Screening and field evaluation of white clover rhizobia for New Zealand pastures
Shengjing Shi


A AgResearch Ltd, Lincoln Science Centre, Christchurch, New Zealand.
B Scion, PO Box 29237, Christchurch 8440, Christchurch, New Zealand.
C Grasslanz Technology Ltd., Hamilton, New Zealand.
D AgResearch Ltd, Grasslands Research Centre, Private Bag 11008, Palmerston North 4410, New Zealand.
E South Australian Research and Development Institute, GPO Box 397, Adelaide, SA 5001, Australia.
Abstract
Biological nitrogen (N) fixation through the rhizobia–legume symbiosis is a sustainable and cost-effective source of N for agriculture. In New Zealand (NZ), white clover (Trifolium repens) is a key component of pastures and rhizobial inoculation of clover is widely used. The current commercial inoculant for white clover, TA1, was isolated in Australia in the 1950s and may not be the best partner for modern white clover cultivars.
To identify Rhizobium leguminosarum bv. trifolii (Rlt) isolates suitable for use in NZ pastures.
The symbiotic potential of >230 isolates collected from throughout NZ was evaluated in plant bioassays. Selected isolates were further evaluated in pot and field trials.
Approximately 40% of NZ isolates supported better clover growth than TA1 under N-limited conditions in vitro. Of 24 Rlt isolates evaluated in a glasshouse trial, five produced significantly higher clover biomass than TA1. Three (S11N9, S20N7, S4N6) of nine isolates evaluated in two field trials in 2018–2019 significantly increased clover growth (12–38%) compared with paired uninoculated clover at several harvests, whereas inoculation with TA1 did not improve yield. In a third trial in 2020, S11N9 and S20N7 increased clover growth compared with the uninoculated control at two of three harvests; S4N6 performed better than TA1 at one harvest. When tested with four white clover cultivars, five Rlt isolates had higher symbiotic potential than TA1.
Inoculating white clover would be beneficial if improved inoculant isolates were available.
We recommend some NZ Rlt isolates could be developed into commercial inoculants to improve white clover performance in NZ.
Keywords: biological N fixation, cultivars, field trials, inoculant, Rhizobium leguminosarum bv. trifolii, sustainability, symbiotic potential, white clover.
Introduction
As concerns over the environmental impacts of intensive agriculture increase, the demand for more sustainable farming practices is becoming urgent. Legumes, through their symbiotic association with rhizobial inoculants, have long been used to increase soil nitrogen (N) and plant productivity, especially prior to widespread use of N fertilisers, which in New Zealand began in the early 1990s (StatsNZ 2021). Increasing the amount of fixed N provided by the legume–rhizobia symbiosis can support reductions in N fertiliser use and reduce undesirable environmental impacts associated with poor fertiliser N utilisation and subsequent leaching, while still maintaining plant production (Caradus et al. 1996; Drew et al. 2011; Braakhekke et al. 2017; Soumare et al. 2020).
In New Zealand, forage legumes are an important component of pastures, where they have been relied upon to provide biologically fixed N to the system to support livestock production (Caradus et al. 1996). White clover (Trifolium repens) is the most widely used forage legume in New Zealand and is integral to temperate agronomy globally. Because of its phenotypic plasticity (Caradus et al. 1993), white clover is adapted to a range of different environments. This is likely underpinned by its allotetraploid genomic architecture where it has retained two progenitor genomes, thus increasing its genetic ‘toolbox’ (Griffiths et al. 2019). Because the rhizobia (Rhizobium leguminosarum bv. trifolii (Rlt)) that nodulate white clover roots are not native to NZ, inoculation of white clover seeds with Rlt became a standard practice in the 1960s (Greenwood 1965). A range of Rlt isolates have been used for white clover inoculation in New Zealand since the 1950s, but by far the most widely used are Australian strains CC275e and TA1. Although CC275e has been reported to fix more N on white clover and have greater persistence in soil than TA1 (Lowther and Kerr 2011), TA1 was selected by the inoculant industry based on its ease of production (Delestre et al. 2015). Thus, the current commercial strain for white clover inoculation in New Zealand is TA1, which was originally isolated from a root nodule of the annual clover T. subterraneum in Tasmania, Australia, in the early 1950s (Bullard et al. 2005). TA1 has been commercially available for use in Australia since 1961 (Bullard et al. 2005) and has been imported and used extensively throughout New Zealand. It is worth reflecting that there has been no replacement of our main legume inoculant in more than six decades, yet selection of improved white clover varieties has been a continuous process. It is the expression of the clover × Rlt that is important for N fixation, and improvement for agronomic and environmental gain should consider both.
Because of the long history of clover seed inoculation, clover rhizobia populations have become naturalised in New Zealand and are widespread and often abundant in pasture soils (see Lowther and Kerr (2011) and references therein). This has led to the proposition that inoculation of white clover is no longer necessary, except where background soil rhizobia populations are expected to be low e.g. after long-term (>10 years) maize cultivation or on land recently cleared of native bush (Lowther and Kerr 2011). However, recent research has found considerable variability in the abundance and symbiotic potential of soil rhizobia populations in 26 long-term pasture soils throughout New Zealand; population densities ranged from less than 100 to >1 × 108 rhizobia per gram of soil, with nitrogen fixation capacity (in comparison with the current commercial strain TA1) ranging from 14 to 143% efficacy (Wakelin et al. 2018). High spatial variability occurred across farms and within fields, suggesting that there are likely many locations and niches where inoculation with improved isolates will be beneficial.
Although many white clover cultivars have been developed in New Zealand over recent decades, there has been no corresponding effort to select optimal rhizobia isolates for these cultivars, meaning TA1 remains the primary inoculant. It is likely that superior inoculants can be identified given that isolates with increased N fixation capacity and desiccation tolerance have already been isolated from New Zealand soils (van Ham et al. 2016). Intrinsic desiccation tolerance is a highly desirable attribute for rhizobia inoculants, with future climate change scenarios indicating increased drought, in addition to poor survival of rhizobia during commercial formulation and seed application, which limits their impact in the field (Deaker et al. 2004; Deaker et al. 2012).
Much of the previous research with strain TA1 was undertaken using white clover cultivar ‘Grasslands Huia’, which was bred in the late 1950s and released in 1964 (Caradus et al. 1995; Lowther and Kerr 2011). However, Huia is now rarely grown in New Zealand because of the availability of several superior cultivars bred specifically for New Zealand conditions (J. Caradus, pers. comm.). In this study, white clover cv. ‘Grasslands Tribute’ (Woodfield et al. 2003) was used as the standard cultivar in most of the evaluations. This medium-large leaved cultivar was selected in New Zealand for its consistent performance under grazing, its strong growth in most seasons, good cool-season growth, and its ability to tolerate New Zealand drought conditions. These characteristics, together with its tolerance to some pests and diseases, have led to its widespread use in sheep and dairy grazing pastures. Tribute is morphologically similar to Huia but has a very different genetic background (Woodfield et al. 2003), although Tribute does have some Huia parentage from the use of ‘Grasslands Sustain’ in the breeding of Tribute (Caradus et al. 1997).
In this study, >230 Rlt isolates from pasture soils across New Zealand were evaluated for their N-fixation capacity in plant bioassays. Promising isolates were further evaluated in a glasshouse pot trial and then in three field trials. Previous studies have shown that cultivars vary in their relationships with different rhizobia isolates (Sherwood and Masterson 1974; Harrison et al. 1989; Rigg et al. 2021), so five isolates that performed well in field trials with cv. Tribute were also evaluated for their ability to fix N with three additional white clover cultivars.
Materials and methods
White clover varieties
White clover (Trifolium repens L.) cv. ‘Grasslands Tribute’ (medium-large leaf, (Woodfield et al. 2003)), hereafter referred to as cv. Tribute, was used as the test clover cultivar to benchmark rhizobium isolate performance. Additional cultivars were used to test the symbiotic potential of a subset of Rlt isolates against a range of clover genetics. These represented modern and legacy white clover cultivars used in New Zealand pastures and included ‘Grasslands Prestige’ (small-medium leaf (Cooper and Chapman 1993)), ‘Grasslands Legacy’ (large leaf, (Ford et al. 2015)) and ‘Grasslands Quartz’ (medium-large leaf, (Jahufer et al. 2021)). Seed lots of the cultivars were sourced either from the Margot Forde Germplasm Centre at AgResearch Grasslands, Palmerston North, New Zealand, or local seed merchants.
Rhizobia isolates
A collection of >500 Rlt isolates curated by AgResearch Lincoln, New Zealand, as reported previously (van Ham et al. 2016), was used for this study. The isolates were recovered from nodules of white clover (Trifolium repens L. cv. Tribute) plants grown in sterile vermiculite inoculated with fresh or room temperature air-dried soil samples collected from 26 pasture sites across New Zealand. Characteristics of the sites and physiochemical properties of the soils have been reported previously (van Ham et al. 2016), but briefly, the farms sampled were selected to be representative of long-term livestock-grazed pastures under low- through to high-intensity grazing systems. Bacterial colonies isolated from nodules were putatively identified as rhizobia by visual assessment of colony characteristics and were re-streaked for purification before being stored at −80°C using the ‘Protect’ microorganism preservation system (Lab M Ltd, Bury, UK). Approximately 20 isolates from each site were placed into storage. Identity of putative Rlt isolates was confirmed by DNA sequencing of a large fragment of the 16S ribosomal RNA (rRNA) gene (~800 bp), as reported previously (van Ham et al. 2016).
Rlt strain TA1 was originally sourced from the Australian Legume Inoculant Research Unit and stored at −80°C using the ‘Protect’ microorganism preservation system in the AgResearch culture collection (AgResearch, Lincoln).
Symbiotic potential of rhizobia isolates
For this study, 231 Rlt isolates recovered from clover plants grown in fresh soils were used. Nitrogen fixation capacity of these Rlt was measured in plant bioassays modified from methods described by Wakelin et al. (2018). Growth of white clover seedlings (cv. Tribute) inoculated with the NZ isolates was compared to the growth of seedlings inoculated with Rlt strain TA1, with all plants grown under N-limited conditions. Plants were grown in 70 mL plastic specimen containers packed with pasteurised vermiculite (Grade 2, Exfoliators Pty Ltd, Dandenong South, Vic., Australia) moistened with 40 mL of sterile McKnight’s nutrient solution (Unkovich et al. 2008) containing only trace N (0.1 mM NH4NO3). Clover seeds were surface sterilised and pre-germinated as described by Wakelin et al. (2018) and one seed planted in each container. Rhizobia isolates and TA1 were cultured on yeast mannitol agar (YMA) plates at 28°C for 4 days. Cell suspensions containing approximately 106 colony-forming units (CFU) of each isolate were prepared by washing colonies from YMA plates with sterile saline (0.85% NaCl). Aliquots (1 mL) of the individual cell suspensions were then used to inoculate clover seedlings when seedlings were 4 days old. Treatments were arranged in a randomised complete block design in a growth room (16 h light at 22°C; 8 h dark at ambient temperature), with eight replicate containers per treatment. Plants were harvested 6 weeks after inoculation and shoot dry weight (SDW) measured after drying at 60°C. The nitrogen fixation capacity of each isolate (hereafter referred to as symbiotic potential, SP) was calculated relative to TA1 as described previously (Wakelin et al. 2018), using the following equation:
where brackets indicate individual isolate (isolate), uninoculated (uninoc) and commercial strain (TA1) treatments.
Eighty-five Rlt isolates showed higher SPs than TA1 in the primary assay described above. Among these, 23 isolates were selected for testing in a glasshouse pot trial based on their SP values and low variability (standard error) across the replicates, together with TA1. Eight pre-germinated white clover seeds (cv. Tribute) were planted in 4 L pots filled with pasteurised vermiculite saturated with an N-deficient McKnight’s nutrient solution (Unkovich et al. 2008). For each inoculation treatment (pot), a 1 mL suspension in 0.85% (w/v) saline of an individual rhizobia isolate (~106 CFU) grown on YMA plates was applied to each seedling near its base when the seedlings were 4 days old. Uninoculated controls had a similar amount of 0.85% saline applied. Four replicate pots were prepared for each treatment (although eight replicates were used for TA1 treatment) and arranged in a randomised complete block design within an unheated glasshouse during spring. After 6 weeks, the clover shoots were cut at the base and dried at 60°C. SDWs were determined, and the SP of each isolate was calculated relative to TA1 as described above.
Field trials
Based on their high SP in both the laboratory assay and glasshouse pot trial, selected rhizobia isolates were then evaluated in the field with white clover cv. Tribute. Selection of isolates for field assessment was also based on their different genotypic profiling (data not shown here). Isolates tested and trial details are provided in Table 1. Trial 1 was conducted at the Plant & Food Research Farm, Lincoln, Canterbury, and Trials 2 and 3 were located near Rolleston, Canterbury (Table 1). One month prior to sowing, existing vegetation was removed by application of a knockdown herbicide (Treflan) at a concentration of 1.2 L ha−1, plant residue was removed, and sites were tilled. From each trial site, 10 soil cores (2 cm diameter and 10 cm depth) were randomly collected from within each replicated block and were combined for determination of soil physicochemical properties at Hills Laboratories (Christchurch, New Zealand, https://www.hill-laboratories.com/analytical-testing/soil-testing/). Soil parameters are provided in Table 1.
Trial number | Trial 1 | Trial 2 | Trial 3 | |
---|---|---|---|---|
Duration of trial | October 2018–March 2019 | October 2018–March 2019 | March 2019–February 2020 | |
Treatments | S22N7, S3N2, S17N1, S10N9, S4N6, S20N8, S11N9, S21N6, S20N7, TA1 and paired uninoculated control | S11N9, S17N1, S20N7, S4N6, S3N2, paired TA1 and paired uninoculated control | ||
Location | Plant & Food Research Farm, Lincoln (−43.627172º, 172.479986º) | Rolleston (−43.624289º, 172.431738º) | Rolleston (−43.623791º, 172.433954º) | |
Soil pH (water) | 5.5 ± 0.04 | 6.1 ± 0.04 | 5.7 ± 0.04 | |
Soil mineral N (mg kg−1) | 40 ± 1.9 | 3 ± 0.3 | 12.6 ± 0.67 | |
Soil Olsen P (mg L−1) | 23.3 ± 1.0 | 18.0 ± 0.6 | 16.8 ± 1.0 | |
Soil total C (%) | 2.8 ± 0.2 | 3.1 ± 0.3 | 2.8 ± 0.1 | |
Soil total N (%) | 0.21 ± 0.01 | 0.23 ± 0.02 | 0.25 ± 0.01 | |
Soil sulfate sulfur (mg kg−1) | 20.2 ± 3.4 | 3. 7 ± 0.5 | 16.4 ± 0.6 | |
MPN of soil rhizobia (number per g soil) | 8506 ± 1668 (range: 2630–15 978) | 4207 ± 2285 (range: 573–16 575) | 3236 ± 1116 (range: 480–13 000) | |
Symbiotic potential of soil rhizobia (% TA1) | 118 ± 10 (range: 94–137%) | 89 ± 13 (range: 45–149%) | 61 ± 11 (range: 12–132%) | |
Fertiliser application during trial | Base fertiliser (Cropmaster 15 at 250 kg ha−1, which is equivalent to 42 kg N ha−1, 10 kg S ha−1 and 42 kg P ha−1) when preparing the site before sowing (September 2018) | No fertiliser applied | Fertiliser (20% potash sulfur super, which contains 0% N, 6.4% P, 10.0% K, 16.4% S and 14.4% Ca, at 270 kg ha −1) was applied in spring after removing clover biomass in October 2019. | |
Sampling date | 1st cut (October 2018–January 2019), 2nd cut (October 2018–February 2019) and 3rd cut (February 2019–March 2019) | 1st cut (October 2019–November 2019), 2nd cut (November 2019–January 2020) and 3rd cut (January 2020–February 2020) | ||
Sampling area | Clover growth (or regrowth) of 2 m sowing line | 2.5 m2 clover growth or regrowth |
MPN, Most Probable Number.
Enumeration of resident soil populations of Rlt at each site was undertaken using the bulked soil samples described above. Soil rhizobia populations were determined using the Most Probable Number (MPN) assay (Brockwell 1980) and as described by van Ham et al. (2016), using cv. Tribute as the host plant. Numbers of Rlt were estimated using the MPN calculator as described by Drew et al. (2011). Symbiotic potential of the resident Rlt populations was determined using the whole-soil inoculation method (Brockwell et al. 1988) and as described in Wakelin et al. (2018). This method assesses the SP of the overall rhizobial population in the soil sample, as opposed to the isolate-specific measurement of SP as described above for individual Rlt isolates.
To account for expected high spatial variability in the resident rhizobia population and for soil variation (Wakelin et al. 2018) (Table 1), the field evaluation of promising rhizobia isolates employed a split plot design with rhizobia isolates (NZ isolates or TA1) forming the main plots and inoculation (plus or minus) forming the sub-plots. Sub-plots were established as two parallel rows in Trials 1 and 2 to allow direct pair-wise comparisons between each rhizobia treatment and the control (seed not inoculated with rhizobia). Rows were 4 m long and separated by 50 cm. There was a 2 m buffer zone between different rhizobia treatments (main plots). Six replicates were established at each trial site, with the main plots arranged in a randomised complete block design. About 400 seeds (by weight) were sown in the 4 m rows by hand (equivalent to the recommended field sowing rate of 6 kg ha−1, based on drill lines at 15 cm apart).
In Trial 3, small plots were sown (3 m × 1.35 m) instead of paired rows at the site. Within each main plot (one of five experimental rhizobia isolates), three adjacent sub-plots were established to allow direct comparison between the experimental rhizobia isolate, uninoculated control and TA1-inoculated seed. Ten replicate blocks were used in Trial 3. Main plot treatments were arranged in a randomised complete block design, with sub-plots randomised within each main plot. In contrast to Trials 1 and 2, seeds were sown to a depth of 10 mm using a seed drill at a rate of 4.5 kg seeds per ha. The drill (including seed core, tubes, and wheels) was cleaned thoroughly with high-pressure air between treatments. Canola seeds were sown in buffer zones in all three trials; these were used as material for non-N-fixing reference plants, needed to estimate the N fixation of the clovers using 15N natural abundance assays.
Rhizobia isolates and TA1 were cultured in 100 mL of yeast mannitol broth in 250 mL Erlenmeyer flasks shaken at 100 rpm for 48 h at 28°C. A 35 mL aliquot of each culture broth (containing approximately 108 CFU mL−1 of culture medium) was injected into bags of sterile peat (150 g per bag, New Edge Microbial Ltd, Australia) and incubated at 25°C for 2 weeks. Rhizobia numbers within the peats were enumerated by dilution plating on YMA plates as described previously (Deaker et al. 2012). Each peat inoculant used in the field trials contained >109 CFU g−1 peat. An inoculant slurry of each isolate was prepared by mixing 6.25 g of peat inoculant with 1.5% methylcellulose, which binds the peat to seeds. From this mixture, 6 g of inoculant slurry was applied to 100 g seeds. Seeds were dried by adding micro-fine lime (approximately 3 g for 10 g seeds) with vigorous hand shaking in a circular motion to ensure even coating of the slurry–lime mix onto the seed. Rhizobia on seeds were enumerated prior to sowing by dilution plating onto YMA plates. All treatments had >107 CFU g−1 seeds (ranged from 1.3 × 107 to 5.1 × 107). For the uninoculated control treatment, seeds were treated in the same way but using a peat slurry that had not been inoculated with rhizobia.
White clover herbage was harvested three times for Trials 1 and 2 by hand clipping 2 m of the clover sward (row) to ~2 cm above the ground (Table 1). Weeds were removed before harvest. Clover shoot biomass was dried at 60°C. As clover growth in Trial 3 was very variable across the site in winter-early spring, clover biomass to approximately 5 cm above ground level was removed in late October 2019 using a mower. A non-N fertiliser (20% potash sulfur super (0% N, 6.4% P, 10.0% K, 16.4% S and 14.4% Ca)) was then applied in early November 2019, resulting in more consistent clover regrowth across replicated blocks. Three harvests were conducted for Trial 3 using a mower to collect herbage >5 cm above the ground from an area of 2.5 m2 in the middle of each plot (Table 1). Fresh weights of the clover biomass were recorded on site, and a subsample (~600 g) from each plot was dried at 60°C to estimate the clover dry weight per hectare for each treatment. For each harvest at each trial site, shoots of three canola plants from each block were randomly selected and sampled for subsequent use in nitrogen fixation calculations described below.
The amount of biologically fixed N in selected treatments was estimated using the 15N natural abundance method (Högberg 1997; Boddey et al. 2000). For use as the reference plant (i.e. no N fixation), canola plants grown at each site were harvested at every harvest, dried at 60°C and ground for 15N analysis. A subsample of oven-dried clover shoots from each treatment was ground to a fine powder and the 15N atomic percentage (at%) in each sample determined using an isotope-ratio mass spectrometry analyser in the Soil Analysis Lab, Lincoln University, New Zealand. The percentage of N derived from the atmosphere (% Ndfa) was then estimated using the following equations:
where the δ15N(ref) value represents the level of δ15N detected in a reference plant (i.e. canola) growing in the same site at the same period as clover plants, δ15Nclover is the 15N abundance in the clover sample, and B is −0.588.
Nitrogen fixation capacity of New Zealand Rlt isolates with additional white clover cultivars
A subset of the rhizobia isolates (S3N2, S4N6, S11N9, S17N1, S20N7 and commercial strain TA1) was assessed for SP with the white clover cultivars Tribute, ‘Grasslands Prestige’, ‘Grasslands Legacy’ and ‘Grasslands Quartz’. Seedlings were inoculated with individual rhizobium isolates and grown in a controlled temperature growth room using methods described in Weith et al. (2022). Briefly, 500 g of Grade 3 vermiculite was packed into a 4.4 L container and saturated with 2 L of low-N McKnight’s solution. Each container was sown with all four cultivars, which each cultivar restricted to one of the four quadrants within the container. Each container was allocated as either a treatment inoculated with one of the six rhizobia isolates (including TA1), or used as a positive control (supplemented with mineral nitrogen), or a negative control (no rhizobia nor supplemented mineral nitrogen). Clover seeds were pre-germinated the day before sowing on sterile tissue paper moistened with sterile water. A total of 16 germinated seeds per cultivar were transplanted individually into the vermiculite of the allocated quadrant at 1 × 1 cm spacing in four rows of four seedlings in each container. Suspensions of rhizobium inoculants (1 × 107 cells mL−1) were prepared using sterile deionised water as described previously (Weith et al. 2022), and a 0.5 mL aliquot of the appropriate inoculant was added to the base of each seedling. Seedlings in the negative control treatment received a 0.5 mL aliquot of sterile deionised water, and seedlings in the N positive control received three separate 1 mL aliquots of 15 mmol L−1 NH4NO3 (1.2 g L−1), with the first dose applied 9 days after sowing and weekly thereafter. Three replicates of containers were established for each rhizobium treatment and placed in a randomised split plot design in a controlled temperature (22°C; 16 h light, 8 h dark) growth room. Each treatment container was watered to approximately 80% of its initial moistened weight at sowing (~2000 g) at intervals of 1–3 days to maintain plant water status. After 35 days of growth, the aboveground shoot dry matter of each seedling was harvested and dried at 60°C. The SP was determined as described above.
Statistical analysis
For the SP assay and pot trial, the symbiotic potential of rhizobia isolates was based on comparison of their mean value to TA1. In the trial assessing five isolates with four white clover cultivars, SP values were compared between all isolates using analysis of variance (ANOVA). Prior to analysis of the clover biomass collected from field trials, the difference in SDW between each rhizobia isolate treatment, and its paired control (uninoculated) was calculated within each block for Trials 1 and 2. Similarly, for Trial 3, the differences in SDW between each experimental isolate treatment and paired control or TA1 were calculated prior to analysis. Each ANOVA consisted of isolate treatment factor for comparison. The same ANOVA method was used for the total biologically fixed N as with the clover biomass. All analyses were carried out using statistical software Minitab version 16 and Genstat (18th edition). In each ANOVA, the Fisher’s least significant difference (l.s.d.) method was used as post hoc test.
Results
Nitrogen fixation capacity of Rlt isolates
The SP of the 232 Rlt isolates, as measured in comparison with TA1 in the in vitro assay, varied from 1% to 227% (Fig. 1). Forty-five isolates (19% of total isolates) were 50% less effective (as measured by shoot biomass produced in N-limited laboratory assay) than TA1, whereas 85 isolates (37%) were significantly more effective than TA1. Among these, the SP of 15 isolates were >150%. In general, there was a high degree of variability in SP among isolates from the same sites with white clover. However, isolates from a few sites (e.g. S12, S17, S23–S26) showed generally lower SP compared to TA1. These sites tended to have low soil pH (5.2–5.4).
The symbiotic potential of isolates collected from 26 pasture sites. The SP of individual isolates was calculated relative to TA1 (i.e. TA1 = 100%). Each value is mean SP of an individual isolate (n = 8). Isolates selected for the pot trial and field trials are shown in cyan, with S11N9 shown in red, S20N7 shown in blue and S4N6 shown in pink.
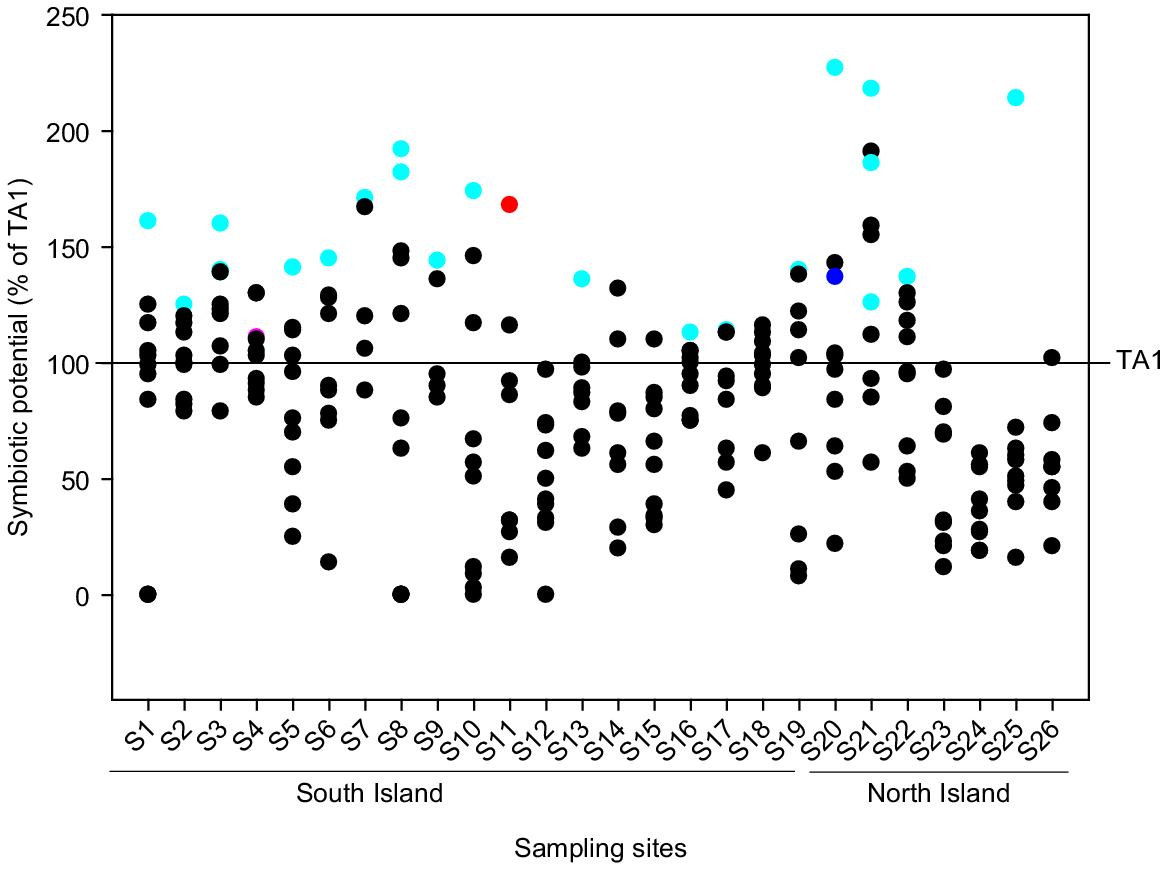
Based on the laboratory symbiotic potential (SP) assays, 23 Rlt isolates with the highest mean SP and smallest standard errors were selected for evaluation in a pot trial with white clover. Clover SDW was significantly (P = 1.2 × 10−5) increased from 0.08 ± 0.01 (s.e.) g plant−1 in the uninoculated control to 0.202 ± 0.01 (s.e.) g plant−1 in the TA1 treatment, indicating adequate viable nodulation by TA1 in the pot trial. All isolates had similar or greater symbiotic potential than TA1, ranging from 85% to 205% of TA1 (Fig. 2). Five isolates had significantly (P < 0.05) higher SP compared to TA1. Visual inspection found small numbers of nodules (mostly white) on some control clover roots, indicating the presence of rhizobia in pots towards the end of experiment. However, many pink nodules were routinely observed in plants inoculated with rhizobia isolates.
Mean symbiotic potential (SP) of selected Rlt isolates with white clover cv. Tribute. The SP of individual isolates was calculated relative to TA1 (i.e. TA1 = 100%). Data shown as mean ± s.e. (n = 4 for Rlt isolates, n = 8 for TA1). Statistical significance (P < 0.05) of SP between Rlt isolates and TA1 is indicated by asterisks. Statistically significant differences were found in each ANOVA with the Fisher’s least significant difference (l.s.d.) method.
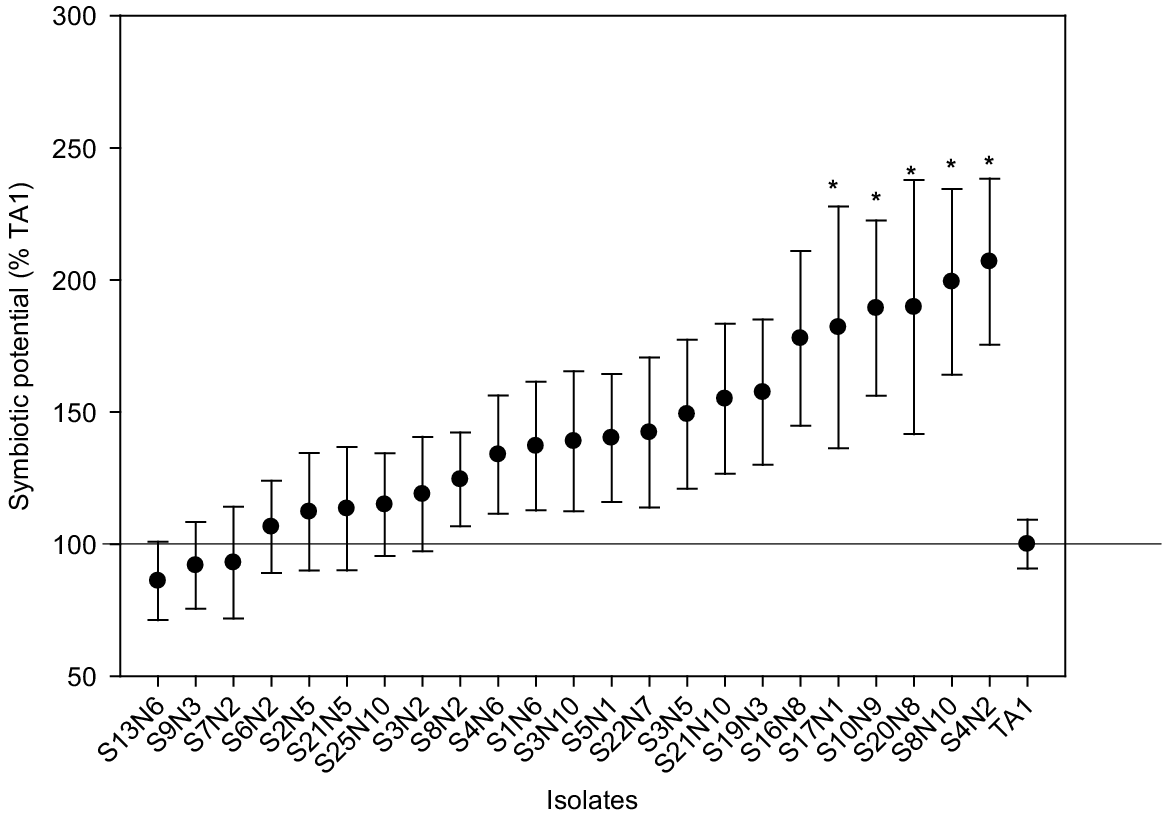
Field evaluation of rhizobia isolates
All trial sites contained resident populations of soil rhizobia. Mean levels across the three sites exceeded 3.20 × 103 rhizobia g−1 soil; however, significant variation was measured within the sites. In Trial 1, Rlt number varied between 2.63 × 103 and 1.60 × 104 rhizobia g−1 soil (Table 1). Trials 2 and 3 had greater spatial variation in rhizobia populations (573–1.66 × 104 and 480–1.30 × 104 rhizobia g−1 soil, respectively). The SP of the resident soil rhizobia also varied between and within sites. The mean SP value was low in Trials 2 and 3 (89% and 61% of TA1, respectively) and was variable across the sites (e.g. ranged from 12% to 132% of TA1 in Trial 3, Table 1). The SP of the resident soil rhizobia in Trial 1 was most consistent and varied between 94% and 137%, with a mean SP value of 118% of TA1.
Of the nine New Zealand isolates evaluated in Trials 1 and 2, six isolates (S22N7, S3N2, S17N1, S10N9, S20N8, and S21N6) did not result in significant improvements in clover growth and are not reported below. Inoculation of clover seed with the remaining isolates (S4N6, S11N9, S20N7) significantly increased clover growth (and N fixation in same cases) at various harvests across the three trials, as detailed below.
The clover SDW in treatments with rhizobium isolates S4N6, S11N9, S20N7, TA1 and their paired control (uninoculated) is shown in Fig. 3. Clover SDW was increased by 12–28% following inoculation with S11N9 compared to the uninoculated control in Trial 1, and by 13–38% in Trial 2, across the three harvests. The average increase in SDW with S20N7 inoculation compared to the uninoculated control was 15–39% in Trial 1 and 0–29% in Trial 2. Isolate S4N6 increased clover SDW (26% increase, P = 0.0068) in Trial 2 in the first harvest (Fig. 3d). Inoculation with commercial strain TA1 did not result in significant yield increases in clover biomass compared to uninoculated control (P > 0.05) in either trial at any harvest. Further, clover SDW following TA1 treatment tended to be lower (P = 0.068) than the uninoculated control in Trial 2 at the first harvest (Fig. 3d).
White clover shoot dry weight (SDW) per 2 m row obtained at three harvest dates for Trials (a, b, c) 1 and (d, e, f) 2. Data shown as box plots with the third quartile (Q3) and first quartile (Q1) range of the data, with the median in the middle. The length of the whiskers is from Q3 or Q1 to the largest or the smallest observation, respectively, which falls within the distance of 1.5 times the interquartile range. Data outside this distance are outliers as indicated by open circles. Data for three of the nine NZ isolates that significantly increased clover SDW compared to the uninoculated treatment are shown. For shoot dry matter, statistical significance is indicated for each rhizobia treatment and its corresponding (paired) uninoculated control treatment on top of the relevant bars as follows: #P < 0.1, *P < 0.05, **P < 0.01. The total biologically fixed N (g N per 2 m line) for three Rlt strains, TA1 and their paired controls are also shown at the bottom of each figure panel (see Table S1 for standard errors). Statistical significance of total fixed N compared to control is indicated by symbols on top of the values in the relevant treatments. Statistically significant differences were found in each ANOVA with the Fisher’s least significant difference (l.s.d.) method.
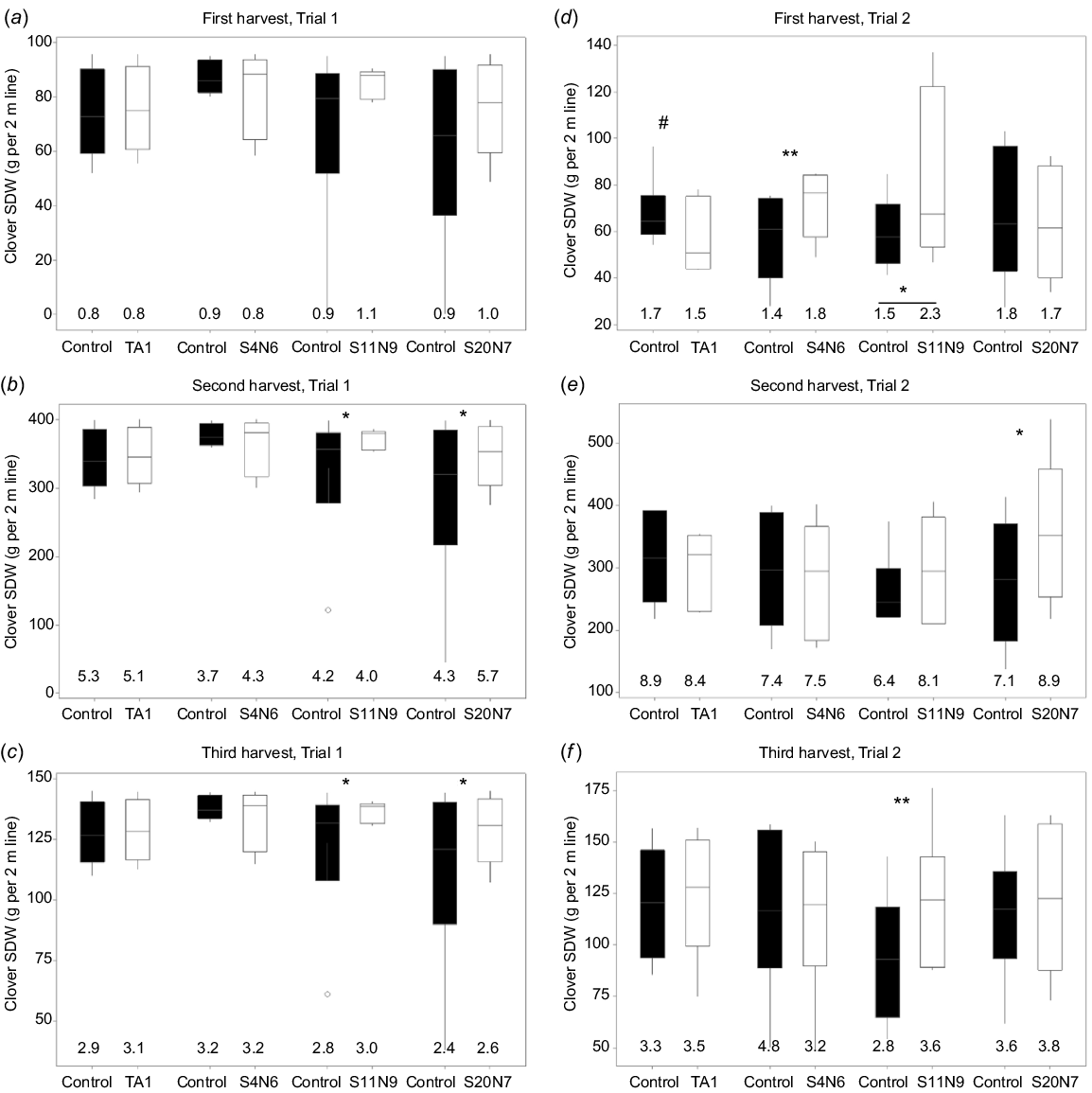
High variability in clover SDW was observed in many rhizobia treatments and controls (Fig. 3). For example, the clover SDW for the uninoculated control treatment in Trial 2 ranged from 27 to 192 g from a 2 m sampled row at the first harvest, 136–451 g per 2 m at the second harvest, and 40–220 g per 2 m at the third harvest. Similarly, large variations of control treatment were observed at Trial 1 for all three harvests. In contrast, the variation across six replicated blocks of clovers inoculated with S11N9 at the Trial 1 was much smaller compared to the paired control at all three harvests (Fig. 3a–c).
To estimate the total N derived from biological N fixation, clover shoot samples from four selected treatments (TA1, S11N9, S20N7, S4N6) and their paired controls were processed for 15N analysis. In most cases, the total fixed N of the rhizobia-treated samples was similar to that of their paired controls (Fig. 3, Supplementary Table S1), with the exception of clover treated with S11N9 in Trial 2 at the first harvest, where a significantly (P < 0.05) larger amount of N was fixed by the S11N9 treatment group compared with the control (Fig. 3d, Table S1).
Five Rlt isolates were further tested in Trial 3, which was established in autumn (March 2019). Cumulative clover SDW at each harvest is shown in Fig. 4. Consistent with Trials 1 and 2, white clover inoculated with isolates S11N9 or S20N7 produced significantly (P < 0.05) more SDW, 9% and 10%, respectively, from late October 2019 to February 2020 relative to the uninoculated control treatment (Fig. 4c). However, although there was one instance where isolate S4N6 significantly increased clover SDW compared to TA1 in the second harvest, SDWs of S4N6-treated clover did not differ significantly from those of the uninoculated control (Fig. 4b). Similar to Trials 1 and 2, high variability of clover SDW among replicates was recorded (Fig. 4). For example, among the 57 uninoculated control plots, clover SDW ranged from 640 to 1652 kg dry mass (DM) ha−1 at the first harvest and 212–2778 kg DM ha−1 at the third harvest (i.e. regrowth from January to February).
Cumulative shoot dry weight from NZ rhizobia isolate treatments paired with uninoculated control and TA1 treatment harvest at (a) November 2019, (b) January 2020 and (c) February 2020 in Trial 3. Data shown as box plots with the third quartile (Q3) and first quartile (Q1) range of the data, with the median in the middle. The length of the whiskers is from Q3 or Q1 to the largest or the smallest observation, respectively, which falls within the distance of 1.5 times the interquartile range. Data outside this distance are outliers as indicated by open circles. Differences between NZ isolates and uninoculated controls and between NZ isolates and TA1 is shown by symbols (*P < 0.05, **P < 0.01, ***P < 0.001) with underlines indicating the treatments for comparison. The total cumulative biologically fixed N (kg N per ha) from three Rlt isolates and their paired controls and paired TA1 is also shown at the bottom of each figure panel (see Table S1 for standard errors). Statistical significance of total fixed N values compared to paired control is indicated by symbols on top of the values in the relevant treatments. Statistically significant differences were found in each ANOVA with the Fisher’s least significant difference (l.s.d.) method.
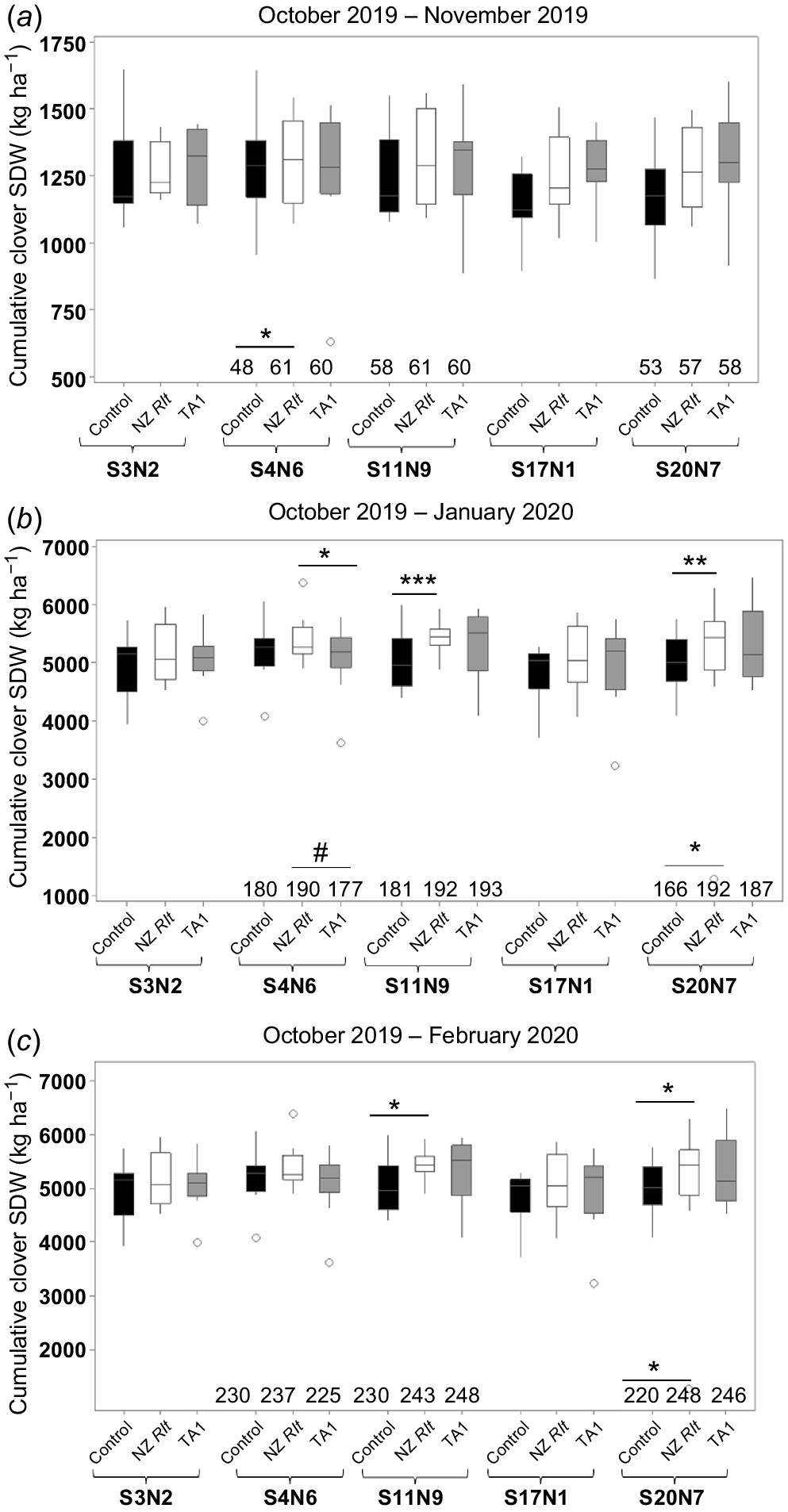
In Trial 3, mean %Ndfa for all three harvests was >90% for all treatments. Clover inoculated with S20N7 had significantly (P < 0.05) higher total fixed N compared to the uninoculated treatment from the second harvest (Fig. 4b, Table S1), but was similar to its paired TA1 treatment. Clover inoculated with S4N6 had significantly higher total fixed N amount than the uninoculated clover at the first harvest (Fig. 4a, Table S1), but only marginally higher total cumulative fixed N amount than clover treated with TA1 from first two harvests (Fig. 4b).
For all three trials, roots from each treatment were collected and assessed visually for the presence of pink nodules. Nodules were present in all the treatment groups, including uninoculated control plants.
Symbiotic potential of Rlt isolates with different clover cultivars
The average SDW of the negative control plants (i.e. no rhizobium, no mineral N addition) across the four cultivars was 2.1 mg per plant, ranging from 2.0 to 2.4 mg. Inoculation of clover plants with TA1 increased the SDW to a mean of 4.9 mg per plant, ranging from 4.3 to 5.3 mg. Although TA1 significantly (P = 3.2 × 10−5) increased clover growth compared to the uninoculated control, its performance was relatively poor in supporting the growth of all four cultivars compared to the five novel Rlt isolates tested (Fig. 5). The average biomass for cultivars inoculated with the novel Rlt isolates ranged from 6.4 to 7.1 mg, indicating the higher symbiotic potential of all four tested cultivars with the novel Rlt inoculants (Fig. 5).
Symbiotic potential of Rlt isolates with four white clover cultivars. The SP of individual isolates was calculated relative to TA1 (i.e. TA1 = 100%). Data shown as mean ± s.e. (n = 3). Statistical significance is indicated by different letters across rhizobia treatments within each cultivar. Treatments that share a common letter within each cultivar do not differ at 5% significance level. Statistically significant differences were found in each ANOVA with the Fisher’s least significant difference (l.s.d.) method.
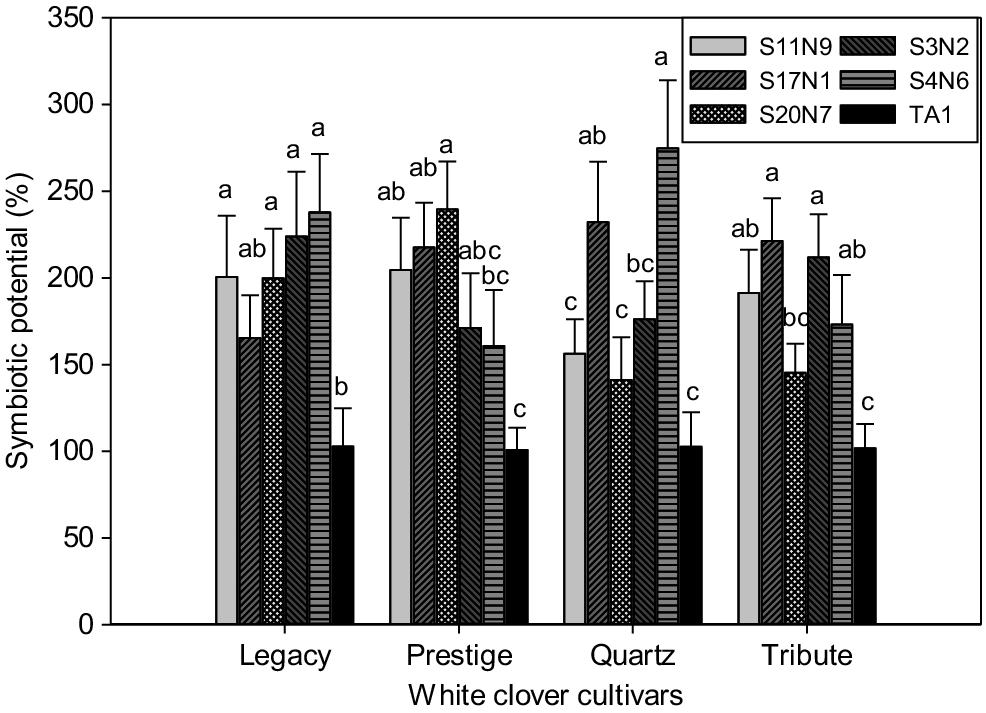
Clover cultivar–rhizobia isolate interactions appeared to have a significant effect (P < 0.05) on symbiosis as measured by SPs, as shown by inconsistency in isolate performance across the four white clover cultivars examined (Fig. 5). The SP of Rlt isolate S20N7 varied across the different cultivars, with highest SPs achieved with Legacy and Prestige but SP similar to TA1 when paired with Quartz or Tribute. S11N9 performed well (as measured by SP assay) with Legacy, Prestige and Tribute. S4N6 also performed well with Legacy, Quartz and Tribute.
When assessing isolate performance from a cultivar perspective, Legacy and Tribute had better symbiotic performance (as measured by shoot biomass) with four of the five novel isolates compared to TA1, although not the same four isolates (Fig. 5). In contrast, Prestige performed significantly better with three isolates than TA1, and Quartz with only two isolates. Interestingly, Quartz grew well with these two isolates (S17N1 and S4N6), resulting in the highest symbiotic potential relative to TA1 (~230–275%) (Fig. 5).
Discussion
Field performance of NZ Rlt isolates
R. leguminosarum bv. trifolii isolates vary in their ability to form nodules with white clover (Howieson et al. 2005; Rigg et al. 2021), and also in the subsequent effectiveness of nitrogen fixation during symbiosis (Rys and Bonish 1981). Although dedicated selection and screening programmes elsewhere have played a vital role in ensuring various legume species are matched with an optimal rhizobia symbiont (Bullard et al. 2005), there has been no significant effort in selection of isolates for white clover under New Zealand conditions for more than 50 years. This is despite the economic importance of white clover and biological nitrogen fixation to New Zealand.
Following decades of seed inoculation, Rlt populations have become naturalised in NZ pasture soils, but it is clear from previous work (Wakelin et al. 2018) and the current study, these naturalised isolates vary widely in their symbiotic potential, as shown in Fig. 1. This finding supports previous observations that inoculation response is plausible in many locations given the poor effectiveness of rhizobia resident in some soils (Wakelin et al. 2018), especially if isolates superior to TA1 can be made available commercially.
This study screened >230 Rlt isolates for their SP with white clover cultivar Tribute and selected 23 isolates for further assessment in a glasshouse pot trial and three field trials. Over 38% of the tested isolates showed significantly higher SP with white clover in comparison to TA1 in the primary bioassay. Although only a handful of these isolates could be tested in the field, two of them (S11N9 and S20N7) significantly increased clover growth compared to the paired uninoculated control at several harvests during three field trials. In comparison, the current inoculum strain TA1 showed no significant impact on clover growth in Trials 1 and 2 (Fig. 3). There was, however, one instance where an isolate (S4N6 at the second harvest in trial 3) increased SDW in direct comparison with TA1. In some cases, amounts of total fixed N were increased with these NZ isolates compared to the control, and in several cases were higher than TA1 (Fig. 4, S4N6). Although more work is needed, the results suggest that isolates S11N9, S20N7, S4N6 are potentially better options than TA1 for the inoculation of white clover in New Zealand.
All three trials were conducted in the presence of large resident populations of clover rhizobia that showed 61–118% of the N fixation capacity of TA1. In comparison, the N fixation capacity of isolates S11N9 and S20N7 exceeded that of TA1 by 68% and 37%, respectively, in the in vitro assays. Even with the competitive pressure for nodulation provided by the resident soil rhizobia, isolates S11N9 and S20N7 delivered significant improvements in clover growth compared to the uninoculated control. Whilst Lowther and Kerr (2011) previously concluded that white clover inoculation is unlikely to be beneficial, this study has shown that there is still potential for inoculation benefit, but that it is reliant on the competence of the inoculant used. Competitiveness for nodulation is an important trait that can increase the likelihood of inoculation response provided by elite rhizobia isolates (Graham 2008; Onishchuk et al. 2017; Irisarri et al. 2019), and is prerequisite in New Zealand where most pasture soils have large resident populations of clover rhizobia (Lowther and Kerr 2011; Wakelin et al. 2018). In a previous study (Shi et al. 2019), where the competitiveness of several NZ rhizobia isolates was tested against TA1 using an in vitro assay, some were more competitive than TA1. For example, when inoculated in similar CFU numbers, 80% of white clover nodules were occupied by isolate S11N9 whereas only 20% of nodules were occupied by TA1. Similar nodule occupancy results were found for S4N6 (Shi et al. 2019) and may explain in part why these NZ isolates increased the growth of white clover in the field. In contrast, TA1 is generally considered to have low competitiveness in soils with the presence of background rhizobia populations. A recent study by Rigg et al. (2021) reported that the dominant nodule occupant for several clover cultivars (including Tribute) in a field trial in Australia was not the inoculant TA1 but a WSM1325-like isolate.
Challenges of assessing performance of inoculants in the field
Field assessments of the performance of Rlt isolates were challenging due to the high variability in the number and symbiotic potential of the resident soil rhizobia at each site (Table 1). High variability of clover biomass was detected in all three trials. In the control treatments (no addition of Rlt inoculant, symbiosis relying on resident soil rhizobia), clover biomass varied by up to 10-fold. The heterogeneity of some soil properties (e.g. micronutrients, pH, soil moisture) in the same plot can be high (Hayes et al. 2022) and could also contribute to the observed variability of control clover plants. The impact of this heterogeneity between treatments was minimised in this study by employing the pair-wise line sowing strategy. However, more replicated blocks may be needed to reliably assess the performance of rhizobia isolates in future field studies.
Inconsistency between lab assay results and field performance
In our study, TA1 and many other isolates (Fig. 1) showed consistent nodulation and high symbiotic potentials in the lab bioassays and the glasshouse pot trial, but their promising performance was not repeated in the field. Such inconsistency between field testing and lab assay/glasshouse trials has been commonly reported (Sessitsch et al. 2019), as often the conditions of laboratory assays are simplified and highly consistent compared to more complex and variable field environments. Studies have shown that soil physicochemical properties (e.g. soil moisture, pH, nutrient levels) influence the performance of rhizobia in forming symbioses with legume hosts and that the effects can be isolate dependent (McNeil 1982; Zahran 1999; Slattery et al. 2001; Rigg et al. 2021). For example, Nguyen et al. (2020) reported that the symbiosis of Bradyrhizobium diazoefficiens USDA110 with soybean was inhibited in the presence of 12.5 mM nitrate, whereas other isolates (NKS4, NKM2 and NKTG2) formed N-fixing nodules even at 20 mM nitrate. The nitrate tolerances of the rhizobia isolates examined in our study are unknown but are likely to vary. Abdel-Salam et al. (2010) reported that variable nodulation and growth occurred under drought conditions and exposure to salinity and heavy metals among tested R. leguminosarum bv. trifolii isolates. Our previous work also demonstrated differences in the desiccation tolerance of Rlt isolates (van Ham et al. 2016), which could further contribute to the inconsistent performance of New Zealand isolates in the field.
To increase the success rates of finding elite isolates for field application, additional steps could be included in the screening stage. Although some factors are likely to be location dependent (e.g. soil pH extremes), others will be common across sites. For example, one of the key challenges for inoculation of New Zealand pastures is the competitiveness and, in some situations, high abundance of naturalised soil rhizobia populations. Assessment of isolates with high SP for their competitiveness against common soil rhizobia could be critical here. In addition, high drought tolerance could be another important trait for successful rhizobia isolates in the field. Isolates encounter desiccation conditions not only in soil but also during the seed coating process commonly used in New Zealand. Furthermore, with improved understanding of rhizobium genetics, genetic screening for key traits ideally in a high-throughput format may become possible. For example, nodD2 was recently shown to be linked to improved competitive performance in Rlt isolates (Ferguson et al. 2020). Understanding the molecular mechanisms of microbial robustness, the stability of phenotypes (e.g. symbiosis) under changing conditions, and quantification of such robustness is a newly emerging topic (Olsson et al. 2022; Trivellin et al. 2022). Incorporation of such knowledge and assessment in the initial screening step would enhance selection of elite isolates.
N fixation capacity with different white clover cultivars
Strain TA1 has been used to inoculate white clover since the 1950s, preceding by decades the development of modern white clover cultivars currently grown in New Zealand. Most previous research with TA1 was undertaken using clover cultivar ‘Grasslands Huia’, which was grown widely during the 1960s (Bonish 1980; Woodfield et al. 2001). It is well known that rhizobia isolates can form symbioses of varying effectiveness with different clover cultivars (Sherwood and Masterson 1974; Harrison et al. 1989; Melino et al. 2012). For example, Rigg et al. (2021) reported varied legume–rhizobium cross-host compatibility and symbiosis effectiveness in both pot and field trials. Similarly, our study demonstrated significant differences in the interactions between the Rlt isolates and white clover cultivars (Fig. 5). For example, S4N6 was the best isolate for use with cultivars Quartz and Legacy, whereas S20N7 performed best with Prestige. Isolates S11N9 and S4N6 showed better performance with Tribute than with the other tested isolates (Fig. 5). These results also suggest that screening for symbiotic potential using a single clover cultivar may not provide an accurate assessment of a novel isolate’s performance across a wider range of white clover genetics. However, due to the resources required to widen isolate screens to more than one clover cultivar, a pragmatic solution is what has been applied in this study – to perform the initial screen with a single test cultivar and then expand to a wider assessment for isolates showing similar or greater performance than TA1. In the assay with multiple cultivars, TA1 had the lowest symbiotic potential (although significantly increased clover growth in comparison to uninoculated control) with all four tested cultivars, indicating TA1 may not be the best choice for the modern white clover cultivars and may explain part of the improved growth of cv. Tribute inoculated with alternative isolates in the field. Although optimising an Rlt isolate with an individual cultivar is feasible (e.g. S4N6 for Quartz), the challenge remains to identify an isolate that has high N fixation across all cultivars and is competent under competitive field conditions.
Fig. 5 also highlights that clover genetics influence the interaction with Rlt isolates and suggests there is value in improving the clover and Rlt isolate pairing. Recent work by Weith et al. (2022) in a white clover breeding pool characterised the clover quantitative genetics of this symbiosis, indicating opportunities to breed for improved white clover growth in symbiosis with Rlt. In contrast, a study by Moeskjær et al. (2022) involved screening 170 European Rlt isolates across 20 clover cultivars. The authors concluded clover × rhizobia interactions were not significant for any trait they measured, and that initial plant size had a greater influence on symbiotic yield than variation among rhizobium isolates themselves. It may be that this was due to the use of clover stolon cuttings rather than seedlings since significant clover cultivar × rhizobium interactions highlighting the effect of Rlt isolate on symbiotic potential are routine outcomes in our experimental protocols and those reported by others (Sherwood and Masterson 1974; Harrison et al. 1989; Melino et al. 2012; Rigg et al. 2021).
Future opportunities
There are large populations of clover rhizobia present in most pasture sites in New Zealand after decades of seed inoculation practice. However, they often vary in abundance and N fixation capacity, which is associated with soil variation across paddocks (Wakelin et al. 2018). Although isolates S11N9 and S20N7 delivered significant improvements in clover growth in the field, work is needed to understand if the benefits extend to other environments that differ in soil texture, moisture, fertility, and especially pH, as both the persistence of rhizobia and nodulation processes are known to be detrimentally affected in very acidic soils (Evans et al. 1988; Melino et al. 2012). Compatibility of the isolates also needs to be confirmed with other white clover cultivars, other perennial clovers and annual clover species (especially subterranean clover) to ensure that ineffective symbiotic associations do not occur. Whilst the formation of ineffective symbioses between resident rhizobia and clovers is commonplace (Drew et al. 2011), such an outcome would not be desirable for an inoculant isolate.
It would be useful to better understand the relative contribution of the traits in the New Zealand isolates that supported their improved field performance to help streamline future selection efforts. The extent to which improved desiccation tolerance, competitive ability and/or N fixation capacity underpinned the better performance of isolates S11N9 and S20N7 (and potentially for S4N6) remains to be understood. For example, intrinsic desiccation tolerance is a desirable attribute for rhizobia inoculants as poor survival of rhizobia during commercial formulation and seed application can limit their efficacy in the field (Deaker et al. 2004, 2012). Equally, it would be useful to understand the primary limitation behind TA1’s poor field performance so that deficiency is not overlooked in future selection programs. Once established, white clover pastures can persist for many years, supported by regeneration from stolons reliant on the resident soil rhizobia for nodulation (Jahufer et al. 2013). Whilst the persistence of inoculant isolates is often regarded as transient (Gibson et al. 1976), variation is reported between isolates. The extent to which the NZ isolates provide enduring benefits to the pasture is an area requiring further investigation.
Conclusions
Although Rlt populations have become naturalised in many New Zealand pasture soils, inoculation of white clover cv. Tribute with rhizobia other than TA1 significantly increased clover SDW in field trials. This demonstrates that there are benefits from inoculating white clover if improved inoculant isolates are available. Two isolates (S11N9 and S20N7) enhanced clover growth in at least one harvest for all three trials. Inoculation with isolate S4N6 also resulted in more clover growth than the paired uninoculated control in Trial 2 and TA1 in Trial 3, although each at one harvest date only. A feature of these isolates is their greater N fixation capacity relative to TA1. This improvement was measured with four common white clover cultivars currently grown in New Zealand. Although more field trials are needed, these isolates have shown sufficient improvement to warrant their further assessment as potential commercial inoculants for use with white clover in New Zealand.
Development of a suitable commercial rhizobial inoculant involves many steps, from initial isolation, identification and characterisation, and assessment for symbiotic potential by laboratory bioassay, through to further validation in pot trials and field trials. Isolates must also be suitable for industrial production (amenable to mass production, formulation, etc.) and perform effectively in field trials under a range of environmental conditions, soil types and differing farming practices (Bashan et al. 2014). This study reports on the first research steps towards identification of new inoculants for white clover, targeting the cultivars currently used in New Zealand. To capture the benefits of these new rhizobia on farm, the isolates need to be amenable to large scale production and tolerant of environmental stresses imposed during formulation and seed inoculation processes. Further work is planned to ensure these requirements are met and to confirm that the benefits of the new isolates extend to other white clover cultivars and regions of New Zealand.
Data availability
The data that support this study may be shared upon reasonable request to the corresponding author if appropriate.
Declaration of funding
This project was funded by New Zealand Ministry of Business, Innovation and Employment (‘Improving legume-rhizobia symbiosis’; Contract No. C10X1308), and DairyNZ (CB1902). The sponsors had no role in the preparation of data or manuscript or in the decision to submit for publication.
Acknowledgements
We would like to thank David Wright, Laura Villamizar, Caitlin Hyde, Jill Gibson, Russell Cory, Anthony Hilditch and Marie Foxwell, Craig Anderson and Sean Weith (AgResearch) for technical support and useful discussions through the whole research program. Also, we thank Shaun Monk (Grasslanz Technology Ltd) for field preparation and maintenance.
References
Abdel-Salam MS, Ibrahim SA, Adb-El-Halim MM, Badawy FM, Abo-Aba SEM (2010) Phenotypic characterization of indigenous Egyptian Rhizobial strains for abiotic stresses performance. Journal of American Science 6, 498-503.
| Google Scholar |
Bashan Y, de-Bashan LE, Prabhu SR, Hernandez J-P (2014) Advances in plant growth-promoting bacterial inoculant technology: formulations and practical perspectives (1998–2013). Plant and Soil 378, 1-33.
| Crossref | Google Scholar |
Boddey RM, Peoples MB, Palmer B, Dart PJ (2000) Use of the 15N natural abundance technique to quantify biological nitrogen fixation by woody perennials. Nutrient Cycling in Agroecosystems 57, 235-270.
| Crossref | Google Scholar |
Bonish PM (1980) Nodulation of white clover: plant influences on the effectiveness of Rhizobium trifolii. New Zealand Journal of Agricultural Research 23, 239-242.
| Crossref | Google Scholar |
Braakhekke MC, Rebel KT, Dekker SC, Smith B, Beusen AHW, Wassen MJ (2017) Nitrogen leaching from natural ecosystems under global change: a modelling study. Earth System Dynamics 8, 1121-1139.
| Crossref | Google Scholar |
Brockwell J, Holliday RA, Pilka A (1988) Evaluation of the symbiotic nitrogen-fixing potential of soils by direct microbiological means. Plant and Soil 108, 163-170.
| Crossref | Google Scholar |
Bullard GK, Roughley RJ, Pulsford DJ (2005) The legume inoculant industry and inoculant quality control in Australia: 1953-2003. Australian Journal of Experimental Agriculture 45, 127-140.
| Crossref | Google Scholar |
Caradus JR, Hay MJM, Mackay AD, Thomas VJ, Dunlop J, Lambert MG, Hart AL, van den Bosch J, Wewala S (1993) Variation within white clover (Trifolium repens L.) for phenotypic plasticity of morphological and yield related characters, induced by phosphorus supply. New Phytologist 123, 175-184.
| Crossref | Google Scholar |
Caradus JR, Clifford PTP, Chapman DF, Cousins GR, Williams WM, Miller JE (1997) Breeding and description of ‘Grasslands Sustain’, a medium-large leaved white clover (Trifolium repens L.) cultivar. New Zealand Journal of Agricultural Research 40, 1-7.
| Crossref | Google Scholar |
Deaker R, Roughley RJ, Kennedy IR (2004) Legume seed inoculation technology – a review. Soil Biology and Biochemistry 36, 1275-1288.
| Crossref | Google Scholar |
Deaker R, Hartley E, Gemell G (2012) Conditions affecting shelf-life of inoculated legume seed. Agriculture 2, 38-51.
| Crossref | Google Scholar |
Delestre C, Laugraud A, Ridgway H, Ronson C, O’Callaghan M, Barrett B, Ballard R, Griffiths A, Young S, Blond C, Gerard E, Wakelin S (2015) Genome sequence of the clover symbiont Rhizobium leguminosarum bv. trifolii strain CC275e. Standards in Genomic Sciences 10, 121.
| Crossref | Google Scholar |
Drew EA, Charman N, Dingemanse R, Hall E, Ballard RA (2011) Symbiotic performance of Mediterranean Trifolium spp. with naturalised soil rhizobia. Crop & Pasture Science 62, 903-913.
| Crossref | Google Scholar |
Evans J, Hochman Z, O’Connor GE, Osborne GJ (1988) Soil acidity and Rhizobium: their effects on nodulation of subterranean clover on the slopes of southern New South Wales. Australian Journal of Agricultural Research 39, 605-618.
| Crossref | Google Scholar |
Ferguson S, Major AS, Sullivan JT, Bourke SD, Kelly SJ, Perry BJ, Ronson CW, Vieille C (2020) Rhizobium leguminosarum bv. trifolii NodD2 enhances competitive nodule colonization in the clover-rhizobium symbiosis. Applied and Environmental Microbiology 86, e01268-20.
| Crossref | Google Scholar |
Ford JL, Cousins GR, Jahufer Z, Baird IJ, Woodfield DR, Barrett BA (2015) Grasslands legacy – a new, large-leaved white clover cultivar with broad adaption. Journal of New Zealand Grasslands 77, 211-218.
| Crossref | Google Scholar |
Gibson AH, Date RA, Ireland JA, Brockwell J (1976) A comparison of competitiveness and persistence amongst five strains of Rhizobium tripolii. Soil Biology and Biochemistry 8, 395-401.
| Crossref | Google Scholar |
Greenwood RM (1965) Populations of rhizobia in New Zealand soils. Proceedings of the New Zealand Grasslands Association 26, 95-101.
| Google Scholar |
Griffiths AG, Moraga R, Tausen M, Gupta V, Bilton TP, Campbell MA, Ashby R, Nagy I, Khan A, Larking A, Anderson C, Franzmayr B, Hancock K, Scott A, Ellison NW, Cox MP, Asp T, Mailund T, Schierup MH, Andersen SU (2019) Breaking free: the genomics of allopolyploidy-facilitated niche expansion in white clover. The Plant Cell 31, 1466-1487.
| Crossref | Google Scholar |
Harrison SP, Young JPW, Jones DG (1989) Rhizobium population genetics: host preference and strain competition effects on the range of Rhizobium leguminosarum biovar Trifolii genotypes isolated from natural populations. Soil Biology and Biochemistry 21, 981-986.
| Crossref | Google Scholar |
Hayes RC, Rohan M, Li GD, Orgill SE, Poile GJ, Oates AA, Conyers MK (2022) The nature of spatial variability of four soil chemical properties and the implications for soil sampling. Journal of Soils and Sediments 22, 3006-3017.
| Crossref | Google Scholar |
Högberg P (1997) Tansley review no. 95 15N natural abundance in soil-plant systems. New Phytologist 137, 179-203.
| Crossref | Google Scholar |
Howieson JG, Yates RJ, O’Hara GW, Ryder M, Real D (2005) The interactions of Rhizobium leguminosarum biovar trifolii in nodulation of annual and perennial Trifolium spp. from diverse centres of origin. Australian Journal of Experimental Agriculture 45, 199-207.
| Crossref | Google Scholar |
Irisarri P, Cardozo G, Tartaglia C, Reyno R, Gutiérrez P, Lattanzi FA, Rebuffo M, Monza J (2019) Selection of competitive and efficient rhizobia strains for white clover. Frontiers in Microbiology 10, 768.
| Crossref | Google Scholar |
Jahufer MZZ, Dunn A, Baird I, Ford JL, Griffiths AG, Jones CS, Woodfield DR, Barrett BA (2013) Genotypic variation for morphological traits in a white clover mapping population evaluated across two environments and three years. Crop Science 53, 460-472.
| Crossref | Google Scholar |
Jahufer MZZ, Ford JL, Cousins GR, Woodfield DR (2021) Relative performance of white clover (Trifolium repens) cultivars and experimental synthetics under rotational grazing by beef cattle, dairy cattle and sheep. Crop & Pasture Science 72, 926-938.
| Crossref | Google Scholar |
Lowther WL, Kerr GA (2011) White clover seed inoculation and coating in New Zealand. Proceedings of the New Zealand Grassland Association 73, 93-102.
| Crossref | Google Scholar |
McNeil DL (1982) Variations in ability of Rhizobium japonicum strains to nodulate soybeans and maintain fixation in the presence of nitrate. Applied and Environmental Microbiology 44, 647-652.
| Crossref | Google Scholar |
Melino VJ, Drew EA, Ballard RA, Reeve WG, Thomson G, White RG, O’Hara GW (2012) Identifying abnormalities in symbiotic development between Trifolium spp. and Rhizobium leguminosarum bv. trifolii leading to sub-optimal and ineffective nodule phenotypes. Annals of Botany 110, 1559-1572.
| Crossref | Google Scholar |
Moeskjær S, Skovbjerg CK, Tausen M, Wind R, Roulund N, Janss L, Andersen SU (2022) Major effect loci for plant size before onset of nitrogen fixation allow accurate prediction of yield in white clover. Theoretical and Applied Genetics 135, 125-143.
| Crossref | Google Scholar |
Nguyen HP, Miwa H, Obirih-Opareh J, Suzaki T, Yasuda M, Okazaki S (2020) Novel rhizobia exhibit superior nodulation and biological nitrogen fixation even under high nitrate concentrations. FEMS Microbiology Ecology 96, fiz184.
| Crossref | Google Scholar |
Olsson L, Rugbjerg P, Torello Pianale L, Trivellin C (2022) Robustness: linking strain design to viable bioprocesses. Trends in Biotechnology 40, 918-931.
| Crossref | Google Scholar |
Onishchuk OP, Vorobyov NI, Provorov NA (2017) Nodulation competitiveness of nodule bacteria: genetic control and adaptive significance: review. Applied Biochemistry and Microbiology 53, 131-139.
| Crossref | Google Scholar |
Rigg JL, Webster AT, Harvey DM, Orgill SE, Galea F, Dando AG, Collins DP, Harris CA, Newell MT, Badgery WB, Hayes RC (2021) Cross-host compatibility of commercial rhizobial strains for new and existing pasture legume cultivars in south-eastern Australia. Crop & Pasture Science 72, 652-665.
| Crossref | Google Scholar |
Rys GJ, Bonish PM (1981) Effectiveness of Rhizobium trifolii populations associated with Trifolium species in Taranaki, New Zealand. New Zealand Journal of Experimental Agriculture 9, 329-335.
| Crossref | Google Scholar |
Sessitsch A, Pfaffenbichler N, Mitter B (2019) Microbiome applications from lab to field: facing complexity. Trends in Plant Science 24, 194-198.
| Crossref | Google Scholar |
Sherwood MT, Masterson CL (1974) Importance of using the correct test host in assessing the effectiveness of indigenous populations of Rhizobium Trifolii. Irish Journal of Agricultural Research 13, 101-108.
| Google Scholar |
Shi S, Villamizar L, Gerard E, Ronson C, Wakelin S, Ballard R, Caradus JR, O’Callaghan M (2019) Increasing biological nitrogen fixation by white clover-rhizobia symbiosis. Journal of New Zealand Grasslands 81, 231-234.
| Crossref | Google Scholar |
Slattery JF, Coventry DR, Slattery WJ (2001) Rhizobial ecology as affected by the soil environment. Australian Journal of Experimental Agriculture 41, 289-298.
| Crossref | Google Scholar |
Soumare A, Diedhiou AG, Thuita M, Hafidi M, Ouhdouch Y, Gopalakrishnan S, Kouisni L (2020) Exploiting biological nitrogen fixation: a route towards a sustainable agriculture. Plants 9, 1011.
| Crossref | Google Scholar |
StatsNZ (2021) Fertilisers – nitrogen and phosphorus. Available at https://www.stats.govt.nz/indicators/fertilisers-nitrogen-and-phosphorus
Trivellin C, Olsson L, Rugbjerg P (2022) Quantification of microbial robustness in yeast. ACS Synthetic Biology 11, 1686-1691.
| Crossref | Google Scholar |
van Ham R, O’Callaghan M, Geurts R, Ridgway HJ, Ballard R, Noble A, Macara G, Wakelin SA (2016) Soil moisture deficit selects for desiccation tolerant Rhizobium leguminosarum bv. trifolii. Applied Soil Ecology 108, 371-380.
| Crossref | Google Scholar |
Wakelin S, Tillard G, van Ham R, Ballard R, Farquharson E, Gerard E, Geurts R, Brown M, Ridgway H, O’Callaghan M (2018) High spatial variation in population size and symbiotic performance of Rhizobium leguminosarum bv. trifolii with white clover in New Zealand pasture soils. PLoS ONE 13, e0192607.
| Crossref | Google Scholar |
Weith SK, Jahufer MZZ, Hofmann RW, Anderson CB, Luo D, Ehoche OG, Cousins G, Jones EE, Ballard RA, Griffiths AG (2022) Quantitative genetic analysis reveals potential to breed for improved white clover growth in symbiosis with nitrogen-fixing Rhizobium bacteria. Frontiers in Plant Science 13, 953400.
| Crossref | Google Scholar |
Woodfield DR, Clifford PTP, Cousins GR, Ford JL, Baird IJ, Miller JE, Woodward SL, Caradus JR (2001) Grasslands Kopu II and Crusader: new generation white clovers. Proceedings of the New Zealand Grasslands Association 63, 103-108.
| Crossref | Google Scholar |
Woodfield DR, Clifford PTP, Baird IJ, Cousins GR, Miller JE, Widdup KH, Caradus JR (2003) Grasslands tribute: a multi-purpose white clover for Australasia. Proceedings of the New Zealand Grassland Association 65, 157-162.
| Crossref | Google Scholar |
Zahran HH (1999) Rhizobium-legume symbiosis and nitrogen fixation under severe conditions and in an arid climate. Microbiology and Molecular Biology Reviews 63, 968-989.
| Crossref | Google Scholar |