FAD-2 on triacylglycerol (TAG) compared with FAD-3 acting on phosphatidylcholine (PC) or monogalactosyldiacylglycerol (MGDG) also contributes to the higher omega-6 fatty acid content of seeds, as the lipid content of seeds is predominantly TAG, whereas leaf material is predominantly PC and MGDG (Harwood 2021).
The higher proportion of C18:1n-9 in oats compared with other species was largely related to a significant increase in C18:1n-9 observed after Zadoks 65 (Harvest 5). Although LCn-3PUFA is considered to be healthy due to lowering the risk of CVD and other inflammatory disorders (Simopoulos 1999; Djuricic and Calder 2021), some research groups have claimed that higher MUFA, particularly C18:1n-9, may have beneficial effects on high density lipoprotein (HDL) cholesterol and triacylglycerols (TAGs) (Adams et al. 2010). The differential effect of these fatty acid profiles in early and later stages of maturity on indicators of human health outcomes should be examined, particularly for oats during seed head development.
Correlation between forage quality and fatty acids
The relationship between omega-3 content and CP was expected given the relationship between nitrogen in the leaf material and presence of omega-3 in leaf chloroplasts (Boufaïed et al. 2003). This relationship occurs as the rate limiting step for omega-3 production is the desaturation of C18:0–C18:1 in the chloroplast (Nagai and Bloch 1968). Following incorporation in glycerolipids, further desaturation to C18:3n-3 occurs (Browse and Somerville 1991) and, as indicated above, these processes are more abundant in leaf material, where growth is stimulated by higher nitrogen availability (Harwood 1980). The lower omega-3 content of clover at any CP concentration could have been related to a lower leaf:stem ratio compared with other annual or perennial species examined (Johns and Lazenby 1973; Khorasani et al. 1997). Unfortunately it was not possible to determine the leaf:stem ratio in the current study, however, future studies should determine whether maintenance of a higher leaf:stem ratio is associated with higher omega-3 at an equivalent CP content. Grazing management strategies that maximise the leaf:stem ratio could also be examined and may lead to improvements in overall forage quality (energy and protein) as well as omega-3 content.
The significant correlation between the total analysed concentration of fat (EE) with total fatty acid concentration indicates good recovery of fatty acids using the extraction and gas chromatography procedures employed, which was similar to previous results with sheep red blood cells (Clayton et al. 2012). The development of strategies to increase the amount of omega-3, such as plant breeding or grazing management, relies on the ability to be able to analyse fatty acid concentrations in large numbers of samples in a timely manner. Future studies should determine whether NIR technologies can be employed to determine changes in fatty acid profiles during growth and development of different plant species, thereby leading to rapid and cost-effective analysis.
Grazing management and maximising omega-3
Although forage yield (t DM ha−1) could not be determined in the current study, the dry matter produced per plant was higher in all species at later stages of development (data not shown). Grazing management usually seeks to maximise production per hectare based on a compromise between high yield and high quality (Bell et al. 2014). The optimum time for grazing was likely to be just prior to boot stage for oats (Zadoks 47) or late stem elongation in ryegrass and phalaris, when development was changing from vegetative to reproductive growth (Piltz et al. 2021). At this stage of growth quality, including CP and M/D, was still high and the rapid increase in the n-6:n-3 ratio had not occurred. If a higher omega-3 concentration is desired in order to supply fatty acids needed to target meat that was a ‘good source’ of omega-3, forages could be grazed earlier if a lower forage yield was acceptable.
The nutrient profile of the soil used in the current study could have affected plant growth and, therefore, fatty acid profile. For example, the soil was relatively high in potassium, which could have lead to reduced plant uptake of magnesium (Haby et al. 1990). Differences in soil nutrient profile would affect plant growth and likely lead to different concentrations of omega-3, particularly changes in omega-3 over different stages of growth. Further research is required in order to determine whether relative differences between species are consistent in soils of different nutrient availability.
The lack of effect of urea on CP and fatty acid composition was unexpected given previously known effects of added nitrogen (Boufaïed et al. 2003; Witkowska et al. 2008). The absence of an effect from urea was likely due to the small physical amount of urea applied, which may not have been distributed evenly across the pot. Future studies could examine a higher dose of nitrogen or liquid application to improve distribution and uptake, or the timing of nitrogen application. A number of samples grew a significant amount of dry matter following harvesting and re-growth. Future studies should also determine the quality and fatty acid profile of re-growth material compared with initial growth to estimate changes following multiple grazings and second year growth for perennials. The effect of grazing new and re-growth material by sheep and cattle on the omega-3 content of beef and lamb meat should also be examined.
Conclusions
The amount of omega-3 in forages was related to CP content, which is likely related to total leaf chloroplasts. A higher CP content was observed in clover compared with the other species examined, however, the proportion of omega-3 was lower in clover than the other species for any given CP level. Grazing forages at earlier stages of maturity and maximising the amount of CP available for growth will increase the amount of omega-3 in plant material. The effect of grazing and re-growth on omega-3 content as well as the consumption of these forages on the omega-3 content of red meat should also be determined. Now that preliminary relationships between stage of growth and CP on the omega-3 content of these common forage species have been established, future studies could also screen different varieties of each species to determine the variability in omega-3 and whether varieties can be selected that contain higher omega-3 at the same stage of growth or CP content.
Declaration of funding
The study was conducted with funding provided by the McCaughey Memorial Institute (Grant No. 20-01-08) examining the concentration of omega-3 in pastures and pasture-fed beef and lamb and the New South Wales Department of Primary Industries, Wagga Wagga Agricultural Institute.
Acknowledgements
We thank Peter Hawkins, Kathleen Bernie, Leanne Groves and Richard Meyer for expert technical assistance with the analysis of samples. We also thank Andrew Price (NSW DPI) and Bryce Elwin (Nutrien) for the provision of seed and inoculants. EHC designed the study and all authors have contributed to the conduct of the study, data interpretation and drafting and editing of the manuscript.
References
Adams TH, Walzem RL, Smith DR, Tseng S, Smith SB (2010) Hamburger high in total, saturated and trans-fatty acids decreases HDL cholesterol and LDL particle diameter, and increases TAG, in mildly hypercholesterolaemic men. British Journal of Nutrition 103, 91-98.
| Crossref | Google Scholar | PubMed |
Bauchart D, Verite R, Remond B (1984) Long-chain fatty acid digestion in lactating cows fed fresh grass from spring to autumn. Canadian Journal of Animal Science 64, 330-331.
| Crossref | Google Scholar |
Bell LW, Moore AD, Kirkegaard JA (2014) Evolution in crop-livestock integration systems that improve farm productivity and environmental performance in Australia. European Journal of Agronomy 57, 10-20.
| Crossref | Google Scholar |
Boufaïed H, Chouinard PY, Tremblay GF, Petit HV, Michaud R, Bélanger G (2003) Fatty acids in forages. I. Factors affecting concentrations. Canadian Journal of Animal Science 83, 501-511.
| Crossref | Google Scholar |
Browse J, Somerville C (1991) Glycerolipid synthesis: biochemistry and regulation. Annual Review of Plant Physiology and Plant Molecular Biology 42, 467-506.
| Crossref | Google Scholar |
Browse J, McCourt P, Somerville C (1986) A mutant of Arabidopsis deficient in C18:3 and C16:3 leaf lipids. Plant Physiology 81, 859-864.
| Crossref | Google Scholar |
Chaves AV, Waghorn GC, Brookes IM, Woodfield DR (2006) Effect of maturation and initial harvest dates on the nutritive characteristics of ryegrass (Lolium perenne L.). Animal Feed Science and Technology 127, 293-318.
| Crossref | Google Scholar |
Cherney DJR, Cherney JH, Lucey RF (1993) In vitro digestion kinetics and quality of perennial grasses as influenced by forage maturity. Journal of Dairy Science 76, 790-797.
| Crossref | Google Scholar |
Clapham WM, Foster JG, Neel JPS, Fedders JM (2005) Fatty acid composition of traditional and novel forages. Journal of Agricultural and Food Chemistry 53, 10068-10073.
| Crossref | Google Scholar | PubMed |
Clayton EH, Gulliver CE, Piltz JW, Taylor RD, Blake RJ, Meyer RG (2012) Improved extraction of saturated fatty acids but not omega-3 fatty acids from sheep red blood cells using a one-step extraction procedure. Lipids 47, 719-727.
| Crossref | Google Scholar | PubMed |
Coblentz WK, Akins MS, Kalscheur KF, Brink GE, Cavadini JS (2018) Effects of growth stage and growing degree day accumulations on triticale forages: 1. Dry matter yield, nutritive value, and in vitro dry matter disappearance. Journal of Dairy Science 101, 8965-8985.
| Crossref | Google Scholar | PubMed |
Colwell JD (1963) The estimation of the phosphorus fertiliser requirements of wheat in southern New South Wales by soil analysis. Australian Journal of Experimental Agriculture and Animal Husbandry 3, 190-197.
| Crossref | Google Scholar |
Condon J (2021) JBS launches price premium for ‘high performers’ among grassfed cattle. Available at https://www.beefcentral.com/markets/jbs-launches-premium-for-high-performers-among-great-southern-cattle/ [accessed 19 January 2022]
Cullis BR, Smith AB, Coombes NE (2006) On the design of early generation variety trials with correlated data. Journal of Agricultural, Biological, and Environmental Statistics 11, 381-393.
| Crossref | Google Scholar |
Dewhurst RJ, Scollan ND, Lee MRF, Ougham HJ, Humphreys MO (2003) Forage breeding and management to increase the beneficial fatty acid content of ruminant products. Proceedings of the Nutrition Society 62, 329-336.
| Crossref | Google Scholar |
Dewhurst RJ, Shingfield KJ, Lee MRF, Scollan ND (2006) Increasing the concentrations of beneficial polyunsaturated fatty acids in milk produced by dairy cows in high-forage systems. Animal Feed Science and Technology 131, 168-206.
| Crossref | Google Scholar |
Djuricic I, Calder PC (2021) Beneficial outcomes of omega-6 and omega-3 polyunsaturated fatty acids on human health: an update for 2021. Nutrients 13, 2421.
| Crossref | Google Scholar | PubMed |
Gillman GP, Sumpter EA (1986) Modification to the compulsive exchange method for measuring exchange characteristics of soils. Australian Journal of Soil Research 24, 61-66.
| Crossref | Google Scholar |
Glasser F, Doreau M, Maxin G, Baumont R (2013) Fat and fatty acid content and composition of forages: a meta-analysis. Animal Feed Science and Technology 185, 19-34.
| Crossref | Google Scholar |
Gulliver CE, Friend MA, King BJ, Clayton EH (2012) The role of omega-3 polyunsaturated fatty acids in reproduction of sheep and cattle. Animal Reproduction Science 131, 9-22.
| Crossref | Google Scholar | PubMed |
Guobin L, Kemp DR, Liu GB (1992) Water stress affects the productivity, growth components, competitiveness and water relations of phalaris and white clover growing in a mixed pasture. Australian Journal of Agricultural Research 43, 659-672.
| Crossref | Google Scholar |
Harwood JL (1996) Recent advances in the biosynthesis of plant fatty acids. Biochimica et Biophysica Acta (BBA) - Lipids and Lipid Metabolism 1301, 7-56.
| Crossref | Google Scholar | PubMed |
Harwood JL (2021) Plant fatty acid synthesis. Available at https://lipidlibrary.aocs.org/chemistry/physics/plant-lipid/plant-fatty-acid-synthesis [accessed 17 August 2023]
Heppard EP, Kinney AJ, Stecca KL, Miao GH (1996) Developmental and growth temperature regulation of two different microsomal [omega]-6 desaturase genes in soybeans. Plant Physiology 110, 311-319.
| Crossref | Google Scholar | PubMed |
Howe P, Meyer B, Record S, Baghurst K (2006) Dietary intake of long-chain ω-3 polyunsaturated fatty acids: contribution of meat sources. Nutrition 22, 47-53.
| Crossref | Google Scholar | PubMed |
Howe P, Buckley J, Meyer B (2007) Long-chain omega-3 fatty acids in red meat. Nutrition & Dietetics 64, S135-S139.
| Crossref | Google Scholar |
Johns GG, Lazenby A (1973) Defoliation, leaf area index, and the water use of four temperate pasture species under irrigated and dryland conditions. Australian Journal of Agricultural Research 24, 783-795.
| Crossref | Google Scholar |
Kaiser AG, Dear BS, Morris SG (2007) An evaluation of the yield and quality of oat-legume and ryegrass-legume mixtures and legume monocultures harvested at three stages of growth for silage. Australian Journal of Experimental Agriculture 47, 25-38.
| Crossref | Google Scholar |
Khan NA, Cone JW, Fievez V, Hendriks WH (2012) Causes of variation in fatty acid content and composition in grass and maize silages. Animal Feed Science and Technology 174, 36-45.
| Crossref | Google Scholar |
Khorasani GR, Jedel PE, Helm JH, Kennelly JJ (1997) Influence of stage of maturity on yield components and chemical composition of cereal grain silages. Canadian Journal of Animal Science 77, 259-267.
| Crossref | Google Scholar |
Kitessa SM, Young P, Nattrass G, Gardner G, Pearce K, Pethick DW (2012) When balanced for precursor fatty acid supply echium oil is not superior to linseed oil in enriching lamb tissues with long-chain n-3 PUFA. British Journal of Nutrition 108, 71-79.
| Crossref | Google Scholar | PubMed |
Lepage G, Roy CC (1986) Direct transesterification of all classes of lipids in a one-step reaction. Journal of Lipid Research 27, 114-120.
| Crossref | Google Scholar | PubMed |
Li GD, Lodge GM, Moore GA, Craig AD, Dear BS, Boschma SP, Albertsen TO, Miller SM, Harden S, Hayes RC, Hughes SJ, Snowball R, Smith AB, Cullis BC (2008) Evaluation of perennial pasture legumes and herbs to identify species with high herbage production and persistence in mixed farming zones in southern Australia. Australian Journal of Experimental Agriculture 48, 449-466.
| Crossref | Google Scholar |
Li GD, Nie Z, Bonython A, Boschma SP, Hayes RC, Craig AD, Lodge GM, Clark B, Dear BS, Smith AB, Harden S, Hughes SJ (2010) Evaluation of chicory cultivars and accessions for forage in south-eastern Australia. Crop & Pasture Science 61, 554-565.
| Crossref | Google Scholar |
Littell RC, Henry PR, Ammerman CB (1998) Statistical analysis of repeated measures data using SAS procedures. Journal of Animal Science 76, 1216-1231.
| Crossref | Google Scholar | PubMed |
Mayland HF, Molloy LF, Collie TW (1976) Higher fatty acid composition of immature forages as affected by N fertilization. Agronomy Journal 68, 979-982.
| Crossref | Google Scholar |
Meyer BJ, Mann NJ, Lewis JL, Milligan GC, Sinclair AJ, Howe PRC (2003) Dietary intakes and food sources of omega-6 and omega-3 polyunsaturated fatty acids. Lipids 38, 391-398.
| Crossref | Google Scholar | PubMed |
Muldoon DK (1986) The yield, quality and profitability of annual forage, perennial pasture and perennial forage systems under irrigation. Agricultural Systems 21, 201-213.
| Crossref | Google Scholar |
Nagai J, Bloch K (1968) Enzymatic desaturation of stearyl acyl carrier protein. Journal of Biological Chemistry 243, 4626-4633.
| Crossref | Google Scholar | PubMed |
Nogoy KMC, Sun B, Sine S, Lee Y, Li XZ, Choi SH, Park S (2022) Fatty acid composition of grain- and grass-fed beef and their nutritional value and health implication. Food Science of Animal Resources 42, 18-33.
| Crossref | Google Scholar | PubMed |
Packer EL, Clayton EH, Cusack PMV (2011) Rumen fermentation and liveweight gain in beef cattle treated with monensin and grazing lush forage. Australian Veterinary Journal 89, 338-345.
| Crossref | Google Scholar | PubMed |
Piltz JW, Rodham CA, Wilkins JF, Hackney BF (2021) A comparison of cereal and cereal/vetch crops for fodder conservation. Agriculture 11, 459.
| Crossref | Google Scholar |
Ponnampalam EN, Butler KL, Pearce KM, Mortimer SI, Pethick DW, Ball AJ, Hopkins DL (2014) Sources of variation of health claimable long chain omega-3 fatty acids in meat from Australian lamb slaughtered at similar weights. Meat Science 96, 1095-1103.
| Crossref | Google Scholar |
Rattray PV, Joyce JP (1974) Nutritive value of white clover and perennial ryegrass IV. Utilisation of dietary energy. New Zealand Journal of Agricultural Research 17, 401-406.
| Crossref | Google Scholar |
Ribeiro CVDM, Karnati SKR, Eastridge ML (2005) Biohydrogenation of fatty acids and digestibility of fresh alfalfa or alfalfa hay plus sucrose in continuous culture. Journal of Dairy Science 88, 4007-4017.
| Crossref | Google Scholar | PubMed |
Scollan ND, Choi N-J, Kurt E, Fisher AV, Enser M, Wood JD (2001) Manipulating the fatty acid composition of muscle and adipose tissue in beef cattle. British Journal of Nutrition 85, 115-124.
| Crossref | Google Scholar | PubMed |
Simopoulos AP (1999) Essential fatty acids in health and chronic disease. The American Journal of Clinical Nutrition 70, 560S-569S.
| Crossref | Google Scholar | PubMed |
Stampa E, Schipmann-Schwarze C, Hamm U (2020) Consumer perceptions, preferences, and behavior regarding pasture-raised livestock products: a review. Food Quality and Preference 82, 103872.
| Crossref | Google Scholar |
Twarakavi NKC, Sakai M, Šimůnek J (2009) An objective analysis of the dynamic nature of field capacity. Water Resources Research 45, W10410.
| Crossref | Google Scholar |
Van Soest PJ (1965) Symposium on factors influencing the voluntary intake of herbage by ruminants: voluntary intake in relation to chemical composition and digestibility. Journal of Animal Science 24, 834-843.
| Crossref | Google Scholar |
Veihmeyer FJ, Hendrickson AH (1931) The moisture equivalent as a measure of the field capacity of soils. Soil Science 32, 181-194.
| Crossref | Google Scholar |
Wang Z, Goonewardene LA (2004) The use of MIXED models in the analysis of animal experiments with repeated measures data. Canadian Journal of Animal Science 84, 1-11.
| Crossref | Google Scholar |
Wathes DC, Abayasekara DRE, Aitken RJ (2007) Polyunsaturated fatty acids in male and female reproduction. Biology of Reproduction 77, 190-201.
| Crossref | Google Scholar | PubMed |
Weihrauch JL, Posati LP, Anderson BA, Exler J (1977) Lipid conversion factors for calculating fatty acid contents of foods. Journal of the American Oil Chemists’ Society 54, 36-40.
| Crossref | Google Scholar | PubMed |
Witkowska IM, Wever C, Gort G, Elgersma A (2008) Effects of nitrogen rate and regrowth interval on perennial ryegrass fatty acid content during the growing season. Agronomy Journal 100, 1371-1379.
| Crossref | Google Scholar |
Zadoks JC, Chang TT, Konzak CF (1974) A decimal code for the growth stages of cereals. Weed Research 14, 415-421.
| Crossref | Google Scholar |