Ability of wheat genotypes to form large rhizosheaths may enhance survival of false-break events in rainfed production
Livinus Emebiri
A
Abstract
Crop production is one of the agricultural sectors most vulnerable to climate change. In order to minimise risks, innovative technologies and management strategies are continually being developed. Early sowing is a strategy used in wheat production; however, with irregular rainfall patterns, false breaks can occur.
We sought to determine the optimal volume of autumn-break rainfall for the start of season, and whether formation of a larger rhizosheath (i.e. the thick layer of soil adhering to roots) can buffer wheat seedlings from false-break conditions, thereby keeping plants alive until the next rainfall.
In glasshouse experiments, six varieties of common wheat (Triticum aestivum L.), two with contrasting rhizosheath-forming ability and the other four untested, were grown on two soil types (Kandosol and Vertosol) under simulated autumn-break rainfall and false-break durations. Rhizosheath size and plant establishment traits were measured.
The ability to form large rhizosheaths explained ~80% of the variability in crop establishment under various scenarios of false-break conditions. Comparative growth performance of the cultivars forming the largest (cv. Flanker) and smallest (cv. Westonia) rhizosheaths showed that they were largely similar for the first 28 days of drought but differed significantly thereafter. Flanker was progressively better able to establish as false-break duration increased and showed significantly greater tiller and leaf production than Westonia.
We demonstrate that genotype selection for formation of large rhizosheaths can help to reduce plant seedling death under false-break conditions.
Although the amount of starting rainfall is critical, cultivars differ significantly with regard to how far they can develop without follow-up rain. Future research is suggested for a field-scale study of the potential for selection for larger rhizosheaths to improve wheat crop establishment under harsh conditions.
Keywords: climate variability, crop establishment, drought tolerance, false-break, genetic evaluation, rhizosheaths, Triticum aestivum, wheat.
Introduction
Common (bread) wheat (Triticum aestivum L.) is the second most widely cultivated crop in the world and, consequently, is pivotal to global food security. In Australia, the growing season of wheat extends from April to the end of October (Pook et al. 2009), with the crop sown after the first significant rain events (known as the autumn break). The autumn break is one of the most anticipated events of the year for a grower and has an immeasurable impact on their confidence and psyche (Smith 2022). The ideal autumn break occurs when a rainfall of 25–30 mm is received over a period of 3–7 days or less; however, since the mid 1990s, occurrence of the ideal break has continued to decline, and this is accompanied by a trend towards breaks happening later in the season (Pook et al. 2009). Some growers, particularly in drier regions, now sow crops in response to a rainfall event that is less than ideal for their location. For crops that are exclusively rainfed, a risk is the occurrence of ‘false breaks’, where the first rains provide sufficient moisture to initiate germination, but this is followed by a period of drought, leading to the widespread death of establishing seedlings (Chapman and Asseng 2001). False-break events in Australia are estimated to occur in two of every three cropping seasons (Chapman and Asseng 2001; Pook et al. 2009), and the risks associated are said to be higher in minimum- or no-till systems (Smith 2022).
Rhizosheaths are the thick layer of soil adhering to roots when wheat seedlings are gently dug up (see Fig. 1b). They were first described by Volkens (1887) on grasses from the Egyptian desert; later, Price (1911) demonstrated their occurrence on several perennial grass species native to the sandy deserts of South Africa. Rhizosheaths are a feature of xerophytic grasses and are thought to increase the efficiency of moisture absorption by roots and reduce desiccation during periods of drought (Price 1911; Hartnett et al. 2013). They have since been recorded on the roots of many crop species, but not all angiosperms (Brown et al. 2017), and have been recorded on roots of wheat (Goodchild and Myers 1987; Young 1995; Rabbi et al. 2022), barley (Hordeum vulgare L.; George et al. 2014; McDonald et al. 2018), foxtail millet (Setaria italica (L.) P.Beauv.; Liu et al. 2019a), maize (Zea mays L.; Watt et al. 1994; Adu et al. 2017), and rice (Oryza sativa L.; Zhang et al. 2020). Multiple research reviews have highlighted the distinct properties of rhizosheaths compared with bulk soil, such as 66% higher water content, 34% higher soil organic carbon, 56% higher water-extractable organic carbon and 71% higher microbial biomass, and their probable role in water and nutrient uptake (Lynch et al. 2014; Brown et al. 2017; Basirat et al. 2019; Ndour et al. 2020; Aslam et al. 2022; Cheraghi et al. 2023; Mo et al. 2023).
(a) PVC tube pots (10 cm by 30 cm) packed to a bulk density of 1.3 g/cm3 by placing a calculated amount of soil in each pot (~2.8 kg); pots are lined with a transparent bag to keep water in the soil. (b) Rhizosheaths adhering to roots when wheat seedlings are gently dug up.
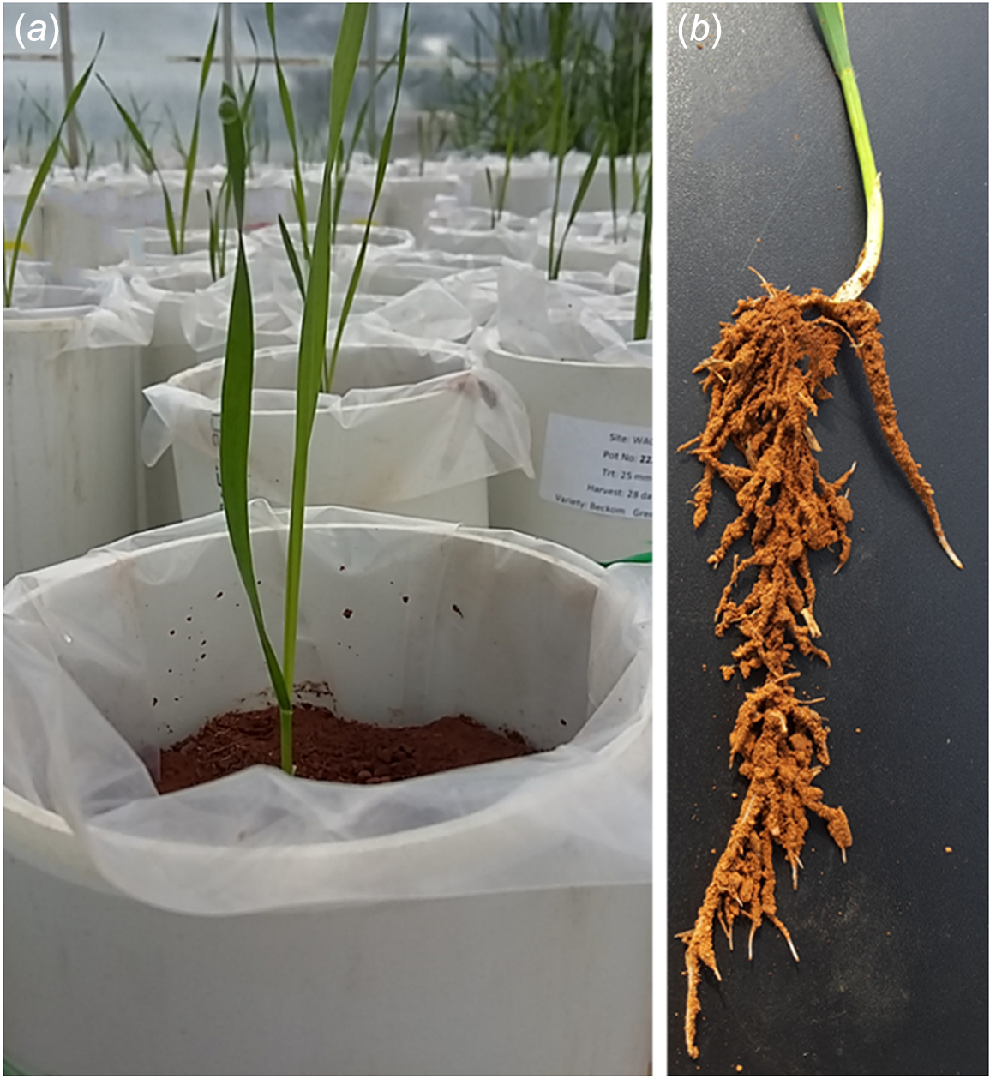
Rhizosheaths form naturally by interactions between exudates from roots and unique colonies of micro-organisms that use the exudates as a carbon source (Watt et al. 1993; Galloway et al. 2020). Formation of rhizosheaths is enhanced by drought (Liu et al. 2019b; Zhang et al. 2020), and there are suggestions that they may aid in seedling establishment under harsh conditions (Bailey and Scholes 1997). Here, we hypothesised that large rhizosheaths may have potential to buffer wheat seedlings during extended false-break conditions and to keep plants alive until the next rainfall. To test this, a glasshouse experiment was performed by growing wheat under simulated autumn-break rainfall and false-break durations. The specific objectives were to determine the optimal volume of autumn-break rainfall for the start of season, and whether rhizosheath formation can buffer wheat seedlings from false-break conditions to keep plants alive until the next rainfall.
Materials and methods
Six cultivars, selected from preliminary studies and comprising a line with high rhizosheath-forming ability (Halberd), a line with low rhizosheath-forming ability (Westonia), and four untested, commercial varieties relevant to major cropping regions in Australia (Mace, Scepter, Beckom, LongReach Flanker), were used in the experiments. Good-quality seeds, free of any visible damage, were sourced. The seeds were pre-germinated in a Petri dish containing filter paper soaked in distilled water and only those at Zadoks Growth Stage 7 (GS07; three roots plus 2–3 mm emerged shoot) were used (Zadoks et al. 1974).
Soil preparation
Two soil types were used in glasshouse experiments. The first was a Kandosol (Isbell and National Committee on Soil and Terrain 2021) sourced from Wagga Wagga Agricultural Institute (35.05 °S, 147.35 °E), New South Wales, Australia, by collecting topsoil to 10 cm depth. The soil was a sandy clay loam with a reddish colour, and field capacity was estimated as 21.3%. The second soil was a Vertosol sourced from Leeton Field Station (34.36 °S, 146.21 °E), New South Wales, by similarly collecting topsoil to 10 cm depth. The soil was greyish in colour, with field capacity estimated as 31.2%. Key physical and chemical characteristics of the soils are summarised in Table 1.
Soil characteristics | Kandosol | Vertosol | |
---|---|---|---|
Colour | LTBR | GRBR | |
Gravel (%) | 5 | 5–10 | |
Texture | Clay | Clay | |
pH (in CaCl2) | 5.3 | 7.2 | |
pH (in H2O) | 5.9 | 7.9 | |
Ammonium-nitrogen (mg/kg) | 7 | 5 | |
Nitrate-nitrogen (mg/kg) | 22 | 18 | |
Phosphorus (Colwell) (mg/kg) | 62 | 36 | |
Potassium (Colwell) (mg/kg) | 438 | 363 | |
Sulfur (mg/kg) | 8.3 | 7.0 | |
Organic carbon (%) | 1.0 | 0.6 | |
Conductivity (dS/m) | 0.1 | 0.1 |
Soils were sourced from 0–10 mm layer of soils at Wagga Wagga Agricultural Institute and Leeton Field Station.
Water content of the soil was confirmed by gravimetric analysis to be close to zero (0.009%) after drying. Then, the soil was reconstituted to achieve 40% of field capacity, which represents the soil water-holding capacity of a typical farm at the end of summer fallows. Soil reconstitution involved mixing soil (30 kg) in a cement mixer with a known amount of water (2.3 L) to achieve 40% of field capacity. The water was delivered by careful spraying of the dry soil to ensure uniform mixing.
Soil potting and watering
Soils were packed into PVC tube pots (10 cm by 30 cm) (Fig. 1a) to a bulk density of 1.3 g/cm3 by placing ~2.8 kg soil in each pot. The inside surfaces of the pots were lined with a plastic bag, which served to keep water in the soil; at harvest, the top of the bags provided ‘handles’ to lift seedlings out of the pots so that soil could be removed with minimal disturbance to the rhizosheath. Plant growth relied on the inherent soil nutrient content (Table 1), and no additional amendments or inputs were added to the soil mix.
The experiments were performed in a glasshouse (Wagga Wagga Agricultural Institute; latitude −35.05°S, longitude 147.35°E) set up to simulate weather conditions during April–May (mean day temperature 24.5°C, night temperature 9.5°C) under natural light. Commercially available Tinytags (https://www.hdl.com.au/) were used to monitor conditions, including soil temperature, for the duration of the experiments. Pots were arranged in a completely randomised design with a split-split-plot arrangement of treatments and six replications. The main plots comprised rainwater treatments applied to represent various amounts of ‘autumn break’ rain, which marks the start of the cropping season. One day before sowing, the treatment units (pots) were watered with 5, 10, 15 or 25 mm, delivered using a Perimatic GP programmable pump dispenser (Jencons Scientific, Leighton Buzzard, UK). The choice of rainfall amounts was based on the sowing rule of Hochman and Horan (2018), whereby ≥15 mm rain over a 3-day period constitutes a seasonal break in the southern parts of the Australian grain zone; 25 mm was a best-case scenario, and the smaller amounts were chosen to simulate potentially lower rainfall with climate change. No further irrigation was applied. The split plots were ‘false break’ durations of drought after the autumn-break rainfall. According to Chapman and Asseng (2001), a rain-free period of 12 days could induce substantial death of establishing seedlings, and on this basis, the following durations were applied randomly to treatment units: 14, 28, 42 and 56 days after sowing. The wheat cultivars were assigned to the split-split plots.
Traits measured
Traits measured included leaf area, derived as leaf length × width of longest leaf blade, multiplied by 0.835 to correct for leaf shape (Miralles and Slafer 1991). Per cent greenness in the canopy (%GCC) was measured using the Canopeo app (Patrignani and Ochsner 2015). Leaf number and tillering were counted at harvest for each treatment unit, and shoot fresh weight was recorded as the weight of above-ground plant parts. Shoot dry weight was determined after drying above-ground plant parts in a dehydrator at 70°C for 3 days, and the difference between fresh weight and dry weight was taken as a measure of plant water content. Plant survival was measured at the end of the false-break period as the proportion of wilted and necrotised leaf portions per plant.
Rhizosheath measurement
Seedlings were harvested at the end of the simulated false-break period for each treatment unit. At harvest, the plastic bag enclosing the soil in the pot was lifted out. The bag was gently massaged to break up the soil, and the seedling was lifted out and gently shaken by hand until no more bulk soil became detached. For older seedlings it was necessary to comb through the soil with fingers and recover as many of the broken root pieces as possible. The entire seedling (i.e. the shoot, root and adhering soil) was weighed to obtain plant fresh weight. After weighing, the root system was excised at the root/shoot interface, transferred to a 250-mL plastic container containing 200 mL water, and kept overnight to allow soil to be loosened from roots. The roots were then washed clean of the soil, and the containers with soil dried in a dehydrator (70°C) for 48 h and weighed to obtain absolute rhizosheath weight (George et al. 2014) as grams dry soil per plant. Specific rhizosheath weight was also calculated by dividing the dry rhizosheath weight (g) by total root length (cm) of each plant (Marin et al. 2021). To measure total root length, washed root samples were suspended in a small amount of water in a rectangular glass dish with black background. An image was collected in greyscale (600 dpi) using an Epson 1680 scanner (Epson, London, UK). The software WinRHIZO (ver. 4.1) was used to map root samples digitally and calculate root length and diameter. The roots washed clean of the soil were dried with paper towels and weighed to obtain fresh root weights.
Statistical analyses
Statistical analysis of the split-split-plot experiment was performed using the software R (The R Foundation, Vienna, Austria). Analyses were done separately for each soil type, and two outcomes were primarily considered: (1) below the soil for root traits such as root length, root diameter, root fresh weight, and absolute and specific rhizosheath weight; and (2) above the soil with shoot traits such as shoot fresh weight, leaf area, canopy cover (%GCC) and leaf number. The four shoot traits were highly correlated and so, to reduce the number of analyses, the z-score was generated using the first principal component (PC1) in a principal component analysis (PCA) and this provided a single measure of seedling/crop establishment. To satisfy the normality assumption, the ‘bestNormalize’ package in R (Peterson 2021) was used to find the best transformation. We found the Ordered Rank Quantile (ORQ) normalisation to be the best transformation and report the results in the transformed scale (Rohan and Sarmah 2023).
The ‘agricolae’ package (de Mendiburu 2023) in R was used for statistical analysis and significance was determined with the minimum significant difference (MSD), after Bonferroni adjustment. Results are displayed either in tables or in graphical format.
Results
Phenotypic variation for rhizosheath size
Results from balanced analysis of variance, performed separately for each soil type, are presented in Tables 2 and 3. In both soil types, cultivar differences in root density (fresh weight) were significant (P < 0.001), but differences in total root length were significant only in the Kandosol (Table 2). When rhizosheath size was expressed per unit length of root (specific rhizosheath size, g/cm), there was no genotypic variation in either soil type, but when expressed in absolute terms (g/plant), the differences in rhizosheath size were highly significant (P < 0.001) (Tables 2 and 3). Rhizosheath size was therefore quantified using just the absolute values in the present study.
Source of variation | d.f. | Absolute rhizosheath size | Specific rhizosheath size | Root length | Root fresh weight | Crop establishment | |
---|---|---|---|---|---|---|---|
Replication | 5 | 0.39n.s. | 68.75n.s. | 0.37n.s. | 0.23n.s. | 0.03n.s. | |
Rainfall | 3 | 2.45** | 40.48n.s. | 6.79*** | 5.96*** | 16.90*** | |
Error A | 15 | 0.35 | 36.23 | 0.23 | 0.20 | 0.14 | |
FBD | 3 | 129.52*** | 121.60** | 137.24*** | 134.85*** | 135.80*** | |
Rainfall × FBD | 9 | 1.15** | 57.54* | 3.18*** | 2.01*** | 2.88*** | |
Error B | 60 | 0.32 | 28.51 | 0.13 | 0.21 | 0.13 | |
Cultivar | 5 | 3.22*** | 32.32n.s. | 1.93*** | 2.23*** | 1.53*** | |
Cultivar × rainfall | 15 | 0.29n.s. | 41.39n.s. | 0.25n.s. | 0.17n.s. | 0.16n.s. | |
Cultivar × FBD | 15 | 1.20*** | 37.13n.s. | 0.72*** | 0.44** | 0.71*** | |
Cultivar × rainfall × FBD | 45 | 0.33* | 35.38n.s. | 0.25* | 0.48*** | 0.28*** | |
Error C | 400 | 0.22 | 32.96 | 0.16 | 0.19 | 0.12 |
*P ≤ 0.05; **P ≤ 0.01; ***P ≤ 0.001; n.s., not significant (P > 0.05).
Source of variation | d.f. | Absolute rhizosheath size | Specific rhizosheath size | Root length | Root fresh weight | Crop establishment | |
---|---|---|---|---|---|---|---|
Replication | 5 | 0.05n.s. | 580.60n.s. | 7.05n.s. | 0.17n.s. | 0.54n.s. | |
Rainfall | 3 | 127.20*** | 612.20n.s. | 1.42n.s. | 119.50*** | 526.40*** | |
Error A | 15 | 0.30 | 769.40 | 0.97 | 0.20 | 0.59 | |
FBD | 3 | 6.22*** | 878.40n.s. | 0.41n.s. | 19.29*** | 10.97*** | |
Rainfall × FBD | 9 | 4.73*** | 1467.90n.s. | 0.92n.s. | 5.28*** | 9.14*** | |
Error B | 60 | 0.21 | 1293.30 | 0.57 | 0.16 | 0.43 | |
Cultivar | 5 | 1.09*** | 848.80n.s. | 0.83n.s. | 2.11*** | 1.00* | |
Cultivar × rainfall | 15 | 0.40* | 1374.80n.s. | 1.16n.s. | 0.19n.s. | 0.79** | |
Cultivar × FBD | 15 | 0.39* | 1292.40n.s. | 1.16n.s. | 0.18n.s. | 0.86** | |
Cultivar × rainfall × FBD | 45 | 0.31* | 1213.80n.s. | 1.06n.s. | 0.22n.s. | 0.57** | |
Error C | 400 | 0.21 | 1233.20 | 0.97 | 0.18 | 0.35 |
*P ≤ 0.05; **P ≤ 0.01; ***P ≤ 0.001; n.s., not significant (P > 0.05).
For a measure of crop establishment, phenotypic values of above-ground traits across the combinations of cultivar (n = 6), simulated autumn rainfall (n = 4), and false-break duration (n = 4) were first analysed by a multivariate approach (PCA) to define a single measure of seedling establishment. In the Kandosol, PC1 explained 81.4% of the variability and showed high correlations with shoot fresh weight (r = 0.97), leaf area (r = 0.94), %GCC (r = 0.78) and leaf number (r = 0.91). In the Vertosol, PC1 explained 83.6% of the variability, and showed good correlations with shoot fresh weight (r = 0.96), leaf area (r = 0.93), %GCC (r = 0.91) and leaf number (r = 0.86). PC1 was therefore used as a measure of crop establishment (score) on both soil types.
The main effects of replication were not significant in either soil type, but all other main factors were significant as sources of variation (Tables 2 and 3). As expected, the amount of autumn-break rainfall, which marks start of the cropping season, and how long it can last until the next rain (false-break duration) were dominant factors affecting rhizosheath size and crop establishment. Their interaction effects were also highly significant (P < 0.01), which agrees with common knowledge that the effect of a false break varies with the amount of starting rainfall. Summarised across soil types, the results showed that at any given amount of starting rainfall, rhizosheath size increased linearly with age until 42 days, after which it slowed or there was a decline (Fig. 2a). Crop establishment followed a similar trajectory (Fig. 2b) indicating that with adequate starting rain (>5 mm), the plants could survive up to 42 days with no follow-up rain. However, the optimal rainfall for start of the cropping season was 25 mm, with which both rhizosheath formation and crop establishment were maximal after 56 days of drought (Fig. 2b).
Cultivar effects
Although the amount of autumn-break rain and length of false break were the major sources of variation in both soil types (Tables 2 and 3), cultivar effect on rhizosheath formation was also highly significant (P < 0.001), indicating inherent genetic variation in the wheat cultivars. In general, cultivars with large rhizosheaths also showed good establishment ability, and vice versa; for instance, cv. Westonia, which was the lowest ranking for rhizosheath size in both soil types, was also the poorest for crop establishment (Fig. 3). In the Kandosol, the top-ranking cultivar for rhizosheath size was Flanker, which also was the best for crop establishment (Fig. 3); however, in the Vertosol, Flanker was not significantly different from Westonia for rhizosheath size and showed poor establishment (Fig. 3). The top-ranking cultivar for rhizosheath size in the Vertosol was Halberd, and it was among the cultivars with significantly better establishment in that soil type.
Interaction effects
The interaction of cultivar with amount of starting rainfall (autumn break) was not significant in the Kandosol, and only marginally significant in the Vertosol (Tables 2 and 3). However, cultivar × false break interaction terms were significant (P < 0.05) in both soil types, indicating that the cultivars differed with regard to how long they could survive until next rainfall.
A significant three-way interaction of cultivar with rainfall and false-break duration was observed in both soil types (Tables 2 and 3). A plot of the mean values showed that, in the Kandosol, significant cultivar differences for rhizosheath size occurred after 42 days of false break (Fig. 4a). The plot showed further that the top-ranking cultivar, Flanker, and the bottom-ranking, Westonia, were significantly different after 42 days of false break, and irrespective of the amount of starting rainfall, the difference persisted as the false break condition was extended. In the Vertosol, significant cultivar differences in rhizosheath size were observed with the lowest rainfall (5 mm), but this was unrelated to false-break events. There was no significant interaction of cultivar with false-break events until the highest rainfall treatment (25 mm) (Fig. 4b). Again, the top-ranking cultivar, Halberd, retained its rank as the false-break event was extended, even to 56 days, although there were some exceptions. The exceptions were with 15 mm rainfall and at 42 days of false break (Fig. 4b), and these were likely due to errors in phenotyping.
Rhizosheath size in six wheat cultivars grown in (a) Kandosol and (b) Vertosol, under a factorial combination of simulated autumn-break rainfall (n = 4) and false-break durations (n = 4). Values are means ± standard error.
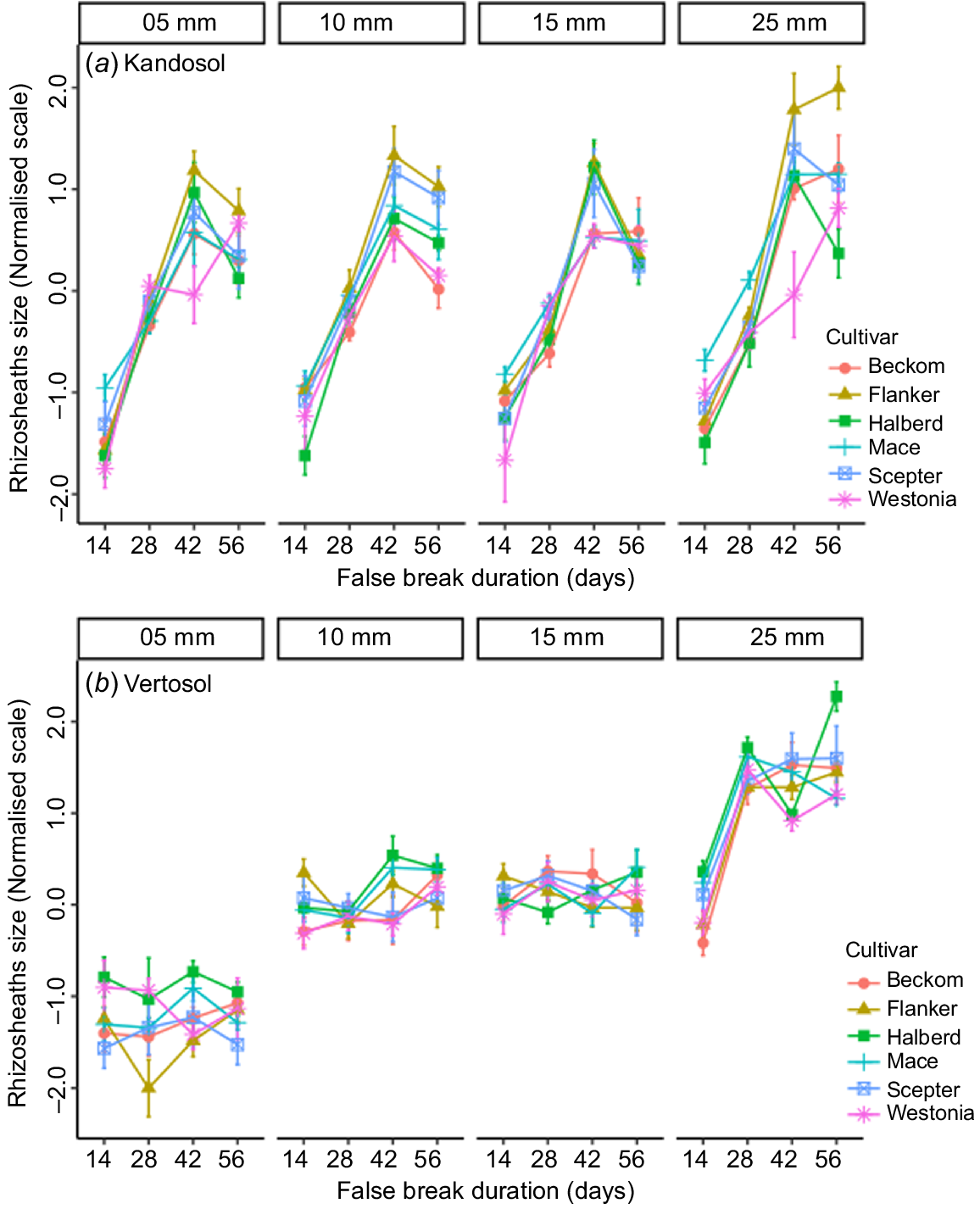
Taken together, the results indicated that the cultivars did not respond differently to the amount of starting rainfall but differed in how long they could survive until the next rainfall. Therefore, we asked whether, in the event of a false break, wheat cultivars with the ability to form large rhizosheaths are better able to survive until follow-up rains.
Relationship with crop establishment
Overall, the relationship of rhizosheath size with crop establishment was positive (R2 = 0.80) and unaffected by soil type, although it was stronger in the Vertosol (R2 = 0.88) than in the Kandosol (R2 = 0.76) (Fig. 5). In the Kandosol, the slope of the regression (b-value) was positive across the various scenarios of autumn-break rainfall amount and false-break duration, and the relationship remained strong (P ≤ 0.05) even after a prolonged dry spell (56 days) (Fig. 5a). The amount of autumn-break rainfall was critical in the Vertosol because some genotypes were able to establish with the minimum amount of starting rainfall (5 mm), whereas 5 mm was not enough for others (Fig. 5b). This is highlighted in Supplementary Fig. S1, presenting seedlings of cv. Scepter grown in the two soil types. With 5 mm rainwater with no follow-up rain for 14 days, seedling root proliferation was restricted in the Vertosol (Fig. S1a) and the only two seminal roots formed were coated with a rhizosheath; however, in the Kandosol, there was greater proliferation of roots, and root hairs provided the seedling with options for water scavenging.
Growth comparison
Two cultivars significantly different for rhizosheath size were chosen for comparison of growth performance traits. Kandosol data were used owing to the better response observed in this soil type. The cultivars with the best (Flanker) and worst (Westonia) rhizosheath formation were largely similar for the first 28 days of drought (Fig. 6), but thereafter, differences in growth performance became apparent. Flanker was progressively better able to establish as false-break duration increased, showing significantly greater tiller and leaf production than Westonia (Fig. 6). Interestingly, the two cultivars did not differ significantly for root growth (root fresh weight) until 56 days of drought, suggesting that the difference in rhizosheath formation may not be related to root growth alone.
Wilting and seedling survival
The ability to survive prolonged false break (>28 days) was further assessed by counting the number of leaves with visible symptoms of wilting, and calculating the proportion of wilted and necrotic (dead) leaf sections per plant. Leaf necrosis was not observed in any of the treatments, but wilting was observed after 56 days of false break. Among the cultivars, differences in the proportion of wilted leaves per plant were statistically significant (P ≤ 0.05), with the highest proportion of wilted leaves observed in Mace (83.4%) and the lowest in Beckom (16.9%). The relationship between rhizosheath size and wilting after 56 days of drought was negative for all cultivars, although the slopes (b-values) varied with cultivar (Fig. 7a), being small for Halberd (−0.15 ± 2.68) and largest for Mace (−4.97 ± 1.93). The results support our hypothesis that a large rhizosheath would be associated with better seedling survival from the effects of declining water availability.
(a) Relationship of rhizosheath weight with leaf wilting after 56 days of false break in six wheat cultivars grown under simulated starting rainfall; comparison of the best and worst rhizosheath-forming cultivars for leaf wilting after 56 days in the (b) Kandosol and (c) Vertosol under different levels of simulated starting rainfall. Values are means ± standard error.
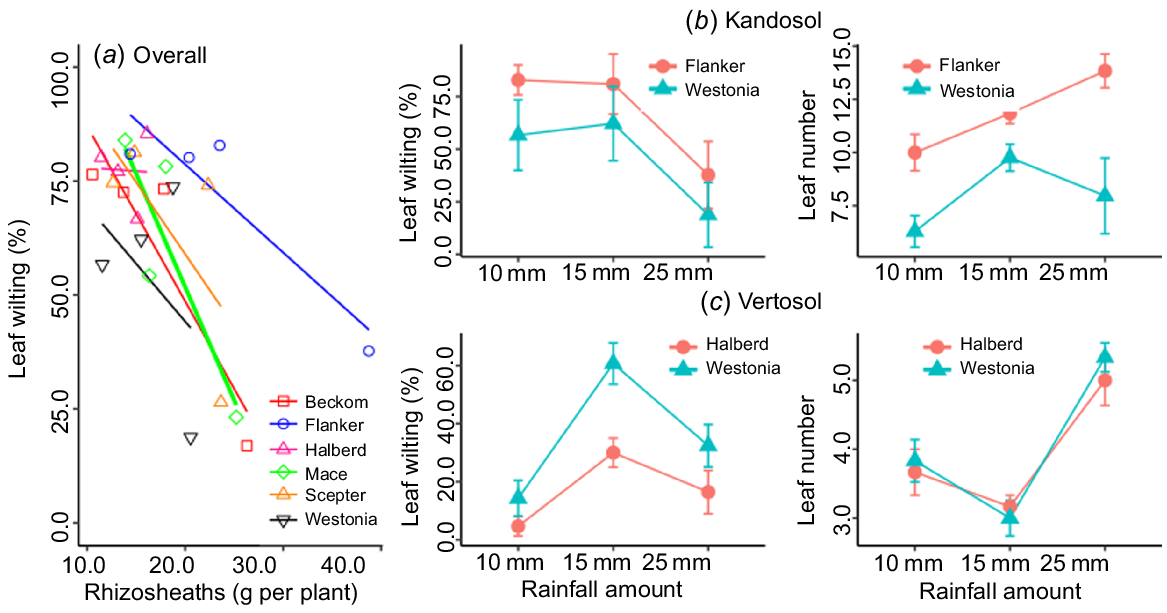
Differences in leaf wilting were also observed between the cultivars with contrasting rhizosheath size. In the Kandosol, leaf wilting tended to be consistently higher (P = 0.12) in Flanker (larger rhizosheath) than in Westonia, with the smaller rhizosheath (Fig. 7b), whereas in the Vertosol, Halberd (larger rhizosheath) showed a lower leaf wilting percentage after the prolonged dry spell than Westonia (Fig. 7c).
Discussions
Innovative technologies and management strategies are continually being developed to enable farmers to minimise their exposure to risks associated with climate. One such strategy is to sow early (Hunt et al. 2019); however, with climate change increasing the occurrence of irregular rainfall patterns, false breaks become more likely. False breaks are reported to occur in other dryland areas, such as in Israel, where a dry period of 3–5 weeks is very common after the initial rain (Klein et al. 2002). The unique properties of rhizosheaths led us to hypothesise that their formation may buffer wheat seedlings during extended false-break conditions to keep plants alive until the next rainfall.
Our research showed that genotype selection and management strategies that encourage formation of a large rhizosheath can help to reduce plant seedling death under false-break conditions. Although the amount of starting rainfall was critical, our study showed that cultivars differed significantly concerning how far they could survive without follow-up rain. In general, cultivar differences for rhizosheath size were stable across the various scenarios of starting rainfall and false breaks, irrespective of soil type (Fig. 4). This suggests that a combination of cultivar choice and management could provide growers with a strategy to minimise the risks of false-break conditions. For instance, rhizosheath formation is severely affected by soil acidity (Haling et al. 2010); therefore, in acidic soils, application of lime to the soil surface or by incorporation in the plough layer (0–10 cm depth) could be coupled with choice of cultivar to improve rhizosheath formation in wheat. However, more research is required, because the performance of Westonia was contrary to expectations. Westonia is reportedly tolerant to soil acidity (Tang et al. 2003), possessing the Al3+-tolerant allele of TaALMT1, a major gene for aluminium tolerance in wheat (Delhaize et al. 2012), and a locus for a large rhizosheath in the Rht-D1 genomic region (Delhaize et al. 2015). It was previously identified as a genotype forming a moderately large rhizosheath (Delhaize et al. 2015), but it was consistently an inferior cultivar in our preliminary trials and in the two different soil types used in this study. The performance of Halberd, the top-ranking cultivar in the Vertosol, is consistent with expectations. Halberd is known for its tolerance to subsoil constraints (boron toxicity) (Paull et al. 1988), and it has a long coleoptile (Rebetzke et al. 2007), a character essential for establishment in harsh soil conditions. Overall, the rhizosheath-forming ability of Flanker was a highlight of this study because it is a relatively new cultivar and previously untested. In the Kandosol, comparative growth performance of the cultivars with the best (Flanker) and worst (Westonia) rhizosheath formation showed that Flanker was progressively better able to establish as false-break duration increased, producing significantly more tillers and leaves than Westonia.
Differences in leaf wilting were also observed between Flanker and Westonia (not significant, P = 0.12), the two cultivars with contrasting rhizosheath size in the Kandosol, and between Halberd and Westonia (P = 0.01), the contrasting cultivars in the Vertosol. In the Kandosol, the proportion of wilted leaves was consistently higher in Flanker (larger rhizosheath) than in Westonia (smaller rhizosheath), probably due to Flanker having more leaves than Westonia (Fig. 7b), which could lead to higher transpiration and more wilting of the leaves. Although transpiration rate was not measured in this study, Rabbi et al. (2022), who normalised rhizosheath by total root length, reported a positive influence of rhizosheath on transpiration of wheat cultivars. In the Vertosol, where the two contrasting cultivars had similar numbers of leaves, the cultivar with the large rhizosheath (Halberd) showed consistently lower leaf wilting percentage than Westonia, the cultivar with the small rhizosheath cultivar (Fig. 7c). This agrees with previous reports (Basirat et al. 2019; Rabbi et al. 2021), but as shown in the Kandosol, the influence of other factors affecting whole-plant transpiration, such as leaf number, requires investigation.
Breeding for rhizosheath architecture and function may be a future avenue for better designing crops for a changing environment (Rabbi et al. 2018). In wheat, the breeding of cultivars for rhizosheath size is feasible, because Gramineae (grass species) have a genetic predisposition to develop rhizosheaths, and they are found on individual plants regardless of the soil texture or rainfall conditions (Bailey and Scholes 1997). Furthermore, a reliable and simple methodology for screening is available that can be adapted to commercial breeding programs (Delhaize et al. 2015). In the present study, rhizosheaths were measured in seedlings of up to 56 days of age, and the process was found to be labour-intensive and prone to errors. In soils with high clay content such as the Vertosol that harden when dried out, loosening the roots from the bulk soil can be difficult.
Overall, varietal selection and/or cultural management for large rhizosheaths may improve the chances of crop survival in the event of false-break conditions. Planting of wheat crops that can establish on little or no follow-up rain will minimise the risk of crop failure and contribute to improved weed control and nutrient use. Although grain yield data were not collected, the results support long-standing suggestions that rhizosheath formation may aid plant survival in conditions of declining water availability (Hartnett et al. 2013; Lynch et al. 2014; Rellán-Álvarez et al. 2016; Brown et al. 2017; Basirat et al. 2019; Ndour et al. 2020; Aslam et al. 2022; Cheraghi et al. 2023; Mo et al. 2023). Improving crop establishment under harsh conditions will translate to higher density of plants that survive and are able to produce grains. It is acknowledged that the results from controlled environments can be quite different from those of field environments and require verification. Further evidence is needed as to the value of the proposition of rhizosheath size as a target trait, and future research is suggested for a field-scale study of rhizosheath potential to improve wheat crop establishment under harsh conditions.
Declaration of funding
This experiment was part of the project ‘Agronomic value of rhizosheaths in improving wheat crop establishment’, BLG122, 2021–22, a joint investment by Grains Research and Development Corporation (GRDC) and NSW Department of Primary Industries, Australia, under the Grains Agronomy and Pathology Partnership (GAPP).
Acknowledgements
We thank the anonymous reviewers of this paper for their invaluable suggestions to improve the original manuscript. The support received from the NSW DPI, Wagga Wagga, Soil Group (team leader, Dr Ehsan Tavakkoli) is gratefully acknowledged. Many thanks to Craig Whiting, for his support during a period that was overshadowed by the COVID pandemic.
References
Adu MO, Asare PA, Yawson DO, Ackah FK, Amoah KK, Nyarko MA, Andoh DA (2017) Quantifying variations in rhizosheath and root system phenotypes of landraces and improved varieties of juvenile maize. Rhizosphere 3, 29-39.
| Crossref | Google Scholar |
Aslam MM, Karanja JK, Dodd IC, Waseem M, Weifeng X (2022) Rhizosheath: an adaptive root trait to improve plant tolerance to phosphorus and water deficits? Plant, Cell & Environment 45(10), 2861-2874.
| Crossref | Google Scholar | PubMed |
Bailey C, Scholes M (1997) Rhizosheath occurrence in South African grasses. South African Journal of Botany 63(6), 484-490.
| Crossref | Google Scholar |
Basirat M, Mousavi SM, Abbaszadeh S, Ebrahimi M, Zarebanadkouki M (2019) The rhizosheath: a potential root trait helping plants to tolerate drought stress. Plant and Soil 445(1–2), 565-575.
| Crossref | Google Scholar |
Brown LK, George TS, Neugebauer K, White PJ (2017) The rhizosheath – a potential trait for future agricultural sustainability occurs in orders throughout the angiosperms. Plant and Soil 418(1–2), 115-128.
| Crossref | Google Scholar |
Chapman R, Asseng S (2001) An analysis of the frequency and timing of false break events in the Mediterranean region of Western Australia. Australian Journal of Agricultural Research 52(3), 367-376.
| Crossref | Google Scholar |
Cheraghi M, Mousavi SM, Zarebanadkouki M (2023) Functions of rhizosheath on facilitating the uptake of water and nutrients under drought stress: a review. Plant and Soil 491, 239-263.
| Crossref | Google Scholar |
de Mendiburu F (2023) agricolae: statistical procedures for agricultural research. R package version 1.3-6. Available at https://CRAN.R-project.org/package=agricolae
Delhaize E, James RA, Ryan PR (2012) Aluminium tolerance of root hairs underlies genotypic differences in rhizosheath size of wheat (Triticum aestivum) grown on acid soil. New Phytologist 195(3), 609-619.
| Crossref | Google Scholar | PubMed |
Delhaize E, Rathjen TM, Cavanagh CR (2015) The genetics of rhizosheath size in a multiparent mapping population of wheat. Journal of Experimental Botany 66(15), 4527-4536.
| Crossref | Google Scholar | PubMed |
Galloway AF, Akhtar J, Marcus SE, Fletcher N, Field K, Knox P (2020) Cereal root exudates contain highly structurally complex polysaccharides with soil-binding properties. The Plant Journal 103(5), 1666-1678.
| Crossref | Google Scholar | PubMed |
George TS, Brown LK, Ramsay L, White PJ, Newton AC, Bengough AG, Russell J, Thomas WTB (2014) Understanding the genetic control and physiological traits associated with rhizosheath production by barley (Hordeum Vulgare). New Phytologist 203, 195-205.
| Crossref | Google Scholar | PubMed |
Goodchild DJ, Myers LF (1987) Rhizosheaths-a neglected phenomenon in Australian agriculture. Australian Journal of Agricultural Research 38(3), 559-563.
| Crossref | Google Scholar |
Haling RE, Simpson RJ, Delhaize E, Hocking PJ, Richardson AE (2010) Effect of lime on root growth, morphology and the rhizosheath of cereal seedlings growing in an acid soil. Plant and Soil 327, 199-212.
| Crossref | Google Scholar |
Hartnett DC, Wilson GWT, Ott JP, Setshogo M (2013) Variation in root system traits among African semi-arid savanna grasses: implications for drought tolerance. Austral Ecology 38(4), 383-392.
| Crossref | Google Scholar |
Hochman Z, Horan H (2018) Causes of wheat yield gaps and opportunities to advance the water-limited yield frontier in Australia. Field Crops Research 228, 20-30.
| Crossref | Google Scholar |
Hunt JR, Lilley JM, Trevaskis B, Flohr BM, Peake A, Fletcher A, Zwart AB, Gobbett D, Kirkegaard JA (2019) Early sowing systems can boost Australian wheat yields despite recent climate change. Nature Climate Change 9(3), 244-247.
| Crossref | Google Scholar |
Klein JD, Mufradi I, Cohen S, Hebbe Y, Asido S, Dolgin B, Bonfil DJ (2002) Establishment of wheat seedlings after early sowing and germination in an arid Mediterranean environment. Agronomy Journal 94(3), 585-593.
| Crossref | Google Scholar |
Liu T-Y, Ye N, Song T, Cao Y, Gao B, Zhang D, Zhu F, Chen M, Zhang Y, Xu W, Zhang J (2019a) Rhizosheath formation and involvement in foxtail millet (Setaria italica) root growth under drought stress. Journal of Integrative Plant Biology 61(4), 449-462.
| Crossref | Google Scholar | PubMed |
Liu T-Y, Chen M-X, Zhang Y, Zhu F-Y, Liu Y-G, Tian Y, Fernie AR, Ye N, Zhang J (2019b) Comparative metabolite profiling of two switchgrass ecotypes reveals differences in drought stress responses and rhizosheath weight. Planta 250(4), 1355-1369.
| Crossref | Google Scholar | PubMed |
Lynch JP, Chimungu JG, Brown KM (2014) Root anatomical phenes associated with water acquisition from drying soil: targets for crop improvement. Journal of Experimental Botany 65(21), 6155-6166.
| Crossref | Google Scholar | PubMed |
Marin M, Feeney DS, Brown LK, Naveed M, Ruiz S, Koebernick N, Bengough AG, Hallett PD, Roose T, Puértolas J, Dodd IC, George TS (2021) Significance of root hairs for plant performance under contrasting field conditions and water deficit. Annals of Botany 128(1), 1-16.
| Crossref | Google Scholar | PubMed |
McDonald GK, Taylor JD, Gong X, Bovill W (2018) Responses to phosphorus among barley genotypes. Crop & Pasture Science 69(6), 574-586.
| Crossref | Google Scholar |
Miralles DJ, Slafer GA (1991) A simple model for non-destructive estimates of leaf area in wheat. Cereal Research Communications 19, 439-444.
| Google Scholar |
Mo X, Wang M, Zeng H, Wang J (2023) Rhizosheath: distinct features and environmental functions. Geoderma 435, 116500.
| Crossref | Google Scholar |
Ndour PMS, Heulin T, Achouak W, Laplaze L, Cournac L (2020) The rhizosheath: from desert plants adaptation to crop breeding. Plant and Soil 456, 1-13.
| Crossref | Google Scholar |
Patrignani A, Ochsner TE (2015) Canopeo: a powerful new tool for measuring fractional green canopy cover. Agronomy Journal 107(6), 2312-2320.
| Crossref | Google Scholar |
Paull JG, Cartwright B, Rathjen AJ (1988) Responses of wheat and barley genotypes to toxic concentrations of soil boron. Euphytica 39, 137-144.
| Crossref | Google Scholar |
Peterson RA (2021) Finding optimal normalizing transformations via bestnormalize. The R Journal 13(1), 310-329.
| Crossref | Google Scholar |
Pook M, Lisson S, Risbey J, Ummenhofer CC, McIntosh P, Rebbeck M (2009) The autumn break for cropping in southeast Australia: trends, synoptic influences and impacts on wheat yield. International Journal of Climatology 29(13), 2012-2026.
| Crossref | Google Scholar |
Price SR (1911) The roots of some North African desert grasses. New Phytologist 10, 328-340.
| Crossref | Google Scholar |
Rabbi SMF, Tighe MK, Flavel RJ, Kaiser BN, Guppy CN, Zhang X, Young IM (2018) Plant roots redesign the rhizosphere to alter the three-dimensional physical architecture and water dynamics. New Phytologist 219(2), 542-550.
| Crossref | Google Scholar | PubMed |
Rabbi SMF, Tighe MK, Warren CR, Zhou Y, Denton MD, Barbour MM, Young IM (2021) High water availability in drought tolerant crops is driven by root engineering of the soil micro-habitat. Geoderma 383, 114738.
| Crossref | Google Scholar |
Rabbi SMF, Warren CR, Macdonald C, Trethowan RM, Young IM (2022) Soil-root interaction in the rhizosheath regulates the water uptake of wheat. Rhizosphere 21, 100462.
| Crossref | Google Scholar |
Rebetzke GJ, Richards RA, Fettell NA, Long M, Condon AG, Forrester RI, Botwright TL (2007) Genotypic increases in coleoptile length improves stand establishment, vigour and grain yield of deep-sown wheat. Field Crops Research 100(1), 10-23.
| Crossref | Google Scholar |
Rellán-Álvarez R, Lobet G, Dinneny JR (2016) Environmental control of root system biology. Annual Review of Plant Biology 67, 619-642.
| Crossref | Google Scholar | PubMed |
Rohan M, Sarmah AK (2023) Computation of standard error for half-life estimation using various dissipation models for regulatory purposes. Science of The Total Environment 893, 164773.
| Crossref | Google Scholar |
Smith A (2022) The autumn break: fact or fiction? Local Land Services, NSW Government. Available at nsw.gov.au
Tang C, Nuruzzaman M, Rengel Z (2003) Screening wheat genotypes for tolerance of soil acidity. Australian Journal of Agricultural Research 54(5), 445-452.
| Crossref | Google Scholar |
Watt M, McCully ME, Jeffree CE (1993) Plant and bacterial mucilages of the maize rhizosphere: comparison of their soil binding properties and histochemistry in a model system. Plant and Soil 151, 151-165.
| Crossref | Google Scholar |
Watt M, McCully ME, Canny MJ (1994) Formation and stabilization of rhizosheaths of Zea mays L. (effect of soil water content). Plant Physiology 106(1), 179-186.
| Crossref | Google Scholar | PubMed |
Young IM (1995) Variation in moisture contents between bulk soil and the rhizosheath of wheat (Triticum aestivum L. cv. Wembley). New Phytologist 130(1), 135-139.
| Crossref | Google Scholar |
Zadoks JC, Chang TT, Konzak CF (1974) A decimal code for the growth stages of cereals. Weed Research 14(6), 415-421.
| Crossref | Google Scholar |
Zhang Y, Du H, Gui Y, Xu F, Liu J, Zhang J, Xu W (2020) Moderate water stress in rice induces rhizosheath formation associated with abscisic acid and auxin responses. Journal of Experimental Botany 71(9), 2740-2751.
| Crossref | Google Scholar | PubMed |