Forage brassicas can enhance the feed base and mitigate feed gaps across diverse environments
Lucinda J. Watt

A
B
Abstract
Spring-sown forage brassicas are commonly used to fill feed gaps in high-rainfall temperate livestock systems, but they have wider potential as an autumn-sown forage in drier environments within Australia’s crop–livestock zone.
We modelled the production potential of autumn-sown forage brassicas grown in diverse environments and tested their ability to alter the frequency and magnitude of feed gaps.
Long-term production potential was simulated in APSIM for four forage brassica genotypes, compared with forage wheat and dual-purpose canola across 22 diverse agro-climatic locations. For seven regions, the change in frequency and magnitude of forage deficits from adding forage brassicas to representative forage–livestock systems was predicted.
Across locations, median yields of forage brassicas ranged from 7 to 19 t DM/ha, and their annual metabolisable-energy yield was higher than that of forage wheat at most sites and nearly always exceeded dual-purpose canola. Forage brassicas performed better than forage wheat in later-sowing events (late April to early May) and maintained growth and quality later into spring. At five of the seven regions, adding 15% of farm forage area to forage brassicas reduced the frequency and magnitude of feed deficits by 35–50% and 20–40%, respectively. However, they were less beneficial where winter–spring feed gaps are uncommon.
We demonstrated that autumn-sown forage brassicas can be reliable and productive contributors to the feed base in drier environments and are a suitable alternative to forage cereals.
Forage brassicas can help reduce feed gaps and improve livestock production in a range of production systems spanning Australia’s crop–livestock zone.
Keywords: APSIM, autumn-sown, canola, crop-livestock zone, forage cereal, forage rape, livestock systems, raphanobrassica, simulation modelling.
Introduction
Forage brassicas have long been used as spring-sown crops in temperate higher-rainfall environments to fill gaps in livestock feed supply during summer–autumn and winter (Lindsay et al. 2007; Nie et al. 2020). They are highly beneficial because of their ability to produce biomass of high nutritive value over longer periods than do many grass-based pastures (Barry 2013). Although they will continue to play an integral role in these intensive forage-based livestock systems, it is increasingly evident that forage brassicas can play a wider role as alternative forage-crop options in drier environments such as those within Australia’s crop–livestock farming zone (Bell et al. 2020; Watt et al. 2021). To fit into these more arid environments (i.e. a lower ratio of mean annual rainfall to potential evapotranspiration), selection of more suitable genotypes and alterations to their pattern of use to target an autumn–winter growing period need to be considered because of the much higher aridity over the summer period in these regions (Bell et al. 2020). Previous field experiments at a selection of sites spanning the crop–livestock zone found that autumn-sown forage brassica genotypes (e.g. forage rapes and raphanobrassica) produced metabolisable energy (ME) yields similar to or higher than those produced by forage cereal options (e.g. oats (Avena sativa L.)) (Watt et al. 2021). These field evaluations were undertaken at a limited number of sites (n = 10) and under particularly dry seasons; so, forage production potential across a broader range of seasonal conditions and other environments still needs to be assessed.
The highly variable rainfall across Australia’s crop-livestock farming zone means that forage production is also likely to be highly variable. This means that livestock farmers regularly face feed gaps, defined as a period of insufficient forage supply to meet livestock demand (Moore et al. 2009). These feed gaps can limit the productivity potential of livestock enterprises, through livestock losing condition, the need to destock during these periods, or else offer expensive supplementary feeding. In many parts of the crop–livestock-farming zone, the autumn and winter period induce periods of low or variable forage supply, where forage crops such as forage cereals (e.g. oats, wheat (Triticum aestivum L.), or barley (Hordeum vulgare L.)) have been traditionally used, and more recently dual-purpose crops of wheat or canola (Brassica napus var. annua L.) (Moore et al. 2009; Bell et al. 2015a). Better understanding how forage brassicas compare to, or complement other components of the feed base to boost farm forage production and/or fill gaps at a farm scale is required.
The development of a robust forage brassica simulation model for a selection of genotypes in APSIM (Watt et al. 2022) has made it possible to predict the potential production and suitability of forage brassicas across a broader range of growing conditions and production systems. This study used this new simulation modelling capability to further establish the wider potential of forage brassicas in Australia’s crop-livestock farming zone by predicting the variability in potential biomass and ME production of autumn-sown forage brassicas across a wide range of environments spanning this farming zone. The productivity of the forage brassicas was then compared with predictions for forage cereals used in livestock systems in a similar way. Modelled growth-rate predictions of other key pasture and forage crops can be used to test the ability of diverse on-farm feed sources to support the energy demands of different livestock production systems, especially their ability to fill seasonal feed gaps (e.g. Bell et al. (2008)). In this study, we included forage brassicas as an additional feed source in the on-farm feed base and tested their ability to alter the frequency and size of feed gaps for representative livestock production systems at several locations differing in agro-climatic conditions. We find that forage brassicas could offer a viable alternative to forage cereals and can significantly reduce the risk of feed gaps in livestock systems across a wide range of conditions.
Materials and methods
To predict potential production of forage brassicas across Australia’s crop-livestock farming zone, 22 simulated locations were selected on the basis of a broad distribution of landscapes and climates to capture the diversity of agro-climatic conditions (Hutchinson et al. 2005) and production environments (Interim Biogeographic Regionalisation for Australia v. 7) (Department of Agriculture, Water and the Environment 2020) (Fig. 1). The Agricultural Production Systems Simulator (APSIM) ver. 7.4 (www.apsim.info) was used for all simulations (Holzworth et al. 2014) and each location was configured with local soil and climate information. Simulations used the APSIM-forage brassica growth model that has been calibrated and widely tested across 23 diverse production environments and agronomic management systems throughout Australia and New Zealand (Watt et al. 2022). The parameterised models for the specified genotypes have been tested against 220 observations of plant biomass and 102 observations of forage nutritive value to predict metabolisable-energy (ME) yield (for further details, refer to Watt et al. (2022)). The Soils and Landscapes Grid of Australia (Grundy et al. 2015) and the SoilMapp iPad app (CSIRO 2020) were used to identify a representative soil for each location that was then selected from the APSoil database (https://www.apsim.info/apsim-model/apsoil/) (Table 1). Meteorological data for all locations were sourced via SILO Long Paddock database (Jeffrey et al. 2001).
Distribution of the 22 simulated locations across different agro-climatic regions of Australia’s crop-livestock farming zone. Black points indicate location of sites with only APSIM predictions of forage biomass production and ME yield, and yellow points indicate the seven locations included in the additional whole-farm feed base analysis.
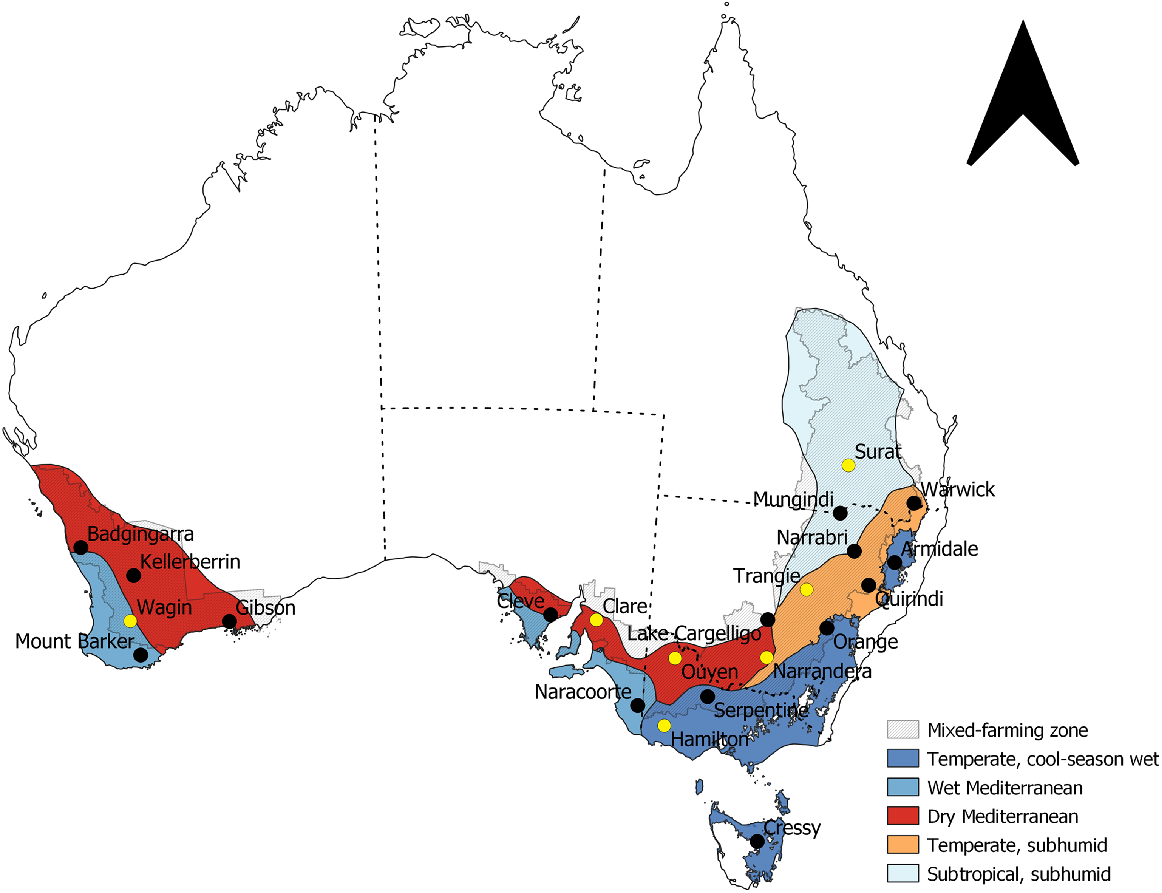
Agro-climatic region | Location | Latitude, longitude | MAR (mm) | GS rainfall (mm) | GS rainfall CV (%) | GS mean temperature (°C) | GS aridity index (mm/mm) | Soil type (APSoil number) | PAWC (mm) | |
---|---|---|---|---|---|---|---|---|---|---|
TCSW | Orange, NSW | −33.3, 149.1 | 910 | 605 | 33 | 9.8 | 0.94 | Sandy loam (704) | 176 | |
Armidale, NSW | −30.5, 151.7 | 792 | 407 | 25 | 10.8 | 0.66 | Red Chromosol (236) | 153 | ||
Cressy, Tas. | −41.7, 147.1 | 619 | 440 | 24 | 9.7 | 0.86 | Sandy loam (652) | 217 | ||
Hamilton, Vic. | −37.7, 142.1 | 617 | 482 | 21 | 11.4 | 0.84 | Clay (632-YP) | 261 | ||
Serpentine, Vic. | −36.4, 144.0 | 507 | 297 | 35 | 12.8 | 0.46 | Clay loam (524) | 202 | ||
WM | Mount Barker, WA | −34.6, 117.6 | 727 | 568 | 18 | 13.4 | 0.88 | Duplex sandy gravel (1237) | 172 | |
Naracoorte, SA | −37.0, 140.7 | 578 | 439 | 25 | 12.5 | 0.67 | Silty loam (1250) | 113 | ||
Wagin, WA | −33.3, 117.3 | 430 | 343 | 22 | 13.8 | 0.48 | Deep sandy duplex (403) | 80 | ||
DM | Clare, SA | −33.8, 138.6 | 634 | 469 | 25 | 12.4 | 0.59 | Clay loam (287) | 251 | |
Gibson, WA | −33.7, 121.8 | 618 | 464 | 19 | 14.9 | 0.55 | Duplex sandy gravel (448) | 100 | ||
Badgingarra, WA | −30.3, 115.5 | 538 | 482 | 25 | 16.3 | 0.48 | Brown deep sand (904) | 113 | ||
Cleve, SA | −33.7, 136.5 | 400 | 312 | 28 | 14.8 | 0.34 | Red sandy clay loam (316) | 98 | ||
Kellerberrin, WA | −31.6, 117.7 | 329 | 257 | 30 | 15.2 | 0.27 | Red clay (415) | 237 | ||
Ouyen, Vic. | −35.1, 142.3 | 328 | 229 | 36 | 14.1 | 0.30 | Loam – swale (642) | 214 | ||
TSH | Warwick, Qld | −28.2, 152.1 | 688 | 354 | 34 | 14.8 | 0.42 | Black Vertosol (31) | 245 | |
Quirindi, NSW | −31.5, 150.7 | 680 | 376 | 33 | 14.0 | 0.46 | Black Vertosol (1166) | 214 | ||
Trangie, NSW | −32.0, 148.0 | 493 | 300 | 42 | 14.7 | 0.34 | Clay (684) | 193 | ||
Narrandera, NSW | −34.7, 146.5 | 433 | 298 | 34 | 13.6 | 0.41 | Brown Chromosol (174) | 191 | ||
Lake Cargelligo, NSW | −33.3, 146.4 | 417 | 278 | 35 | 14.5 | 0.33 | Red Dermosol (1196) | 199 | ||
STSH | Narrabri, NSW | −30.3, 149.8 | 659 | 331 | 35 | 15.9 | 0.35 | Grey Vertosol (97) | 218 | |
Surat, Qld | −27.2, 149.1 | 573 | 279 | 38 | 17.5 | 0.27 | Black Vertosol (1282) | 289 | ||
Mungindi, NSW | −29.0, 149.0 | 502 | 263 | 39 | 17.3 | 0.26 | Grey Vertosol (1279) | 204 |
TCSW, temperate, cool-season wet; WM, wet Mediterranean; DM, dry Mediterranean; TSH, temperate, subhumid; STSH, subtropical, subhumid; NSW, New South Wales; SA, South Australia; WA, Western Australia; Vic., Victoria; Qld, Queensland; Tas., Tasmania.
Management of simulated crops
To enable direct comparisons between locations and genotypes, all forage crops were simulated with common agronomic management, with the only differences among locations being soil type, starting soil water conditions and meteorological data. Simulations at each location were conducted over 60 years (1960–2020) for four forage brassica genotypes that have been calibrated in APSIM (i.e. Winfred, Goliath, and HT-R24 forage rapes, and Pallaton raphanobrassica) and for comparison crops, a forage cereal (wheat) and dual-purpose canola. The three forage rape cultivars differ in maturity and, hence, length of the vegetative phase (Winfred, 1100°C days; HT-R24, 1200°C days; and Goliath, 1400°C days); otherwise, they have common plant-growth parameters. The raphanobrassica cv. Pallaton model differs from the others in several parameters that drive leaf development and size in addition to a longer vegetative phase (1600°C days) (Watt et al. 2022).
The sowing window for all crops across all locations commenced on 1 March and ended on the 31 May each year. Longer-season winter cultivars were used to simulate production from forage wheat (cv. Wedgetail) and dual-purpose canola (cv. Taurus) for early sowing opportunities before 1 May and a shorter-season variety (cv. Gregory wheat; cv. CBI406 canola) for sowing after this date. Sowing of all crops was initiated at the first instance in the sowing window where minimal allowable available soil water was 50 mm, and rainfall exceeded pan-evaporation over a 7-day period (Unkovich 2010). Row spacing was set at 250 mm and sowing depth of all brassica crops (including canola), and forage wheat was set at 20 mm and 40 mm, respectively. Plant density varied among the crops owing to differences in seed size and/or sowing rate, with some adjustments being based on laboratory germination counts and an estimated emergence of 85%, with forage wheat set at 120 plants/m2 (sown at 50 kg/ha), dual-purpose canola, and forage rapes Goliath and Winfred set at 60 plants/m2 (sown a 3 kg/ha), and forage rape HT-R24 and raphanobrassica Pallaton set at 50 plants/m2 (sown at 3 kg/ha and 6 kg/ha, respectively).
Starting soil water and nitrogen (N) were reset on 15 January of each simulated year at levels expected to mimic the soil water conditions following a cereal crop sown the previous year. Starting soil water was set at 30% of the plant-available water, for locations where summer rainfall occurs with some frequency (i.e. locations in the following agro-climatic zones: temperate, cool-season wet; wet Mediterranean; temperate, subhumid; and subtropical, subhumid). Starting soil water was set at 0% for locations with little summer rainfall (i.e. locations in the dry Mediterranean agro-climatic zone). After this reset, rainfall and evaporative processes proceeded in a realistic way prior to sowing. At sowing, starting soil nitrate-N was set at 50 kg/ha, distributed in the first 1000 mm of soil, and 150 kg/ha of urea-N was applied. A maximum of two additional applications of urea-N were applied from late-emergence to floral initiation (APSIM stage 3.5–5.0) when topsoil N (i.e. N in soil layers 1 and 2) reached a 30 kg/ha threshold, which best represented recommended agronomic practices. All crops were terminated on 31 December, unless already harvested (e.g. dual-purpose canola). Although most forages would experience numerous defoliation or grazing events over this period, we simulated potential growth without defoliation events to maintain consistency across all simulations and because of uncertainty in the capacity of the model to simulate regrowth dynamics of these crops (Watt et al. 2022).
Production metrics calculated
On a monthly basis, net biomass production (i.e. sum of daily biomass growth) and potential ME yield were calculated for each of the simulated crops (Eqn 1). Total annual forage-production potential was estimated for each of the crops by accumulating net biomass production from sowing to a stage when grazing by livestock would cease to estimate ungrazed growth potential over this period. For forage brassicas and forage wheat, this was accumulated up until flowering (APSIM stage 6.0), whereas for dual-purpose canola, this was calculated only until bud-elongation (APSIM stage 4.9) to account for the time when the crop would be locked-up from livestock grazing for grain production.
Whole-farm feed base risk analysis
To examine the potential for forage brassicas to complement the existing feed base, an additional analysis examined continuity of whole-farm forage supply and demand for a representative livestock enterprise at seven selected regions spanning different agro-climatic regions across the crop–livestock-farming zone (Fig. 1). This analysis was performed using the farm feed base risk calculator, a simple spreadsheet model that computes the ME balance of a farm to predict the frequency and size of monthly feed surpluses and deficits over the long term (see Bell et al. 2018 for details). In brief, the annual cycle of monthly energy demand of a representative livestock enterprise is derived from widely used calculations of energy requirements for each class of livestock that accounts for their annual growth, lactation, and pregnancy cycles (Table 2). Monthly energy demands of the livestock enterprise are then compared against long-term simulations (1960–2010) of monthly production and nutritive value for forages and their relative contribution to the feed base (i.e. % area of farm) to calculate the ME available (Table 2). To account for carry-over of forage, two-thirds of any surplus forage is carried forward to the next month. Using this model, the timing and frequency of edible biomass fell below 500 kg DM/ha and, when a negative feed balance occurred, were calculated as indicators of the need for livestock supplementary feeding or when livestock numbers would need to be reduced.
Region and location | |||||||||||||||
---|---|---|---|---|---|---|---|---|---|---|---|---|---|---|---|
Maranoa, Qld | Central west, NSW | Riverina, NSW | South-west, Vic. | Mallee, Vic. | Mid-north, SA | Great Southern, WA | |||||||||
Roma/Surat | Trangie/Condobolin | Narrandera | Hamilton | Birchip | Clare | Katanning/Wagin | |||||||||
Livestock system specification | |||||||||||||||
Enterprise | Bos indicus-cross cows, store weaners | Merino ewes, fat lambs | Dual-purpose ewes, store lambs | First-cross ewes, fat lambs | Merino ewes, store lambs | Merino ewes, fat lambs | Merino ewes, store lambs | ||||||||
Calving/lambing window | 1 November – 15 December | 15 August – 15 September | 15 June – 15 July | 15 August – 15 September | 15 August – 15 September | 1 June – 15 July | 1 June – 15 July | ||||||||
Weaning date | 30 May | 1 November | 1 September | 1 November | 1 November | 1 September | 1 September | ||||||||
Progeny sale date | 30 May | 15 March | 1 December | 15 March | 1 January | 1 February | 1 December | ||||||||
Stocking rate A (BU/grazed ha) | 0.35 | 1.56 | 2.75 | 3.80 | 1.87 | 3.35 | 2.91 | ||||||||
Stocking rate (DSE/ha) | 5.3 | 4.3 | 8.0 | 11.9 | 4.6 | 8.5 | 8.0 | ||||||||
Baseline-farm feed base | |||||||||||||||
Feed base composition (% area) | Buffel grass | 80 | Subclover + barley grass | 30 | Subclover + barley grass | 40 | Phalaris | 33 | Medic + barley grass + capeweed | 100 | Medic + barley grass + capeweed | 100 | Subclover + ryegrass + capeweed | 100 | |
Panic grass + medic | 20 | Phalaris | 40 | Lucerne | 30 | Tall fescue | 33 | ||||||||
Lucerne | 30 | Phalaris | 30 | Lucerne | 33 | ||||||||||
Median forage utilisation (%) | 44 | 42 | 44 | 44 | 40 | 42 | 40 |
The Baseline feed base analysed for each region reflected the main pasture or forage sources available, and the proportion of farm area typically allocated to each. These were chosen on the basis of those reported previously, which were obtained through consultations with local producers (see Bell et al. (2018)). In each Baseline scenario, the stocking rate of the livestock enterprise was adjusted to achieve a median annual utilisation of the farm feed base of ~40–45% (i.e. livestock demand as a percentage of the ME produced by all forages grown on farm), to allow for reasonable comparisons of the feed base risk impacts among regions (Table 2). This baseline scenario was then compared to three additional feed base scenarios where the farm stocking rate was maintained constant (i.e. same number of breeding units per grazed hectare including any additional forages). The first involved an additional 15% of the farm area allocated to a forage brassica (+forage brassica). The second, involved allocating 15% of the farm area to a forage cereal (+cereal). The third involved allocating a combination of 15% each of both the forage brassica and forage cereal, to a total of 30% (+both). These scenarios used inputs of monthly forage production and nutritive value derived from the long-term simulations described previously for forage rape cv. Winfred and forage wheat.
Finally, although the previous scenarios maintained a constant stocking rate, an additional analysis used the farm feed base risk calculator to calculate the potential to increase the stocking rate of the farm while achieving a similar level of risk when only forage brassicas were introduced into the farm feed base. This was undertaken by adjusting the stocking rate per grazed hectare until the same net farm feed deficit was achieved over the long-term simulation. At this point, both the previous Baseline scenario and the +forage brassica scenario were deemed to have equivalent feed-gap risk.
Results
Productivity potential of forage brassicas across environments
The predicted productivity of forage brassicas was highest (>14 t DM/ha.year, on average) in those locations with a winter/uniform rainfall distribution and annual average rainfall exceeding 600 mm, and, hence, a longer growing season for winter-growing crops. Simulated annual forage production was surprisingly similar across a range of locations in the temperate, cool-season wet environments and higher-rainfall Mediterranean environments (Fig. 2), with growth in the cooler locations (e.g. Cressy, Orange) being often limited over winter because of the much cooler temperatures. At these locations forage brassica production was relatively consistent among years, with 50% of years varying around the median by only 2–3 t DM/ha.year. The drier locations in these agro-climatic zones, such as Serpentine, Wagin and Badgingarra, had an upper growth potential similar to that of other locations but had higher variability in production because of more frequent water stress that limited their growth in drier seasons.
Summary of simulated potential annual biomass production and sowing window for forage brassicas (forage rape cvv. Goliath, Winfred and HT-R24, and raphanobrassica cv. Pallaton) across environments in Australia’s crop–livestock farming zone. Variation over the 60-year simulation is shown by the box (25th and 75th percentile) either side of the median, and whiskers (5th and 95th percentile). Sowing window represents the upper and lower quartile for simulated sowing dates.
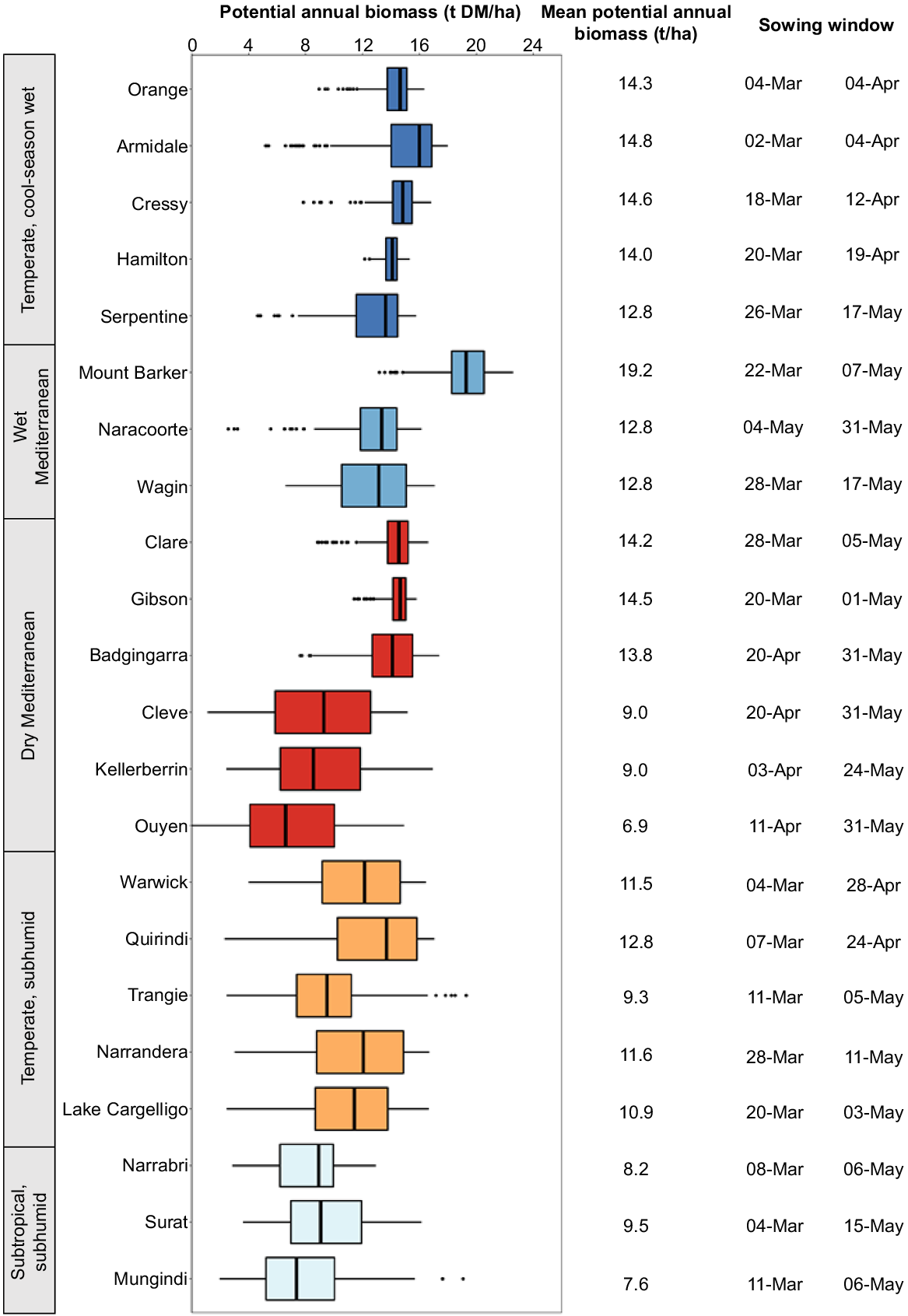
Simulated productivity potential of the forage brassicas was lower (~11.2 t DM/ha.year, on average) and more variable still in locations in the dry Mediterranean environment (e.g. Cleve, Kellerberrin, and Ouyen) and locations in the temperate, subhumid environment (Fig. 2), where water limitations are more likely to limit forage growth. Here, annual production varied significantly, with 5–8 t DM/ha.year variance between the upper and lower quartiles of simulated seasons. Simulated production potential was lowest and equally variable in locations in the subtropical, subhumid environment (8.4 t DM/ha.year average) where winter rainfall is less and more variable.
There were negligible differences in the predicted annual production among the four forage brassica genotypes, at least under the simulated crop management used here where differences in grazing response were not captured (Fig. 3). The only exception was at Mount Barker where the simulated production of Goliath forage rape and Pallaton was higher by ~20–30 GJ ME/year (approximately 1.5–2.5 t DM/ha) (Fig. 3f). There were some small differences in the timing of forage production through the year, with the raphnobrassica having lower production in the cooler months but then higher production in spring (data not shown).
Relative difference in simulated annual metabolisable energy (ME) yield (GJ ME/ha.year) of Goliath (dark blue; solid), Winfred (light blue; solid), HT-R24 (dark blue; dotted), and Pallaton (light blue; dotted) compared with forage wheat (black; solid) over 60 simulated years across temperate, cool season wet (a–e), wet Mediterranean (f–h), dry Mediterranean (i–n), temperate, subhumid (o–s), and subtropical, subhumid (t–v) environments in Australia’s crop–livestock farming zone.
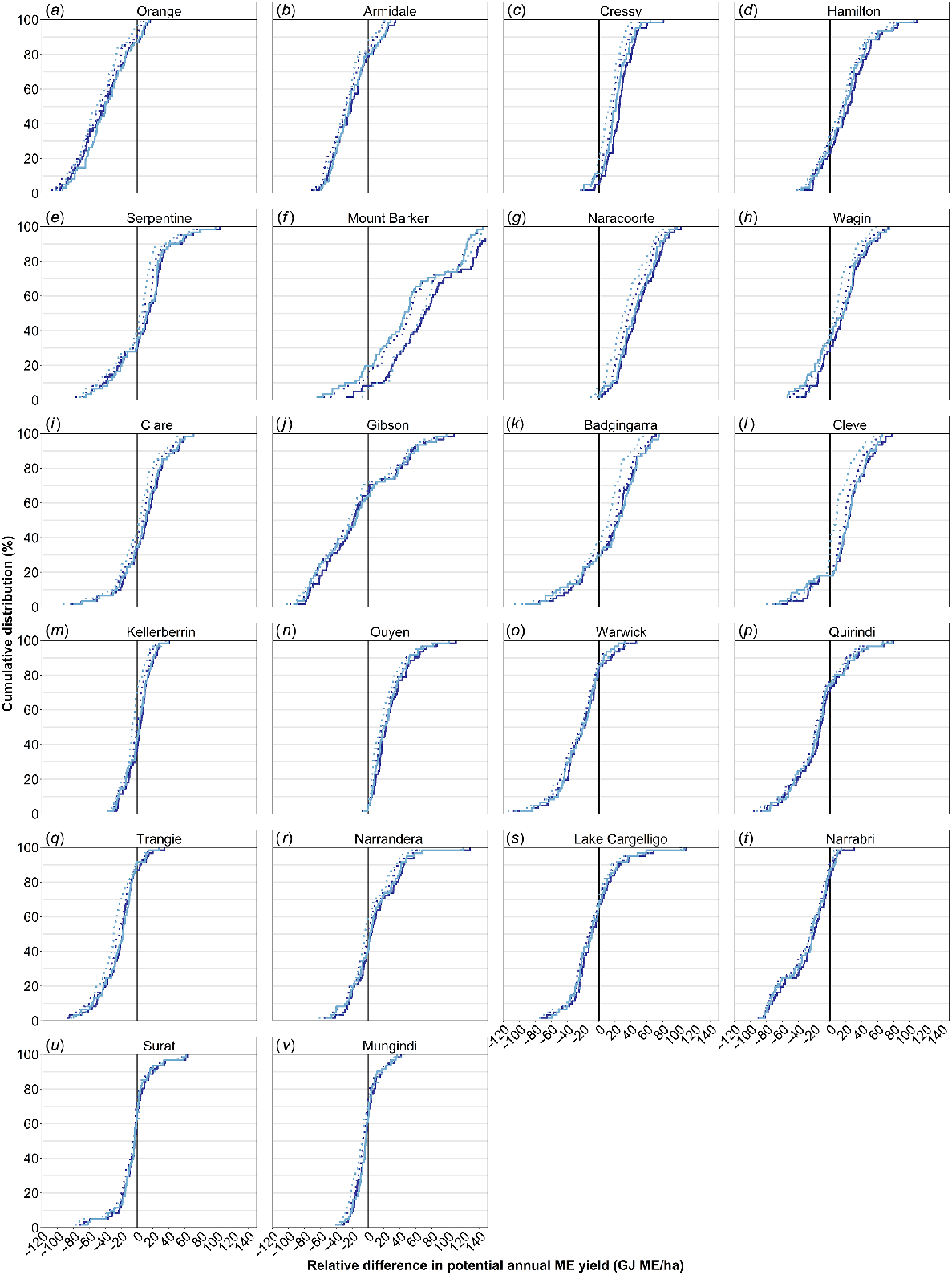
Using the 60-year simulated period, the sowing window of the forage brassicas for each location was established on the basis of the date when at least 50 mm of available soil water, and rainfall exceeded pan evaporation over a 7-day period. This highlighted significant differences in the probable sowing window of the forage brassicas (i.e. the inter-quartile range in simulated sowing dates) for the various agro-climatic environments and locations. Early and consistent sowing opportunities occurred during early autumn (early March to early April) in locations in the temperate, cool-season wet environment (e.g. Orange, Armidale, Cressy, and Hamilton). Locations in the higher-rainfall Mediterranean climates (e.g. Mount Barker, Wagin, Clare, and Gibson) were sown later, most often sowing from late March until early/mid-May. Similarly, the southern locations with more winter-dominant rainfall in the temperate, subhumid environment (i.e. Trangie, Narrandera, and Lake Cargelligo) tended to have a sowing window from mid-/late March until early/mid-May (Fig. 2). By comparison, locations in the drier Mediterranean environment (e.g. Ouyen, Cleve, and Badgingarra) had much later and a more concentrated sowing window, starting from late April until the end of May (Fig. 2). In contrast to the winter-dominant rainfall regions, the five most northern locations (i.e. locations in the subtropical, subhumid environment, and Warwick and Quirindi) had a very wide sowing window (early/mid-March until early/mid-May, reflecting their highly variable autumn and early winter rainfall (Fig. 2).
Relative productivity of forage brassicas compared with alternatives
In general, across the 22 simulated locations, the ME content of the vegetative biomass of forage brassicas and dual-purpose canola was comparable at 11.8 MJ ME/kg DM, but forage wheat was marginally higher at 12.1 MJ ME/kg DM. However, the productivity potential of the forage brassicas was nearly always greater than that of dual-purpose canola (data not shown). These differences reflect the much longer vegetative growing season of forage brassicas, whereas grazing would stop earlier at the onset of reproductive development in dual-purpose canola. This production advantage for the forage brassicas over dual-purpose canola varied among locations and seasons, often being associated with the longer growing seasons. In temperate, cool-season wet, and wet Mediterranean environments, the forage brassicas produced 50–120 GJ ME/ha more than did the dual-purpose canola. These advantages were less (commonly ~30–80 GJ ME/ha higher) in the other environments that have typically shorter winter growing seasons.
At over half of the locations, the forage brassicas consistently exceeded the predicted ME yield of forage wheat (Fig. 3). The forage brassicas were predicted to produce a higher annual ME yield than did forage wheat in more than 80% of simulated years at the following 6 of the 22 locations: Cressy (Fig. 3c), Hamilton (Fig. 3d), Mount Barker (Fig. 3f), Naracoorte (Fig. 3g), Cleve (Fig. 3l) and Ouyen (Fig. 3n). At another six locations, the forage brassicas produced more than did forage wheat in most seasons (i.e. 60–80% of years), including Serpentine (Fig. 3e), Wagin (Fig. 3h), Clare (Fig. 3i) Badgingarra (Fig. 3k), Kellerberrin (Fig. 3m), and Narrandera (Fig. 3r), but there were some years where the forage wheat was predicted to be superior. At the remaining sites (10 of the 22), forage wheat was consistently more productive than the forage brassicas, with the annual ME yield of forage brassicas being higher than that of forage wheat in only 10–30% of simulated years. The advantage of forage brassicas was not isolated or related to any particular agro-climatic environment, occurring at locations ranging from higher-rainfall temperate, cool season wet environment (e.g. Armidale and Orange) to drier Mediterranean environments (e.g. Kellerberrin). However, what is apparent is that the sowing windows across locations did influence the relative performance of forage brassicas compared with forage wheat (discussed below).
Effect of sowing time on forage brassica productivity potential
The simulated total annual forage productivity of the forage brassicas was shown to be more consistent across a range of sowing times at most locations. Only at locations with a short growing season (e.g. Cleve, Ouyen, or Mungindi) did production significantly decline as sowing was delayed. In contrast, forage wheat production consistently declined with later sowing across almost all locations (Fig. 4). Hence, at most locations, when forage wheat and brassicas are sown earlier, the forage wheat often produced higher annual ME yields, whereas at later sowing dates, forage brassicas often achieved similar or higher ME yields than did forage wheat. The point at which this transition occurred varied with location, although the simulated annual ME yield of forage brassicas often exceeded that of forage wheat when sowing occurred after late April to early May (Fig. 4). Differences in the interaction between sowing time and forage production for forage brassicas and forage wheat reflect the much higher vernalisation requirement, and thus a longer period of vegetative growth of the forage brassicas than forage wheat, meaning that they are more capable of maintaining production and nutritive value for longer under later sowing conditions. This was especially advantageous in environments where early season rainfall is more unpredictable or where late-autumn breaks are more common.
Comparison of forage brassica (blue) and forage wheat (orange) potential annual metabolisable-energy (ME) yield in response to sowing date across temperate, cool season wet (a–e), wet Mediterranean (f–h), dry Mediterranean (i–n), temperate, subhumid (o–s), and subtropical, subhumid (t–v) environments in Australia’s crop–livestock farming zone.
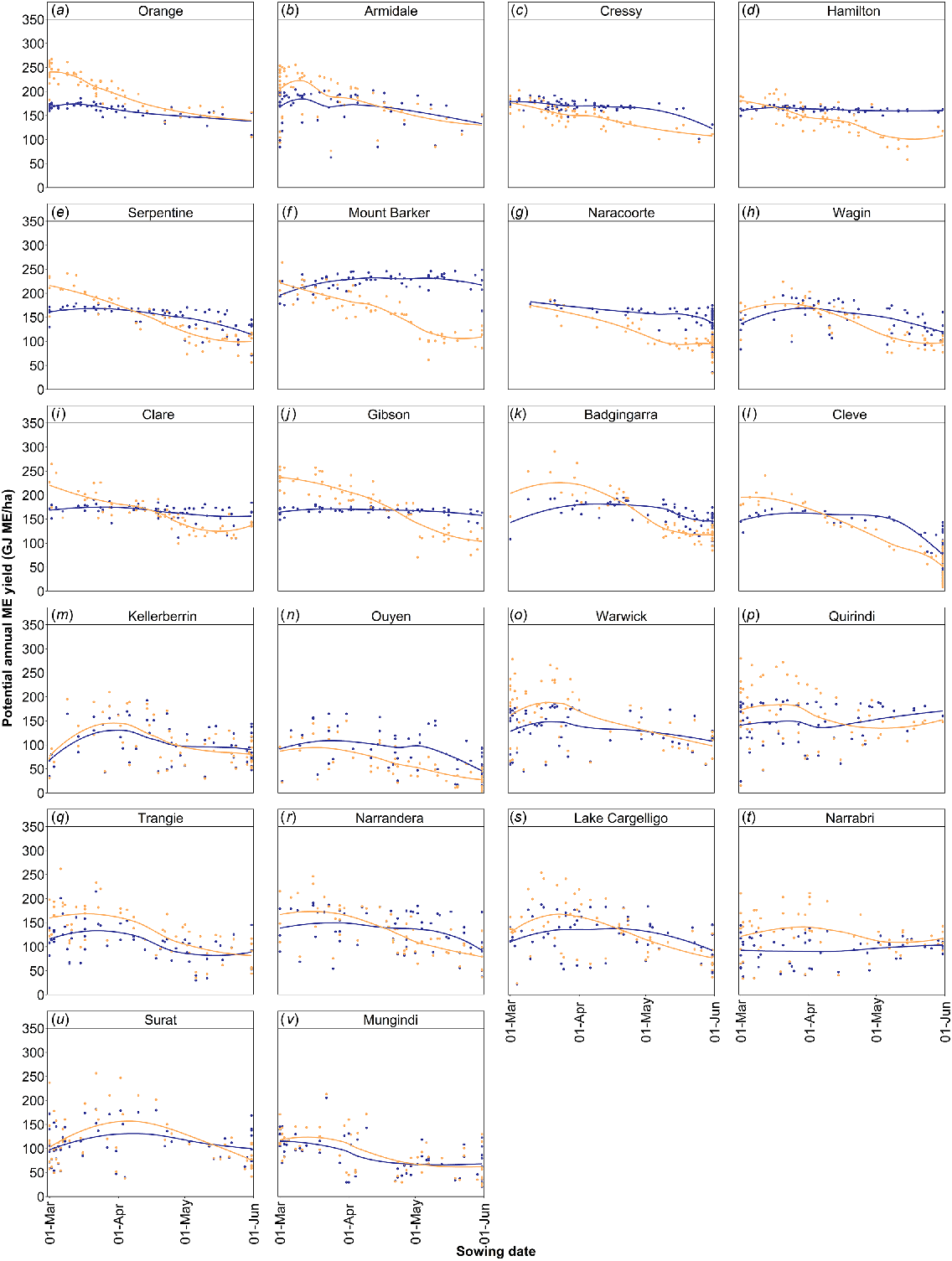
Whole-farm feed base analysis
For the seven simulated regions, there were significant differences in the impacts of integrating autumn-sown forage brassicas into the farm feed base. At five of the seven regions, adding forage brassicas to 15% of the farm forage area reduced the frequency of farm feed deficits by 35–50% (Table 3). In these regions, the frequency that edible biomass fell below a threshold of 500 kg/ha was also reduced by 20–40% (Table 3). This reduction in the frequency of feed gaps occurred because of both an increase in total forage production offered by the forage brassicas compared with the simulated baseline pasture feed base (ranging from 20% to 35% more GJ ME/ha.year across these regions), as well as changing the timing of forage supply (discussed below). Much smaller benefits from the addition of forage brassicas to the feed base were predicted in south-west Victoria and Mallee Victoria, with the frequency of feed-gaps reduced by only 10–20%. The predicted increase in total productivity of the feed base was also smaller at these two regions (~15%).
Region | ||||||||
---|---|---|---|---|---|---|---|---|
Maranoa, Qld | Central west, NSW | Riverina, NSW | South-west, Vic. | Mallee, Vic. | Mid-north, SA | Great Southern, WA | ||
Farm feed deficit frequency (i.e. feed supply insufficient to meet livestock demand) | ||||||||
Baseline | 16.1 | 19.3 | 13.8 | 4.1 | 17.8 | 7.7 | 13.4 | |
+forage brassica | 8.9 | 12.4 | 8.8 | 3.6 | 14.4 | 3.9 | 6.9 | |
+forage cereal | 8.1 | 12.3 | 9.8 | 3.4 | 16.5 | 2.3 | 9.5 | |
+both | 7.9 | 10.3 | 8.7 | 3.8 | 14.7 | 2.0 | 5.7 | |
Available edible biomass falls below 500 kg/ha | ||||||||
Baseline | 48 | 55 | 40 | 20 | 51 | 33 | 42 | |
+forage brassica | 33 | 43 | 32 | 17 | 47 | 28 | 34 | |
+cereal | 35 | 41 | 35 | 17 | 50 | 27 | 37 | |
+both | 28 | 37 | 30 | 17 | 47 | 25 | 33 | |
Average feed base productivity (t DM/ha.year) | ||||||||
Baseline | 4.3 | 3.6 | 6.2 | 9.6 | 4.5 | 6.9 | 6.6 | |
+forage brassica | 4.9 | 4.4 | 6.9 | 10.2 | 4.9 | 8.0 | 7.4 | |
+forage cereal | 5.0 | 4.6 | 6.8 | 10.0 | 4.6 | 7.8 | 7.3 | |
+both | 5.6 | 5.2 | 7.4 | 10.5 | 4.9 | 8.7 | 8.0 | |
Average feed base productivity (GJ ME/ha.year) | ||||||||
Baseline | 37 | 30 | 53 | 74 | 37 | 58 | 59 | |
+forage brassica | 47 | 41 | 64 | 86 | 44 | 74 | 72 | |
+forage cereal | 46 | 43 | 61 | 82 | 40 | 71 | 70 | |
+both | 54 | 51 | 70 | 92 | 46 | 84 | 80 |
The stocking rate per grazed ha (i.e. including all pastures and forage crops grazed) is held constant in all scenarios.
In most regions, both forage brassicas and forage cereals grown under similar management achieved similar impacts on reducing feed gaps in the respective livestock enterprises. At a few regions (e.g. Riverina NSW, Mallee Victoria, and Great Southern WA), there was some small advantage of the forage brassicas over the forage cereals for reducing the frequency of farm feed deficits, often owing to the higher nutritive value of the forage brassica crops in spring. In four of the seven simulated regions, there were also complementary benefits of having a combination of both forage brassicas and forage cereals (Table 3).
Despite large differences in seasonality of growth of the baseline pasture feed base across the different simulated regions, and hence timing and frequency of feed deficits, the incorporation of forage brassicas (and forage cereals) consistently reduced late autumn to late spring feed gaps across all regions. This benefit was greatest in regions with frequent feed deficits at that time of the year (e.g. Maranoa Qld; Fig. 5a), but even at other regions where winter and spring feed gaps were less common, there were significant reductions in the frequency of farm feed deficits over that period (e.g. Riverina NSW, Mallee Victoria; Fig. 5c, d). In regions with a Mediterranean climate (i.e. Great Southern WA and mid-north SA, Fig. 5e, f), a surprising outcome from model predictions was the extent that feed deficits over late summer and autumn were mitigated by the addition of winter-growing forage brassicas to the farm feed base. In these regions, livestock grazing over the summer often rely on dry pasture residue grown during the previous spring, and even though the forage brassica did not directly produce forage over summer, its addition meant that more forage was carried over and then made available over the summer period. In both Victorian regions, autumn-sown brassicas very rarely benefitted the farm feed base because forage deficits rarely occurred from late autumn (May) to spring and brassicas did not effectively mitigate the regular feed deficits that occur in early autumn (Fig. 5g).
Frequency of predicted farm feed deficits throughout the year over 50 years (1960–2010) under the baseline pature-only feed base (black), or when 15% (by area) of forage brassica (blue) or 15% of forage cereal (orange), or a combination of both (15% of each to total of 30%) (red), are added to the farm feed base at seven regions (a–g) spanning Australia’s mixed crop–livestock farming zone.
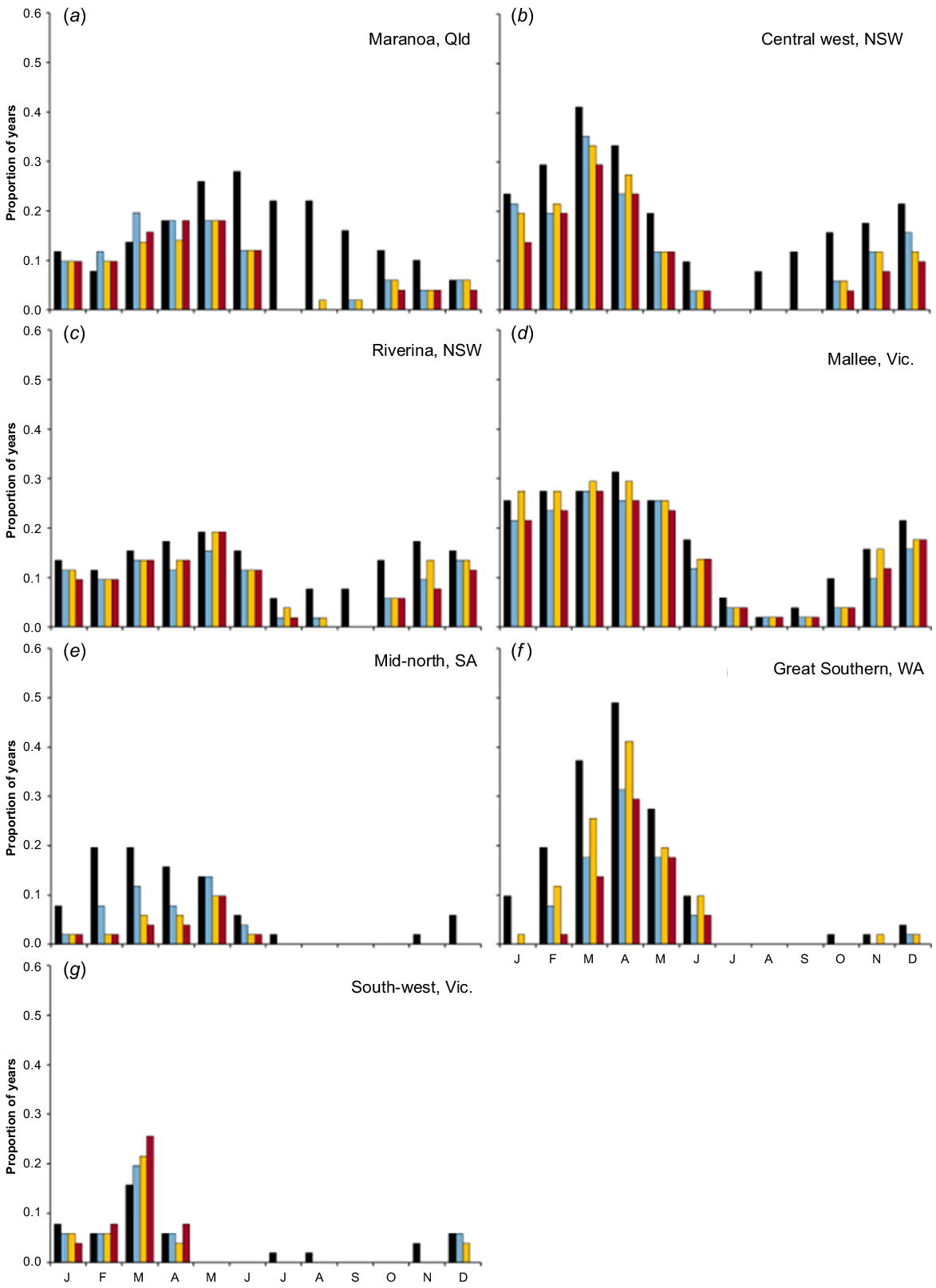
One benefit of forage brassicas is to reduce feed deficits and, hence, supplementary feed requirements, or alternatively, they could offer potential to increase stocking rates while maintaining the same feed deficit risk. This analysis predicts that a feed system where 15% of the grazed area is allocated to forage brassicas has the potential to increase stocking rate by 10–30%, depending on the region (Table 4). As above, the least benefits were obtained in south-west Victoria, but large increases in safe stocking rates (>20%) could be achieved in regions with summer-dominant rainfall (e.g. Maranoa Qld, and central west NSW) or Mediterranean environments (e.g. Mid-north SA, and Great Southern WA).
Region | Baseline | +15% forage brassica | %Change | |
---|---|---|---|---|
Maranoa, Qld | 0.35 | 0.45 | 28 | |
Central west, NSW | 1.56 | 2.00 | 28 | |
Riverina, NSW | 2.74 | 3.19 | 16 | |
South-west, Vic. | 3.81 | 4.17 | 9 | |
Mallee, Vic. | 1.87 | 2.15 | 15 | |
Mid-north, SA | 2.92 | 3.63 | 24 | |
Great Southern, WA | 3.33 | 4.45 | 33 |
Discussion
In this study, we have shown that simulated productivity potential of forage brasicas varied considerably (range of 7–19 t DM/ha) across 22 locations within diverse agro-climatic environments spanning Australia’s crop-livestock farming zone, with productivity being highest in environments with a long growing season associated with winter-dominant rainfall patterns, higher annual rainfall (>600 mm) and lower aridity. Despite these large differences in productivity potential, feed base analysis for seven diverse livestock production systems showed that integrating forage brassicas into the farm feed base may consistently reduce feed gaps over late autumn to late spring, especially in those environments that experience frequent feed deficits at that time of the year, such as subtropical, subhumid environments. This could help mitigate farm risk and improve total farm productivity via reduced supplementary feed cost and stable stocking density in poorer seasons.
Drivers of forage brassica productivity
In-crop rainfall and seasonal aridity that influence water availability and the length of growing season were critical drivers of forage brassica production potential. Autumn-sown forage brassicas were predicted to be most productive and reliable in environments with a longer growing season and lower aridity, such as wet Mediterranean, and temperate, cool season wet environments, where they could be sown early (March to early April) under optimal available soil water and rainfall conditions and encountered a mild spring that allowed them to grow into early summer. These conditions are typically where forage brassicas are already commonly used, but where they are most often sown in spring to provide forage of high nutritive value in late summer and autumn (Lindsay et al. 2007; Nie et al. 2020). Total biomass production of spring-sown forage rape crops grown in similar temperate environments can range from ~3.0 to 9.0 t DM/ha, depending on the length of the growing season (de Ruiter et al. 2009; Pembleton et al. 2013), which is much lower than what we have predicted here (~14 t DM/ha). Previous simulations of spring-sown forage brassicas in wet Mediterranean and temperate, cool season wet environments have also produced lower long-term predictions of biomass production (4.5–6.0 t DM/ha) (Pembleton et al. 2016). We have shown that sowing forage brassicas in autumn rather than spring in these environments has the potential for higher overall production potential via an extended growing season and this alternative use pattern may have considerable productivity benefits for livestock grazing systems.
Drier environments within the crop–livestock farming zone, such as those with a dry Mediterranean, temperate subhumid, and subtropical subhumid climate, represent new potential areas for forage brassicas and, as such, there is limited comparative data. We showed in this simulation study that forage brassicas grown in dry Mediterranean environments with a lower annual rainfall (<400 mm) and higher aridity were often sown under lower available soil water and rainfall conditions that resulted in a forced sowing event at the end of May. The later sowing along with water limitations from the lower overall mean growing-season rainfall (i.e. <300 mm) contributed to the lower and much more variable predictions of total production potential (Fig. 2) than in the wetter temperate environments. Forage brassicas grown in subtropical, subhumid environments also had lower and more variable total production potential, although this was more an artefact of the low and more variable winter–spring rainfall over the growing season and overall higher aridity, which is typical for this environment. Data of forage rapes and raphanobrassica grown in field experiments under Decile 1 and 2 conditions (Watt et al. 2021) closely matched the lower end of our total production predictions for dry Mediterranean (~3.0–4.5 t DM/ha; comparable to Kellerberrin, WA) and subtropical, subhumid environments (~3.6–9.2 t DM/ha; comparable to Warwick, Qld). Our simulations showed that in more favourable seasons, greater total production is possible in these drier environments than what has been demonstrated in field experiments.
Although we showed that predictions of total production potential were generally greatest at locations where earlier sowing opportunities occurred, total production potential of the forage brassicas for each location did not tend to vary with sowing date, as was the case for the forage wheat (Fig. 4). Thus, time of sowing was not considered a critical driver of total productivity potential among environments. This contrasts to dual-purpose canola where the grazing period is limited by the onset of reproductive development, and so total grazing provided is strongly driven by the period from sowing to this time of the year (Lilley et al. 2015). For forage brassicas that offer a longer grazing window, climatic conditions that influenced water availability at sowing and during the growing season into spring (including both rainfall and evaporative demand) were considered more critical drivers of overall production.
Filling feed gaps to support livestock systems
We showed from our predictions and whole-farm feed base analysis that forage brassicas have considerable potential to fill critical feed gaps and serve as an alternative to other forages (e.g. forage cereals) across a broad range of environments. A significant driver that contributes to this potential is the much wider and more stable sowing window of forage brassicas than of forage wheat, with the ability to produce higher or similar yields of ME at later sowing dates (late April to early May; Fig. 4). Forage brassicas can also maintain nutritive value for longer than do forage cereals, because they are slower to reach maturity (i.e. initiate and reach reproductive development) (Barry 2013; Watt et al. 2021). They are especially advantageous when compared with shorter-season forage cereal cultivars that are faster to mature, and, hence, have a shorter grazing window than do longer-season winter cultivars (Dove and Kirkegaard 2014; Lilley et al. 2015).
We have shown that at locations with regular early sowing opportunities (e.g. Orange or Armidale; Fig. 3a, b), longer-season forage cereal cultivars produced greater potential annual ME yield than did forage brassicas. This was supported by the results of our whole-farm feed base analysis, that showed for these temperate, cool season wet environments (e.g. south-west Vic.), integration of forage brassicas alone results in a marginally higher frequency of farm feed deficits than for forage cereals. In seasons or environments where later sowing and use of shorter-season forage cereal cultivars is more likely (e.g. Clare, Badgingarra, Cleve, Kellerberrin, or Ouyen; Fig. 3i, k–n), forage brassicas may offer an advantage to better fill feed gaps. We showed from our whole-farm feed base analysis that in these dry Mediterranean environments (e.g. Mallee Vic. region), forage brassicas may also alter the distribution of feed, filling feed gaps over the winter–spring period, through to summer and early autumn, and allow other on-farm forage sources to be spelled and used in later seasons when pasture supply would normally be limited. (Note: the farm feed base calculator does account for decline in nutritive value and loss of biomass that would occur if forages were deferred and used later). In contrast, integration of a short-season forage cereal crop in these drier environments may help fill the winter feed gap, but the lower total biomass potential and shorter grazing window provide little to no benefit to the farm feed base in summer and early autumn when pastures are also limited in supply (Fig. 5d).
Because forage brassicas have a wider sowing window, this also provides an opportunity for them to be used as a complementary crop with other forages (e.g. cereals). Systems that integrated both forage brassicas and forage cereals had a greater average feed base productivity than did sowing a single forage crop, although the magnitude of these benefits was highly variable among environments. We showed that this complementary benefit is greatest in temperate, subhumid environments (e.g. Trangie NSW region), where there are more opportunities for sowing long-season forage wheat and forage brassicas that together contribute to a bulk of feed as well as the deferment of pasture grazing for later use. Similar complementary effects of pasture deferment and benefits to whole-farm grazing potential are afforded by dual-purpose canola and cereals in these environments (Bell et al. 2015b; Dove et al. 2015; Watt et al. 2023). Although we did not consider staggered sowing dates for these autumn-sown crops in our analysis, in environments where long-season forage cereals are more likely to be sown, staggered sowing of these crops may have greater complementary benefits than what we have shown here via a consistent supply of high nutritive-value feed across winter, spring and early summer with improved forage utilisation rates.
Complementary systems with forage brassicas and cereals are less likely to provide benefits in dry Mediterranean environments that receive <350 mm of annual rainfall and where short-season forage cereals are typically sown (e.g. Mallee Vic. region). Hot, dry summers, which are typical for this environment, also increase the risk of a ‘crop-penalty’ that is more likely to occur when short-season forage cereals are used. In environments where the sowing of multiple autumn-sown forage crops presents this risk, sowing fewer paddocks with a mixture of forage brassica and forage cereals with different growth patterns may help mitigate feed gaps, without reducing pasture area for grazing over the late-summer and early autumn months.
In addition to feed base productivity benefits, we also showed that integration of forage brassicas can also allow for a safe increase in stocking rates. Although this increase in stocking rate is unlikely to translate into a direct gain in farm profit because of other associated costs, it does show that increases in returns of livestock enterprises of >10% are likely to be achievable using forage brassicas. However, as for dual-purpose crops, once a sufficient proportion of the farm has been allocated to fill the limiting winter feed-gap period, the benefits of additional area are likely to decline as they replace other forage sources critical at other times of the year (Bell et al. 2015b). In this analysis, the proportional allocation that optimised their benefit to the farm feed system was not explored. This is likely to vary substantially across farm systems with different livestock enterprises, stocking intensities, feed base constituents, and production environments.
Uncertainties modelling forage brassicas in farming systems
Our predictions of potential biomass production indicated that forage brassicas reduce winter feed gaps and offer a longer grazing period that extends into spring and early summer. Because of the longer grazing window, forage brassicas have more total grazing potential than do forage cereals and dual-purpose canola that have been increasingly adopted in the crop–livestock farming zone. However, the simulations here may either over- or under-estimate the amount of grazing and biomass achievable by these crops, because the forage models in APSIM are not currently well calibrated to simulate the regrowth under grazing, and thus the effect of repeat defoliation on crop regrowth. Hence, the impact of grazing on the total potential biomass production is unknown and requires further research and refinement. Furthermore, some forage brassica cultivars, such as raphanobrassica, are known to have superior regrowth potential compared with other brassicas (Dumbleton et al. 2021). These differences are currently not captured in our current model framework and so the implications of these cultivar attributes on continuity of feed supply are not evident from our simulations. Further data to validate models to capture regrowth after grazing and crop nutritive value, particularly crude protein (Watt et al. 2022), would further help guide decisions on ideal cultivars, optimal grazing management and animal growth response to allow more precise estimates of the relative value of different forage brassicas within diverse environments and production systems. Improving these regrowth processes in the model would enable for a more dynamic whole-farm systems modelling approach to be applied, rather than the simple feed-balance analysis conducted here. These more complex analyses could better capture the intricacies in regrowth patterns of the different forages throughout the year under different grazing management strategies and their potential interactions with different livestock enterprises.
Our estimates of potential forage brassica production are likely to be higher than what may be achieved on farm for several reasons. Crop nutrition and agronomic management is likely to play a significant role in dictating forage brassica production under farm practice, whereas here we have simulated crops under optimal agronomic management, and production is limited only by water availability. Although we have simulated situations with adequate N supplied, in the real world, crop response to N fertilisation and N availability are likely to vary across environments, soil types and growing seasons. Hence, the economic decisions that influence N application are also likely to vary, especially between high- and low-production environments. Higher-rainfall environments with higher production potential are likely to induce a higher N demand and responses, whereas in low-production environments responses to N are likely to be lower and more variable and, hence, forage crops are often managed under low-input conditions for economic reasons. Other agronomic aspects such as sowing rate/plant density and sowing dates are also likely to require further exploration to optimise forage brassica production relative to inputs and the production environment. Further on-farm testing is needed across environments to better understand optimal agronomic management (e.g. fertiliser requirements and responses) and to explore how brassicas are used in different ways in the system (e.g. in multi-species mixtures).
Conclusions
While forage brassicas are widely accepted and used to fill feed gaps in intensive livestock systems in high-rainfall temperate environments, this long-term simulation modelling across Australia’s crop–livestock farming zone adds further evidence that they can provide a reliable and valuable contribution to forage systems across a diversity of growing conditions experienced in these drier environments. Autumn-sown brassicas can complement the existing feed base in a range of livestock production systems, helping reduce the size and frequency of feed deficits in situations where this occurs regularly in winter and spring, while also providing legacy benefits of more residual forage available into summer. This can reduce the need for supplementary feed or allow increased livestock productivity without increasing the risk. Forage brassicas allow greater flexibility, with a long grazing window and stable production over a range of sowing dates, providing advantages over traditionally used forage cereals in environments with unpredictable winter rainfall and late-autumn breaks.
Data availability
The data that support this study will be shared upon reasonable request to the corresponding author.
Declaration of funding
This paper was prepared during a Meat and Livestock Australia Donor Co. project (P.PSH.1044: Improving the use of forage brassicas in mixed farming systems).
Acknowledgements
Authors thank Tiemen Rhebergen from CSIRO, Agriculture and Food, for his feedback on the draft manuscript.
References
Barry TN (2013) The feeding value of forage brassica plants for grazing ruminant livestock. Animal Feed Science and Technology 181, 15-25.
| Crossref | Google Scholar |
Bell LW, Robertson MJ, Revell DK, Lilley JM, Moore AD (2008) Approaches for assessing some attributes of feed-base systems in mixed farming enterprises. Australian Journal of Experimental Agriculture 48, 789-798.
| Crossref | Google Scholar |
Bell LW, Harrison MT, Kirkegaard JA (2015a) Dual-purpose cropping-capitalising on potential grain crop grazing to enhance mixed-farming profitability. Crop & Pasture Science 66, i-iv.
| Crossref | Google Scholar |
Bell LW, Dove H, McDonald SE, Kirkegaard JA (2015b) Integrating dual-purpose wheat and canola into high-rainfall livestock systems in south-eastern Australia. 3. An extrapolation to whole-farm grazing potential, productivity and profitability. Crop & Pasture Science 66, 390-398.
| Crossref | Google Scholar |
Bell LW, Moore AD, Thomas DT (2018) Integrating diverse forage sources reduces feed gaps on mixed crop–livestock farms. Animal 12, 1967-1980.
| Crossref | Google Scholar | PubMed |
Bell LW, Watt LJ, Stutz RS (2020) Forage brassicas have potential for wider use in drier, mixed crop–livestock farming systems across Australia. Crop & Pasture Science 71, 924-943.
| Crossref | Google Scholar |
CSIRO (2020) SoilMapp for iPad: soil information at your fingertips. Available at https://www.csiro.au/soilmapp
Department of Agriculture, Water and the Environment (2020) Interim biogeographic regionalisation for Australia v. 7 (IBRA) [ESRI shapefile]. Available at https://www.environment.gov.au/fed/catalog/search/resource/details.page?uuid=%7B4A2321F0-DD57-454E-BE34-6FD4BDE64703%7D
Dove H, Kirkegaard J (2014) Using dual-purpose crops in sheep-grazing systems. Journal of the Science of Food and Agriculture 94, 1276-1283.
| Crossref | Google Scholar | PubMed |
Dove H, Kirkegaard JA, Kelman WM, Sprague SJ, McDonald SE, Graham JM (2015) Integrating dual-purpose wheat and canola into high-rainfall livestock systems in south-eastern Australia. 2. Pasture and livestock production. Crop & Pasture Science 66, 377-389.
| Crossref | Google Scholar |
Dumbleton A, Foley F, Westwood CT, Box GM (2021) The development of Pallaton Raphanobrassica for New Zealand farming systems. Journal of New Zealand Grasslands 83, 107-114.
| Crossref | Google Scholar |
Grundy MJ, Rossel RAV, Searle RD, Wilson PL, Chen C, Gregory LJ (2015) Soil and landscape grid of Australia. Soil Research 53, 835-844.
| Crossref | Google Scholar |
Holzworth DP, Huth NI, deVoil PG, Zurcher EJ, Herrmann NI, McLean G, Chenu K, van Oosterom EJ, Snow V, Murphy C, Moore AD, Brown H, Whish JPM, Verrall S, Fainges J, Bell LW, Peake AS, Poulton PL, Hochman Z, Thorburn PJ, Gaydon DS, Dalgliesh NP, Rodriguez D, Cox H, Chapman S, Doherty A, Teixeira E, Sharp J, Cichota R, Vogeler I, Li FY, Wang E, Hammer GL, Robertson MJ, Dimes JP, Whitbread AM, Hunt J, van Rees H, McClelland T, Carberry PS, Hargreaves JNG, MacLeod N, McDonald C, Harsdorf J, Wedgwood S, Keating BA (2014) APSIM – Evolution towards a new generation of agricultural systems simulation. Environmental Modelling & Software 62, 327-350.
| Crossref | Google Scholar |
Hutchinson MF, McIntyre S, Hobbs RJ, Stein JL, Garnett S, Kinloch J (2005) Integrating a global agro-climatic classification with bioregional boundaries in Australia. Global Ecology and Biogeography 14, 197-212.
| Crossref | Google Scholar |
Jeffrey SJ, Carter JO, Moodie KB, Beswick AR (2001) Using spatial interpolation to construct a comprehensive archive of Australian climate data. Environmental Modelling & Software 16, 309-330.
| Crossref | Google Scholar |
Lilley JM, Bell LW, Kirkegaard JA (2015) Optimising grain yield and grazing potential of crops across Australia’s high-rainfall zone: a simulation analysis. 2. Canola. Crop & Pasture Science 66, 349-364.
| Crossref | Google Scholar |
Moore AD, Bell LW, Revell DK (2009) Feed gaps in mixed-farming systems: insights from the Grain & Graze program. Animal Production Science 49, 736-748.
| Crossref | Google Scholar |
Nie ZN, Slocombe L, Behrendt R, Raeside M, Clark S, Jacobs JL (2020) Feeding lambs proportional mixtures of lucerne (Medicago sativa) and forage brassica (Brassica napus) grown under warm and dry conditions. Animal Production Science 61, 1181-1188.
| Crossref | Google Scholar |
Pembleton KG, Rawnsley RP, Jacobs JL, Mickan FJ, O’Brien GN, Cullen BR, Ramilan T (2013) Evaluating the accuracy of the Agricultural Production Systems Simulator (APSIM) simulating growth, development, and herbage nutritive characteristics of forage crops grown in the south-eastern dairy regions of Australia. Crop & Pasture Science 64, 147-164.
| Crossref | Google Scholar |
Pembleton KG, Cullen BR, Rawnsley RP, Harrison MT, Ramilan T (2016) Modelling the resilience of forage crop production to future climate change in the dairy regions of southeastern Australia using APSIM. The Journal of Agricultural Science 154, 1131-1152.
| Crossref | Google Scholar |
Unkovich, M (2010) A simple, self-adjusting rule for identifying seasonal breaks for crop models. In ‘Security from Sustainable Agriculture. Proceedings of the 15th Australian Agronomy Conference’, Lincoln, New Zealand. (Eds H Dove, R Culvenor). (Australian Society of Agronomy Inc.: Lincoln, New Zealand)
Watt LJ, Bell LW, Cocks BD, Swan AD, Stutz RS, Toovey A, De Faveri J (2021) Productivity of diverse forage brassica genotypes exceeds that of oats across multiple environments within Australia’s mixed farming zone. Crop & Pasture Science 72, 393-406.
| Crossref | Google Scholar |
Watt LJ, Bell LW, Pembleton KG (2022) A forage brassica simulation model using APSIM: model calibration and validation across multiple environments. European Journal of Agronomy 137, 126517.
| Crossref | Google Scholar |
Watt LJ, Bell LW, Herrmann NI, Hunt PW (2023) Integrating dual-purpose crops mitigates feedbase risk and facilitates improved lamb production systems across environments: a whole-farm modelling analysis. Animal Production Science 63, 782-801.
| Crossref | Google Scholar |