Effect of Epichloë gansuensis endophyte on rhizosphere bacterial communities and nutrient concentrations and ratios in the perennial grass species Achnatherum inebrians during three growth seasons
Wenpeng Hou
A State Key Laboratory of Grassland Agro-ecosystems Lanzhou University; Center for Grassland Microbiome; Key Laboratory of Grassland Livestock Industry Innovation (Ministry of Agriculture and Rural Affairs); Engineering Research Center of Grassland Industry (Ministry of Education); College of Pastoral Agriculture Science and Technology, Lanzhou University, Lanzhou, Gansu 730000, PR China.
B Retired from AgResearch, Grasslands Research Centre, Private Bag 11-008, Palmerston North 4442, New Zealand.
C State Key Laboratory of Plateau Ecology and Agriculture, Qinghai Academy of Animal and Veterinary Sciences, Qinghai University, Xining, Qinghai 810016, PR China.
D Corresponding author. Email: wangjf12@lzu.edu.cn
Crop and Pasture Science 71(12) 1050-1066 https://doi.org/10.1071/CP20145
Submitted: 4 May 2020 Accepted: 29 October 2020 Published: 7 December 2020
Journal Compilation © CSIRO 2020 Open Access CC BY-NC-ND
Abstract
Achnatherum inebrians is an invasive perennial grass widespread in natural grasslands of north-west China and plays an important role in grassland ecological restoration. The presence of the seed-borne endophytic fungus Epichloë gansuensis in A. inebrians promotes grass growth, increases resistance to abiotic stress, and affects the rhizosphere microbial community of host plants. However, the relationships among E. gansuensis, rhizosphere bacteria and plant contents of carbon (C), nitrogen (N), phosphorus (P) and potassium (K) during different growing seasons are not clear. We examined changes in the rhizosphere bacterial community and in nutrient contents and ratios in A. inebrians with (E+) and without (E−) E. gansuensis in May, August and December. The Shannon diversity index was higher for rhizosphere bacteria of E+ than E− plants in the three different seasons. Leaf C, N and P contents and root P and K contents were higher in E+ than E− plants in May, and leaf K and root C were higher in E+ than E− plants in August. Leaf C : N ratios were lower in E+ than E− plants in December, and leaf C : K ratios were lower in E+ than E− plants in August and December. In addition, our results indicate significant interactions among rhizosphere bacteria, C, N, P and K contents, and endophyte treatment in three different seasons. In conclusion, E. gansuensis enhanced the C, N, P and K contents of host plants, and affected nutrient ratios of A. inebrians probably by increasing rhizosphere bacterial diversity and altering rhizosphere bacterial community structure. This study provides new findings on the ecological function of the endophyte E. gansuensis, including its potential role in enhancing soil fertility. The improvements in soil fertility were utilised in extrapolating to forage grass–endophyte associations.
Keywords: different growth phase, endophyte, rhizosphere bacterial diversity, stoichiometry.
Introduction
Maintaining high agricultural productivity and forage yield in the face of increased global food demand and natural resource consumption is a principal problem (Cassman 1999; Tilman et al. 2002). Over the last few decades, crop yields have not increased in proportion with increasing fertiliser inputs, resulting in increasing environmental risks and inefficient use of nutrients (Zhang et al. 2010a). The need to develop strategies to overcome this challenge has drawn attention to the importance of harnessing the biological potential inherent in rhizosphere processes for improving the use efficiency of crop nutrients. In the rhizosphere zone, important interactions occur among the plant, soil and microorganisms, and rhizosphere microbes can be regarded as an important factor for plant health. Rhizosphere microbial communities could govern plant nutrient chemistry and regulate plant growth and root health (Marschner et al. 2004; Dotaniya and Meena 2015). In addition, rhizosphere microorganisms can also impact nutrient uptake and plant growth by releasing growth-inhibiting or growth-stimulating substances that influence root structure and root physiology (Calvo et al. 2017), and stimulate hormonal regulation (Nihorimbere et al. 2011). Marschner et al. (2004) used PCR-DGGE (denaturing gradient gel electrophoresis) technology to analyse specific microbial groups in the microbial community, and found that many different factors contribute to shaping species composition and numerical predominance of specific bacteria; these factors include pH, soil type, soil fertility, plant nutrition status, plant species and plant age. Therefore, plant microbial ecologists are interested in the extent to which these communities are associated with changes in various environmental factors (Marschner et al. 2004; Ryu et al. 2005; Govindasamy et al. 2009). The biological activity of both the microorganisms and the root structure could play a central role in nutrient availability (Marschner 2012).
The rhizosphere microbial communities of different plants in the same soil are distinct (Ibekwe and Kennedy 1998; Schlatter et al. 2019). Much evidence has shown that the rhizosphere bacterial community will change during the growth and development of the plant (Houlden et al. 2008; Ziegler et al. 2013), which indicates that the plant growth phase has a key function in regulating the structure of the rhizosphere soil bacterial community (Kourtev et al. 2002; Fierer et al. 2013). Studies also showed that rhizosphere microbial structure and function are influenced by discrepancies of rhizodeposition and root exudation in the different zones in root, related to soil types, cultural practices, crop rotation and tillage (Miller et al. 1989; Westover et al. 1997; Grayston et al. 1998; Horwath et al. 1998; Lupwayi et al. 1998; Latour et al. 1999; Maarastawi et al. 2018; Man et al. 2020). Interactions between rhizosphere bacteria and plants also have an important effect on the mineral content of plants (Pii et al. 2015), and nutrient deficiencies might be overcome by rhizosphere bacteria (Zhang et al. 2010a). Microorganisms such as plant-growth-promoting rhizobacteria and mycorrhizal fungi decompose organic matter by releasing hydrolytic enzymes, thereby increasing soil nutrient availability (Miransari 2011; Ollivier et al. 2011; Meena et al. 2017). If the benefits of rhizosphere communities to plants are to be positively manipulated, a better understanding of the importance of plant factors and soil for the rhizosphere microbiome is required.
Plant nutrients play an important function in stoichiometry, ecosystem nutrient cycling and their relationship (Yang and Luo 2011; Reed et al. 2012). Carbon (C) accounts for 99% of the living biomass of plants. Nitrogen (N), potassium (K) and phosphorus (P) are indispensable macronutrients in the process of plant growth; however, in ecosystems they are usually a major factor limiting plant growth and development (Reef et al. 2010). Nitrogen, P, and K govern both larger scale biogeochemical patterns and physiological constraints of organisms (Sterner and Elser 2002). The demand for these elements is not fully understood in different plant growth stages and plant organs (Ågren 2008; Yang and Luo 2011). Some studies demonstrated that plant age and tissue are key factors regulating stoichiometry and nutrient levels (Niklas and Cobb 2006; Yang and Luo 2011). In addition, increasing plant age alters the distribution pattern of metabolites, which leads to changes in nutrient ratios and levels (Ågren 2008; Niklas and Cobb 2006; Yang and Luo 2011). The P : C, K : C and N : C ratios of leaves all decrease over a growth season (Ågren 2008).
Achnatherum inebrians (Hance) Keng (Stipa inebrians Hance) is a perennial grass species, and is widespread in natural grasslands of north-west China, including the subalpine and alpine grasslands of Gansu, Inner Mongolia, Xinjiang, Tibet and Qinghai (Li et al. 2004b). In western China, A. inebrians has become a dominant invasive species and plays an important role in grassland ecological restoration. A major characteristic of A. inebrians is that almost all plants are hosts of asexual endophytic fungi transmitted by seeds. This finding led to ongoing research into this grass–endophyte association, and included the initial assigning of the name Neotyphodium gansuense to the endophyte (Li et al. 2004b; Nan and Li 2004). In 2015, a re-examination of the endophytes in A. inebrians found that there were in fact two species, and these were assigned the names Epichloë gansuensis and E. inebrians (Chen et al. 2015). Li et al. (2004a) reported the characteristics of the Epichloë gansuensis endophyte: conidia solitariae, subglobosae, ellipsoidea, semiglobularea, reniformia, hyalina, saepe transversaliter affixa, hyalinae, laterales vel intercalares.
Extensive research has examined the consequences of the presence of the endophyte on A. inebrians and the ecosystem. The root system of A. inebrians is very developed, including the length of primary root and the numbers of lateral and fibrous roots. Xia (2018) showed that the root surface area of A. inebrians with endophyte (E+) was significantly greater than of A. inebrians without endophyte (E−). The root surface area of A. inebrians, both E+ and E−, increased first and then decreased with soil depth, and the maximum superficial area of root was at 10–30 cm soil depth under drought stress. The endophyte E. gansuensis dramatically promoted growth over the total root length in A. inebrians plants, with the greatest root length of both E+ and E− plants at 10–30 cm soil depth (Xia 2018). Epichloë gansuensis improved A. inebrians growth under low-N stress by modulating stoichiometry and increasing N-utilisation efficiency of the plant (Wang et al. 2018b). Epichloë gansuensis significantly affects bacterial diversity in A. inebrians rhizosphere soil; Li (2017) found the dominant bacteria of A. inebrians–E+ rhizosphere soil to be Bacillus, Hydrogenophaga and Pseudomonas spp. Several studies have demonstrated that the presence of E. gansuensis increases the tolerance of A. inebrians to biotic and abiotic stresses, including resistance to herbivores, diseases and pests (Li et al. 2007; Zhang et al. 2012), cadmium stress (Zhang et al. 2010b), NaCl stress (Wang et al. 2019, 2020a, 2020c), cold stress (Chen et al. 2016), drought stress (Xia et al. 2018), and low N stress (Wang et al. 2018a, 2018b). In addition, Epichloë symbionts in other grasses increase tolerance to abiotic stresses, including salt tolerance in tall fescue (Festuca arundinacea) (Reza and Mirlohi 2010) and Hordeum brevisubulatum (Song et al. 2015a), drought tolerance in tall fescue (White et al. 1992), and waterlogging tolerance in H. brevisubulatum (Song et al. 2015b). One study demonstrated that the interaction of grazing and Epichloë endophytes affected the contents of P, silicon and phenolic compounds in meadow fescue (Schedonorus pratensis), with silicon content ~16% higher in E+ than E− plants (Huitu et al. 2014).
Epichloë endophytes have powerful biological functions that affect the economic and ecological value of artificial and natural grasslands; therefore, further study to explore possible mechanisms and applications is warranted. Recent studies demonstrated that E. gansuensis influences rhizosphere bacterial community structure and root-associated fungal community structure, and clarified the effect of this endophyte on the relationship between soil nutrients and rhizosphere soil (Zhong et al. 2018; Ju et al. 2020). However, whether the presence of E. gansuensis can affect both the rhizosphere bacterial community structure and the leaf and root nutrient contents (C, N, P and K) in A. inebrians is poorly understood. The above information indicates that the rhizosphere microbial community plays an important role in nutrient absorption by plants. How E. gansuensis influences rhizosphere bacteria and regulates nutrient accumulation by the host grass is little understood. The effect of E. gansuensis on the rhizosphere bacterial community in host Achnatherum inebrians during different seasons of the yearly growing period remains unclear. Therefore, to study the effect of E. gansuensis on rhizosphere bacteria and nutrient accumulation by host grasses during different growth seasons, it is useful to gain understanding of the symbiotic relationship between A. inebrians and E. gansuensis.
The aims of this study are to explore: (i) the influence of E. gansuensis on rhizosphere bacterial community structure and diversity under A. inebrians in spring (May), autumn (August) and early winter (December); (ii) the influence of E. gansuensis on C, N, P and K contents in A. inebrians leaves and roots in May, August and December; and (iii) the influence of E. gansuensis on the relationship between rhizosphere bacteria and plant nutrients during the three growth seasons.
Materials and methods
Experimental site and rhizosphere sample collection
The experimental site was established at Yuzhong campus of Lanzhou University in 2011. Two regions of a site were established: one A. inebrians with E. gansuensis (E+), the other A. inebrians without E. gansuensis (E−). The seeds of E+ and E− A. inebrians plants derive from a single E+ plant. Half of the seeds were maintained as E+ for use in the study. The other half were treated with a 100-fold dilution of the fungicide thiophanate-methyl (active ingredient 70% methyltobizine; Jiangsu Longdeng Chemical Co., Ltd) for 2 h, and then the seeds were washed with sterile water. This is an effective method to kill the seedborne fungal endophyte of A. inebrians (Li et al. 2016; Hou et al. 2020). In order to ensure that the field plants in the E+ and E− plots were as required, aniline blue staining was used to check the infection of seedlings from the fungicide-treated and untreated seeds by microscopic examination of leaf-sheath pieces. The existence of endophyte on plants in the experimental areas was also evaluated by aniline blue staining to observe the presence of characteristic hyphae of E. gansuensis in leaf sheaths.
The study had three independent replicates. Each replicate comprised two blocks (one E+ and one E–) of dimensions 1.05 m by 2.45 m. Each block had 12 plants arranged in six rows × two lines, all 35 cm apart. Each plant represented one plot. The distance between E+ and E− blocks in a replicate was 2.5 m. There are 36 plants of each of E+ and E– per replicate, respectively. In the 6 years after establishment, the experimental area was affected by only natural environmental conditions, with no impact of livestock or wildlife. Three rhizosphere soil samples were collected from plants in E+ and E− plots in 2016; all samples from E+ plots showed E+ plants, and all samples from E− plots showed E− plants. The mean annual temperature was 11.6°C, and the annual rainfall was 400 mm year–1 in 2016.
Rhizosphere soil, leaves and roots were collected on 15 May, 15 August and 15 December 2016. The leaves and roots were dried at 80°C to constant weight, and were used to measure contents of C, N, P and K. Samples were transferred to sterile and dry polyethylene bags with a sterilised spoon. Five individual E+ or E− A. inebrians plants were sampled for the rhizosphere soil (0–20 cm soil depth) and root samples in five random plots, and these five individual samples were blended into a composite E+ or E− sample. The leaves from the same five individual E+ or E− A. inebrians plants were blended into a composite E+ or E− leaf sample. Sampling was performed with three independent repetitions. All samples of rhizosphere soil were sieved through a 2-mm sieve, and stored 4°C for subsequent isolation of culturable rhizosphere soil bacteria.
Field soil nutrient compositions during the two respective growth seasons of May and August were found in our recently published paper (Hou et al. 2020), and the field soil nutrient compositions in December were as follows (mean ± s.e.): organic C 0.45 ± 0.05% in E+ soil, and 0.48 ± 0.03% in E– soil; total N (mg g–1) 0.35 ± 0.01 in E+ soil, and 0.34 ± 0.01 in E– soil; total P (mg g–1) 0.63 ± 0.08 in E+ soil, and 0.59 ± 0.02 in E– soil; nitrate-N (mg kg–1) 1.57 ± 0.01 in E+ soil, and 1.48 ± 0.12 in E– soil; ammonium-N (mg kg–1) 29.30 ± 1.87 in E+ soil, 23.34 ± 1.47 in E– soil.
Isolation and identification of rhizosphere bacteria
The culture media used in the study are shown in Table 1. Each rhizosphere soil sample (1 g) was added to 100 mL of the different sterilised liquid media, and cultured with constant shaking (150 rpm) at 28°C. After 12 h, the soil suspension was plated on the corresponding agar culture media using the dilution plate method, and incubated for another 24 h at 28°C until colonies appeared. Each colony was transferred to fresh liquid medium of the same type to establish a pure culture. After 12 h of constant shaking (150 rpm) at 28°C, bacterial suspensions were plated onto corresponding agar media, and then incubated for another 24 h at 28°C until colonies appeared. For preservation of bacteria, each colony from the different agar media was transferred to 20 mL KB liquid medium, which was subsequently cultured with constant shaking (150 rpm) at 28°C. After 24 h, 700 μL each bacterial suspension and 700 μL 50% glycerol were mixed for storage at –80°C. Identification of rhizosphere soil bacteria was performed by PCR with 16S primers 27F (5′-AGAGTTTGATCCTGGCTCAG-3′) and 1492R (5′-GGTTACCTTGTTACGACTT-3′) (Wang et al. 2015). The PCR products were sequenced, and the 16S rDNA sequences were compared with the GenBank databases by using BLAST.
![]() |
Assay of plant nutrient contents
Total organic C in leaves and roots was measured according to the method of Sparks and Bartels (1996). Total P and N contents in the leaf and root were measured according to Song et al. (2015a), and K content in leaves and roots was measured using the method of Bao et al. (2016). Data on the contents of C, N, P and K from the leaves and roots were used to calculating C : N, C : P, N : P, N : K and P : K ratios of the leaves and roots.
Calculations and statistical analyses
Calculation of relative frequency of a taxon was by the formula of the number of colonies in a particular taxon divided by the total number of colonies in all taxa (Su et al. 2010). The diversity was assessed with the Shannon index (H′):
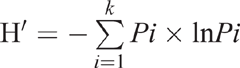
where k is the total number of each replicate in the genus level, and Pi is the relative frequency of each replicate in the genus level (Su et al. 2010).
SPSS 17.0 software (SPSS, Chicago, IL, USA) was used to analyse data. The significance difference between E+ and E− plant rhizosphere soils for all of the parameters was determined by independent t-tests. Two-way analysis of variance (ANOVA) was used to analyse the effects of endophyte and growth seasons on the Shannon index. Three-way ANOVA was used to analyse the effects of endophytic fungi, growth seasons and plant tissue on the contents of plant C, N), P and K. Three-way ANOVA tested the effects of endophyte, growth season and tissue on plant C : N, C : P, C : K, N : P, N : K and P : K ratio. Statistical significance was defined at the P = 0.05 confidence level, and means are evaluated with standard errors. RDA was performed using Canoco 4.5 software and PCA was performed using Statistic 6.0.
Results
Composition of rhizosphere bacterial community of E+ and E− plants during different growth seasons
The composition of the culturable rhizosphere bacterial communities for the different growth seasons of E+ and E− plants is shown in Supplementary Material, Table S1 (available at the journal’s website). In total, 201 rhizosphere bacterial colonies were obtained from E+ and E− A. inebrians plant rhizosphere soil sampling in May, of which 116 were from E+ samples, and 85 from E− samples. In August, 275 rhizosphere bacterial colonies were obtained, of which 170 were from E+ samples and 105 from E− samples. In December, 225 rhizosphere bacterial colonies were obtained, of which 127 were from E+ samples, and 98 from E− samples.
The isolates of rhizosphere bacteria from E+ plants in May were classified into eight orders and 11 genera. The orders (with genera in parentheses) were Actinomycetales (Paenarthrobacter, Microbacterium), Streptomycetales (Streptomyces), Rhizobiales (Rhizobium), Enterobacterales (Enterobacter), Pseudomonadales (Acinetobacter, Pseudomonas), Bacillales (Bacillus), Sphingobacteriales (Sphingobacterium) and Flavobacteriales (Chryseobacterium, Flavobacterium) (Supplementary Table S1). Isolation of rhizosphere bacteria from E− plants in May found five orders and nine genera: Actinomycetales (Pseudarthrobacter, Microbacterium), Rhizobiales (Rhizobium, Ochrobactrum), Xanthomonadales (Stenotrophomonas), Pseudomonadales (Pseudomonas) and Bacillales (Bacillus, Brevibacillus, Lysinibacillus) (Supplementary Table S1). Bacillus, Rhizobium and Microbacterium were the common genera that occurred in rhizosphere soil of E+ and E− plants at the May sampling (Supplementary Table S1).
Rhizosphere bacteria from E+ plants in August belonged to nine orders and were classified into 19 genera (Supplementary Table S1). The orders and genera were Actinomycetales (Glutamicibacter, Arthrobacter, Pseudarthrobacter, Microbacterium), Corynebacteriales (Rhodococcus), Rhizobiales (Rhizobium), Burkholderiales (Variovorax), Enterobacterales (Enterobacter, Erwinia, Pantoea, Serratia), Pseudomonadales (Acinetobacter, Pseudomonas), Xanthomonadales (Stenotrophomonas), Bacillales (Bacillus, Brevibacillus, Lysinibacillus, Paenibacillus) and Sphingobacteriales (Olivibacter). By contrast, the rhizosphere bacteria from E− plants in August belonged to seven orders and were classified into 11 taxa: Actinomycetales (Arthrobacter, Microbacterium), Corynebacteriales (Rhodococcus), Rhizobiales (Ensifer), Enterobacterales (Enterobacter, Kluyvera, Pantoea), Pseudomonadales (Acinetobacter, Pseudomonas) and Xanthomonadales (Stenotrophomonas) (Supplementary Table S1). Kluyvera and Ensifer were unique to the E-plant rhizosphere soil in August. Acinetobacter, Arthrobacter, Bacillus, Enterobacter, Microbacterium, Pantoea, Pseudomonas and Stenotrophomonas were the common genera that occurred in rhizosphere soil of E+ and E− plants in the August sampling (Supplementary Table S1).
At the December sampling, bacteria in the rhizosphere soil of E+ plants belonged to four order and 13 genera. These were: Actinomycetales (Arthrobacter, Microbacterium, Paenarthrobacter), Burkholderiales (Massilia, Limnobacter), Enterobacterales (Enterobacter, Leclercia, Erwinia, Pantoea), Pseudomonadales (Pseudomonas, Acinetobacter) and Bacillales (Bacillus and Lysinibacillus). The rhizosphere bacteria from E− plants in December belonged to five orders and 10 genera: Actinomycetales (Paenarthrobacter, Glutamicibacter), Burkholderiales (Massilia), Enterobacterales (Enterobacter, Leclercia, Citrobacter, Kosakonia), Pseudomonadales (Acinetobacter, Pseudomonas) and Bacillales (Bacillus). Acinetobacter, Bacillus, Enterobacter, Leclercia, Massilia, Paenarthrobacter and Pseudomonas were the genera that were present in rhizosphere soil of both E+ and E− plants at the December sampling (Supplementary Table S1). Isolates of Arthrobacter, Erwinia, Pantoea, Microbacterium, Limnobacter and Lysinibacillus were confined to rhizosphere soil of E+ plants in December. Isolates of Glutamicibacter, Kosakonia and Citrobacter were isolated only in rhizosphere soil of E− plants during this sampling season.
In summary, the presence of E. gansuensis markedly influenced the A. inebrians rhizosphere soil bacterial community, and Bacillus was dominant genus in rhizosphere soil of E+ plants and E− plants during the three different growth seasons.
Shannon index of rhizosphere bacteria of E+ and E− plants during different growth seasons
The Shannon index is used to reflect the alpha diversity and can be used to estimate the microbial diversity in the sample. The larger the Shannon index, the higher diversity of the community. Here, use of the Shannon index can reflect the richness and uniformity of each species in the community. Our data showed that two genera, Pseudomonas and Bacillus, both had high relative frequency in rhizosphere soil of E+ and E− plants in May (Fig. 1a), and that the relative frequency of Pseudomonas was highest (27.51%) and Acinetobacter lowest (2.59%) in rhizosphere soil of E+ plants in May (Fig. 1a). The relative frequency of Bacillus was highest (49.38%) and Pseudarthrobacter lowest (3.53%) in rhizosphere soil of E− plants in May (Fig. 1a). Two genera, Acinetobacter and Bacillus, both had high relative frequency in rhizosphere soil of E+ and E− plants in August (Fig. 1b), and the relative frequency of Bacillus was highest (14.71%) and Olivibacter lowest (1.82%) in rhizosphere soil of E+ plants in August (Fig. 1b). The relative frequency of Arthrobacter was highest (18.00%) and Ensifer lowest (2.82%) in rhizosphere soil of E− plants in August (Fig. 1b). Three genera, Pseudomonas, Bacillus and Enterobacter, had high relative frequency in rhizosphere soil of E+ and E− plants in December (Fig. 1c), and the relative frequency of Bacillus was highest (19.60%) and Massilia lowest (2.36%) in rhizosphere soil of E+ plants in December (Fig. 1c). The relative frequency of Pseudomonas was highest (28.74%) and Citrobacter lowest (3.30%) in rhizosphere soil of E− plants in December (Fig. 1c).
We also found that the Shannon index was different between the rhizosphere bacteria communities of E+ and E− A. inebrians in May, August and December. The Shannon index of the rhizosphere bacteria of E+ plants was higher than that of E− plants in all seasons, and was enhanced by 28.5% in May, 21.4% in August and 11.4% in December compared with E− plants (Fig. 1d). ANOVA showed that endophyte treatment had a significant (P < 0.001) impact on the Shannon index, as did season (Table 2). There was also a significant (P = 0.007) endophyte × season interaction effect on the Shannon index (Table 2). In summary, E. gansuensis significantly increased the Shannon index of the community of rhizosphere soil bacteria in A. inebrians during the three different growth seasons.
![]() |
Contents of C, N, P and K in leaves and roots of E+ and E− plants during the different growth seasons
The presence of E. gansuensis had significant effects on the C, N, P and K contents of leaves and C, P and K contents of roots during one or more of the growth seasons (Fig. 2). Leaf C content was significantly different between E+ and E− plants in May, being 18.9% higher in E+ plants (Fig. 2a). Similarly, E+ leaves had a higher content of N than E− leaves in May and December, with N content enhanced by 11.9% in May and 41.0% in December (Fig. 2b). Leaf P content was significantly different between E+ and E− plants in May and December, being higher in E+ than E− plants, with a relative increase of 20.8% in May (Fig. 2c). Leaf K content was significantly different between E+ and E− plants in August, being 12.5% higher in E+ plants (Fig. 2d). Root C content of E+ plants was higher than of E− plants in only August, with a relative enhancement of 9.8% (Fig. 2e). Root P and K contents of E+ plants were increased by 35.0% and 22.6%, respectively, compared with E− plants in May (Fig. 2g, h). However, there was no clear difference in root N content between E+ and E− plants in any season (Fig. 2f). Three-way ANOVA showed a significant (P = 0.002) main effect of endophyte treatment on content of N (but not C, P or K), whereas season and tissue effects were significant (P < 0.001) on contents of all four elements (Table 3). Significant two-way interaction effects included endophyte × season on N content (P = 0.001); endophyte × tissue on N (P < 0.001) and K (P = 0.030) contents; and season × tissue on N (P < 0.001), P (P < 0.001) and K (P < 0.001) contents (Table 3). The three-way interaction effect of endophyte × season × tissue was significant on K content (P < 0.001, Table 3). In summary, E. gansuensis had significantly different effects on the contents of C, N, P and K in A. inebrians leaves and roots during the three growth seasons.
Ratios among C, N, P, and K contents leaves and roots of E+ and E− A. inebrians during the different growth seasons
The ratios C : N, C : P and C : K increased over the growing season. The C : K ratio of E+ leaves was significantly (P < 0.05) higher than of E− leaves in May, but lower in August and December. The C : P ratios of E+ and E− leaves were not significantly different at any sampling. In December, the C : N ratio of E + leaves was remarkably (P < 0.05) lower than of E– leaves (Fig. 3a–c). The N : K ratio of E+ leaves was higher than of E− leaves in May and December, and the P : K ratio of E+ leaves was higher than of E− plants in May (Fig. 3e, f). The C : N and C : K ratios of E+ roots were significantly higher than of E− roots in August (Fig. 3g, i). Three-way ANOVA showed no significant main effect of endophyte treatment on the ratios C : N, C : P, C : K, N : P, N : K and P : K; however, season and tissue effects were significant on C : N, C : P, C : K, N : P and P : K (Table 4). Significant two-way interaction effects included endophyte × tissue on N : P ratio (P = 0.039), and season × tissue on C : N, C : K, N : P, N : K and P : K ratios (Table 4). The three-way interaction effect of endophyte × season × tissue was significant on C : K ratio (P = 0.043, Table 4).
Redundancy analysis of E. gansuensis, the different growth seasons, and C, N, P and K contents of leaves and roots
The first axis of the redundancy analysis explained 28.6% of the variance, and the second axis explained 27.4% of the variance (Fig. 4). According to the analysis, E+ was an important factor affecting the N, P and K contents of leaves and the P content of roots, whereas E− was a key factor affecting the C, N and K contents of roots and the C content of leaves (Fig. 4). Further, our results showed that May was positively correlated with the N, P and K contents of leaves and with P and N contents of roots; however, August was positively correlated with the C and K contents of leaves and with C and K contents of roots. December had a positively correlation with the C, N and K contents of roots (Fig. 4). The presence of E. gansuensis clearly affected the different nutrients of leaves and roots, and the growth season affected the different nutrients of leaves and roots in A. inebrians.
![]() |
Correlations of the composition of the rhizosphere bacterial community with C, N, P and K contents of E+ and E− A. inebrians during different growth seasons
Effects of all of the genera of rhizosphere bacteria of E+ and E− A. inebrians on the plant contents of C, N, P and K during the different growth seasons were estimated.
We found significant effects of rhizosphere bacteria on the C, N, P and K contents of leaves and roots between E+ and E− A. inebrians plants in May (Fig. 5). For example, the C, N, P and K contents of leaves of E+ plants were positively correlated with Bacillus, Acinetobacter, Paenarthrobacter and Streptomyces; and the N and P contents of leaves of E− plants were positively correlated with Bacillus and Stenotrophomonas (Fig. 5). The C and N contents of roots of E+ plants were positively correlated with Microbacterium and Flavobacterium; and the N and K contents of roots of E− plants were positively correlated with Bacillus and Stenotrophomonas (Fig. 5). The P content of roots of E+ plants was positively correlated with Pseudomonas, Enterobacter, Sphingobacterium, Chryseobacterium and Microbacterium; and the P content of roots of E− plants was positively correlated with Pseudomonas (Fig. 5).
![]() |
Similarly, our results also demonstrated significant effects of rhizosphere bacteria on the contents of C, N, P and K in leaves and roots between E+ and E− A. inebrians plants in August (Fig. 6). For example, the C and P contents of E+ leaves were positively correlated with Arthrobacter, Pantoea, Stenotrophomonas, Rhizobium, Paenibacillus and Bacillus; and the C, N and P contents of E− leaves were positively correlated with Microbacterium, Arthrobacter, Acinetobacter and Ensifer (Fig. 6). The N content of E+ leaves was positively correlated with Olivibacter and Lysinibacillus. The K content of E− leaves was positively correlated with Pseudomonas, Bacillus, Pantoea and Rhodococcus (Fig. 6). The C, N and K contents of E+ roots were positively correlated with Variovorax, Brevibacillus and Rhodococcus. The N and P contents of E− roots were positively correlated with Microbacterium, Arthrobacter, Acinetobacter and Ensifer (Fig. 6). The P content of E+ roots was positively correlated with Glutamicibacter and Acinetobacter. The C and K contents of E− roots were positively correlated with Kluyvera, Enterobacter and Stenotrophomonas (Fig. 6).
![]() |
Significant effects of rhizosphere bacteria on the C, N, P and K contents of leaves and roots between E+ and E− A. inebrians plants were also evident in December (Fig. 7). For instance, the C, P and K contents of E+ leaves were positively correlated with Pseudomonas, Lysinibacillus and Leclercia in December; and the C, N and K contents of E− leaves were positively correlated with Citrobacter, Pseudomonas and Leclercia (Fig. 7). The N content of E+ leaves was positively correlated with Bacillus, Erwinia, Pantoea, Microbacterium, Limnobacter and Leclercia. The P content of E− leaves was positively correlated with Enterobacter and Paenarthrobacter (Fig. 7). Further, the C and P contents of E+ roots were positively correlated with Enterobacter, Acinetobacter, Massilia, Arthrobacter and Paenarthrobacter. The N and P contents of E− roots were positively correlated with Enterobacter and Paenarthrobacter. The N and K contents of E+ roots were positively correlated with Bacillus and Pseudomonas. The C and K contents of E− roots were positively correlated with Leclercia and Kosakonia (Fig. 7).
![]() |
Figure 8 summarises results of principal component analysis for endophyte treatment in May, August and December; it shows that factors 1 and 2 explained >78% of total variance.
![]() |
Discussion
Endophytes of the genus Epichloë have been found in many cool-season grasses including Achnatherum inebrians, Elymus dahuricus, Festuca sinensis, F. elata, Hordeum brevisubulatum and Lolium perenne (Schardl et al. 2004; Song et al. 2015a; Wang et al. 2020a). A previous study showed that the relationships between Epichloë endophytes and grasses are considered mutually beneficial (Müller and Krauss 2005). Studies have reported that the relative abundances of the fungal component were dramatically distinct in different endophyte-treated bulk soils or rhizosphere soils, and that the presence of endophyte influences the structure of soil fungal community, but not the fungal total biomass in fescue soils (Rojas et al. 2016). Similarly, Zhong et al. (2018) found that A. inebrians infected with wild E. gansuensis endophyte had higher root-associated fungal diversity than A. inebrians infected with cultivated E. gansuensis; however, the presence of E. gansuensis obviously decreased root-associated fungal diversity compared with E− A. inebrians. Guo (2014) reported that, compared with other endophytes, Epichloë coenophiala AR584E+ and E. coenophiala CTE+ increased soil organic C content in rhizosphere soil. Franzluebbers et al. (1999) also showed that Neotyphodium coenophialum (Epichloë coenophiala) endophyte leads to small differences in soil microbial community structure between tall fescue plants with 0% and 100% endophyte infection.
In our study, communities of rhizosphere bacteria, and A. inebrians nutrients, were mediated by E. gansuensis endophyte infection and these are critical for ecosystem function and productivity. This research provides insights into how the A. inebrians–E. gansuensis symbiosis influences the rhizosphere microbial community, the C, N, P and K contents of the host grass, and the relationship between rhizosphere bacterial community and nutrient content leaves and roots of A. inebrians during three seasons of the growth period. Epichloë gansuensis increased the Shannon index of the rhizosphere bacterial community and changed the rhizosphere bacterial community (Fig. 1, Table 1). Zhong et al. (2018) reported that the interaction between soil disturbance and E. gansuensis leads to changes in fungi communities in the root association. Ju et al. (2020) demonstrated by using Illumina pyrosequencing that E. gansuensis increased the Shannon index of the A. inebrians rhizosphere soil bacterial community, which was consistent with our results. Further, the study of Hou et al. (2020) showed that E. gansuensis increased the activities of soil invertase, alkaline phosphatase and urease, and the contents of soil organic C, total N, available N and available P. Therefore, E. gansuensis perhaps regulates soil enzyme activity through reprograming soil microbial structure. Meanwhile soil enzymes participate in the soil nutrient cycle, which probably increases nutrient accumulation of host grasses to improve plant growth. Bansal et al. (2014) showed that with the increase in microbial diversity and richness, the activity of soil urease was also enhanced, and this increased N cycling, which is beneficial to the accumulation of host grass nutrients and plant growth. Similarly, our results indicated that E. gansuensis increased the uptake of nutrients (N, P and K) during the different growth seasons (Fig. 2). The study of Wang et al. (2020b) also indicated that beneficial bacteria (plant-growth promoting rhizobacteria, PGPR) activate nutrients (N, P and K) and promote wheat growth under reduced fertiliser application conditions. These PGPR function as biofertilisers; and likewise, the presence of E. gansuensis has a role as a biofertilisers because it enhances the presence of bacterial genera regarded as PGPR. Pseudomonas and Bacillus are dominant genera in May, Acinetobacter and Bacillus in August, and Pseudomonas, Bacillus and Enterobacter in December (Fig. 1).
The effect of an Epichloë endophyte on root-associated and rhizosphere microorganisms differs in different grass–Epichloë associations. The presence of an Epichloë endophyte in perennial ryegrass (Lolium perenne) and A. sibiricum suppressed arbuscular mycorrhizal fungi (AMF) colonisation, although this effect depended on endophyte strain, ryegrass cultivar and P supply (Liu et al. 2011; Zhou et al. 2016). A previous study demonstrated that Acremonium coenophialum (E. coenophiala) endophyte modulated N metabolism in tall fescue (Lyons et al. 1990). It is also reported that under salt stress and soil-water deficit conditions, the presence of an Epichloë endophyte affected the N and P contents in shoot and root of H. brevisubulatum and A. inebrians, and altered the stoichiometry of host grasses (Song et al. 2015a; Wang et al. 2019; Xia et al. 2018). Similarly, we found that E. gansuensis infection influenced C, N, P and K contents in leaves and roots of A. inebrians in May, August and December (Fig. 2), and changed the stoichiometry (elemental ratios) of host grasses (Fig. 3). Therefore, E. gansuensis could promote growth of host A. inebrians probably by regulating soil microbial community structure, soil enzyme activity and nutrient content, to increase nutrient accumulation. We found that E. gansuensis increased the N content of leaves compared with E− leaves in December (Fig. 2). However, in a previous study, endophyte infection had no clear influence on N-use efficiency of chewings fescue (Festuca rubra) (Richardson et al. 1999). Li et al. (2012) found that the benefits to A. sibiricum from endophyte infection largely depended on the supply of N and/or P, and in the case of P supply and N deficiency, there was no dramatic difference of N content in E+ and E− roots. According to our results, there were different E. gansuensis treatment effects on the contents of C, N, P and K in leaves and roots during the three different growth seasons (Fig. 2). Ju et al. (2020) reported that soil moisture had a clear influence on soil nutrients, especially nitrate-N, available N and P and total N; and E. gansuensis had obvious impacts on soil nutrients, especially available P and nitrate-N. Differences in soil moisture and temperature in the different growth seasons would likely have regulated soil nutrient content, and may be why E. gansuensis has seasonal effects on the nutrient content of the host grass.
Plants take up CO2, soil available N, available K, available P and other nutrient elements, and synthesise metabolites required for plant growth. The C, N, P and K contents of leaves and roots and their respective ratios changed with the plant growth season (Fig. 3). Owing to changes in C, N, P and K contents in different growth seasons and tissues, the C : N, C : P and C : K ratios in the leaves increased with growth stage. It is possible that the reduction in leaf C : N ratio of E+ plants was due to increased synthesis of N content compared with E− plants in December. Many researchers have proposed the growth-rate hypothesis, and this is one of the important paradigms for ecological stoichiometry (Elser et al. 1996, 2010). Low ratios of C : P and N : P exist in high-growth-rate species (Main et al. 1997). Our results show that the C : P ratios of leaves and roots decreased in May, supporting the growth-rate hypothesis. The N : P ratio of leaves is a key indicator to define the nutrient element limitations (Koerselman and Meuleman 1996). Our results reflected that the stoichiometry of E+ and E− plants should consider other elements such as K, and its elemental ratios N : K, C : K and K : P, which may respond to changing environment (Rivas-Ubach et al. 2012). Calvo et al. (2017) demonstrated that a mixture of four strains of plant-growth-promoting Bacillus spp. could enhance nutrient contents (N, P and K) by influencing root morphology at the early stages of maize (Zea mays) growth. A previous study by Xia (2018) indicated that E. gansuensis can regulate the root-system structure of A. inebrians. Therefore, our research implies that E. gansuensis has the role of promoting plant growth and stimulating the absorption of nutrients for the host grass, probably in part by regulating the root morphology of A. inebrians.
Epichloë endophytes have important biological and ecological function (Franzluebbers et al. 1999; Reza and Mirlohi 2010; Zhang et al. 2010b, 2012; Song et al. 2015a, 2015b; Rojas et al. 2016; Tian et al. 2017; Xia et al. 2018; Wang et al. 2020a). It was reported that root-associated fungi of uncultivated, wild, endophyte-infected A. inebrians show positive correlation with soil properties, but root-associated fungi of cultivated A. inebrians show negative correlation with soil properties (Zhong et al. 2018). However, there was no previous study into the relationship between rhizosphere bacteria and plant nutrients under E. gansuensis endophyte infection during the different growth seasons, and this led to the present study. Our results showed that E+ was positively correlated with the N, P and K contents of leaves and the P content of roots; however, E− was positively correlated with the C, N and K contents of roots and the C content of leaves (Fig. 4). Interestingly, Ju et al. (2020) reported that Proteobacteria was positively related with pH, available N and K, and nitrate-N in the rhizosphere, and negatively related with soil organic C and total N in the rhizosphere. Therefore, E. gansuensis probably influenced the rhizosphere soil bacterial community, and the microbial community is highly correlated with soil nutrients, and this would influence the absorption of nutrients for plants. A critical characteristic of the association between grass and Epichloë is that the endophyte is systemic within host grasses, but importantly, hyphae of these endophytic fungi are not present in roots (Christensen et al. 2008). The evidence from this present study is that E. gansuensis is an important factor positively affecting the nutrient content of leaves, with the exception of C.
There was a significant difference of relationship between rhizosphere bacteria and nutrient content of E+ and E− plants in the three different growth seasons. The grass A. inebrians grows in poor soil environments and E+ plants are becoming increasingly abundant on the barren grassland in north-west China, with nearly all A. inebrians plants being endophyte-infected. This suggests that the endophyte is beneficial, or at least not disadvantageous, to the host grass. Our previous study also showed that E. gansuensis improved tolerance to low N by regulating enzyme activity related to N metabolism and glucose-6-phosphate dehydrogenase activity (Wang et al. 2018a; Wang et al. 2018b). The present results confirm that E. gansuensis also provides an advantage by enhancing rhizosphere bacterial diversity, which probably further influenced enzyme activity and nutrient contents of soils, and positively affects absorption of nutrients by plants in natural conditions. As noted earlier, E. gansuensis enhances the activity of soil enzymes and contents of available N and P (Hou et al. 2020), and influences the diversity of the community of rhizosphere soil bacteria (Ju et al. 2020). Importantly, a study investigating response of rhizosphere soil properties, enzyme activities and microbial diversity to intercropping types on the Loess Plateau of China found that activities of soil invertase, soil phosphatase and soil urease had a positive correlation with the diversity of rhizosphere bacteria (Gong et al. 2019). The growing conditions of A. inebrians are often harsh with low nutrient availability. The present research shows that E. gansuensis increased rhizosphere bacterial diversity and enabled the A. inebrians host grass to have better growth status by accumulating greater quantities of nutrient elements when exposed to natural conditions. A study involving tall fescue indicated that Epichloë endophyte could increase adaptability of the grass to a P-deficient environment by regulating nutrient absorption (Malinowski et al. 1999, 2000). Feedback in the plant–soil system shows that plants affect characteristics of the rhizosphere, and then the rhizosphere affects the growth of the same or other plants (Ehrenfeld et al. 2005). Further, positive plant–soil feedback could come from increased availability of nutrients (Wardle et al. 1999; Chapman et al. 2006), or the accumulation of a mutualistic symbiont in the rhizosphere (Klironomos 2002). Until now, the key focus of studies into plant–soil feedback has been the effects of different plant species on soil properties (Burke et al. 1998), or on soil community composition (Vandenkoornhuyse et al. 2003; De Deyn et al. 2004; Innes et al. 2004; Kennedy et al. 2005). Therefore, E. gansuensis probably plays a significant role in positive plant–soil feedback by changing rhizosphere bacterial communities, which in turn enhances the nutritional status of A. inebrians.
Rhizosphere bacterial composition in A. inebrians was significantly related to the nutrient content of leaves and root (Figs 5 7). Many studies have confirmed the effect of rhizosphere microorganisms on plant nutrients; for example, Höflich et al. (1995) isolated two rhizosphere bacteria (Pseudomonas and Rhizobium) from soil that stimulated root length and branching, and promoted shoot and root dry weight of pea (Pisum sativum). Inoculation of PGPR enhanced wheat height and biomass, and enhanced the content of N, P and K in wheat shoots; further, after combined application of N fertiliser and PGPR, the speed of increase in nutrient accumulation and wheat yield was even higher (Abbasi et al. 2011). This was consistent with our results. Inoculations of Bacillus cereus, Brevibacillus reuszeri and Rhizobium rubi with manure enhanced plant weight, chlorophyll content, and N, K, and P content of broccoli compared with control (Yildirim et al. 2011). The present research also indicated that Bacillus had a positive correlation with N content of leaves of E+ and E− plants at May (Fig. 5). Bacillus promotes the recovery and absorption of K in Sudan grass (Sorghum vulgare Pers. var. sudanense) (Basak and Biswas 2009). The mechanism by which rhizosphere bacteria promote nutrient absorption may be related to their function in nutrient availability, because some rhizosphere bacteria have special biological functions. For example, Brevibacillus reuszeri and Ochrobactrum cytisi have P-solubilising function; Bacillus cereus, Bacillus, Pseudomonas and Rhizobium have N2-fixing function; and Rhizobium rubi has the of P-solubilising and N2-fixing functions (Glick 2012; Yildirim et al. 2011; Meena et al. 2017). All of these functions can be beneficial for promoting nutrient absorption. In addition, some rhizosphere bacteria can produce indole-3-acetic acid (IAA), for example, strains WPR-32, WPR-42 and WPR-51 from wheat rhizosphere (Abbasi et al. 2011), and IAA can contribute to the growth of plants. Our results also showed that Pseudomonas had a positive correlation with P content of roots of E+ plants in August (Fig. 6). Strains of Pseudomonas and Bacillus significantly increased the content of N, P and K in the roots and leaves of cotton (Gossypium sp.) and peas, and both can produce IAA (Egamberdiyeva and Höflich 2004). However, one study proved that some rhizosphere microorganisms are beneficial to plant yield and growth but they do not increase plant nutrient absorption; for example, some of the P-solubilising rhizobacteria isolated from field-grown crops can increase plant height and seed yield of canola but cannot promote P absorption of plants (De Freitas et al. 1997). Pseudomonas alcaligenes PsA15, Mycobacterium phlei MbP18 and Bacillus polymyxa BcP26 have a much better stimulatory role on N, P and K absorption of maize in nutrient-deficient soil than fertile soil (Egamberdiyeva 2007). Betaproteobacteria are very important in the process of plant nutrient cycling and growth; different growth periods strongly affect communities of Betaproteobacteria in potato rhizospheres, and different potato cultivars have differing rhizosphere effects on their communities (Inceoglu et al. 2010). In our study, E. gansuensis increased P content of leaves and roots in May, but had no effects on P content of leaves and roots in August and December, possibly because E. gansuensis activity and quantity were greater in May and the host A. inebrians plants were experiencing rapid growth. The development and structure of the rhizosphere bacterial community are affected by different factors such as plant species, plant age and soil type (Canbolat et al. 2006; Roesti et al. 2006; Qiao et al. 2017). This is consistent with our results, with the diversity and community structure of rhizosphere soil being different at the three sampling dates. The N level of plant tissue and soil N availability were significantly related to the change of rhizosphere bacterial community structure. One study found that at the peak of plant growth, the absorption rate of N by plants was inversely proportional to the level of microbial biomass N, which caused a change of bacterial community structure in the rhizosphere (Bell et al. 2015). This may explain the differences observed in our study of the rhizosphere bacterial community between E+ and E– plants in different growth seasons, which led to changes of plant nutrients.
Important findings of this study are that E. gansuensis enhanced A. inebrians rhizosphere bacterial community diversity at three different growth phases, with the Shannon index highest in August. Contents of C, N, P and K in E+ leaves and of C, P and K in E+ roots were also greater than of E− plants during one or more of the growth seasons. Moreover, the N, P and K contents of leaves and the P content of roots were positively correlated with E. gansuensis endophyte infection, whereas the C, N and K contents of roots and the C content of leaves were positively correlated with E−. There were significant impacts of rhizosphere bacteria on the C, N, P and K contents of E+ and E− A. inebrians leaves and roots in May, August and December. Bacillus had positive correlation with N content of leaves of E+ and E− host grasses in May; Pseudomonas had positive correlation with P content of roots of E+ plants in August; Pseudomonas had positive correlation with the C content of leaves and roots in E+ and E− plants in December. Overall, our results indicate that the mutualistic grass–Epichloë symbiosis has potential to affect the community structure of rhizosphere bacteria and promote host-grass nutrient uptake, improving the yield and quality of grass. The results suggest that host grasses–Epichloë endophyte symbiosis could enhance the competitive ability of host growth and the economic value of grasses in natural rangelands.
Author contributions
Jianfeng Wang and Zhibiao Nan designed the research. Jianfeng Wang, Wenpeng Hou and Zhibiao Nan performed the research. Jianfeng Wang, Zhibiao Nan, Chao Xia, Tao Chen, Xiuzhang Li and Wenpeng Hou analysed the data. Jianfeng Wang, Wenpeng Hou and Michael J. Christensen wrote the paper.
Conflicts of interest
The authors declare that there are no conflicts of interest.
Acknowledgements
This research was financially supported by Program for Changjiang Scholars and Innovative Research Team in University (IRT_17R50), the Joint Fund of the Natural Science Foundation of China and the Karst Science Research Center of Guizhou Province (Grant No. U1812401), the Natural Science Foundation of China (32001399), the Technical Service Agreement for Research and Development of Beneficial Microbial Agents of Rhododendron Alpine (071200001), Lanzhou University ‘Double First-Class’ guiding special project-team construction fund – scientific research start-up fee standard (561119206), Guizhou Education Department Program (Qianjiaohe-KY-2018–130), Major Science and Technology Sub-project of Guizhou Science and Technology Program (Qiankehe-2019-3001-2).
References
Abbasi MK, Sharif S, Kazmi M, Sultan T, Aslam M (2011) Isolation of plant growth promoting rhizobacteria from wheat rhizosphere and their effect on improving growth, yield and nutrient uptake of plants. Plant Biosystems 145, 159–168.| Isolation of plant growth promoting rhizobacteria from wheat rhizosphere and their effect on improving growth, yield and nutrient uptake of plants.Crossref | GoogleScholarGoogle Scholar |
Ågren GI (2008) Stoichiometry and nutrition of plant growth in natural communities. Annual Review of Ecology, Evolution, and Systematics 39, 153–170.
| Stoichiometry and nutrition of plant growth in natural communities.Crossref | GoogleScholarGoogle Scholar |
Bansal OP, Singh G, Katiyar P (2014) Effect of untreated sewage effluent irrigation on heavy metal content, microbial population and enzymatic activities of soils in Aligarh. Journal of Environmental Biology 35, 641–647.
Bao AK, Du BQ, Touil L, Kang P, Wang QL, Wang SM (2016) Co-expression of tonoplast Cation/H+ antiporter and H+-pyrophosphatase from xerophyte Zygophyllum xanthoxylum improves alfalfa plant growth under salinity, drought and field conditions. Plant Biotechnology Journal 14, 964–975.
| Co-expression of tonoplast Cation/H+ antiporter and H+-pyrophosphatase from xerophyte Zygophyllum xanthoxylum improves alfalfa plant growth under salinity, drought and field conditions.Crossref | GoogleScholarGoogle Scholar | 26268400PubMed |
Basak BB, Biswas DR (2009) Influence of potassium solubilizing microorganism (Bacillus mucilaginosus) and waste mica on potassium uptake dynamics by sudan grass (Sorghum vulgare Pers.) grown under two Alfisols. Plant and Soil 317, 235–255.
| Influence of potassium solubilizing microorganism (Bacillus mucilaginosus) and waste mica on potassium uptake dynamics by sudan grass (Sorghum vulgare Pers.) grown under two Alfisols.Crossref | GoogleScholarGoogle Scholar |
Bell CW, Asao S, Calderon F, Wolk B, Wallenstein MD (2015) Plant nitrogen uptake drives rhizosphere bacterial community assembly during plant growth. Soil Biology & Biochemistry 85, 170–182.
| Plant nitrogen uptake drives rhizosphere bacterial community assembly during plant growth.Crossref | GoogleScholarGoogle Scholar |
Burke IC, Lauenroth WK, Vinton MA, Hook PB, Kelly RH, Epstein HE, Aguiar MR, Robles MD, Aguilera MO, Murphy KL (1998) Plant–soil interactions in temperate grasslands. Biogeochemistry 42, 121–143.
| Plant–soil interactions in temperate grasslands.Crossref | GoogleScholarGoogle Scholar |
Calvo P, Watts DB, Kloepper JW, Torbert HA (2017) Effect of microbial-based inoculants on nutrient concentrations and early root morphology of corn (Zea mays). Journal of Plant Nutrition and Soil Science 180, 56–70.
| Effect of microbial-based inoculants on nutrient concentrations and early root morphology of corn (Zea mays).Crossref | GoogleScholarGoogle Scholar |
Canbolat MY, Bilen S, Çakmakçı R, Şahin F, Aydın A (2006) Effect of plant growth-promoting bacteria and soil compaction on barley seedling growth, nutrient uptake, soil properties and rhizosphere microflora. Biology and Fertility of Soils 42, 350–357.
| Effect of plant growth-promoting bacteria and soil compaction on barley seedling growth, nutrient uptake, soil properties and rhizosphere microflora.Crossref | GoogleScholarGoogle Scholar |
Cassman KG (1999) Ecological intensification of cereal production systems: yield potential, soil quality, and precision agriculture. Proceedings of the National Academy of Sciences of the United States of America 96, 5952–5959.
| Ecological intensification of cereal production systems: yield potential, soil quality, and precision agriculture.Crossref | GoogleScholarGoogle Scholar | 10339523PubMed |
Chapman SK, Langley JA, Hart SC, Koch GW (2006) Plants actively control nitrogen cycling: uncorking the microbial bottleneck. New Phytologist 169, 27–34.
| Plants actively control nitrogen cycling: uncorking the microbial bottleneck.Crossref | GoogleScholarGoogle Scholar | 16390416PubMed |
Chen L, Li X, Swoboda GA, Young CA, Sugawara K, Leuchtmann A, Schardl CL (2015) Two distinct Epichloë species symbiotic with Achnatherum inebrians, drunken horse grass. Mycologia 107, 863–873.
Chen N, He RL, Chai Q, Li CJ, Nan ZB (2016) Transcriptomic analyses giving insights into molecular regulation mechanisms involved in cold tolerance by Epichloë endophyte in seed germination of Achnatherum inebrians. Plant Growth Regulation 80, 367–375.
| Transcriptomic analyses giving insights into molecular regulation mechanisms involved in cold tolerance by Epichloë endophyte in seed germination of Achnatherum inebrians.Crossref | GoogleScholarGoogle Scholar |
Christensen MJ, Bennett RJ, Ansari HA, Koga H, Johnson RD, Bryan GT, Simpson WR, Koolaard JP, Nickless EM, Voisey CR (2008) Epichloë endophytes grow by intercalary hyphal extension in elongating grass leaves. Fungal Genetics and Biology 45, 84–93.
| Epichloë endophytes grow by intercalary hyphal extension in elongating grass leaves.Crossref | GoogleScholarGoogle Scholar | 17919950PubMed |
De Deyn GB, Raaijmakers CE, Ruijven JV, Berendse F, Putten WHVD (2004) Plant species identity and diversity effects on different trophic levels of nematodes in the soil food web. Oikos 106, 576–586.
| Plant species identity and diversity effects on different trophic levels of nematodes in the soil food web.Crossref | GoogleScholarGoogle Scholar |
De Freitas JR, Banerjee MR, Germida JJ (1997) Phosphate-solubilizing rhizobacteria enhance the growth and yield but not phosphorus uptake of canola (Brassica napus L.). Biology and Fertility of Soils 24, 358–364.
| Phosphate-solubilizing rhizobacteria enhance the growth and yield but not phosphorus uptake of canola (Brassica napus L.).Crossref | GoogleScholarGoogle Scholar |
Dotaniya ML, Meena VD (2015) Rhizosphere effect on nutrient availability in soil and its uptake by plants: a review. Proceedings of the National Academy of Sciences. India. Section B, Biological Sciences 85, 1–12.
| Rhizosphere effect on nutrient availability in soil and its uptake by plants: a review.Crossref | GoogleScholarGoogle Scholar |
Egamberdiyeva D (2007) The effect of plant growth promoting bacteria on growth and nutrient uptake of maize in two different soils. Applied Soil Ecology 36, 184–189.
| The effect of plant growth promoting bacteria on growth and nutrient uptake of maize in two different soils.Crossref | GoogleScholarGoogle Scholar |
Egamberdiyeva D, Höflich G (2004) Effect of plant growth-promoting bacteria on growth and nutrient uptake of cotton and pea in a semi-arid region of Uzbekistan. Journal of Arid Environments 56, 293–301.
| Effect of plant growth-promoting bacteria on growth and nutrient uptake of cotton and pea in a semi-arid region of Uzbekistan.Crossref | GoogleScholarGoogle Scholar |
Ehrenfeld JG, Ravit B, Elgersma K (2005) Feedback in the plant–soil system. Annual Review of Environment and Resources 30, 75–115.
| Feedback in the plant–soil system.Crossref | GoogleScholarGoogle Scholar |
Elser JJ, Dobberfuhl DR, MacKay NA, Schampel JH (1996) Organism size, life history, and N: P stoichiometry: toward a unified view of cellular and ecosystem processes. Bioscience 46, 674–684.
| Organism size, life history, and N: P stoichiometry: toward a unified view of cellular and ecosystem processes.Crossref | GoogleScholarGoogle Scholar |
Elser JJ, Fagan WF, Kerkhoff AJ, Swenson NG, Enquist BJ (2010) Biological stoichiometry of plant production: metabolism, scaling and ecological response to global change. New Phytologist 186, 593–608.
| Biological stoichiometry of plant production: metabolism, scaling and ecological response to global change.Crossref | GoogleScholarGoogle Scholar | 20298486PubMed |
Fierer N, Ladau J, Clemente JC, Leff JW, Owens SM, Pollard KS, Knight R, Gilbert JA, McCulley RL (2013) Reconstructing the microbial diversity and function of pre-agricultural tallgrass prairie soils in the united states. Science 342, 621–624.
| Reconstructing the microbial diversity and function of pre-agricultural tallgrass prairie soils in the united states.Crossref | GoogleScholarGoogle Scholar | 24179225PubMed |
Franzluebbers AJ, Nazih N, Stuedemann JA, Fuhrmann JJ, Schomberg HH, Hartel PG (1999) Soil carbon and nitrogen pools under low- and high-endophyte-infected tall fescue. Soil Science Society of America Journal 63, 1687–1694.
| Soil carbon and nitrogen pools under low- and high-endophyte-infected tall fescue.Crossref | GoogleScholarGoogle Scholar |
Glick BR (2012) Plant growth-promoting bacteria: mechanisms and applications. Scientifica 2012, 1–15.
| Plant growth-promoting bacteria: mechanisms and applications.Crossref | GoogleScholarGoogle Scholar |
Gong X, Liu C, Li J, Luo Y, Yang Q, Zhang W, Yang P, Feng B (2019) Responses of rhizosphere soil properties, enzyme activities and microbial diversity to intercropping patterns on the Loess Plateau of China. Soil & Tillage Research 195, 104355
| Responses of rhizosphere soil properties, enzyme activities and microbial diversity to intercropping patterns on the Loess Plateau of China.Crossref | GoogleScholarGoogle Scholar |
Govindasamy V, Senthilkumar M, Mageshwaran V, Annapurna K (2009) Detection and characterization of ACC deaminase in plant growth promoting rhizobacteria. Journal of Plant Biochemistry and Biotechnology 18, 71–76.
| Detection and characterization of ACC deaminase in plant growth promoting rhizobacteria.Crossref | GoogleScholarGoogle Scholar |
Grayston SJ, Wang S, Campbell CD, Edwards AC (1998) Selective influence of plant species on microbial diversity in the rhizosphere. Soil Biology & Biochemistry 30, 369–378.
| Selective influence of plant species on microbial diversity in the rhizosphere.Crossref | GoogleScholarGoogle Scholar |
Guo JQ (2014) The influence of tall fescue cultivar and endophyte status on root exudate chemistry and rhizosphere processes. PhD Thesis, University of Kentucky, Lexington, KY, USA.
Höflich G, Wiehe W, Hecht-Buchholz C (1995) Rhizosphere colonization of different crops with growth promoting Pseudomonas and Rhizobium bacteria. Microbiological Research 150, 139–147.
| Rhizosphere colonization of different crops with growth promoting Pseudomonas and Rhizobium bacteria.Crossref | GoogleScholarGoogle Scholar |
Horwath WR, Elliott LF, Lynch JM (1998) Influence of soil quality on the function of inhibitory rhizobacteria. Letters in Applied Microbiology 26, 87–92.
| Influence of soil quality on the function of inhibitory rhizobacteria.Crossref | GoogleScholarGoogle Scholar |
Hou WP, Wang JF, Nan ZB, Christensen MJ, Xia C, Chen T, Zhang ZX, Niu XL (2020) Epichloë gansuensis endophyte-infection alters soil enzymes activity and soil nutrients at different growth stages of Achnatherum inebrians. Plant and Soil
| Epichloë gansuensis endophyte-infection alters soil enzymes activity and soil nutrients at different growth stages of Achnatherum inebrians.Crossref | GoogleScholarGoogle Scholar |
Houlden A, Timmswilson TM, Day MJ, Bailey MJ (2008) Influence of plant developmental stage on microbial community structure and activity in the rhizosphere of three field crops. FEMS Microbiology Ecology 65, 193–201.
| Influence of plant developmental stage on microbial community structure and activity in the rhizosphere of three field crops.Crossref | GoogleScholarGoogle Scholar | 18616582PubMed |
Huitu O, Forbes KM, Helander M, Julkunen-Tiitto R, Lambin X, Saikkonen K, Stuart P, Sulkama S, Hartley S (2014) Silicon, endophytes and secondary metabolites as grass defenses against mammalian herbivores. Frontiers in Plant Science 5, 478
| Silicon, endophytes and secondary metabolites as grass defenses against mammalian herbivores.Crossref | GoogleScholarGoogle Scholar | 25278951PubMed |
Ibekwe AM, Kennedy AC (1998) Phospholipid fatty acid profiles and carbon utilization patterns for analysis of microbial community structure under field and greenhouse conditions. FEMS Microbiology Ecology 26, 151–163.
| Phospholipid fatty acid profiles and carbon utilization patterns for analysis of microbial community structure under field and greenhouse conditions.Crossref | GoogleScholarGoogle Scholar |
Inceoglu O, Salles JF, van Overbeek L, van Elsas JD (2010) Effects of plant genotype and growth stage on the betaproteobacterial communities associated with different potato cultivars in two fields. Applied and Environmental Microbiology 76, 3675–3684.
| Effects of plant genotype and growth stage on the betaproteobacterial communities associated with different potato cultivars in two fields.Crossref | GoogleScholarGoogle Scholar | 20363788PubMed |
Innes L, Hobbs PJ, Bardgett RD (2004) The impacts of individual plant species on rhizosphere microbial communities in soils of different fertility. Biology and Fertility of Soils 40, 7–13.
| The impacts of individual plant species on rhizosphere microbial communities in soils of different fertility.Crossref | GoogleScholarGoogle Scholar |
Ju YW, Zhong R, Christensen MJ, Zhang XX (2020) Effects of Epichloë gansuensis endophyte on the root and rhizosphere soil bacteria of Achnatherum inebrians under different moisture conditions. Frontiers in Microbiology 11,
| Effects of Epichloë gansuensis endophyte on the root and rhizosphere soil bacteria of Achnatherum inebrians under different moisture conditions.Crossref | GoogleScholarGoogle Scholar |
Kennedy N, Connolly J, Clipson N (2005) Impact of lime, nitrogen and plant species on fungal community structure in grassland microcosms. Environmental Microbiology 7, 780–788.
| Impact of lime, nitrogen and plant species on fungal community structure in grassland microcosms.Crossref | GoogleScholarGoogle Scholar | 15892697PubMed |
Klironomos JN (2002) Feedback with soil biota contributes to plant rarity and invasiveness in communities. Nature 417, 67–70.
| Feedback with soil biota contributes to plant rarity and invasiveness in communities.Crossref | GoogleScholarGoogle Scholar | 11986666PubMed |
Koerselman W, Meuleman AF (1996) The vegetation N:P ratio: a new tool to detect the nature of nutrient limitation. Journal of Applied Ecology 33, 1441–1450.
| The vegetation N:P ratio: a new tool to detect the nature of nutrient limitation.Crossref | GoogleScholarGoogle Scholar |
Kourtev PS, Ehrenfeld JG, Häggblom M (2002) Exotic plant species alter the microbial community structure and function in the soil. Ecology 83, 3152–3166.
| Exotic plant species alter the microbial community structure and function in the soil.Crossref | GoogleScholarGoogle Scholar |
Latour X, Philippot L, Corberand T, Lemanceau P (1999) The establishment of an introduced community of fluorescent pseudomonads in the soil and in the rhizosphere is affected by the soil type. FEMS Microbiology Ecology 30, 163–170.
| The establishment of an introduced community of fluorescent pseudomonads in the soil and in the rhizosphere is affected by the soil type.Crossref | GoogleScholarGoogle Scholar | 10508941PubMed |
Li X (2017) Study on the evolution and interactions of Epichloë gansuensis with host seed-borne fungi and rhizospheric microorganism. PhD Thesis, Lanzhou University, Lanzhou, Gansu, China.
Li CJ, Nan ZB, Paul VH, Dapprich PD, Liu Y (2004a) A new Neotyphodium species symbiotic with drunken horse grass (Achnatherum inebrians) in China. Mycotaxon 90, 141–147.
Li CJ, Nan ZB, Gao JH, Tian P (2004b) Detection and distribution of Neotyphodium–Achnatherum inebrians association in China. In ‘Proceedings of 5th International Neotyphodium/Grass Interactions Symposium’. 23–26 May, 2004 Fayetteville, AR, USA. (Eds R Kallenbach, C Rosenkrans, T Ryan Loch)
Li CJ, Zhang XX, Li F, Nan ZB, Schardl CL (2007) Disease and pests resistance of endophyte infected and non-infected drunken horse grass. In ‘Proceedings of the 6th International Symposium on Fungal Endophytes of Grasses’. 25–28 March 2007, Christchurch, New Zealand, (Eds AJ Popay, ER Thom) (New Zealand Grassland Association: Dunedin, New Zealand)
Li X, Ren A, Han R, Yin LJ, Wei MY, Gao YB (2012) Endophyte-mediated effects on the growth and physiology of Achnatherum sibiricum are conditional on both N and P availability. PLoS One 7, e48010
| Endophyte-mediated effects on the growth and physiology of Achnatherum sibiricum are conditional on both N and P availability.Crossref | GoogleScholarGoogle Scholar | 23300928PubMed |
Li NN, Zhao YF, Xia C, Zhong R, Zhang XX (2016) Effects of thiophanate methyl on seed borne Epichloë fungal endophyte of Achnatherum inebrians. Pratacultural Science 33, 1306–1314.
Liu Q, Parsons AJ, Xue H, Fraser K, Ryan GD, Newman JA, Rasmussen S (2011) Competition between foliar Neotyphodium lolii endophytes and mycorrhizal Glomus spp. fungi in Lolium perenne depends on resource supply and host carbohydrate content. Functional Ecology 25, 910–920.
| Competition between foliar Neotyphodium lolii endophytes and mycorrhizal Glomus spp. fungi in Lolium perenne depends on resource supply and host carbohydrate content.Crossref | GoogleScholarGoogle Scholar |
Lupwayi NZ, Rice WA, Clayton GW (1998) Soil microbial diversity and community structure under wheat as influenced by tillage and crop rotation. Soil Biology & Biochemistry 30, 1733–1741.
| Soil microbial diversity and community structure under wheat as influenced by tillage and crop rotation.Crossref | GoogleScholarGoogle Scholar |
Lyons PC, Evans JJ, Bacon CW (1990) Effects of the fungal endophyte Acremonium coenophialum on nitrogen accumulation and metabolism in tall fescue. Plant Physiology 92, 726–732.
| Effects of the fungal endophyte Acremonium coenophialum on nitrogen accumulation and metabolism in tall fescue.Crossref | GoogleScholarGoogle Scholar | 16667341PubMed |
Maarastawi SA, Frindte K, Linnartz M, Knief C (2018) Crop rotation and straw application impact microbial communities in Italian and Philippine soils and the rhizosphere of Zea mays. Frontiers in Microbiology 9, 1295
| Crop rotation and straw application impact microbial communities in Italian and Philippine soils and the rhizosphere of Zea mays.Crossref | GoogleScholarGoogle Scholar | 29963033PubMed |
Main TM, Dobberfuhl DR, Elser JJ (1997) N:P stoichiometry and ontogeny of crustacean zooplankton: a test of the growth rate hypothesis. Limnology and Oceanography 42, 1474–1478.
| N:P stoichiometry and ontogeny of crustacean zooplankton: a test of the growth rate hypothesis.Crossref | GoogleScholarGoogle Scholar |
Malinowski D, Brauer D, Belesky D (1999) The endophyte Neotyphodium coenophialum affects root morphology of tall fescue grown under phosphorus deficiency. Journal Agronomy & Crop Science 183, 53–60.
| The endophyte Neotyphodium coenophialum affects root morphology of tall fescue grown under phosphorus deficiency.Crossref | GoogleScholarGoogle Scholar |
Malinowski DP, Alloush GA, Belesky DP (2000) Leaf endophyte Neotyphodium coenophialum modifies mineral uptake in tall fescue. Plant and Soil 227, 115–126.
| Leaf endophyte Neotyphodium coenophialum modifies mineral uptake in tall fescue.Crossref | GoogleScholarGoogle Scholar |
Man Y, Wang JX, Tam NF, Wan X, Huang WD, Zheng Y, Tang JP, Tao R, Yang Y (2020) Responses of rhizosphere and bulk substrate microbiome to wastewater-borne sulfonamides in constructed wetlands with different plant species. The Science of the Total Environment 706, 135955
| Responses of rhizosphere and bulk substrate microbiome to wastewater-borne sulfonamides in constructed wetlands with different plant species.Crossref | GoogleScholarGoogle Scholar | 31855648PubMed |
Marschner P (2012) ‘Marschner’s mineral nutrition of higher plants.’ 2nd edn. (Academic Press: Cambridge, MA, USA)
Marschner P, Crowley D, Yang CH (2004) Development of specific rhizosphere bacterial communities in relation to plant species, nutrition and soil type. Plant and Soil 261, 199–208.
| Development of specific rhizosphere bacterial communities in relation to plant species, nutrition and soil type.Crossref | GoogleScholarGoogle Scholar |
Meena VS, Meena SK, Verma JP, Kumar A, Aeron A, Mishra PK, Bisht JK, Pattanayak A, Naveed M, Dotaniya ML (2017) Plant beneficial rhizospheric microorganism (PBRM) strategies to improve nutrients use efficiency: a review. Ecological Engineering 107, 8–32.
| Plant beneficial rhizospheric microorganism (PBRM) strategies to improve nutrients use efficiency: a review.Crossref | GoogleScholarGoogle Scholar |
Miller HJ, Henken G, van Veen JA (1989) Variation and composition of bacterial populations in the rhizospheres of maize, wheat, and grass cultivars. Canadian Journal of Microbiology 35, 656–660.
| Variation and composition of bacterial populations in the rhizospheres of maize, wheat, and grass cultivars.Crossref | GoogleScholarGoogle Scholar |
Miransari M (2011) Arbuscular mycorrhizal fungi and nitrogen uptake. Archives of Microbiology 193, 77–81.
| Arbuscular mycorrhizal fungi and nitrogen uptake.Crossref | GoogleScholarGoogle Scholar | 21136040PubMed |
Müller CB, Krauss J (2005) Symbiosis between grasses and asexual fungal endophytes. Current Opinion in Plant Biology 8, 450–456.
| Symbiosis between grasses and asexual fungal endophytes.Crossref | GoogleScholarGoogle Scholar | 15946893PubMed |
Nan ZB, Li CJ (2004) Roles of the grass–Neotyphodium association in pastoral agriculture systems. Acta Ecologica Sinica 24, 605–616.
Nihorimbere V, Ongena M, Smargiassi M, Thonart P (2011) Beneficial effect of the rhizosphere microbial community for plant growth and health. Biotechnologie, Agronomie, Société et Environnement 15, 327–337.
Niklas KJ, Cobb ED (2006) Biomass partitioning and leaf N, P-stoichiometry: comparisons between tree and herbaceous current-year shoots. Plant, Cell & Environment 29, 2030–2042.
| Biomass partitioning and leaf N, P-stoichiometry: comparisons between tree and herbaceous current-year shoots.Crossref | GoogleScholarGoogle Scholar |
Ollivier J, Töwe S, Bannert A, Hai B, Kastl EM, Meyer A, Su MX, Kleineidam K, Schloter M (2011) Nitrogen turnover in soil and global change. FEMS Microbiology Ecology 78, 3–16.
| Nitrogen turnover in soil and global change.Crossref | GoogleScholarGoogle Scholar | 21707675PubMed |
Pii Y, Mimmo T, Tomasi N, Terzano R, Cesco S, Crecchio C (2015) Microbial interactions in the rhizosphere: beneficial influences of plant growth-promoting rhizobacteria on nutrient acquisition process. A review. Biology and Fertility of Soils 51, 403–415.
| Microbial interactions in the rhizosphere: beneficial influences of plant growth-promoting rhizobacteria on nutrient acquisition process. A review.Crossref | GoogleScholarGoogle Scholar |
Qiao QH, Wang FR, Zhang JX, Chen Y, Zhang CY, Liu GD, Zhang H, Ma CL, Zhang J (2017) The variation in the rhizosphere microbiome of cotton with soil type, genotype and developmental stage. Scientific Reports 7, 3940
| The variation in the rhizosphere microbiome of cotton with soil type, genotype and developmental stage.Crossref | GoogleScholarGoogle Scholar |
Reed SC, Townsend AR, Davidson EA, Cleveland CC (2012) Stoichiometric patterns in foliar nutrient resorption across multiple scales. New Phytologist 196, 173–180.
| Stoichiometric patterns in foliar nutrient resorption across multiple scales.Crossref | GoogleScholarGoogle Scholar | 22882279PubMed |
Reef R, Ball MC, Feller IC, Lovelock CE (2010) Relationships among RNA:DNA ratio, growth and elemental stoichiometry in mangrove trees. Functional Ecology 24, 1064–1072.
| Relationships among RNA:DNA ratio, growth and elemental stoichiometry in mangrove trees.Crossref | GoogleScholarGoogle Scholar |
Reza SM, Mirlohi A (2010) Neotyphodium endophytes trigger salt resistance in tall and meadow fescues. Journal of Plant Nutrition and Soil Science 173, 952–957.
| Neotyphodium endophytes trigger salt resistance in tall and meadow fescues.Crossref | GoogleScholarGoogle Scholar |
Richardson MD, Cabrera RI, Murphy JA, Zaurov DE (1999) Nitrogen‐form and endophyte‐infection effects on growth, nitrogen uptake, and alkaloid content of chewings fescue turf grass. Journal of Plant Nutrition 22, 67–79.
| Nitrogen‐form and endophyte‐infection effects on growth, nitrogen uptake, and alkaloid content of chewings fescue turf grass.Crossref | GoogleScholarGoogle Scholar |
Rivas-Ubach A, Sardans J, Pérez-Trujillo M, Estiarte M, Peñuelas J (2012) Strong relationship between elemental stoichiometry and metabolome in plants. Proceedings of the National Academy of Sciences of the United States of America 109, 4181–4186.
| Strong relationship between elemental stoichiometry and metabolome in plants.Crossref | GoogleScholarGoogle Scholar | 22371578PubMed |
Roesti D, Gaur R, Johri BN, Imfeld G, Sharma S, Kawaljeet K, Aragno M (2006) Plant growth stage, fertiliser management and bio-inoculation of arbuscular mycorrhizal fungi and plant growth promoting rhizobacteria affect the rhizobacterial community structure in rain-fed wheat fields. Soil Biology & Biochemistry 38, 1111–1120.
| Plant growth stage, fertiliser management and bio-inoculation of arbuscular mycorrhizal fungi and plant growth promoting rhizobacteria affect the rhizobacterial community structure in rain-fed wheat fields.Crossref | GoogleScholarGoogle Scholar |
Rojas X, Guo J, Leff JW, McNear DH, Fierer N, McCulley RL (2016) Infection with a shoot-specific fungal endophyte (Epichloë) alters tall fescue soil microbial communities. Microbial Ecology 72, 197–206.
| Infection with a shoot-specific fungal endophyte (Epichloë) alters tall fescue soil microbial communities.Crossref | GoogleScholarGoogle Scholar | 26992401PubMed |
Ryu CM, Hu CH, Locy RD, Kloepper JW (2005) Study of mechanisms for plant growth promotion elicited by rhizobacteria in Arabidopsis thaliana. Plant and Soil 268, 285–292.
| Study of mechanisms for plant growth promotion elicited by rhizobacteria in Arabidopsis thaliana.Crossref | GoogleScholarGoogle Scholar |
Schardl CL, Leuchtmann A, Spiering MJ (2004) Symbioses of grasses with seedborne fungal endophytes. Annual Review of Plant Biology 55, 315–340.
| Symbioses of grasses with seedborne fungal endophytes.Crossref | GoogleScholarGoogle Scholar | 15377223PubMed |
Schlatter DC, Hansen JC, Schillinger WF, Sullivan TS, Paulitz TC (2019) Common and unique rhizosphere microbial communities of wheat and canola in a semiarid Mediterranean environment. Applied Soil Ecology 144, 170–181.
| Common and unique rhizosphere microbial communities of wheat and canola in a semiarid Mediterranean environment.Crossref | GoogleScholarGoogle Scholar |
Song ML, Chai Q, Li XZ, Yao X, Li CJ, Christensen MJ, Nan ZB (2015a) An asexual Epichloë endophyte modifies the nutrient stoichiometry of wild barley (Hordeum brevisubulatum) under salt stress. Plant and Soil 387, 153–165.
| An asexual Epichloë endophyte modifies the nutrient stoichiometry of wild barley (Hordeum brevisubulatum) under salt stress.Crossref | GoogleScholarGoogle Scholar |
Song ML, Li X, Saikkonen K, Li CJ, Nan ZB (2015b) An asexual Epichloë endophyte enhances waterlogging tolerance of Hordeum brevisubulatum. Fungal Ecology 13, 44–52.
| An asexual Epichloë endophyte enhances waterlogging tolerance of Hordeum brevisubulatum.Crossref | GoogleScholarGoogle Scholar |
Sparks D, Bartels J (1996) Chemical methods. In ‘Methods of soil analysis’. (Soil Science Society of America: Madison, WI, USA)
Sterner RW, Elser JJ (2002) Ecological stoichiometry: the biology of elements from molecules to the biosphere. Journal of Plankton Research 9, 9
Su YY, Guo LD, Hyde KD (2010) Response of endophytic fungi of stipa grandis to experimental plant function group removal in Inner Mongolia steppe, China. Fungal Diversity 43, 93–101.
| Response of endophytic fungi of stipa grandis to experimental plant function group removal in Inner Mongolia steppe, China.Crossref | GoogleScholarGoogle Scholar |
Tian ZP, Wang RY, Ambrose KV, Clarke BB, Belanger FC (2017) The Epichloë festucae antifungal protein has activity against the plant pathogen Sclerotinia homoeocarpa, the causal agent of dollar spot disease. Scientific Reports 7, 5643
| The Epichloë festucae antifungal protein has activity against the plant pathogen Sclerotinia homoeocarpa, the causal agent of dollar spot disease.Crossref | GoogleScholarGoogle Scholar |
Tilman D, Cassman KG, Matson PA, Naylor R, Polasky S (2002) Agricultural sustainability and intensive production practices. Nature 418, 671
| Agricultural sustainability and intensive production practices.Crossref | GoogleScholarGoogle Scholar | 12167873PubMed |
Vandenkoornhuyse P, Ridgway KP, Watson IJ, Fitter AH, Young JP (2003) Co-existing grass species have distinctive arbuscular mycorrhizal communities. Molecular Ecology 12, 3085–3095.
| Co-existing grass species have distinctive arbuscular mycorrhizal communities.Crossref | GoogleScholarGoogle Scholar | 14629388PubMed |
Wang JF, Zhang YQ, Li Y, Wang XM, Nan WB, Hu YF, Zhang H, Zhao CZ, Wang F, Li P, Shi HY, Bi YR (2015) Endophytic microbes Bacillus sp. LZR216-regulated root development is dependent on polar auxin transport in Arabidopsis seedlings. Plant Cell Reports 34, 1075–1087.
| Endophytic microbes Bacillus sp. LZR216-regulated root development is dependent on polar auxin transport in Arabidopsis seedlings.Crossref | GoogleScholarGoogle Scholar |
Wang JF, Nan ZB, Christensen MJ, Li CJ (2018a) Glucose-6-phosphate dehydrogenase plays a vital role in Achnatherum inebrians plants host to Epichloë gansuensis by improving growth under nitrogen deficiency. Plant and Soil 430, 37–48.
| Glucose-6-phosphate dehydrogenase plays a vital role in Achnatherum inebrians plants host to Epichloë gansuensis by improving growth under nitrogen deficiency.Crossref | GoogleScholarGoogle Scholar |
Wang JF, Nan ZB, Christensen MJ, Zhang XX, Tian P, Zhang ZX, Niu XL, Gao P, Chen T, Ma LX (2018b) Effect of Epichloë gansuensis endophyte on the nitrogen metabolism, nitrogen use efficiency, and stoichiometry of Achnatherum inebrians under nitrogen limitation. Journal of Agricultural and Food Chemistry 66, 4022–4031.
| Effect of Epichloë gansuensis endophyte on the nitrogen metabolism, nitrogen use efficiency, and stoichiometry of Achnatherum inebrians under nitrogen limitation.Crossref | GoogleScholarGoogle Scholar |
Wang JF, Tian P, Christensen MJ, Zhang XX, Li CJ, Nan ZB (2019) Effect of Epichloë gansuensis endophyte on the activity of enzymes of nitrogen metabolism, nitrogen use efficiency and photosynthetic ability of Achnatherum inebrians under various NaCl concentrations. Plant and Soil 435, 57–68.
| Effect of Epichloë gansuensis endophyte on the activity of enzymes of nitrogen metabolism, nitrogen use efficiency and photosynthetic ability of Achnatherum inebrians under various NaCl concentrations.Crossref | GoogleScholarGoogle Scholar |
Wang JF, Hou WP, Christensen MJ, Xia C, Chen T, Zhang ZX, Nan ZB (2020a) The fungal endophyte Epichloë gansuensis increases NaCl-tolerance in Achnatherum inebrians through enhancing the activity of plasma membrane H+-ATPase and glucose-6-phosphate dehydrogenase. Science China. Life Sciences
| The fungal endophyte Epichloë gansuensis increases NaCl-tolerance in Achnatherum inebrians through enhancing the activity of plasma membrane H+-ATPase and glucose-6-phosphate dehydrogenase.Crossref | GoogleScholarGoogle Scholar |
Wang JJ, Li RC, Zhang H, Wei GH, Li ZF (2020b) Beneficial bacteria activate nutrients and promote wheat growth under conditions of reduced fertilizer application. BMC Microbiology 20, 38
| Beneficial bacteria activate nutrients and promote wheat growth under conditions of reduced fertilizer application.Crossref | GoogleScholarGoogle Scholar |
Wang JF, Hou WP, Christensen MJ, Li XZ, Xia C, Li CJ, Nan ZB (2020c) Role of Epichloë endophytes in improving host grass resistance ability and soil properties. Journal of Agricultural and Food Chemistry 68, 6944–6955.
| Role of Epichloë endophytes in improving host grass resistance ability and soil properties.Crossref | GoogleScholarGoogle Scholar |
Wardle DA, Bonner KI, Barker GM, Yeates GW, Nicholson KS, Bardgett RD, Watson RN, Ghani A (1999) Plant removals in perennial grassland: vegetation dynamics, decomposers, soil biodiversity, and ecosystem properties. Ecological Monographs 69, 535–568.
| Plant removals in perennial grassland: vegetation dynamics, decomposers, soil biodiversity, and ecosystem properties.Crossref | GoogleScholarGoogle Scholar |
Westover KM, Kennedy AC, Kelley SE (1997) Patterns of rhizosphere microbial community structure associated with co-occurring plant species. Journal of Ecology 85, 863–873.
| Patterns of rhizosphere microbial community structure associated with co-occurring plant species.Crossref | GoogleScholarGoogle Scholar |
White RH, Engelke MC, Morton SJ, Johnson-Cicalese JM, Ruemmele BA (1992) Acremonium endophyte effects on tall fescue drought tolerance. Crop Science 32, 1392–1396.
| Acremonium endophyte effects on tall fescue drought tolerance.Crossref | GoogleScholarGoogle Scholar |
Xia C (2018) Responses of Epichloë gansuensis-Achnatherum inebrians symbiont to drought stress. PhD thesis, Lanzhou University, Lanzhou, China.
Xia C, Christensen MJ, Zhang XX, Nan ZB (2018) Effect of Epichloë gansuensis endophyte and transgenerational effects on the water use efficiency, nutrient and biomass accumulation of Achnatherum inebrians under soil water deficit. Plant and Soil 424, 555–571.
| Effect of Epichloë gansuensis endophyte and transgenerational effects on the water use efficiency, nutrient and biomass accumulation of Achnatherum inebrians under soil water deficit.Crossref | GoogleScholarGoogle Scholar |
Yang JW (2012) Study on soil microbial quantity, soil enzyme activity and physicochemical factor in various saline-alkali soil in Hexi Corridor. Master’s thesis, Northwest Normal University, Lanzhou, China.
Yang YH, Luo YQ (2011) Carbon: nitrogen stoichiometry in forest ecosystems during stand development. Global Ecology and Biogeography 20, 354–361.
| Carbon: nitrogen stoichiometry in forest ecosystems during stand development.Crossref | GoogleScholarGoogle Scholar |
Yildirim E, Karlidag H, Turan M, Dursun A, Goktepe F (2011) Growth, nutrient uptake, and yield promotion of broccoli by plant growth promoting rhizobacteria with manure. HortScience 46, 932–936.
Zhang XB (2008) Study on microflora and characteristics of plant growth promoting rhizobacteria (PGPR) in rhizosphere of Kentucky bluegrass. PhD thesis, Beijing Forestry University, Beijing.
Zhang FS, Shen JB, Zhang JY, Zuo N, Li L, Chen XP (2010a) Rhizosphere processes and management for improving nutrient use efficiency and crop productivity: implications for China. Advances in Agronomy 107, 1–32.
| Rhizosphere processes and management for improving nutrient use efficiency and crop productivity: implications for China.Crossref | GoogleScholarGoogle Scholar |
Zhang XX, Li CJ, Nan ZB (2010b) Effects of cadmium stress on growth and anti-oxidative systems in Achnatherum inebrians symbiotic with Neotyphodium gansuense. Journal of Hazardous Materials 175, 703–709.
| Effects of cadmium stress on growth and anti-oxidative systems in Achnatherum inebrians symbiotic with Neotyphodium gansuense.Crossref | GoogleScholarGoogle Scholar |
Zhang XX, Li CJ, Nan ZB, Matthew C (2012) Neotyphodium endophyte increases Achnatherum inebrians (drunken horse grass) resistance to herbivores and seed predators. Weed Research 52, 70–78.
| Neotyphodium endophyte increases Achnatherum inebrians (drunken horse grass) resistance to herbivores and seed predators.Crossref | GoogleScholarGoogle Scholar |
Zhong R, Xia C, Ju YW, Li NN, Zhang XX, Nan ZB, Christensen MJ (2018) Effects of Epichloë gansuensis on root-associated fungal communities of Achnatherum inebrians under different growth conditions. Fungal Ecology 31, 29–36.
| Effects of Epichloë gansuensis on root-associated fungal communities of Achnatherum inebrians under different growth conditions.Crossref | GoogleScholarGoogle Scholar |
Zhou Y, Li X, Qin JH, Liu H, Chen W, Niu Y, Ren AZ, Gao YB (2016) Effects of simultaneous infections of endophytic fungi and arbuscular mycorrhizal fungi on the growth of their shared host grass Achnatherum sibiricum under varying N and P supply. Fungal Ecology 20, 56–65.
| Effects of simultaneous infections of endophytic fungi and arbuscular mycorrhizal fungi on the growth of their shared host grass Achnatherum sibiricum under varying N and P supply.Crossref | GoogleScholarGoogle Scholar |
Ziegler M, Engel M, Welzl G, Schloter M (2013) Development of a simple root model to study the effects of single exudates on the development of bacterial community structure. Journal of Microbiological Methods 94, 30–36.
| Development of a simple root model to study the effects of single exudates on the development of bacterial community structure.Crossref | GoogleScholarGoogle Scholar | 23611840PubMed |