Liming acidic soils creates profits, land use options but often more emissions
S. Kharel A , C. d’Abbadie A , A. Abadi Ghadim A , C. Gazey A and R. Kingwell
A
B
Abstract
Soil acidity constrains crop production in Australia. The practice of liming can reduce soil acidity but produces greenhouse gas emissions.
By examining land use sequences over three decades at a range of locations in Western Australia, this study aims to identify firstly where and when liming might boost farm profits and secondly, what emissions and land use management flexibilities are generated by liming.
Bioeconomic simulation modelling is used to identify the gross margins and emissions associated with liming in land use sequences at 14 locations in Western Australia. Three intensities of cropping and three different rotational sequences are considered. The simulations account for price and weather–year variations across a 30-year period of analysis.
Liming is profitable at almost all locations and across all rotation sequences examined. Where problematic soil acidity is a feature or is poised to soon become a problem at a location, liming is a profitable ameliorative practice that enables greater diversity in land use. For most situations assessed, liming increases emissions. The exceptions are at locations where liming prevents a switch away from a crop-dominant system, due to soil acidity reducing crop yields, into additional sheep production that increases emissions.
Liming is profitable in most acidic soil situations and preserves land use flexibility, although additional greenhouse gas emissions are often generated.
Liming acidic soils bolsters land use profitability and helps sustain biologically diverse land use sequences, despite often increasing greenhouse gas emissions.
Keywords: biological diversity, crop sequences, dryland cropping, greenhouse gas emissions, lime, net margins, soil acidity, soil amelioration.
Introduction
Soil acidity is known to deleteriously affect plant growth in many parts of the world (Baligar et al. 1997; Sumner and Noble 2003; Caires et al. 2008). At low soil pH many elements become less available to plants, and others such as iron, aluminium and manganese become toxic to plants. Aluminium, iron and phosphorus also combine to form insoluble compounds (Robson 1989; Rengel 2003). The pH range 5–6 is considered ideal for most plants.
Technically an acidic soil is one with a soil pH (CaCl2) below 4.8 in the root zone (0–20 cm) (Department of Primary Industries 2024). Soil acidity has multifaceted impacts on crop health, with reduced crop yields being the primary concern (Kunhikrishnan et al. 2016). An acidic soil environment hinders nitrogen fixation in legume crops by subduing the rhizobia inoculation (Thilakarathna and Raizada 2017). Additionally, acidity limits the availability of other essential nutrients which further restricts plant growth and productivity. Often the most damaging effect of soil acidity occurs in the subsurface layers, where increased aluminium toxicity can limit root growth. Restricted root growth and limited access to water and nutrients due to aluminium toxicity become significant limitations to crop production (Davies et al. 2006; Gazey et al. 2013; Anderson and Bell 2019; Li et al. 2019).
In Australia, as in some other global regions, soil acidity is a major constraint to crop production. Acidity of agricultural soils in Australia is estimated to cost Australian farmers about AUD 1.6 billion a year (in constant 2005-dollar terms) (Hajkowicz and Young 2005). In Australia’s southwest, soil acidity is a widespread problem, yet the region is a main source of Australia’s grain exports. The region supplied over 51% of Australia’s wheat exports between 2016 and 2022 and when drought affected grain production in eastern Australia in 2019, the southwest of Australia supplied 80% of the nation’s wheat exports.
Subsurface acidity affects approximately one third, or 5.3 million hectares of soils in this region (Davies et al. 2006). Since being cleared for agriculture, the region’s soils have become more acidic and subsurface acidity is now a common soil problem in this region, as well as in several other countries (Rengel 2003; FAO and ITPS 2015). Deep sands, sandy earths, gravels, and duplex soils with low levels of organic carbon and clay, as well as a reduced ability to buffer pH, are among the soil types susceptible to acidification in the region.
In Australia’s southwest, acid soils are at risk of becoming more acidic through nitrogen fixation by legumes and via on-going use of nitrogenous fertilisers (Schroder et al. 2011; Kalkhoran et al. 2020; Zhu et al. 2024). Soil acidity poses a significant threat to agricultural productivity in the region (Dolling et al. 1991, 1994; Brennan et al. 2004; Anderson and Bell 2019).
A remedy for soil acidification being adopted in Australia as in many other global regions is the application of lime (Chien et al. 2010; Gazey et al. 2013; Wortman et al. 2015; Kalkhoran et al. 2020). Applying lime to counteract soil acidity, worsened by product removal and fertiliser applications, can improve crop and pasture yields (Anderson et al. 2005; Gazey et al. 2013; Azam and Gazey 2021). These yield increases are attributable to liming causing an increase in the availability of essential plant nutrients (Zhao et al. 2018; Pang et al. 2019; Mkhonza et al. 2020).
Liming can ameliorate subsurface acidification, but it may take more than 5 years to elevate the soil’s pH below 10 cm (Davies et al. 2006). Attempts to quickly remediate subsurface acidity with direct insertion of lime beneath deep ripping tines have delivered mixed results (Davies et al. 2019). Hence, surface liming is the most common management strategy for preventing further acidification of the subsurface in the south-west agricultural region of Australia (Davies et al. 2006).
Although liming is widely recommended to combat soil acidification, farmers face several considerations when making lime application decisions. Crop rotation practices and the type of fertiliser used significantly influence the optimal amount of lime required. Additionally, the cost of applying lime is an important consideration for farmers whose farm profitability is additionally exposed to climate and commodity price volatility (Whitten et al. 2000; Edmeades and Ridley 2003; Li et al. 2019; Kingwell et al. 2020; Azam and Gazey 2021; Dempster et al. 2021).
The relationship between lime application and nitrogenous fertiliser use presents a complex challenge (Kalkhoran et al. 2020). Ammonium-based fertilisers, commonly used in agriculture, exacerbate soil acidity. Their use necessitates adjustments in lime application strategies to counteract their acidifying effect. Yet nitrogenous fertilisers facilitate land uses dominated by profitable cereals and oilseeds. Alternatively, integrating legumes into crop rotations enables the fixing of nitrogen from the atmosphere, thereby reducing reliance on nitrogenous fertilisers, and consequently lowers overall greenhouse gas emissions from the production and use of these fertilisers (Coventry et al. 2003; Kalkhoran et al. 2019; Sevenster et al. 2022). However, dependence on legumes to lessen emissions that might otherwise arise from dependence on nitrogenous fertilisers alters rotation gross margins.
The complexities surrounding liming decisions have led several researchers to adopt modelling approaches to investigate and facilitate choice of the rate of lime applied. Hochman et al. (1989), Verburg et al. (2003) and Kalkhoran et al. (2020) are all examples of models developed to examine liming strategies. These models typically simulate the effect of rate of lime applied to a soil of some initial level of acidity and then determine the most profitable rate to apply over some period of years (e.g. 10 years) for some assumed land use. However, apart from the study by Kalkhoran et al. (2019), these models exclude consideration of greenhouse gas emissions associated with land uses and lime applications. The Kalkhoran et al. (2019) study however has other limitations. It only considers three locations and assumes lime is only applied in the first year and then every 10 years as subsequent fixed maintenance applications. No pasture phases are considered, and prices of all crops and urea are fixed. Only emissions associated with the production, transport and use of lime and nitrogenous fertilisers are considered.
To provide a more fulsome analysis of land use at more locations, encompassing a wider range of rotations with greater flexibility in the timing and rates of liming, and covering a greater range in the sources of agricultural emissions (described later in the methodology), this study employs bio-economic simulation modelling. Lime and nitrogen fertiliser application strategies are examined in a range of land use sequences across a 30-year period at 14 locations in the agricultural region of Australia’s southwest, where acid soils or soils prone to acidity exist. The use of bio-economic simulation modelling enables trade-offs between economic outcomes and greenhouse gas emissions to be examined. The modelling incorporates regional variations in rainfall, a key factor influencing the effectiveness of lime application and the leaching of nitrogen. Liu et al. (2004) recommend that models of liming should consider rainfall and soil water content as they found these factors influenced the rate and extent of dissolution of limestone and subsequent plant response in some Australian semi-arid environments.
In this current study, management strategies that include liming practices are contrasted against those that exclude liming. Economically attractive land use sequences and associated liming practices are identified, along with their greenhouse gas emissions. We examine under what specific conditions (e.g. agroecological zones, climate, crop rotations) is the application of lime most profitable, with the modelling considering the impacts of seasonal variation in rainfall and commodity prices.
Methodology
This study compares the gross margins and greenhouse gas emissions related to the incorporation of lime in three rotational sequences at a range of locations in south western Australia, using a bio-economic simulation model that accounts for price and weather–year variation. Assessing these variations across a 30-year study period helps generate robust findings.
Below is a description of the research region and its agricultural outputs.
Study region
The study region (pale yellow to orange shades in Fig. 1) in Australia’s southwest is dominated by a Mediterranean-type climate, which supports annual winter cropping and pasture production. The average growing season rainfall in the study region ranges from 450 mm near the region’s wetter margins down to only 175 mm on the drier inland margins. The size of farms in the study region is negatively correlated with growing season rainfall. The northern and eastern parts of the study region are dominated by large crop-dominant farms whereas smaller mixed crop and sheep farms are common in the southern parts of the study region.
Fourteen different locations (see Fig. 1) across the study region were selected to represent different rainfall and crop-growing environments. The study region supplies around half of Australia’s wheat exports (DAFF 2023), equivalent to almost 4% of global wheat exports (Observatory of Economic Complexity 2024). In 2022 the study region produced 26 million tonnes of grain (GIWA 2023) but in 2023, a year of very low rainfall, grain production was 15 million tonnes (GIWA 2024). The region also supported 12 million sheep in 2021 (DPIRD 2021) but the sheep population is trending downwards in response to the intended closure of the live sheep export trade (MLA and AWI 2024).
Modelling
The Economic Valuation of Alternative Land Use Sequence (EVALUS) (Kharel 2022; Kharel et al. 2022) model was used to study the impact of liming on land use sequence profitability and emissions. At each of the 14 locations, for a representative farmer’s field, its land use sequences, profits and emissions were simulated for 30 years. The model tracks fertiliser uses and soil indicators, including soil acidity, over the specified period. The Optlime model (Gazey 2008), later known as iLime (Fisher et al. 2019; DPIRD 2019), was incorporated into EVALUS to study the relationships between fertiliser applications, acidification, and liming practices.
The EVALUS model calculates the fertiliser demand based on a yield target, as explained in d’Abbadie et al. (2024a). Based on the fertiliser input and crop yield, the Optlime model within EVALUS calculates the acidification of the soil, based on factors like soil type and soil carbon. Then the available aluminium at each pH level is calculated and yield is penalised based on the crop’s tolerance to aluminium. When lime is added, based on different strategies, it reacts with hydrogen ions in the soil to neutralise the soil and increase soil pH over time. The Optlime model also simulates the movement of pH through the soil profile depending on rainfall and the neutralisation rate of the surface soil.
The lime used in the model is Generic West Cost Limesand as defined in the iLime app (DPIRD 2019) where the net weight of CaCO3 per tonne of lime is 89.3% and the surface area is 21,837 m2 per tonne for applied CaCO3. The lime is assumed to be sourced from the nearest coastal lime pit.
The gross margin data generated in EVALUS reflects crop yields that are gradually affected by the changes in soil pH as influenced by applying lime at various amounts and frequencies or by not applying lime at all.
Greenhouse gas emissions
In this study, we report the three categories of greenhouse gas emissions, as stated in the Greenhouse Gas Protocol Agricultural Guidance (GGP 2015). Scope 1 emissions are those produced directly by farm operations, whereas Scope 2 emissions are those associated with energy purchases. Indirect emissions in the value chain are the source of scope 3 emissions. Scope 3 emissions in this study refer to emissions generated during the manufacture of fertilisers and other agricultural chemicals as well as from transport of farm inputs. The Scope 1, 2 and 3 emissions in this study were calculated using the framework for greenhouse gas accounting created by the Primary Industry Climate Change Centre (PICCC) (Lopez et al. 2022, 2023). Within those three categories of emissions, this study accounts for three gases: Carbon Dioxide (CO2), Methane (CH4) and Nitrous Oxide (N2O).
In the natural environment, lime dissolves over a few years, depending on the soil’s acidity, and thereby produces carbon dioxide. However, the National Inventory on emissions and the Intergovernmental Panel on Climate Change (IPCC) assumes all emissions associated with lime in agriculture are accounted for in the year of application (Barton et al. 2014; DCCEEW 2023). This study reports the impact of liming on emissions over 30 years and uses the total emissions over that period when comparing the various land use sequences and liming strategies. This study has not considered any abatement cost of emissions, so land use gross margins are not affected by any assumed cost of emission abatement. However, gross margins are affected by the likely gradual change in soil pH that is associated with the practice or absence of liming.
In summary, this study employs the IPCC and National Inventory assumption that all emissions associated with lime in agriculture are accounted for in the year of application. We realise that in practice those emissions would in fact be spread over several years. However, by aggregating emissions over a 30 year period the sum of assumed pulses of emissions will be very similar to the sum of a pattern of more graduated emissions. Importantly, the calculation of land use gross margins does reflect the graduated change in soil pH associated with liming. Soil organic carbon sequestration is not included in this study. We link changes in crop yields to changes in soil pH, although rainfall, crop sequence and soil type are also key yield factors considered in this study.
Lime scenarios
The simulation model assumes that the soil pH across the soil profile (from surface to 30 cm) is 4.5. This is considered a degraded and acidified profile. In the analysis a baseline and three other scenarios are assessed:
The baseline scenario considers that no lime is applied during the 30 years of the study period. However, at the end of the period, the cost of soil quality recovery via lime incorporation is included (i.e. to put the soil back to its original pH condition). This scenario considers that a rotation would need to be modified whenever the potential yield of the crop in the rotation is reduced by more than 40% due to worsening soil acidity. Canola is the first acid-sensitive crop in a land use sequence that is replaced by another less acid-sensitive break crop, lupin. The second crop to be removed due to acidity is barley, keeping wheat and lupin as the remaining crops in the rotation. Finally, when the acidity effect on wheat and lupin reduces their yield by more than 40%, then volunteer pasture is introduced for grazing by sheep.
Scenario 1 involves surface liming every 3 years with the same quantity of lime applied every 3 years. The quantity applied ensures a pH equal to 5 in the 10–20 cm layer at the end of the analysis period.
Scenario 2 considers surface liming at a rate of application of 2 tonnes per hectare whenever acidity reduces the potential yield by more than 10% from the previous year.
Scenario 3 also applies 2 tonnes of lime to the surface in the year following whenever the pH in the layer from the surface to 10 cm is lower than 4.8. When the pH between 10 cm and 30 cm in the soil profile is lower than 4.8, the model considers incorporating 2 tonnes of lime with deep incorporation.
Fig. 2 illustrates how and when liming occurs under the various scenarios, using the location, York, and its rotation sequences, as an example. Over the 30 years, in various years under each scenario for the initial wheat:barley:canola (WBC) rotation, liming applications are triggered by reductions in yields of the various crop phases caused by acidification of the soil profile.
Comparison of the Baseline and Scenarios 1, 2 and 3, for the location of York, considering an initial 100% cropping rotation based on Wheat (W), Barley (B) and Canola (C). Due to soil acidification, the baseline rotation shifts to a rotation that includes Lupin (L), and then finally transitions to volunteer pasture (VP). Scenarios 1, 2 and 3 show the tonnage of lime applied in two ways: a surface application (s) or a deep incorporation application (d).
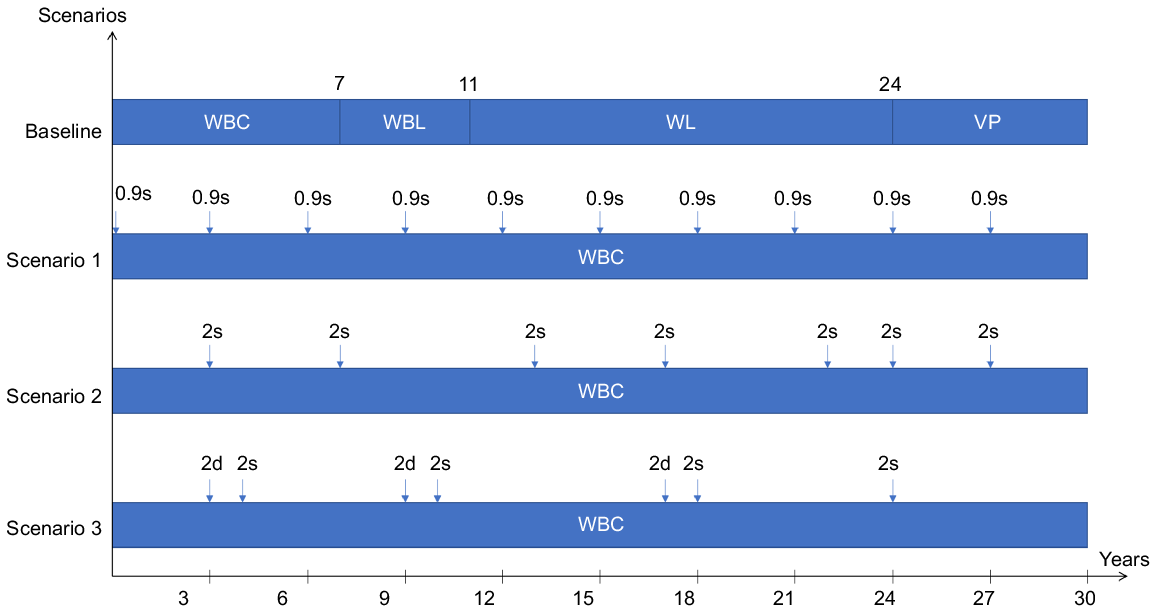
Rotations
The crop rotations assessed at the 14 locations are listed in Table 1. The rotation selected at each location was the rotation in common practice at that location, according to industry experts (Harries et al. (2015) and Planfarm (2016–2020)).
Location | Code | Soil type | Rotations | |||
---|---|---|---|---|---|---|
100% Cropping | 75% Cropping | 50% Cropping | ||||
Northampton | NORn | Deep sand | WWC | WWCLp | WLpLpC | |
Moora | MOO | Deep sand | WBC | WBCLp | WLpLpC | |
York | YOR | Shallow sandy duplex | WBC | WBCLp | WLpLpC | |
Katanning | KAT | Shallow sandy duplex | WBC | WBCLp | BLpLpC | |
Kojonup | KOJ | Shallow sandy duplex | WBC | WBCLp | BLpLpC | |
Carnamah | CAR | Deep sand | WWC | WWCLp | WLpLpC | |
Wongan Hills | WON | Deep sand | WWC | WBCLp | WLpLpC | |
Cunderdin | CUN | Shallow sandy duplex | WWC | WBCLp | WLpLpC | |
Lake Grace | LAK | Shallow sandy duplex | WBC | WBCLp | BLpLpC | |
Ravensthorpe | RAV | Shallow sandy duplex | WBC | WBCLp | WLpLpC | |
Mullewa | MUL | Deep sand | WWC | WWCLp | WLpLpC | |
Kalannie | KAL | Deep sand | WWC | WWLp | WLpLpC | |
Merredin | MER | Shallow sandy duplex | WWC | WWCLp | WWLpLp | |
Hyden | HYD | Shallow sandy duplex | WBC | WBCLp | WBLpLp |
W, wheat; B, barley; C, canola; Lp, legume pasture.
Results and discussion
Liming is profitable at almost all locations and for all rotations considered
Fig. 3 shows the net present value of gross margin differences across the 30 years between each scenario and the baseline (no lime) at each location. A 7% discount rate was applied to the calculations of net present values. For 100% cropping rotations, across all the locations, an economic incentive to apply lime, irrespective of the scenario, was revealed. Moreover, the economic incentive was greater in higher rainfall locations compared to low rainfall locations.
Net present value (NPV) of the net margin difference between the three scenarios analysed versus the baseline at each of the 14 locations. The NPV of the net margin of the baseline scenario, i.e. no lime, was subtracted from the NPV of each scenario and the box and whisker present the difference. Data are presented as box and whisker charts, in which the centre value is the median. The start and finish of the whiskers are the 25th and 75th percentiles. (a) 100% cropping, (b) 75% cropping, and (c) 50% cropping.
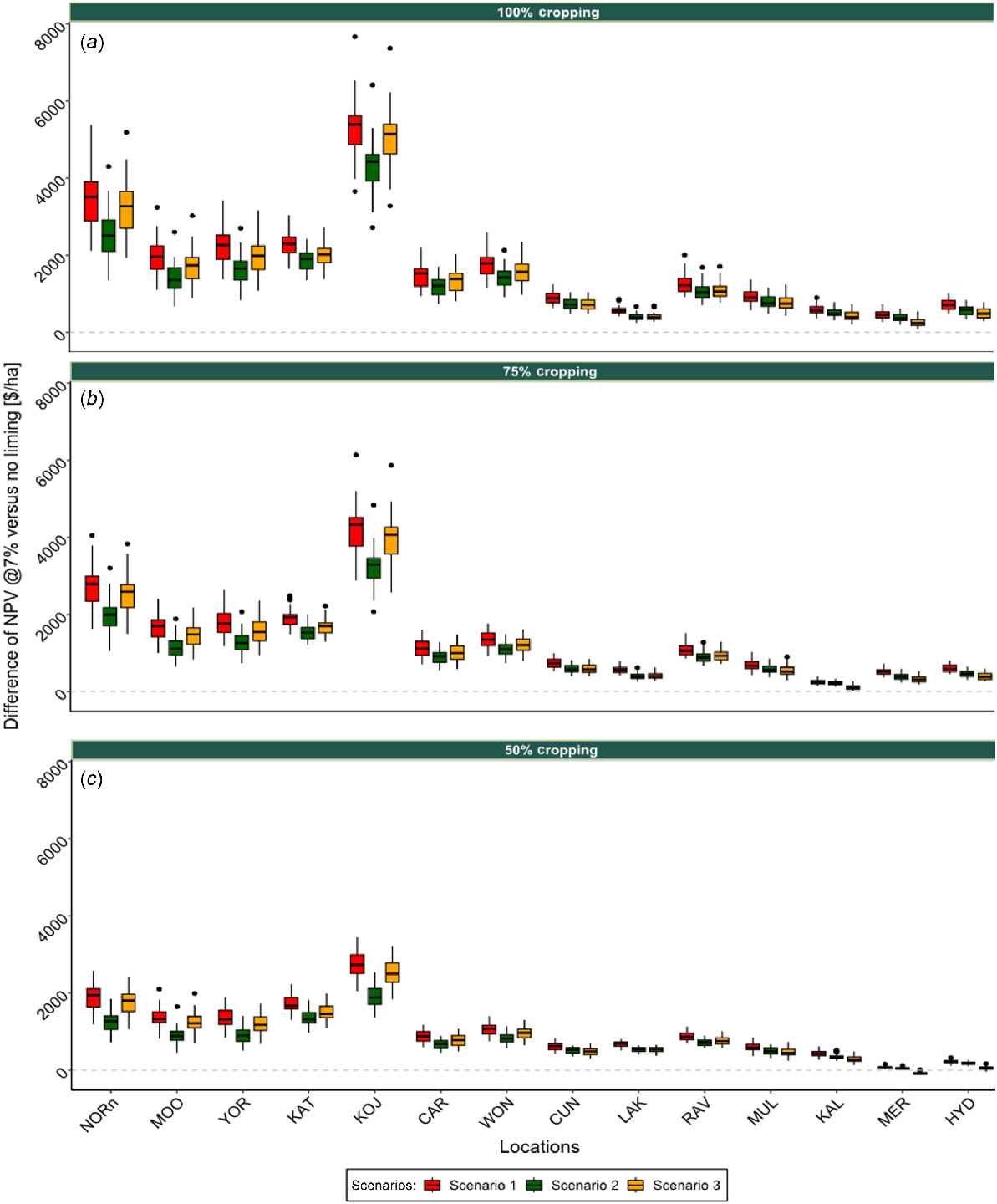
For rotations that featured less emphasis on cropping (i.e. 75% and 50% cropping), the incentive to apply lime generally remained. Only at a couple of locations was there little incentive to apply lime. For instance, at Merredin with 50% cropping, there was virtually no incentive to apply lime under any of the scenarios.
At locations with higher rainfall, there was a much wider spread in the net present value (NPV) of gross margin differences across the 30 years between each analysed scenario and the baseline (no lime). For some farmers, this variation in the commercial attractiveness of liming could complicate their liming choices. Nonetheless, the positive and large expected returns to liming, mostly in high and medium rainfall locations where crop-dominant land use occurs, suggest that liming is a commercially sensible business choice. Moreover, the much higher net present values from liming applications at higher rainfall locations indicated liming helped protect the high values of farmland at those locations; noting that farmland valuations are mostly conditional on the magnitude and durability of profit streams from agriculture.
At low rainfall locations, the NPVs of the per-hectare net margin differences between rotations inclusive of liming versus the absence of liming were small. These results suggest that liming is far less commercially attractive at these low rainfall locations. Over 30 years, the average NPV of benefits provided from liming was AUD 280 $/ha in Merredin (low rainfall) for 100% cropping whereas it was approximately AUD 1468 $/ha in Katanning (high rainfall). However, unlike the situation at high rainfall locations, in low rainfall locations farm sizes are often large (Fig. 4). Accordingly, when small positive per-hectare margins are multiplied by thousands of hectares, as is the case at several low rainfall locations, then the aggregate effect on farm business finances could be large. As shown in Fig. 4 there can be a three or four-fold difference in farm size between low and high-rainfall locations (e.g. Kalannie versus Katanning), causing small per-hectare commercial advantages to translate into sizeable business returns.
The results in Fig. 3 highlight the importance of understanding the framing of a farmer’s liming decision. Is it simply a paddock-based decision where the focus is solely on returns from liming in that paddock; or is the liming decision one that can be replicated across the farm and therefore it is the aggregate impact of liming on the entire farm business that needs to be stressed? As farms are not only agricultural systems but also business entities, our view is that the impact on the entire business should be given precedence rather than simply highlighting a per-hectare impact.
The source of the additional profit generated by liming is worth emphasising. In the absence of liming many current farm practices gradually worsen soil acidity and eventually restrict land use to less profitable grazing options. Liming, by lessening the productivity restrictions imposed by soil acidity, enables a wider and more prolonged profitable sequence of land uses. Hence, liming is a profitable and productivity-enhancing soil management practice in almost all situations where problematic soil acidity is a feature or is poised to soon become a problem.
Liming increases emissions, with some exceptions
Fig. 5 shows the summation across the 30 years of the difference in emissions between the three scenarios analysed and the baseline, at each of the 14 locations for the three intensities of cropping. At many locations, irrespective of the initial land use strategy (i.e. 50%, 75% or 100% cropping), including lime in the rotations increased emissions. However, at some locations given some land use strategies, reductions in emissions occurred when liming was practised.
Summation across the 30 years of the difference in greenhouse gas (GHG) emissions between the three scenarios analysed and the baseline for each location at different intensities of cropping. (a) 100% cropping, (b) 75% cropping, and (c) 50% cropping. CO2e, CO2 equivalents.
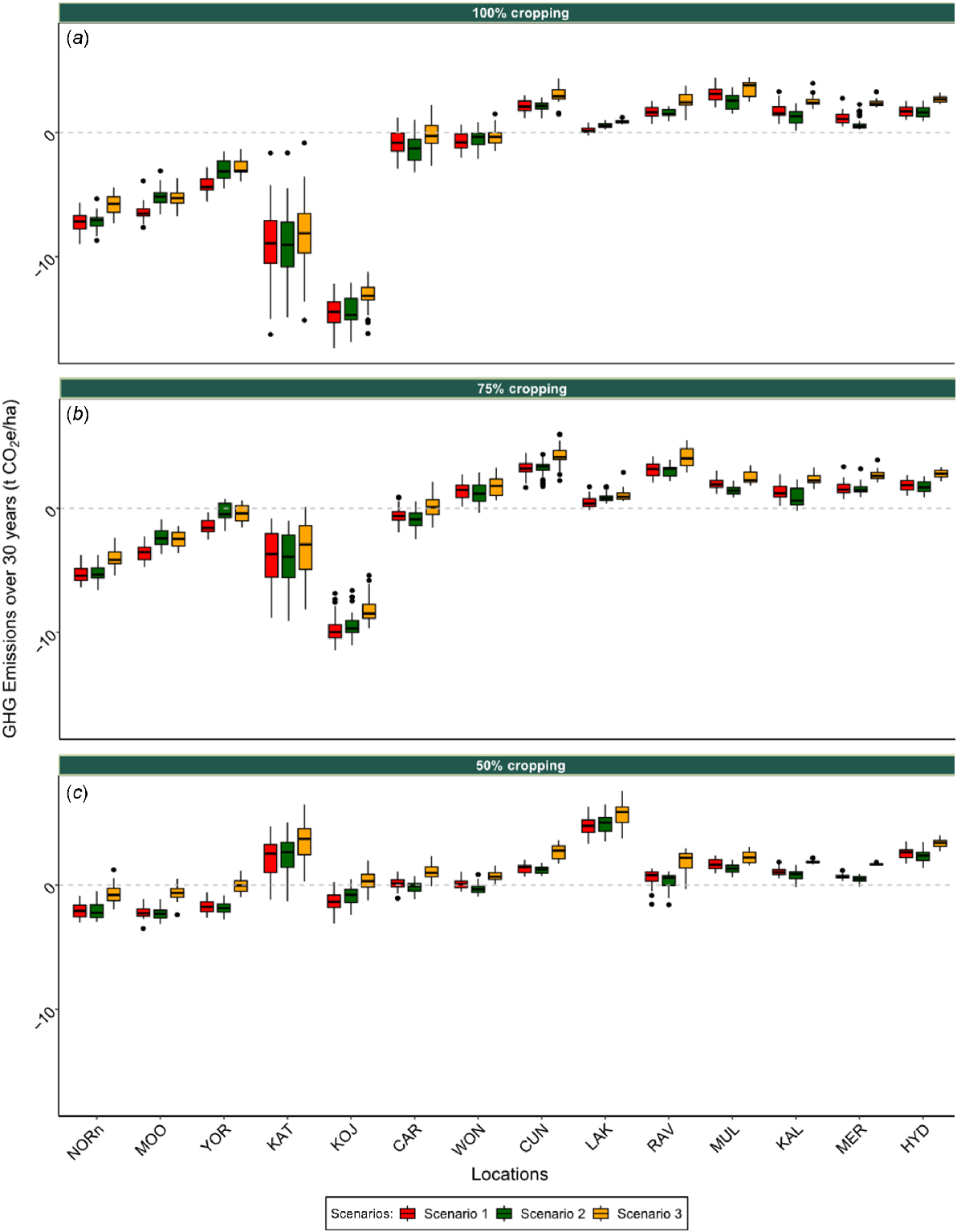
For example, at some northerly locations with deep sands (Northampton, Moora), their relatively high rates of application of nitrogenous fertilisers and low soil carbon content led to their high rates of soil acidification. In the absence of liming, soil acidification eventually led to an inability to profitably grow canola and subsequently wheat, forcing the introduction of volunteer pasture for sheep production. The upshot of the transition into sheep production caused an increase in emissions. Hence, over the 30-year period of land use at these sites, the overall emissions from land use with liming were less than emissions in the absence of liming.
Similarly, at southern sites like Katanning and Kojonup with their higher rainfall, in cases where their farming systems might already be crop dominant (i.e. 75% and 100% cropping), if soil acidification was not prevented via liming, then eventually those initially crop-dominant systems became less viable. Absence of liming at these locations caused a gradual reduction in yields of canola and then wheat, forcing a land use change into sheep production that then generated more emissions. Liming, by preventing a land use change towards sheep production, caused lesser emissions when crop-dominant land use was able to be maintained.
However, at the southern sites, Katanning and Kojonup, in cases where 50% cropping occurred, the higher rainfall firstly supported sheep enterprises with higher stocking rates yet secondly caused more nitrogen to be fixed during pasture phase, thereby reducing the need for nitrogen fertiliser and hence slowing the rate of acidification. The overall impact was that at 50% cropping, the average emissions associated with liming were greater than emissions in the absence of liming, despite the higher stocking rate.
Overall, at many locations, and especially with crop-dominant land use sequences (i.e. 75% and 100% cropping) at medium and low rainfall locations, lime applications generated more emissions over the 30 years of analysis. By illustration, at Cunderdin (medium rainfall) and Merredin (low rainfall), lime strategies generated an average of 1.82 and 1.07 t CO2e/ha respectively more emissions over 30 years compared to no lime at 100% cropping and 2.64 and 1.46 t CO2e/ha more emissions at 75% cropping. At medium and low rainfall locations often rates of application of nitrogenous fertilisers were lower and rates of soil acidification were lower. Hence at these locations the forced alteration of land use due to acidification towards sheep production was far less likely and so on average liming increased emissions.
Liming allows greater land use flexibility
Barley, canola and lupin are major break crops in Western Australia’s wheat-dominant farming systems that characterise much of the study region (Seymour et al. 2012; Harries et al. 2015). Pulses like faba beans, chickpeas and field peas play much smaller roles (Paterson and Wilkinson 2023) as break crops. All these traditional break crops are sensitive to soil acidity, with aluminium toxicity being a major limitation on crop yield (French and White 2005; Wang et al. 2006; Lofton et al. 2010). Under acidic conditions choice of break crop becomes problematic, forcing farmers to apply higher rates of herbicides and fungicides as the break crop option for pest management becomes limited. The increase in chemical use increases both farm costs and emissions. Furthermore, the limited choices affect the farmer’s ability to respond to market and weather signals.
Soil acidity not only limits farmers’ crop choices, but it also decreases crop competitiveness against weeds. The survival rate of weeds increases under acidic conditions and crop herbicide efficiency decreases (Hashem and Borger 2018; Borger et al. 2020). Hence, liming a soil can increase the competitiveness of crops and decrease weed burdens, enabling more effective weed control (Gazey et al. 2014).
Although legume crops like lupins are not specifically considered in this study, it is worth noting that the ability to reduce farm emissions is aided whenever legume crops can be profitably included in land use sequences, as these legume crops sequester nitrogen for following cereals (d’Abbadie et al. 2024b) and lessen reliance of applications of nitrogen fertilisers with all their associated emissions generated during their manufacture and transport. Acidity in a soil reduces the chances of rhizobium inoculation that forms nitrogen-fixing nodules on legume crops, especially when the pH is less than 5.5 CaCl2, hence reducing the nitrogen available for a following crop (GRDC 2022). Poor nodulation causes increases in fertiliser applications in cereal phases that follow legume crops. Furthermore, whenever plant growth is limited by acidity then resulting ground cover can also reduce, thereby increasing the risk of soil loss or degradation from wind or water erosion. Loss of topsoil can generate expensive long-lasting impacts. However, in this analysis we ignore this additional environmental benefit (i.e. wind erosion costs avoided) of liming.
Conclusion
The bio-economic simulation modelling of land use sequences on acid soils or soils prone to acidity, at 14 locations in the agricultural region of Western Australia, reveals that liming is in most situations a profitable soil ameliorant. However, in many cases, but not all, liming generates additional greenhouse gas emissions.
Yet by preventing worsening soil acidity, liming enables a wider array of farm enterprises to remain commercially attractive. Hence, liming often boosts farm profits, and supports greater species diversity, although at many locations and under some land use scenarios increased greenhouse gas emissions occur.
Caveats
Soil systems are inherently complex, characterised by intricate bio-physical-chemical interactions that surpass the scope of this paper’s models. The primary aim of this study is not to model the biogeochemical carbon cycle but to assess the economic aspects of liming and greenhouse gas (GHG) accounting. Specifically, this work evaluates the effect of lime dissolution on soil pH over several years and its subsequent impact on crop yield, while also considering aggregate emissions. Additionally, it is important to note that CO2 release in biological systems can occur through various pathways (Page et al. 2009). However, this study assumes that all emissions originate directly from the amended soil.
Data availability
The data that support this study will be shared upon reasonable request to the corresponding author.
Author contributions
Sud Kharel: Conceptualisation, Data curation, Formal analysis, Investigation, Methodology, Software, Supervision, Validation, Visualisation, Writing – original draft. Christophe d’Abbadie: Data curation, Formal analysis, Investigation, Methodology, Software, Validation, Visualisation, Writing – original draft. Amir Abadi Ghadim: Conceptualisation, Methodology, Project administration, Resources, Supervision. Chris Gazey: Data curation, Methodology, Resources, Validation. Ross Kingwell: Supervision, Validation, Writing – original draft, Writing – review and editing.
Acknowledgements
This study was supported by the Western Australian Department of Primary Industries and Regional Development and the Grains Research and Development Corporation through the project Western Australia Farming Systems project (DAW2204-003RTX) and the Western Australian Government Climate Action policy program.
References
Anderson G, Bell R (2019) Wheat grain-yield response to lime application: relationships with soil pH and aluminium in Western Australia. Crop & Pasture Science 70, 295-305.
| Crossref | Google Scholar |
Anderson WK, Hamza MA, Sharma DL, D’Antuono MF, Hoyle FC, Hill N, Shackley BJ, Amjad M, Zaicou-Kunesch C (2005) The role of management in yield improvement of the wheat crop – a review with special emphasis on Western Australia. Australian Journal of Agricultural Research 56, 1137-1149.
| Crossref | Google Scholar |
Azam G, Gazey C (2021) Slow movement of alkali from surface-applied lime warrants the introduction of strategic tillage for rapid amelioration of subsurface acidity in south-western Australia. Soil Research 59, 97-106.
| Crossref | Google Scholar |
Baligar VC, Pitta GVE, Gama EEG, Schaffert RE, Bahia Filho AFdC, Clark RB (1997) Soil acidity effects on nutrient use efficiency in exotic maize genotypes. Plant and Soil 192, 9-13.
| Crossref | Google Scholar |
Barton L, Thamo T, Engelbrecht D, Biswas WK (2014) Does growing grain legumes or applying lime cost effectively lower greenhouse gas emissions from wheat production in a semi-arid climate? The Journal of Cleaner Production 83, 194-203.
| Crossref | Google Scholar |
Borger CPD, Azam G, Gazey C, van Burgel A, Scanlan CA (2020) Ameliorating soil acidity–reduced growth of rigid ryegrass (Lolium rigidum) in wheat. Weed Science 68, 426-433.
| Crossref | Google Scholar |
Brennan RF, Bolland MDA, Bowden JW (2004) Potassium deficiency, and molybdenum deficiency and aluminium toxicity due to soil acidification, have become problems for cropping sandy soils in south-western Australia. Australian Journal of Experimental Agriculture 44, 1031-1039.
| Crossref | Google Scholar |
Caires EF, Garbuio FJ, Churka S, Barth G, Corrêa JCL (2008) Effects of soil acidity amelioration by surface liming on no-till corn, soybean, and wheat root growth and yield. European Journal of Agronomy 28, 57-64.
| Crossref | Google Scholar |
Chien SH, Kallenbach RL, Gearhart MM (2010) Liming requirement for nitrogen fertilizer-induced soil acidity: a new examination of AOAC Guidelines. Better Crops 94(2), 8-10.
| Google Scholar |
DAFF (2023) Snapshot – Western Australia agricultural exports 2022. Department of Agriculture, Fisheries and Forestry. Available at https://www.agriculture.gov.au/about/news/western-australia-agricultural-export-snapshot-2022
Davies S, Gazey C, Bowden B, van Gool D, Gartner D, Liaghati T, Gilkes B (2006) Acidification of Western Australia’s agricultural soils and their management. In ‘“Groundbreaking Stuff”, Proceedings of the 13th Australian Agronomy Conference,’ Warragul, Australia. (Australian Society of Agronomy Inc.)
Davies S, Armstrong R, Macdonald L, Condon J, Petersen E (2019) Chapter 8: Soil constraints: a role for strategic deep tillage. In ‘Australian agriculture in 2020: from conservation to automation’. (Eds J Pratley, J Kirkegaard) pp. 117–135. (Agronomy Australia and Charles Sturt University: Wagga Wagga, NSW)
DCCEEW (2023) National inventory report 2021. Department of Climate Change, Energy, the Environment and Water. Available at https://www.dcceew.gov.au/sites/default/files/documents/national-inventory-report-2021-volume-1.pdf
Dempster F, Davies S, Gazey C, Piggott P, Kragt M (2021) Will regenerative agriculture disrupt industry practice? The Farm Policy Journal 18, 26-34.
| Google Scholar |
Department of Primary Industries (2024) What is an acidic soil? Available at https://www.dpi.nsw.gov.au/components/module/module-tabs/acidity-and-liming-ac.19/what-is-an-acidic-soil [accessed 13 May 2024]
Dolling PJ, Porter WM, Robson AD (1991) Effect of soil acidity on barley production in the south-west of Western Australia. 1. The interaction between lime and nutrient application. Australian Journal of Experimental Agriculture 31, 803-810.
| Crossref | Google Scholar |
Dolling PJ, Porter WM, Rowland IC (1994) Acidification rates in the central wheatbelt of Western Australia. 2. On a sandy duplex soil. Australian Journal of Experimental Agriculture 34, 1165-1172.
| Crossref | Google Scholar |
DPIRD (2019) iLime Technical Notes. Department of Primary Industries and Regional Development. Available at https://www.agric.wa.gov.au/sites/gateway/files/iLimeTechnicalNotes.pdf
DPIRD (2021) Farming systems. Department of Primary Industries and Regional Development. Available at https://www.wa.gov.au/system/files/2021-08/DPIRD%20Research%20Highlights%2C%20Farming%20Systems.pdf
d’Abbadie C, Kharel S, Kingwell R, Abadi Ghadim A (2024a) Profitable, low-emission nitrogen application strategies in Western Australian dryland cropping. Crop & Pasture Science 75, CP23057.
| Crossref | Google Scholar |
d’Abbadie C, Kharel S, Kingwell R, Abadi Ghadim A (2024b) Should crop sequences in Western Australia include more lupins? Crop & Pasture Science 75, CP23110.
| Crossref | Google Scholar |
Fisher J, Clausen J, Evans F, Gazey C (2019) iLime – an app for assessing the management of soil acidity in agricultural systems. Available at https://library.dpird.wa.gov.au/cgi/viewcontent.cgi?article=1022&context=ssswa_conf
French B, White P (2005) Soil and environmental factors affecting pulse adaptation in Western Australia. Australian Journal of Agricultural Research 50, 375-387.
| Google Scholar |
Gazey C, Davies S, Master R (2014) Soil acidity: a guide for WA farmers and consultants. Bulletin 4858. (Department of Agriculture and Food: Western Australia) Available at https://library.dpird.wa.gov.au/bulletins/223/
GGP (2015) GHG Protocol Agricultural Guidance. p. 103. Greenhouse Gas Protocol. Available at https://ghgprotocol.org/sites/default/files/standards/GHG%20Protocol%20Agricultural%20Guidance%20%28April%2026%29_0.pdf [Accessed 21 June 2022]
GIWA (2023) GIWA crop report – February 2023. Grains Industry Association of Western Australia. Available at https://www.giwa.org.au/wa-crop-reports/2022-season/giwa-crop-report-february-2023/#:~:text=The%20total%20grain%20production%20in,record%20year%20up%20until%202021
GIWA (2024) GIWA crop report – February 2024. Grains Industry Association of Western Australia. Available at https://www.giwa.org.au/wa-crop-reports/2023-season/giwa-crop-report-february-2024/
GRDC (2022) Paddock practices: sowing legumes in acidic soils – the steps to success. Grain Research and Development Corporation. Available at https://grdc.com.au/resources-and-publications/all-publications/paddock-practices/2022/south/march/paddock-practice-sowing-legumes-in-acidic-soils-the-steps-to-success
Hajkowicz S, Young M (2005) Costing yield loss from acidity, sodicity and dryland salinity to Australian agriculture. Land Degradation & Development 16, 417-433.
| Crossref | Google Scholar |
Harries M, Anderson GC, Huberli D (2015) Crop sequences in Western Australia: what are they and are they sustainable? Findings of a four-year survey. Crop & Pasture Science 66, 634-647.
| Crossref | Google Scholar |
Hashem A, Borger C (2018) Lime improves control of wild radish and annual ryegrass in acid soils of Western Australia. In ‘21st Australasian Weeds Conference’. (The Weed Society of New South Wales Inc.) Available at https://caws.org.nz/old-site/awc/2018/awc201811531.pdf
Kalkhoran SS, Pannell DJ, Thamo T, White B, Polyakov M (2019) Soil acidity, lime application, nitrogen fertility, and greenhouse gas emissions: optimizing their joint economic management. Agricultural Systems 176, 102684.
| Crossref | Google Scholar |
Kalkhoran SS, Pannell D, Thamo T, Polyakov M, White B (2020) Optimal lime rates for soil acidity mitigation: impacts of crop choice and nitrogen fertiliser in Western Australia. Crop & Pasture Science 71, 36-46.
| Crossref | Google Scholar |
Kharel S (2022) ‘EVALUS user manual.’ 1st edn. (Western Australian Department of Primary Industries and Regional Development: Perth, WA, Australia) Available at https://www.researchgate.net/publication/375120988_Version_1_EVALUS_User_Manual
Kharel S, d’Abbadie C, Abadi A, Kingwell R (2022) Reducing farming system emissions via spatial application of payoff functions. Agricultural Systems 203, 103534.
| Crossref | Google Scholar |
Kingwell R, Islam N, Xayavong V (2020) Farming systems and their business strategies in south-western Australia: a decadal assessment of their profitability. Agricultural Systems 181, 102827.
| Crossref | Google Scholar |
Kunhikrishnan A, Thangarajan R, Bolan NS, Xu Y, Mandal S, Gleeson DB, Seshadri B, Zaman M, Barton L, Tang C, Luo J, Dalal R, Ding W, Kirkham MB, Naidu R (2016) Functional relationships of soil acidification, liming, and greenhouse gas flux. In ‘Advances in agronomy. Vol. 139’. (Ed. DL Sparks) pp. 1–71. (Academic Press) doi:10.1016/bs.agron.2016.05.001
Li GD, Conyers MK, Helyar KR, Lisle CJ, Poile GJ, Cullis BR (2019) Long-term surface application of lime ameliorates subsurface soil acidity in the mixed farming zone of south-eastern Australia. Geoderma 338, 236-246.
| Crossref | Google Scholar |
Liu DL, Helyar KR, Conyers MK, Fisher R, Poile GJ (2004) Response of wheat, triticale and barley to lime application in semi-arid soils. Field Crops Research 90, 287-301.
| Crossref | Google Scholar |
Lofton J, Godsey CB, Zhang H (2010) Determining aluminum tolerance and critical soil pH for winter canola production for acidic soils in temperate regions. Agronomy Journal 102, 327-332.
| Crossref | Google Scholar |
Lopez MB, Ekonomou A, Eckard RJ (2022) A greenhouse accounting framework for crop production (G-GAF) based on the Australian National Greenhouse Gas Inventory methodology. (Primary Industries Climate Challenges Centre, University of Melbourne: Melbourne, Vic, Australia) Available at http://www.piccc.org.au/resources/Tools [updated October 2022]
Lopez MB, Dunn J, Wiedemann S, Eckard R (2023) A greenhouse accounting framework for beef and sheep properties based on the Australian National Greenhouse Gas Inventory methodology. Primary Industries Climate Challenges Centre, University of Melbourne, Melbourne, Vic, Australia. Available at http://www.piccc.org.au/resources/Tools
Mkhonza NP, Buthelezi-Dube NN, Muchaonyerwa P (2020) Effects of lime application on nitrogen and phosphorus availability in humic soils. Scientific Reports 10, 8634.
| Crossref | Google Scholar | PubMed |
MLA, AWI (2024) Sheep producers intentions survey, May 2024. Meat and Livestock Australia and Australian Wool Innovation. Available at https://www.mla.com.au/contentassets/c72d8679f1e74579baba6bdcceeac0cc/spis---06-may-2024---final-report.pdfNSW
Observatory of Economic Complexity (2024) Wheat. Available at https://oec.world/en/profile/hs/wheat?yearSelector1=2021
Page KL, Allen DE, Dalal RC, Slattery W (2009) Processes and magnitude of CO2, CH4, and N2O fluxes from liming of Australian acidic soils: a review. Australian Journal of Soil Research 47, 747-762.
| Crossref | Google Scholar |
Pang Z, Tayyab M, Kong C, Hu C, Zhu Z, Wei X, Yuan Z (2019) Liming positively modulates microbial community composition and function of sugarcane fields. Agronomy Journal 9, 808.
| Crossref | Google Scholar |
Paterson J, Wilkinson I (2023) Western Australian pulse industry. Department of Primary Industries and Regional Development. Available at https://www.agric.wa.gov.au/pulses/western-australian-pulse-industry
Planfarm (2016–2020) Planfarm Benchmarks. Available at https://www.planfarm.com.au/resources/
Rengel Z (2003) ‘Handbook of soil acidity.’ 1st edn. (Marcel Dekker Inc.) Available at https://www.routledge.com/Handbook-of-Soil-Acidity/Rengel/p/book/9780824708900
Schroder JL, Zhang H, Girma K, Raun WR, Penn CJ, Payton ME (2011) Soil acidification from long-term use of nitrogen fertilizers on winter wheat. Soil Science Society of America Journal 75, 957-964.
| Crossref | Google Scholar |
Seymour M, Kirkegaard JA, Peoples MB, White PF, French RJ (2012) Break-crop benefits to wheat in Western Australia – insights from over three decades of research. Crop & Pasture Science 63, 1-16.
| Crossref | Google Scholar |
Thilakarathna MS, Raizada MN (2017) A meta-analysis of the effectiveness of diverse rhizobia inoculants on soybean traits under field conditions. Soil Biology and Biochemistry 105, 177-196.
| Crossref | Google Scholar |
Wang J-P, Raman H, Zhang G-P, Mendham N, Zhou M-X (2006) Aluminium tolerance in barley (Hordeum vulgare L.): physiological mechanisms, genetics and screening methods. Journal of Zhejiang University Science B 7, 769-787.
| Crossref | Google Scholar | PubMed |
Whitten MG, Wong MTF, Rate AW (2000) Amelioration of subsurface acidity in the south-west of Western Australia: downward movement and mass balance of surface-incorporated lime after 2-15 years. Soil Research 38, 711-728.
| Crossref | Google Scholar |
Zhao W, Zhang J-B, Müller C, Cai Z-C (2018) Effects of pH and mineralisation on nitrification in a subtropical acid forest soil. Soil Research 56, 275-283.
| Crossref | Google Scholar |
Zhu X, Ros GH, Xu M, Xu D, Cai Z, Sun N, Duan Y, de Vries W (2024) The contribution of natural and anthropogenic causes to soil acidification rates under different fertilization practices and site conditions in southern China. Science of The Total Environment 934, 172986.
| Crossref | Google Scholar |