Taking shortcuts: lowering harvest height to restrict colonisation of cereal stubble by Fusarium pseudograminearum
Toni Petronaitis

A
B
C
Abstract
Many wheat producers are increasing the biomass of cereal stubble retained after harvest through the adoption of stripper front harvesters, which result in taller standing stubble.
We investigated whether taller stubble affects the survival and dispersal of Fusarium pseudograminearum (Fp), the causative agent of Fusarium crown rot (FCR).
Field experiments at two sites in northern New South Wales were run for 3 years to investigate whether taller cereal stubble in Year 1 facilitated additional Fp colonisation, and subsequent effects on dispersal of Fp inoculum from chickpea harvest in Year 2 and FCR infection and expression in cereal crops in Year 3. Culturing and quantitative polymerase chain reaction (qPCR) methods assessed Fp colonisation and future disease risks.
In taller cereal stubble, Fp colonised an additional 91–92% of the stubble length in the 6 months post-harvest and persisted at higher levels for at least 1 year than did the shorter cereal stubble. Cutting cereal stubble short (in Year 1) therefore successfully restricted further colonisation by Fp. Significant displacement of Fp in the crown 6 months post-harvest resulted in significant decreases in Fp DNA overall; however, long-term survival of Fp was observed 10–20 cm above the crown.
Different residue management scenarios did not increase FCR risk for Year 3, likely owing to high inoculum levels across all treatments and unseasonably wet conditions in Years 2–3.
We provide important field-validation of Fp colonisation in standing cereal stubble and discuss implications for FCR management across regions and seasons.
Keywords: chickpea, disease management, Fusarium crown rot, harvest, rotation, stubble, tillage, wheat.
Introduction
Fusarium crown rot (FCR) is a significant disease of winter cereal crops across the northern grain region of Australia (NGR) (northern New South Wales and southern Queensland). Fusarium pseudograminearum (Fp) is the main Fusarium species causing FCR in this region (Akinsanmi et al. 2004). This pathogen is particularly difficult to control in conservation agriculture systems because of its ability to survive within cereal residues for up to 3 years (Summerell and Burgess 1988). Fungal hyphae surviving within infected cereal or grass weed residues provide inoculum for future seasons. Infection of susceptible cereal crops, such as wheat and barley, occurs through the roots, crown, lower culm, or leaf sheath. This can result in symptoms characteristic of FCR such as basal browning, dead (white) heads, and pinched grain, which ultimately result in yield loss (Alahmad et al. 2018).
Changes in tillage practices, particularly stubble retention, has led to an increase in FCR incidence in Australia (reviewed in Simpfendorfer et al. 2019). Potential yield loss caused by FCR alone increased by 19.2% in the 20 years from 1988 to 2008 (Murray and Brennan 2009). The increase in risk and yield loss to FCR has been associated with the adoption of cereal residue retention (particularly standing cereal stubble), because pathogen survival is favoured in intact residues (Wildermuth et al. 1997; Verrell et al. 2017). The additional soil moisture provided by retention of cereal crop residues may also promote infection of new crops by meeting water potential requirements of pathogens such as Fp to infect a susceptible host (Swan et al. 2000). The positive benefits that retained cereal stubble have on soil structure, soil moisture, and soil fertility are a major priority for growers. As such, adoption of this practice has continued regardless of any associated yield penalties from the increase in FCR risk (Alahmad et al. 2018). Tillage and burning are effective at reducing FCR inoculum, but these are often deemed incompatible with conservation agriculture practices. As such, integrated disease management strategies that allow for stubble retention have become industry standard (Simpfendorfer et al. 2019). Some strategies can lower FCR incidence through inter-row sowing to avoid Fp in cereal rows from previous years or allowing additional time for inoculum break-down by using non-host rotations (Verrell et al. 2017). Even so, FCR often recurs due to persistence of background inoculum or growers returning to susceptible cereal crops prematurely to maximise short-term profits.
Complicating FCR management further is the adoption of new cropping practices that could increase the persistence and distribution of Fp in stubble retention systems. Stripper front harvesting systems, for example, increase standing stubble biomass and harvest efficiency through rapid ‘stripping’ of heads during harvest (Tado et al. 1998). This results in taller standing stubble (50–60 cm height) than with a conventional/combine harvester (~30 cm height). Taller stubble means more stubble biomass, and the potential to influence stubble microclimatic conditions such as wind speed and temperature (Broster et al. 2020). A significant consequence of taller cereal stubble is that Fp could colonise the additional stubble (a readily available carbon resource) as a pioneer coloniser. Pathogenic Fusarium species have an advantage over other field saprotrophs when it comes to colonising stubble, allowing rapid colonisation with little competition (Pereyra et al. 2004). In the laboratory, Fp can colonise sterile bread wheat (Triticum aestivum), durum wheat (Triticum durum), oat (Avena sativa) and barley (Hordeum vulgare) as quickly as 1 cm per day under moist (~98% relative humidity) conditions (Petronaitis et al. 2022). Colonisation of cereal stubble by Fp has been observed within cereal residues following wet summer fallow conditions (Summerell and Burgess 1988), but remains to be experimentally validated in the field.
In Australia, pulses have become an important rotation crop choice for cereal growers, with market demand being expected to increase in future (reviewed in Olita et al. 2024). Chickpea is the predominating pulse crop in both Australia and the NGR (reviewed in Olita et al. 2024). Chickpea crops are of short stature, requiring low mechanical harvest heights to capture grain set in pods produced at the bottom of the chickpea canopy, while still allowing for header ground clearance (Singh et al. 2019). Hypothetically, chickpea planted into retained standing cereal stubble infected with Fp could result in the dispersal of Fp inoculum across the harvested area mixed in with the chickpea header trash. This could undo any efforts to restrict FCR inoculum to retained cereal rows from previous years, reducing the benefits of inter-row sowing in a cereal–chickpea–cereal rotation.
Kelly-chaining (a form of shallow tillage) may also be applied to cereal stubble and results in the spreading and shallow (0–10 cm) incorporation of cereal stubble into the soil surface (Kelly Tillage 2022). It is typically applied before sowing chickpea to increase the rate of cereal stubble decomposition and improve planting efficiency. Kelly-chaining may alternatively be applied after sowing to flatten furrows and reduce the risk of herbicide residue damage to chickpea seedlings. However, tillage can redistribute any FCR inoculum originally contained within retained standing cereal rows (Simpfendorfer et al. 2019). Following Kelly-chaining, Fp surviving within cereal stubble fragments may be incorporated into the soil surface layer where the pathogen can more readily infect susceptible cereal crops within future rotation sequences.
Stripper front headers and short-stature break crop rotations (with or without Kelly-chaining) have the potential to increase Fp inoculum and inoculum dispersal, yet their effect on FCR risk is currently unknown. We therefore investigated whether retaining taller standing cereal stubble (a likely outcome of the use of stripper front headers) could promote further saprotrophic growth of Fp compared with short or conventional-height cereal stubble. We hypothesised that taller standing cereal stubble would result in more extensive vertical saprotrophic colonisation by Fp after harvest compared to shorter harvest heights. If true, stripper front headers may not be recommended in high FCR-risk scenarios; reducing the harvest height of cereals infected with Fp may be beneficial to prevent further saprotrophic colonisation of stubble in these cases. We also tracked Fp DNA in a range of cereal stubble management treatments across multiple seasons (including a chickpea rotation) to determine whether any of the stubble management approaches tested were associated with a reduction in FCR risk over time.
Materials and methods
Site preparation and establishment of cereal stubble treatments: Years 1–2 (2019–20)
Two field experiments were conducted in the NGR, at the Australian Cotton Research Institute located in Narrabri NSW (30°20′S, 149°60′E) (flood irrigated) and the Liverpool Plains Field Station located in Breeza NSW (31°17′S, 150°42′E) (dryland). Soil types were grey vertisol with high clay content at both Narrabri and Breeza (Isbell 2021). Rainfall is highest between November and February, with a mean annual rainfall of 584 mm at Narrabri and 684 mm at Breeza. Site-wide background pathogen DNA concentrations were determined using PREDICTA® B Northern panel test (South Australian Research and Development Institute, Adelaide, SA, Australia) prior to sowing in 2019.
Durum wheat cultivar DBA Lillaroi was mixed with Fp-colonised grain inoculum (2 g/m row) at sowing in May 2019 (Year 1) to establish cereal stubble with extensive Fp colonisation as described by Forknall et al. (2019). The Fp inoculum contained a mixture of five Fp isolates collected post-harvest from experiments conducted across NSW in 2018. Irrigation was used to establish the crop at both sites because of persisting dry conditions.
Each experiment consisted of a total of 54 plots (10 × 2 m), arranged in a rectangular array of 6 columns × 9 rows (Narrabri) or 18 columns × 3 rows (Breeza). This enabled the application of 18 treatments in total, where each treatment was randomly applied to plots in each experiment according to a randomised complete block design, with three replicate blocks. The experiment consisted of nine cereal stubble management treatments, formed through a combination of harvest height (short, medium, or tall) (Table 1), harvest trash (retained or removed) and shallow tillage (Kelly-chained or intact). Two crop species treatments (durum wheat or bread wheat) were then applied in 2021 (Year 3). Supplementary Table S2 shows the number of duplicated crops per replicate. Timings of each treatment in the context of seasonal conditions and crop rotation are presented in Fig. 1.
Harvest height | Breeza, NSW | Narrabri, NSW | |
---|---|---|---|
Short (cm) | 13 | 17 | |
Medium (cm) | 25 | 32 | |
Tall (cm) | 38 | 45 |
Differences in height between sites are due to differences in final crop height.
Treatment timings imposed on field experiments at Breeza and Narrabri in northern NSW, in relation to the seasonal rotation and rainfall experienced at each site. Months with an asterisk (*) indicate supplementary flood irrigation timing of 1 ML/ha (approximately equivalent to 75 mm of rainfall) applied to the Narrabri site only.
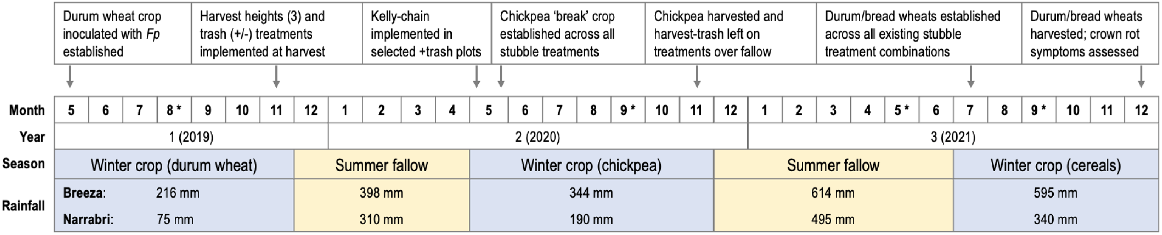
The harvest height and harvest trash treatments were applied at harvest of durum wheat in Year 1 and examples of these can be seen in Supplementary Fig. S1. The ‘trash-retained’ treatment involved retaining the header trash fraction during harvest and spreading trash evenly over the plot. The ‘trash-removed’ treatment involved collection of the trash fraction from the back of the header using a woolpack, which was subsequently transported off the experimental area. Prior to sowing chickpea in May 2020 (Year 2), the shallow tillage treatment (Kelly prickle chain, Kelly Engineering, South Australia) was imposed to the assigned Kelly-chain plots. This included two passes with a single prickle chain module on a 30 degree working angle. Stubble fragmentation was minimal, with majority of the residue spread across the plot surface and at the same length of the original harvest height, although some fragments were incorporated to a soil depth of approximately 3 cm. The treatment was applied in combination with the harvest height treatments, exclusively to plots that had previously had trash retained.
Soil moisture profiling: Years 1–2 (2019–20)
Soil moisture content (SMC) was measured on a plot basis in November 2019, May 2020, and November 2020. The SMC was not measured again in May 2021 because of significant rainfall experienced at both sites, preventing tractor access to the fields and the likelihood of fully recharged soil moisture profiles as a result. One 1.2 m soil core was sampled per plot and cut into 0–30 cm, 30–60 cm, 60–90 cm, and 90–120 cm segments. The wet weight and dry weight of each soil segment were measured to calculate gravimetric SMC (%) as follows:
whereby the wet weight (g) represents field moisture, and dry weight (g) was measured after the sample had been dried for 48 h at 105°C.
Recovery of pathogens in standing durum wheat stubble using agar culturing: Years 1–2 (2019–20)
Durum wheat stubble was collected from 30 plants that were still standing at random across each plot in November 2019 (durum wheat harvest, Year 1), May 2020 (chickpea sowing, Year 2), and November 2020 (chickpea harvest, Year 2). Plants were separated into individual tillers and 20 tillers per plot were then selected randomly for culturing and another 20 tillers per plot were retained for quantitative polymerase chain reaction (qPCR) analysis. Starting at the stem base (crown), a 1.5 cm segment was removed from each tiller every 5 cm along the entire tiller length. Stem portions were surface sterilised with 0.2% w/v sodium hypochlorite in 45% v/v ethanol for 1 min then washed with sterile distilled water. Samples were air-dried overnight and plated on 1/4 strength potato dextrose agar (PDA) + novobiocin (10 g PDA, 15 g technical agar plus 0.1 g novobiocin/L water) and incubated under alternating white and near ultraviolet lights (12 h photoperiod) for 7 days at 25°C. Pathogen incidence was recorded as the number of segments producing typical Fp colonies on the basis of morphology, with maximum vertical colonisation recorded as the top-most height (tiller segment) from which the pathogen was isolated.
Estimation of pathogen DNA in standing durum wheat stubble and header trash: Years 1–2 (2019–20)
For quantification of Fp DNA in standing stubble, the 20 standing durum wheat stubble tillers retained for qPCR analysis from each plot were cut into portions according to harvest height and site, i.e. three portions for the long harvest height treatments (0–17 cm, 17–32 cm and 32–45 cm at Narrabri, and 0–13 cm, 13–25 cm and 25–38 cm at Breeza), two portions for the medium harvest height treatments (0–17 cm and 17–32 cm at Narrabri, and 0–13 cm and 13–25 cm at Breeza), whereas short harvest height treatments remained as a single portion (0–17 cm at Narrabri, and 0–13 cm at Breeza). Samples were air-dried and excess dirt was removed with a brush. The stubble portions were then chopped into fragments of approximately 2.5 cm long and submitted to the South Australian Research and Development Institute (SARDI), Adelaide. Recombinant DNA probe sequences specific to Fp, F. culmorum and F. graminearum were used for pathogen quantification via qPCR. Protocols for DNA extraction from the cereal residues and subsequent analysis are proprietary with SARDI (Ophel-Keller et al. 2008).
For quantification of Fp DNA on the plot surface (purposefully excluding standing stubble), samples were collected at the following three times: following Kelly-chain implementation in May 2020, immediately prior to harvest in November 2020 and again immediately after harvest in November 2020. For the first two time points, samples were collected from the trash-retained and Kelly-chain plots only (the trash-removed plots had no material to collect). For the third time point, all plots were sampled and contained a mix of chickpea and wheat stubble. Sampling involved collecting five 1 L subsamples of residue from across the plot area, bulked on a plot basis. The subsamples were air-dried and chopped into fragments approximately 2.5 cm long. A final 200 mL subsample of this chopped and dried harvest residue was submitted to the South Australian Research and Development Institute (SARDI), Adelaide, as above.
Chickpea growth and yield: Year 2 (2020)
A chickpea crop (cv. PBA Seamer) was established in Year 2 (2020 season) of experimentation, with the chosen cultivar sown across all experimental plots in late May 2020. Chickpea was sown between the rows of standing stubble (if present) as per industry standard. Chickpea plant populations (plants/m2) were counted in each plot 30 days after planting. Lowest pod heights were measured on two random plants per plot prior to harvest in 2020 as the distance from ground level to lowest pod. Chickpea grain yield was determined from machine harvested samples taken from 2 × 10 m plots in November 2020.
Wheat crop: Year 3 (2021)
Durum wheat rated very susceptible to FCR (cv. DBA Lillaroi), and bread wheat rated moderately susceptible to FCR (cv. LRPB Hellfire) (Matthews et al. 2024) were established in Year 3 (2021 season). Significant rainfall experienced between May and July 2021 at both sites delayed sowing until the end of the sowing window (July 2021). The experiment was hand-sown at the Breeza site owing to wet soil preventing machinery access. The Year 3 crop was sown directly over the previous chickpea rows, corresponding to the inter-row of the Year 1 durum wheat crop, as per industry standard. Plant populations (plants/m2) were counted in each plot 30 days after planting. Grain yield was determined from machine harvested samples taken from 2 × 10 m plots in December 2021 (delayed because of minor flooding at both sites). Grain quality was determined for screenings (proportion of grain passing through 2.0 mm slotted screen), grain protein using near infrared spectroscopy (NIRS) and grain size based on 1000-grain weight.
The incidence and severity of FCR infection in the Year 3 durum and bread wheat crops were assessed after physiological maturity in post-harvest stubble collected on the day of harvest from each plot. Plants were randomly sampled (25 plants per plot) and the crown rot index (CRI) was visually determined as a measure of FCR incidence and severity, as described by Forknall et al. (2019). A 1.5 cm section was removed from the base of each primary tiller (starting approximately 1 cm above the roots) and cultured as described previously, to determine the incidence of plants infected by Fp (Fp incidence).
Statistical analysis
In total, 15 response variables were analysed separately for each experiment, by using a linear mixed model framework. Response variables were not analysed across experiments, as the harvest heights (short, medium and tall) represented different actual heights in each experiment (Table 1). The response variables considered broadly fit into four categories, being those measured at the plot level, those measured at the plot level over time, those measured at the plot level over time and depth, and those measured at the tiller segment level over time (Table S1). Transformations were applied as needed to ensure the model assumptions of normality and homogeneity of residual variance across the range of fitted values were met (Table S1).
The maximal treatment structure of the model fit to the response variables measured at the plot level can be specified using the notation of Wilkinson and Rogers (1973), as follows:
where × corresponds to the crossing operator. This treatment structure was adjusted to remove those treatments that had not been applied at the time the variable was measured. For example, the Crop species term was omitted from the treatment structure in the analysis of variables measured prior to this treatment being applied in Year 3 (Table S1). All terms resulting from this treatment structure were fit as fixed effects, and terms corresponding to the experimental design structure were fitted as random effects. To respect repeated sampling of the same plot, extensions to the random effects and residual variance structures are documented in Table S1 for each model category.
Two embedded factorial treatment structures were used to investigate consistent treatment effects across sampling times for Fp DNA variables (Table S1). The first treatment structure included the combination of all three sampling times (November 2019, May 2020, November 2020), stubble heights and the trash treatments that were consistent across all sampling times for the trait of interest, as the embedded factorial. The second treatment structure included the combination of sampling time (May 2020, November 2020), stubble height and trash treatments that were consistent across the two sampling times for the trait of interest, as the embedded factorial. Although the composition of the fixed effects varied between the two treatment structures, the random effects fitted in the model were consistent.
Predictions of the fixed effects from each model fit were provided as empirical best linear unbiased estimates (eBLUEs), and back transformed, where necessary, to the original scale for presentation. Approximate standard errors of the back-transformed eBLUEs were obtained using a Taylor series approximation (Butler et al. 2017). Models were fitted using the ASReml-R package version 4.2 (see https://vsni.co.uk/software/asreml-r/) (Butler et al. 2017) in the R statistical computing environment (R Core Team 2019), whereby variance components were estimated using residual maximum likelihood (Patterson and Thompson 1971). A Fisher’s least significant difference test was used to compare significant fixed effects. The significance level for all testing was set at the 5% level.
Results
Taller stubble had the highest Fp colonisation after harvest
In the year following implementation of the different harvest heights, the medium and tall harvest heights led to significant (P < 0.001) additional colonisation by Fp (Fig. 2a, b). In the first 6 months after harvest, the maximum height of Fp colonisation in the tall stubble increased by 92% in Narrabri and 91% in Breeza. In medium stubble, increases of 54–77% in Narrabri and 54% in Breeza were observed. Maximum colonisation did not significantly (P < 0.001) change in the short stubble for the same period, in both experiments (Fig. 2a, b).
Maximum vertical colonisation by Fp in cereal stubble of different heights (mean observed height, in cm) at harvest of an inoculated durum crop (November 2019), following a summer fallow (May 2020) and then a chickpea break crop (November 2020) at (a) Narrabri and (b) Breeza in NSW. Note harvest heights were unique to each site owing to differences in final crop height in 2019, with slight variability in actual height achieved between and across plots for each target height treatment. Error bars represent the approximate back-transformed standard error of the mean (Breeza) or standard error of the mean (Narrabri).
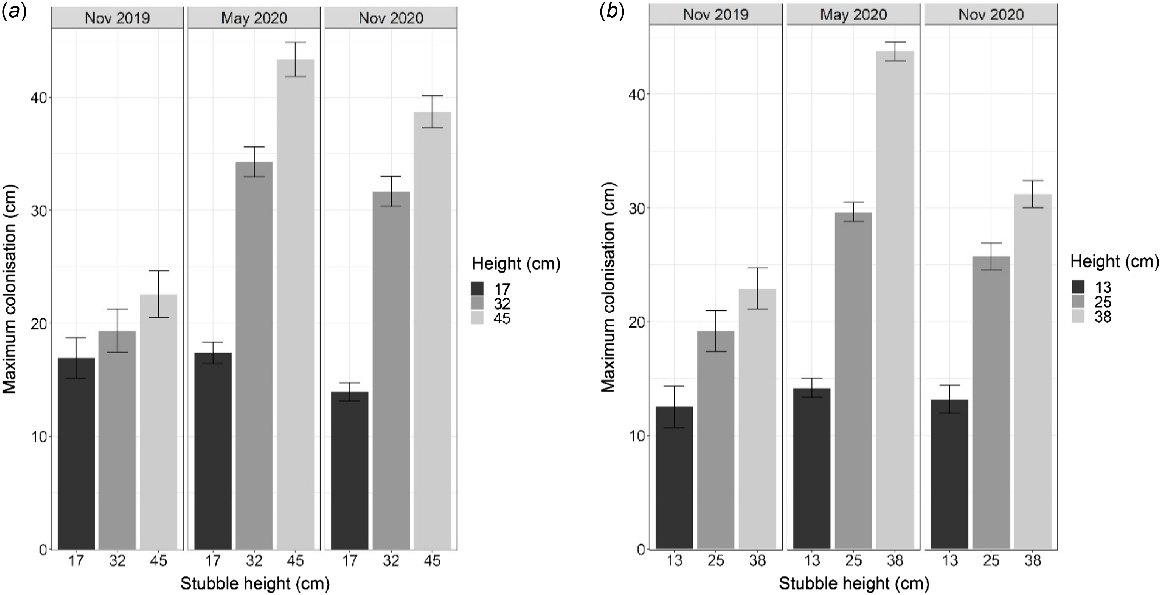
Some decline in Fp colonisation was detected in November 2020 (12 months post-harvest) for most treatments compared with levels in May 2020 (P < 0.001; Fig. 2a, b). At Narrabri, a 13–20% reduction in Fp colonisation was observed across all harvest heights (P < 0.001; Fig. 2a). At Breeza, Fp colonisation reduced by 29% in tall stubble and 13% in medium height stubble (P < 0.001; Fig. 2b). Still, after 1 year, the maximum colonisation height of Fp in both the medium and tall stubble remained significantly higher than was Fp colonisation in shorter stubble.
Fp survival was prolonged in above-ground portion of stubble
The frequency of Fp recovery changed over time at different heights, with the pathogen being best preserved at a stubble height of 10 cm (P < 0.001; Table 2). Incidence of Fp at 0 cm (the crown portion) declined steeply over the period from November 2019 to November 2020 at both experiments (P < 0.001; Table 2). However, the Fp incidence at 10 cm above the crown was stable (Narrabri) or increased (Breeza) in the first 6 months after harvest (May 2020). Incidence also increased (by approximately 30%) at 20 cm above the crown at both experiments during this time (Table 2). This was followed by a decrease in Fp incidence in the following 6 months (November 2020); however, there was still a greater incidence of Fp at 20 cm height in November 2020 than were the levels at harvest in November 2019 (Table 2).
Time | Narrabri | Breeza | |||||
---|---|---|---|---|---|---|---|
0 cm | 10 cm | 20 cm | 0 cm | 10 cm | 20 cm | ||
November 2019 | 98.5a | 69.7b | 4.5f | 44.2a | 14.2d | 1.1f | |
May 2020 | 45.1c | 68.5b | 34.1d | 36.0b | 26.2c | 29.2bc | |
November 2020 | 19.4e | 46.2c | 15.8e | 7.8e | 17.7d | 14.9de |
Heights were measured from the base of the crown towards the head end, and as such 0 cm represents the crown portion. Values followed by the same letter (l.s.d.) are not significantly different within each experiment, at the 5% confidence level.
The incidence of Fp in the crown section of the main stem was initially much lower at Breeza (44.2%) than at Narrabri (98.5%), indicating that more plants were initially infected with FCR at Narrabri in 2019, even though the same Fp inoculum and application rate was used in both field experiments (Table 2). After 12 months, Fp incidence at Narrabri was still more than twice that of Breeza at 0 and 10 cm (Table 2). Although the two experimental sites could not be compared statistically, the difference in colonisation may reflect the impact of irrigation at the Narrabri experiment during the 2019 season, because of extreme drought conditions, which facilitated greater Fp infection.
Total Fp DNA declined over time
Although the height at which Fp had colonised the durum wheat stubble increased in the 6 months after harvest, the total amount of Fp DNA declined significantly (P < 0.05) over the course of the experiments (Fig. 3a, b). At Narrabri, the shorter stubble contained the lowest concentrations of Fp DNA (pg DNA/g stubble) at harvest in November 2019 (P < 0.001; Fig. 3a). After 1 year, the Fp DNA concentrations across the different stubble height treatments were not significantly different at this experiment. However, at Breeza, the Fp DNA concentrations were similar between treatments at the initial harvest of infected durum wheat in November 2019 (P = 0.04, Fig. 3b). In the 6–12 months following harvest, Fp DNA was lowest in the short stubble (Fig. 3b), which aligned with the prevention of Fp saprotrophic vertical colonisation in short stubble treatments (Fig. 2b).
Total concentrations of Fp DNA (pg DNA/g stubble × 103) in different stubble height treatments (mean observed height, in cm) after harvest of an inoculated durum crop (November 2019), a summer fallow (May 2020) and a chickpea break crop (November 2020) at (a) Narrabri and (b) Breeza in northern NSW. Note the differences in scale for the Y-axis between locations. Error bars represent the approximate back-transformed standard error of the mean.
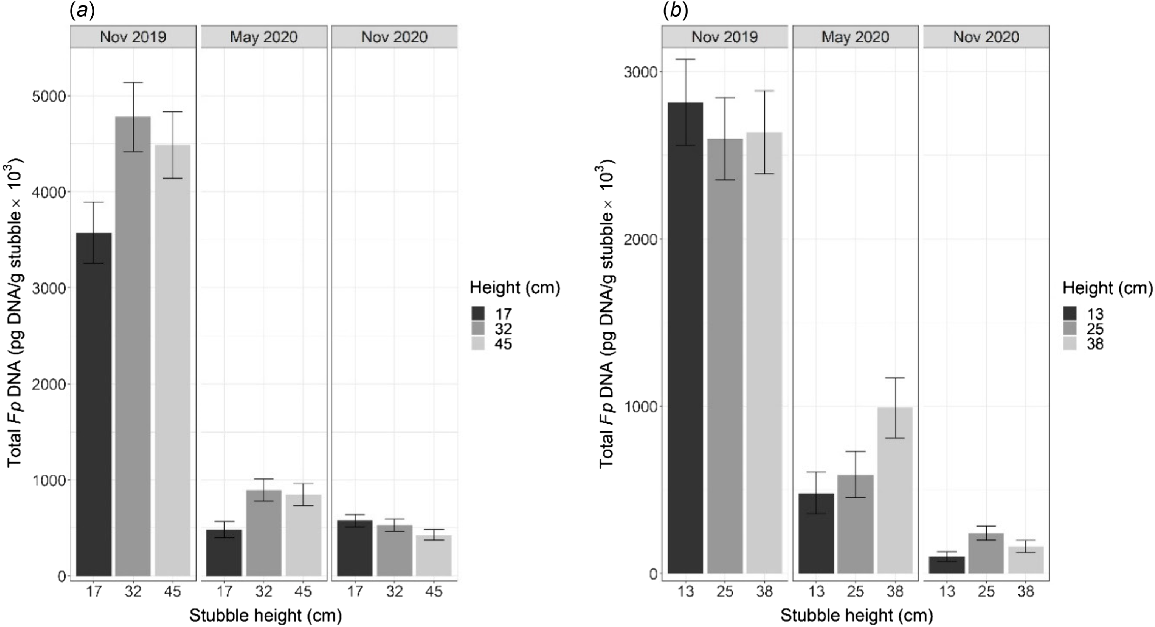
The Kelly-chain treatment resulted in lower concentrations of total Fp DNA across the duration of the experiment (Fig. 4a, b). At both experiments, Fp DNA was more than double in the standing stubble treatments compared with the Kelly-chained treatments for most treatment combinations (Narrabri, P = 0.007; Breeza, P = 0.002; Fig. S2). Importantly, however, the amount of Fp DNA present within the inter-row space (avoiding the stubble rows) was significantly higher in the Kelly-chain treatment at Narrabri (P = 0.004; Fig. 4a) and Breeza (P < 0.001; Fig. 4b). Interestingly, Fp DNA also increased over time within the inter-row spaces of the treatments with standing stubble (Fig. 4a, b; P < 0.004).
The total amount of Fp DNA (pg DNA/g of residue) within residues collected from inter-row spaces at (a) Narrabri and (b) Breeza in northern NSW. Residue in the retained treatments was maintained as standing stubble throughout the experiments, whereas residue in the Kelly-chained treatment was incorporated to a depth of approximately 3 cm in May 2020 prior to sowing chickpea. The durum wheat stubble infected with Fp was originally harvested at different heights (mean observed height, in cm) in November 2019. Results were averaged for stubble height at Breeza because there was no significant effect of stubble height at this experiment. Error bars represent the approximate back-transformed standard error of the mean.
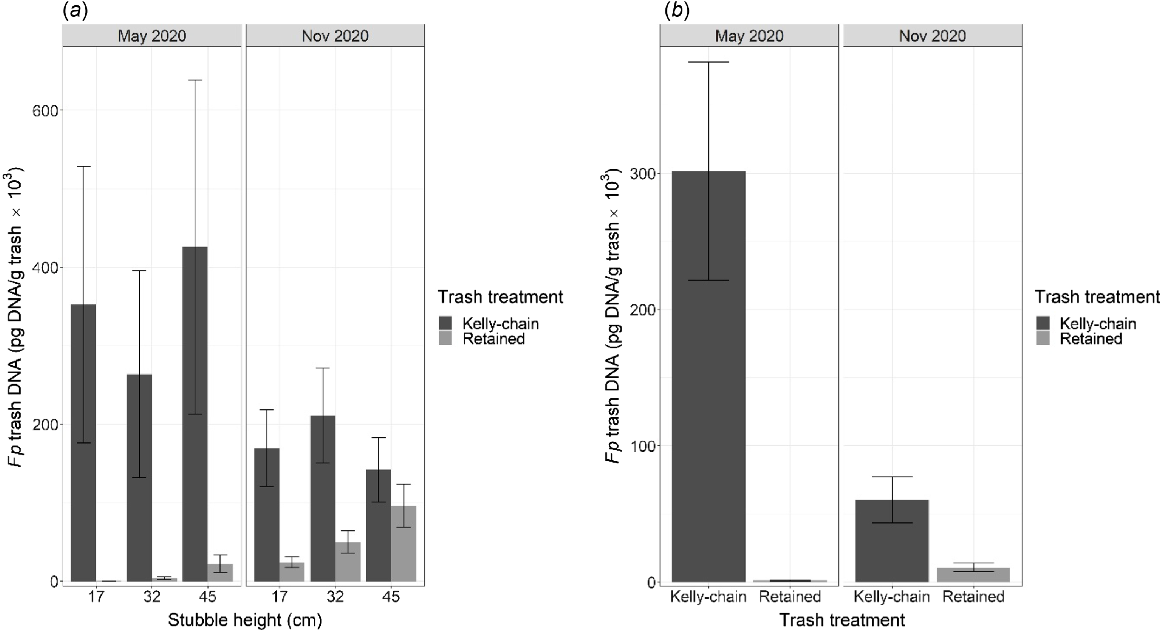
The amount of Fp DNA detected in the chickpea header trash at chickpea harvest in November 2020 can be seen in Fig. S3. Although some significant effects were observed, no trends could be ascertained in the data and DNA concentrations appeared to be highly variable between and within treatments (from 0 to 9508 pg Fp DNA/g chickpea stubble).
Pre-planting Fp soil DNA analysis was conducted in Year 3 to estimate FCR risk for the durum and bread wheat treatments to be grown that season. At Narrabri, Kelly-chain treatments (log1.61 pg DNA/g soil) had significantly higher Fp soil DNA concentration than did the trash-removed treatments (log1.29 pg DNA/g soil) (P = 0.004, data not shown). Even so, all treatments fell within the same FCR ‘high’ risk category (>1.2 log pg DNA/g soil, as defined by SARDI 2019). At Breeza, stubble management treatments did not significantly (P > 0.2) affect the Fp soil DNA. Prior to soil sampling, a large proportion of the original durum stubble from Year 1 was observed to be washed off the experimental plots at Breeza by high rainfall and surface water movement, which may have contributed to this result. However, values were still within the same ‘high’ risk category for Breeza, with a mean of log 1.38 pg DNA/g soil detected. The ‘high’ risk category reported here by SARDI (2019) is specific to soil-only samples; stubble was not added (as per commercial protocol) to avoid potential bias.
Different cereal stubble treatments did not affect soil moisture under high rainfall conditions
Overall, the stubble treatments at both sites did not appear to affect SMC, and presumably fallow efficiency. Rainfall at both sites in the summer of 2019–20 significantly (P < 0.001) increased SMC at most soil depths by May 2020 (Table 3). A significant (P < 0.001) height × trash × time interaction was observed at Narrabri, although differences were small (<1.65% SMC) and with no clear trend (data not shown). At Breeza, there were no significant (P > 0.12) effects of cereal stubble treatments on SMC. The different stubble treatments may have had a more meaningful impact on fallow efficiency and SMC if the drier conditions experienced in 2019 had persisted into 2020–21. From 2021, further SMC measurements could not be taken because of waterlogging at both sites.
Time | Narrabri SMC (%) | Breeza SMC (%) | |||||||
---|---|---|---|---|---|---|---|---|---|
0–30 cm | 30–60 cm | 60–90 cm | 90–120 cm | 0–30 cm | 30–60 cm | 60–90 cm | 90–120 cm | ||
November 2019 | 14.1f | 17.0e | 17.0e | 17.0e | 25.5e | 23.7f | 24.0f | 24.0f | |
May 2020 | 23.8a | 23.5a | 22.1b | 18.8cd | 34.3a | 34.3a | 31.0bcd | 23.8f | |
November 2020 | 23.4a | 18.8c | 18.2d | 19.2c | 29.8d | 32.0b | 32.1b | 31.0c |
Values followed by the same letter (l.s.d.) are not significantly different within each experiment, at the 5% confidence level.
Chickpea crop performance
Overall, the cereal stubble treatments did not appear to have many meaningful effects on chickpea performance in Year 2. At Breeza, the Kelly-chained treatment resulted in a slightly higher chickpea establishment (32 plants/m2) than did the trash-retained treatment (28 plants/m2) (P = 0.05, data not shown). This was possibly due to better seed–soil contact at sowing when using the disk seeder. Pod height was similarly unaffected by cereal stubble treatments at either site (P > 0.32, data not shown). There was no significant effect of standing stubble height (P > 0.96), trash treatment (P > 0.19) or the interaction of harvest height and trash treatments (P > 0.14) applied in Year 1 (November 2019) on chickpea yield at both sites in Year 2 (November 2020). Yield averaged 2.19 Mg/ha at Narrabri and 1.19 Mg/ha at Breeza. Cereal stubble height, therefore, had little effect on chickpea production at these sites.
Final wheat crop performance: yield and grain quality
Like the chickpea results, the stubble height and trash treatments implemented to the Year 1 durum wheat stubble did not appear to meaningfully affect wheat crop performance in Year 3. Variety was the most consistent driver of differences in yield and grain quality, with DBA Lillaroi outperforming LRPB Hellfire at both experiments (Table 4), despite DNA results suggesting that these experiments were high risk for FCR in the 2021 season. Although some additional significant effects were observed for the Narrabri experiment, such as a main effect of trash treatment on grain protein, differences between treatments were small (e.g. <0.24% for grain protein).
Trait | Narrabri | Breeza | |||
---|---|---|---|---|---|
Significant terms | P-value | Significant terms | P-value | ||
Yield | Height × trash × variety interaction | 0.02 | Variety main effect | <0.001 | |
Screenings | Variety main effect | <0.001 | Variety main effect | <0.001 | |
Grain protein | Variety main effect Trash main effect | <0.01 0.02 | Height × trash × variety interaction | 0.04 | |
1000-grain weight | Variety main effect | <0.001 | Variety main effect | <0.001 | |
CRI | Variety main effect | <0.001 | No significant terms | NA | |
Fp incidence | No significant terms | NA | Height × trash × variety interaction | 0.05 |
The significance of treatment effects was reported for each trait, where treatments are defined as harvest height (‘height’: low, medium, and high), header trash (‘trash’: trash-retained, trash-removed and Kelly-chain) and variety (‘variety’: DBA Lillaroi and LRPB Hellfire).
At Narrabri, DBA Lillaroi also had lower screenings and a higher 1000-grain weight (2.2% and 44.1 g respectively) than did LRPB Hellfire (7.2% and 32.1 g respectively) (P < 0.001). Grain protein showed significant main effects for variety (P < 0.001) and trash treatment (Table 4, P = 0.02), with DBA Lillaroi having higher protein content (13.2%) than LRPB Hellfire (13.0%). Differences in grain protein were very small, between trash-retained (13.2%) and trash-removed (12.9%) treatments, whereas the Kelly-chain (13.0%) treatment was not significantly different from either of the other trash treatments.
At Breeza, DBA Lillaroi yielded 3.74 Mg/ha, which was 0.3 Mg/ha higher than LRPB Hellfire (Table 4, P < 0.001). DBA Lillaroi also had a higher 1000-grain weight (42.6 g) than did LRPB Hellfire (35.3 g) and lower screenings (1.4%) than did LRPB Hellfire (4.5%) (P < 0.001). Although a significant height × trash × variety interaction was observed for grain protein (P = 0.04), the most notable trend in the data was lower protein in Lillaroi (14.0–14.9%) than in LRPB Hellfire (14.6–15.3%) (data not shown).
Final wheat crop performance: FCR infection and CRI
The Fp incidence and severity (CRI) in Year 3 was less affected by the individual stubble management treatments and more influenced by inoculum persisting from Year 1 across all treatments (Table 4). At Narrabri, a mean Fp incidence of 27% was observed across the experiment, with no significant treatment differences detected (P ≥ 0.07 for all terms). However, CRI was significantly (P < 0.001) higher in DBA Lillaroi (30.3%) than in LRPB Hellfire (18.3%), reflecting the increased relative susceptibility of durum wheat to FCR under similar disease pressure (S-VS rating for DBA Lillaroi versus the MS-S rating for LRPB Hellfire) (Matthews et al. 2024). There were no significant effects of any treatment on CRI at Breeza (mean 21.6%, P ≥ 0.17 for all terms). There was a significant height × trash × variety effect for Fp incidence; however, no trends were obvious from reviewing the results (P = 0.05, Fig. S4). It is possible that the colder and wetter conditions at Breeza during Year 3 (2021) were not as conducive to FCR expression (despite higher Fp incidence on average). Nonetheless, the presence of FCR at moderate-to-high levels in both cereal crop types following a chickpea break crop, and two unusually wet summer fallows, exemplifies the persistence of Fp inoculum in the NGR.
Discussion
This study has made an important link between cereal harvest height and the potential for Fp inoculum accumulation of post-harvest stubble, which has not been previously identified. Within 6 months after harvest, a 61–70% increase in the height of Fp saprotrophic colonisation was observed in taller stubble (38–45 cm) compared with short stubble (13–17 cm) at two different field locations. Our findings add to the existing evidence that Fp can saprotrophically colonise cereal stubble in controlled environments (Melloy et al. 2010; Percy et al. 2012; Petronaitis et al. 2022) and in field stubble that has been flattened (Summerell and Burgess 1988). In our study, Fp persisted higher within taller and conventional (medium) height stubble for a full 12 months after harvest (potentially longer if sampling was possible beyond Year 2). Our technique of cutting cereal stubble short therefore limited the stubble available for Fp colonisation, supporting the hypothesis that short harvest heights can limit saprotrophic colonisation of cereal stubble by Fp. In practice, growers could similarly choose to limit inoculum accumulation in their cereal stubble by using this simple technique.
A major aim of this research was to test whether taller cereal stubble increases FCR risk, by facilitating additional stubble biomass that could allow Fp to proliferate. If true, the widespread use of stripper front headers has the potential to further increase FCR. We observed both significant increases in inoculum (via Fp saprotrophic colonisation) in medium and tall stubble, as well as higher Fp DNA concentrations in taller stubble at times (via qPCR). Interestingly, this did not translate to an increase in the overall FCR risk or disease expression in Year 3. However, FCR risk was also unmitigated by light tillage (and increased stubble–soil contact), rotation through a non-host break crop, or unusually wet seasonal conditions, all of which are known to facilitate decomposition of cereal stubble and displacement of Fp (Wildermuth et al. 1997; Swan et al. 2000). These results speak to the overall persistence of Fp in the NGR, where Fp has been detected in 100% of cereal crops in random crop surveys (n = 264, with majority in the ‘high’ pathogen load category) (Milgate and Simpfendorfer 2020). It is perhaps unsurprising, then, that no differences in stubble height treatments on FCR risk were observed in the present study. It is also possible that the Fp inoculation in Year 1 of the experiments was ‘too successful’, increasing FCR risk so significantly that any differences implemented by the harvest height treatments were undetectable in the following seasons. Given the extent of post-harvest colonisation and persistence of Fp inoculum identified in this study, further investigation of the effects of Fp saprotrophic colonisation on FCR risks are warranted. Longer-term farming system experiments would be required to define whether harvest height modification is effective for FCR management across locations and seasons.
A combination of both traditional culturing methods and qPCR were used to capture the growth and survival of Fp within stubble in this study. In the past, research and diagnostic protocols have used culturing of either crown tissue (2 cm or or lower) (Nelson and Burgess 1994; Swan et al. 2000) or qPCR (Hogg et al. 2010; Liu et al. 2012; Sabburg et al. 2015; Knight and Sutherland 2016; Knight et al. 2021) to assess Fp in stubble. Our research suggested that these methods used in isolation may underestimate the persistence of Fp, particularly in aboveground stubble. We found that Fp decreased rapidly in the crown, surviving best longer term (6–12 months post-harvest) at 10–20 cm above the crown. This may be a ‘sweet spot’ for Fp survival, because competition with other saprotrophs would be limited compared with stubble in contact with the soil (Summerell and Burgess 1988; Bockus 1998; Yi et al. 2002; Pereyra et al. 2004). Displacement from crown tissue also resulted in an 87% reduction in total Fp DNA in the first 6 months after harvest. This made sense, given that vascular bundles of the crown tissue are more densely colonised during aggressive pathogenic colonisation (Knight et al. 2017); so, saprotrophic colonisation of stubble may not contribute equally to fungal load. Subsequently, the increases in saprotrophic colonisation in medium and tall stubble were not successfully ‘captured’ by qPCR because the decrease in Fp DNA in the crown was much larger in magnitude. Culturing, particularly of above-ground stubble parts (10–20 cm), may therefore be a better long-term indicator of the persistence of Fp in post-harvest cereal stubble.
Another aim of this study was to investigate whether Kelly-chaining (a popular tool among chickpea growers in the NGR) had any effects on FCR in a cereal–chickpea–cereal rotation. The Kelly-chain treatment resulted in the lowest levels of total Fp DNA across the duration of the experiment (when stubble was collected off the surface of the plots). This could reflect more rapid decomposition and subsequent displacement of Fp when stubble is incorporated into the soil surface (Summerell and Burgess 1988). However, in the inter-row spaces the amount of Fp DNA was significantly higher for Kelly-chained treatments, which could be problematic when using inter-row sowing strategies for Fp inoculum avoidance (Simpfendorfer et al. 2019). Surprisingly, Fp DNA also increased in row spaces in the standing stubble treatments over the course of the experiment. Some standing tillers may have fallen over as crown tissue rotted out, causing more inoculum to enter row spaces. This could be an important consideration when using inter-row sowing as a main strategy to mitigate FCR and reinforces the importance of determining pre-planting inoculum to assess FCR risk (Simpfendorfer et al. 2019).
Infection by Fp and disease severity in Year 3 appeared to be most influenced by inoculum persisting from Year 1, as well as differences in cultivar susceptibility. The durum wheat (DBA Lillaroi) performed better (higher yield and 1000-grain weights, and lower screenings) than did the moderately susceptible bread wheat (LRPB Hellfire) at both experiments. Although the symptoms of FCR (such as stem browning) can be more severe in more susceptible cereal crops such as durum wheat (Hollaway et al. 2013), durum wheat can also have a better tolerance to FCR than do some bread wheat varieties under increasing inoculum levels (Forknall et al. 2019). This means that decline in crop performance is not as steep in durum as it is in some bread wheats under the same disease pressure. Although these factors explain the result seen in our experiment, it is important to note that durum wheat is extremely susceptible to Fp (Simpfendorfer et al. 2019), as seen by higher CRI in DBA Lillaroi at the Narrabri experiment. Undoubtedly, the high in season rainfall and stored soil moisture during the present study would also have reduced FCR expression (Alahmad et al. 2018).
One potential benefit of taller cereal stubble is the possibility of improved soil moisture storage (Francis 2018). Interestingly, in both the present study and previous work by Broster et al. (2020), soil moisture was not meaningfully increased by taller cereal stubble treatments (up to 60 cm height). Wet fallows and mild temperatures may have influenced our study by providing additional moisture across all treatments. However, the experiment by Broster et al. (2020) was run during the 2019 drought where increased stubble biomass was more likely to have significant effects on SMC. We also found that Kelly-chaining had no meaningful effect on SMC, even though it is a form of shallow tillage that may be associated with lower soil moisture storage across seasons (Alahmad et al. 2018). However, with the high rainfall experienced in Years 2 and 3, any form of retained residue is likely to have provided sufficient coverage for SMC preservation.
The high rainfall conditions experienced from Year 2 onward also likely to have promoted the saprotrophic colonisation of stubble seen in our study. Similar findings have been demonstrated in laboratory studies using different types of cereal stubble, whereby more extensive colonisation of stubble by Fp was observed under high humidity conditions (Petronaitis et al. 2022). In the field, Summerell and Burgess (1988) observed Fp increasing in cereal stubble following wet winter fallow conditions (with stubble laying on or lightly incorporated to 10 cm soil depth). Similar stubble management treatments in drier-than-average conditions resulted in no saprotrophic colonisation of cereal stubble by Fp (Summerell et al. 1989). Saprotrophic colonisation may therefore be more frequently observed following persistent high humidity or rainfall conditions and may not occur every season. A long-term survey over a large geographical scale would be necessary to model the effects of weather on saprotrophic colonisation.
Leaving cereal stubble tall in fields infected with Fp, for example, when using stripper front headers, could result in extensive saprotrophic colonisation after harvest. Hypothetically, this could increase disease risk in subsequent cereal crops, although we did not observe this in the present study (likely owing to seasonal conditions). More research is required across different cropping systems and seasons to define the risks posed by stripper front headers and/or higher cereal stubble loads. Cutting stubble shorter at harvest may therefore be a useful strategy to restrict saprotrophic colonisation of Fp in post-harvest cereal stubble but should not replace existing integrated disease management strategies for FCR.
Data availability
The data used to generate the results in the paper are held by Research UNE (rUNE) and may be accessed via https://doi.org/10.25952/a6wd-h734.
Declaration of funding
This research was funded by the Grains Agronomy and Pathology Partnership (GAPP), a bilateral agreement between the Grains Research and Development Corporation and New South Wales Department of Primary Industries (Code BLG211).
Acknowledgements
This research was made possible by the significant contributions of growers through their support of the Grains Research and Development Corporation (GRDC). Dr Petronaitis thanks the GRDC and New South Wales Department of Primary Industries for co-funding her Grains Agronomy and Pathology Partnership PhD scholarship. Thanks go to Dr Rick Graham, Dr Kristy Hobson and Dr Gururaj Kadkol for providing seed for the experiments. Thanks also go to Kerry Bell for providing valuable feedback on the statistical components of this paper. Technical assistance provided by Chrystal Fensbo, Finn Fensbo, Alana Johnson, Jason McCulloch, Barbara Jones, Stephen Morphett, Jim Perfrement, Michael Dal Santo, and Luke Neale is gratefully acknowledged.
References
Akinsanmi OA, Mitter V, Simpfendorfer S, Backhouse D, Chakraborty S (2004) Identity and pathogenicity of Fusarium spp. isolated from wheat fields in Queensland and northern New South Wales. Australian Journal of Agricultural Research 55, 97-107.
| Crossref | Google Scholar |
Alahmad S, Simpfendorfer S, Bentley AR, Hickey LT (2018) Crown rot of wheat in Australia: Fusarium pseudograminearum taxonomy, population biology and disease management. Australasian Plant Pathology 47(3), 285-299.
| Crossref | Google Scholar |
Bockus WW (1998) Control strategies for stubble-borne pathogens of wheat. Canadian Journal of Plant Pathology 20, 371-375.
| Crossref | Google Scholar |
Broster J, Hatty N, Eberbach P, Walsh M (2020) Influence of header front on stubble micro-climate and winter crop growth. GRDC Update Paper. Available at https://grdc.com.au/resources-and-publications/grdc-update-papers/tab-content/grdc-update-papers/2020/02/influence-of-header-front-on-stubble-micro-climate-and-winter-crop-growth [accessed 9 March 2021]
Forknall CR, Simpfendorfer S, Kelly AM (2019) Using yield response curves to measure variation in the tolerance and resistance of wheat cultivars to Fusarium crown rot. Phytopathology 109, 932-941.
| Crossref | Google Scholar | PubMed |
Francis J (2018) The economics of changing from draper to stripper fronts for increased standing crop residue and improved harvest efficiency. GRDC Update article. Available at https://grdc.com.au/resources-and-publications/grdc-update-papers/tab-content/grdc-update-papers/2018/08/the-economics-of-changing-from-draper-to-stripper-fronts [accessed 14 January 2019]
Hogg AC, Johnston RH, Johnston JA, Klouser L, Kephart KD, Dyer AT (2010) Monitoring fusarium crown rot populations in spring wheat residues using quantitative real-time polymerase chain reaction. Phytopathology 100, 49-57.
| Crossref | Google Scholar | PubMed |
Hollaway GJ, Evans ML, Wallwork H, Dyson CB, McKay AC (2013) Yield loss in cereals, caused by Fusarium culmorum and F. pseudograminearum, is related to fungal DNA in soil prior to planting, rainfall, and cereal type. Plant Disease 97, 977-982.
| Crossref | Google Scholar | PubMed |
Kelly Tillage (2022) Tillage: make stubble management simple. Available at https://kellytillage.com/ [accessed 7 July 2022]
Knight NL, Sutherland MW (2016) Histopathological assessment of Fusarium pseudograminearum colonization of cereal culms during crown rot infections. Plant Disease 100, 252-259.
| Crossref | Google Scholar | PubMed |
Knight NL, Macdonald B, Sutherland MW (2017) Colonization of durum wheat (Triticum turgidum L. var. durum) culms exhibiting premature senescence (dead heads) associated with Fusarium pseudograminearum crown rot. Plant Disease 101, 1788-1794.
| Crossref | Google Scholar | PubMed |
Knight NL, Macdonald B, Percy C, Sutherland MW (2021) Disease responses of hexaploid spring wheat (Triticum aestivum) culms exhibiting premature senescence (dead heads) associated with Fusarium pseudograminearum crown rot. European Journal of Plant Pathology 159, 191-202.
| Crossref | Google Scholar |
Liu Y, Ma J, Yan W, Yan G, Zhou M, Wei Y, Zheng Y, Liu C (2012) Different tolerance in bread wheat, durum wheat and barley to Fusarium crown rot disease caused by Fusarium pseudograminearum. Phytopathology 160, 412-417.
| Crossref | Google Scholar |
Matthews P, McCaffery D, Jenkins L (2024) Winter crop variety sowing guide 2024. NSW Department of Primary Industries. Available at https://www.dpi.nsw.gov.au/agriculture/broadacre-crops/guides/publications/winter-crop-variety-sowing-guidehttps://www.dpi.nsw.gov.au/agriculture/broadacre-crops/guides/publications/nsw-winter-crop-variety-sowing-guide [accessed 27 March 2025]
Melloy P, Hollaway G, Luck J, Norton R, Aitken E, Chakraborty S (2010) Production and fitness of Fusarium pseudograminearum inoculum at elevated carbon dioxide in FACE. Global Change Biology 16, 3363-3373.
| Crossref | Google Scholar |
Milgate A, Simpfendorfer S (2020) Pathogen burden in NSW winter cereal cropping. GRDC Update Paper. Available at https://grdc.com.au/resources-and-publications/grdc-update-papers/tab-content/grdc-update-papers/2020/08/pathogen-burden-in-nsw-winter-cereal-cropping [accessed 5 April 2022]
Murray GM, Brennan JP (2009) Estimating disease losses to the Australian wheat industry. Australasian Plant Pathology 38,, 558-570.
| Crossref | Google Scholar |
Nelson KE, Burgess LW (1994) Reaction of Australian cultivars of oats and barley to infection by Fusarium graminearum Group 1. Australian Journal of Experimental Agriculture 34, 655-658.
| Crossref | Google Scholar |
Olita T, Cao Z, Gibberd M (2024) Economic analysis of crop protection strategies: comparing the value of increased fungicide inputs and crop genetic improvement in managing Ascochyta blight in Australian chickpeas. Pest Management Science 80(11), 5887-5897.
| Crossref | Google Scholar | PubMed |
Ophel-Keller K, McKay A, Hartley D, Herdina, Curran J (2008) Development of a routine DNA-based testing service for soilborne diseases in Australia. Australasian Plant Pathology 37, 243-253.
| Crossref | Google Scholar |
Patterson HD, Thompson R (1971) Recovery of inter-block information when block sizes are unequal. Biometrika 58, 545-554.
| Crossref | Google Scholar |
Percy CD, Wildermuth GB, Sutherland MW (2012) Symptom development proceeds at different rates in susceptible and partially resistant cereal seedlings infected with Fusarium pseudograminearum. Australasian Plant Pathology 41, 621-631.
| Crossref | Google Scholar |
Pereyra SA, Dill-Macky R, Sims AL (2004) Survival and inoculum production of Gibberella zeae in wheat residue. Plant Disease 88, 724-730.
| Crossref | Google Scholar | PubMed |
Petronaitis T, Forknall C, Simpfendorfer S, Backhouse D, Flavel R (2022) Stubble trouble! Moisture, pathogen fitness and cereal type drive colonisation of cereal stubble by three fungal pathogens. Australasian Plant Pathology 51, 363-368.
| Crossref | Google Scholar |
Sabburg R, Obanor F, Aitken E, Chakraborty S (2015) Changing fitness of a necrotrophic plant pathogen under increasing temperature. Global Change Biology 21, 3126-3137.
| Crossref | Google Scholar | PubMed |
SARDI (2019) Crown rot. In ‘Broadacre Soilborne Disease Manual, version 11.0.’ (Ed. B Cay). Available at https://rootdisease.aweb.net.au/ [accessed 29 June 2022]
Simpfendorfer S, McKay A, Ophel-Keller K (2019) New approaches to crop disease management in conservation agriculture. In ‘Australian Agriculture in 2020: from Conservation to Automation’. (Eds J Pratley, J Kirkegaard) pp. 173–188. (Agronomy Australia and Charles Sturt University: Wagga Wagga, NSW, Australia)
Singh U, Gaur PM, Chaturvedi SK, Hazra KK, Singh G (2019) Changing plant architecture and density can increase chickpea productivity and facilitate for mechanical harvesting. International Journal of Plant Production 13, 193-202.
| Crossref | Google Scholar |
Summerell BA, Burgess LW (1988) Stubble management practices and the survival of Fusarium graminearum Group 1 in wheat stubble residues. Australasian Plant Pathology 17, 88-93.
| Crossref | Google Scholar |
Summerell BA, Burgess LW, Klein TA (1989) The impact of stubble management on the incidence of crown rot of wheat. Australian Journal of Experimental Agriculture 29, 91-98.
| Crossref | Google Scholar |
Swan LJ, Backhouse D, Burgess LW (2000) Surface soil moisture and stubble management practice effects on the progress of infection of wheat by Fusarium pseudograminearum. Australian Journal of Experimental Agriculture 40, 693-698.
| Crossref | Google Scholar |
Tado CJM, Wacker P, Kutzbach HD, Suministrado DC (1998) Development of stripper harvesters: a review. Journal of Agricultural Engineering Research 71, 103-112.
| Crossref | Google Scholar |
Verrell AG, Simpfendorfer S, Moore KJ (2017) Effect of row placement, stubble management and ground engaging tool on crown rot and grain yield in a no-till continuous wheat sequence. Soil and Tillage Research 165, 16-22.
| Crossref | Google Scholar |
Wildermuth GB, Thomas GA, Radford BJ, McNamara RB, Kelly A (1997) Crown rot and common root rot in wheat grown under different tillage and stubble treatments in southern Queensland. Soil and Tillage Research 44, 211-224.
| Crossref | Google Scholar |
Wilkinson G, Rogers C (1973) Symbolic description of factorial models for analysis of variance. Journal of the Royal Statistical Society, Series C (Applied Statistics) 22, 392-399.
| Crossref | Google Scholar |
Yi C, Kaul HP, Kübler E, Aufhammer W (2002) Populations of Fusarium graminearum on crop residues as affected by incorporation depth, nitrogen and fungicide application. Journal of Plant Diseases and Protection 109, 252-263.
| Google Scholar |