Expanding on the plecstatin anticancer agent class: exchange of the chlorido ligand for N-heterocyclic ligands
Saawan Kumar A B , Mie Riisom A C , Stephen M. F. Jamieson C , Tilo Söhnel A , Suresh Bhargava D , Jing Sun E and Christian G. Hartinger
A
B
C
D
E
Abstract
Metal piano-stool complexes based on pyridinecarbothioamide (PCA) have shown promising antiproliferative and in vivo anticancer activity, in particular [Ru(cym)(p-F-PCA)Cl]PF6 (cym is η6-p-cymene; plecstatin-1). The impact of modifications of the PCA and π-bound ligands on biological properties has been extensively investigated. Herein, we explored the influence of exchanging the chlorido ligand with the N-heterocycles 1-methylimidazole, 1-methylbenzimidazole and pyridine. In solution, an equilibrium between the protonated and deprotonated forms of the thioamide bond was observed, which was found dictated by the solvent system with both species detected in polar solvents. [Ru(cym)(PCA)Cl]+ complexes exhibit unique behaviour in an aqueous environment where they rapidly form dimeric species after substitution of the chlorido ligand for the sulfur donor of the PCA ligand of a second complex molecule. This was also observed for the synthesised complexes with the N-heterocyclic ligands being cleaved from the Ru centre allowing for dimerisation, which may be reversed by acidification of the solution resulting in the formation of equivalent mononuclear compounds. This behaviour explains the similar biological properties of the complexes with respect to that of plecstatin-1.
Keywords: anticancer compounds, antiproliferative activity, bioinorganic chemistry, bioorganometallic chemistry, dimerisation, ligand exchange reactions, piano-stool compounds, plecstatin-1, pyridinecarbothioamide complexes, synthesis.
Introduction
Piano-stool metal complexes with the general formula [M(π-bound ligand)(X)(Y)(Z)]+ have been investigated as anticancer agents due to the scaffold being highly modifiable to enhance and tailor the biological and chemical properties.1–5 This is exemplified by the different biological activity of the Ru complexes [Ru(arene)(en)Cl]+ (RAED; where en is 1,2-ethylenediamine) and [Ru(arene)(PTA)Cl2] (RAPTA; where PTA is 1,3,5-triaza-7-phophaadamantane).1,5–7 The RAED complexes exhibit antiproliferative activity and were found to bind to DNA,1,6,8 whereas the RAPTA compounds are inactive in in vitro anticancer activity assays but show antimetastatic properties and bind preferentially to proteins over DNA.5,7,9–14 Consequently, a large number of compounds based on these scaffolds have been reported, often with bioactive ligands coordinated to the metal centres.15–19
Pyridinecarbothioamides (PCAs) are a class of bioactive molecules,20,21 and PCA-based organometallics of the general formula [M(L)(PCA)X]n+ (where M is Ru, Os, Rh, Ir; L is cym [η6-p-cymene], biphenyl, Cp* [Cp* is η5-pentamethylcyclopentadienyl]; X is Cl, Br, I, PTA, PPh3) exhibited potent antiproliferative activity, showed activity in in vivo assays and displayed unique chemical reactivity.18,21–28 PCA complexes demonstrated low reactivity to amino acids such as L-cysteine and L-histidine and high stability in acidic solutions. In particular, the high stability under acidic conditions may make them suitable as orally administered anticancer agents.22 We investigated a wide range of related derivatives and functionalised the phenyl group of PCAs, exchanged the chlorido ligand with bromido and iodido ligands, substituted cym for other arenes and replaced the Ru(cym) moiety with Rh(Cp*) and Ir(Cp*) groups.19 The Ru complexes were highly potent in various cancer cell lines with the p-fluoro-substituted derivative (Scheme 1) being the most active.29 The numerous binding interactions that metal complexes can have in biological systems30,31 makes it challenging to determine their key biological target.32 Through pull-down studies, the biological target of [Ru(cym)(p-F-PCA)Cl]Cl (Scheme 1) was identified as plectin, a key protein involved in the cytoskeletal framework of cells, and the compound was termed plecstatin-1.29,32 In an attempt to improve the delivery of such compounds, we also investigated plecstatin-1 as a payload in peptide–drug conjugates through arene functionalisation,33 while substitution of the chlorido ligand could provide another means of derivatisation in the future.34,35
Synthetic routes A and B to coordinate N-heterocycles to plecstatin-1 to give complexes 4‒6. Counterion X is OTf− or PF6− depending on if pathway A or B was used respectively.
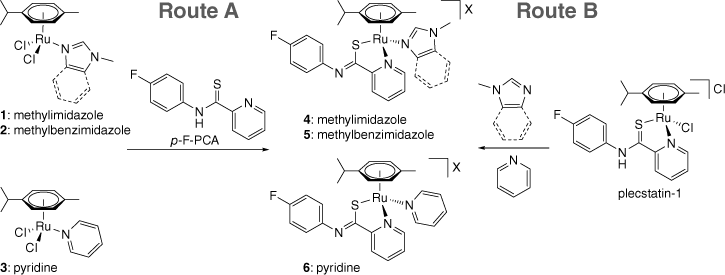
Monodentately coordinated labile ligands in metal complexes can be replaced with aqua ligands in biological environments, which facilitates covalent interactions with their targets. Although in some cases, altering this ligand can reduce the activity of compounds,2,36–38 in other cases the substitution for more lipophilic ligands improves the biological activity.37 In the case of the PCA complexes, the chlorido ligand of the p-Cl-PCA analogue of plecstatin-1 was exchanged with the phosphines PTA and triphenylphosphine to examine the influence of hydrophilic and lipophilic ligands on the biological performance of PCA complexes. Interestingly, the triphenylphosphine derivatives were more active than the PTA derivatives and plecstatin-1 in the cancer cell lines tested.28 Consequently, we explore here the impact of substituting the monodentate chlorido ligand of plecstatin-1 with N-methylimidazole, N-methylbenzimidazole and pyridine on its chemical and biological properties.
Results and discussion
In order to substitute the chlorido ligand of plecstatin-1 [Ru(cym)(p-F-PCA)Cl]Cl with N-heterocycles, two pathways were explored (Scheme 1). In route A, we aimed to exploit the strong interaction between the bidentate PCA ligand with metal centres to introduce p-F-PCA by substituting the two chlorido ligands in [Ru(cym)(N-heterocycle)Cl2] precursors 1–3. For this purpose, the Ru precursor complexes 1–3 were treated with AgOTf in acetonitrile to replace the chlorido ligands with solvent molecules. It was anticipated that this would allow facile coordination of p-F-PCA to the Ru centre to give the target complexes 4–6. Conversely, route B was based on the chlorido ligand of plecstatin-1 being replaced in a methanol and water solution with the N-heterocycle, followed by the addition of NH4PF6 to induce precipitation of 4–6 from the solution. Unlike in the former pathway, approach B avoids the use of Ag salts such as Ag2O or AgX (X is PF6−, OTf−) that may compete with the Ru centres in the precursors for ligands, further complicating the reaction and requiring additional purification steps.
Both synthetic methods gave the products in similar yields of 60–80%, but the synthesis by pathway B proved indeed to be more facile. The approach requires a single metal complex as a precursor rather than the preparation of 1–3. Furthermore, the products precipitated from the aqueous solution, simplifying purification to just filtration and washing with diethyl ether.
Inspection of the 1H NMR spectra of complexes 4–6 in polar solvents such as d6-acetone or d4-methanol (for the NMR spectra see Supplementary Fig. S1–S9) revealed two sets of signals that suggested the formation of two species. However, only one species was observed when the 1H NMR spectrum was collected in CDCl3. Metal piano-stool complexes bearing the PCA ligand were reported with the thioamide group in protonated and deprotonated forms with the ligand being either charge neutral to anionic respectively.22,24,25,27,28 In the case of complexes 4–6 without an anionic co-ligand, this results in the overall charge of the complex being either +1 or +2. The 1H NMR spectra for complexes 4–6 in CDCl3 (Fig. 1) showed downfield shifts of protons adjacent to the coordinating nitrogen atom, indicating their coordination to the Ru centre due to π-back donation effects. In addition, the 1H–1H nuclear Overhauser effect spectroscopy (NOESY) spectra showed nuclear Overhauser effect (NOE) correlations between the cym and PCA ligand protons and the N-heterocycle, providing further evidence of their coordination (Supplementary Fig. S3, S6 and S9). The aromatic cym protons of 5 and 6 became more chemically distinct as the size of the N-heterocyclic ligand increased, as evident by the larger separation of the four doublets between 5.5 and 6.2 ppm (Fig. 1). This contrasts with 4 for which two of the aromatic proton doublets overlapped to form a multiplet. The pseudo-tetrahedral geometry of the complex results in the protons becoming increasingly more chemically distinct with larger N-heterocyclic ligands. This also extended to the methyl groups on the isopropyl substituent of cym, with the spectra of 5 and 6 displaying them as two doublets whereas in that of 4 they were observed as a triplet due to the overlap of two doublets.
1H NMR spectra of 4‒6 in CDCl3 showing the downfield shifts of signals assigned to the coordinated N-heterocycle relative to spectra collected for the heterocyclic compounds. The arrows indicate the extent of the downfield shift of the highlighted protons relative to the spectra of free N-heterocycles.
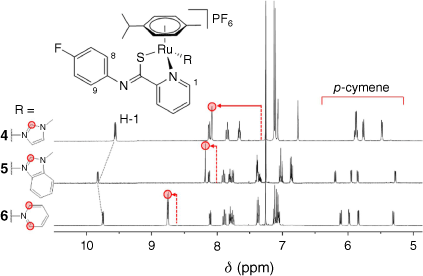
The complexes showed all the expected signals in the 13C{1H} NMR spectra, with the PCA and p-cymene carbon atoms detected with similar chemical shifts. The carbon atoms adjacent to the coordinating N-heterocycle shifted downfield relative to the uncoordinated compound. Exchanging the chlorido ligand with the larger N-heterocyclic ligand, the chemical environment of the carbon atoms on the N-fluorophenyl ring became inequivalent, resulting in C-8 and C-9 being observed in the 13C{1H} NMR spectrum as two signals that were 0.2 ppm apart.
To confirm the coordination of the N-heterocyclic ligands to plecstatin-1, complexes 4–6 were analysed by mass spectrometry. In all cases, the electrospray ionisation (ESI)-mass spectra feature the [M – N-heterocycle]+ ion as the N-heterocycle ligands were cleaved during the ionisation process (Supplementary Fig. S10–S12). Analysis of the complexes by elemental analysis provided further evidence of the coordination of the N-heterocyclic ligands to plecstatin-1 with the PCA ligand being deprotonated. The elemental analysis data match compositions with complex cations that are singly positively charged as a result of N-heterocyclic ligand coordination, and the overall charge is balanced by a PF6− counterion.
A single crystal of 4 was grown from an NMR sample in d6-acetone, and it crystallised as a pair of enantiomers together with disordered acetone. X-Ray diffraction analysis confirmed the expected piano-stool configuration with the PCA ligand coordinating bidentately through its pyridinyl-N and S atoms (Fig. 2). The thioamide moiety was found to be deprotonated, as evident by shorter C6–N2 and longer S1–C6 bonds compared to plecstatin-1 (Table 1),22 and a single PF6− counterion being present to balance the charge of the singly charged complex cation. In addition to the cym ligand, the coordination sphere was completed by methylimidazole bonded to the Ru centre by the imine-N atom. The length of the Ru–Nimidazole bond (Ru–N3) at 2.1071(16) Å) was in the similar range as other Ru–Nimidazole bonds39,40 but slightly longer than the Ru–Npyridine bond in 4 (Ru–N1; 2.094(16) Å) and much shorter than the Ru–Cl distance found in plecstatin-1 (2.392(9) Å).22 The bond angles around the coordination sphere of the Ru centre increased to accommodate the larger N-methylimidazole instead of the chlorido ligand in plecstatin-1. The torsion angle N1–C5–C6–S1 was 10.5(2)° for 4, suggesting that the pyridine ring and thioamide form a conjugated system and to a greater extent than plecstatin-1 (15.9(4)°), in which however the PCA ligand was found protonated.22
Molecular structure of one of the enantiomers of 4 drawn at 50% probability level. Co-crystallised solvent molecules and counterions were omitted for clarity.
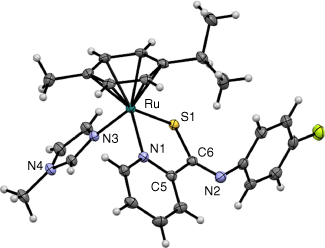
4 | plecstatin-1 | ||
---|---|---|---|
Bond lengths (Å) | |||
Ru–N1 | 2.0935(16) | 2.095(3) | |
Ru–S1 | 2.3625(5) | 2.3414(9) | |
Ru–N3 | 2.1071(16) | – | |
Ru–Cl | – | 2.3924(4) | |
C6–N2 | 1.283(3) | 1.314(4) | |
S1–C6 | 1.750(2) | 1.687(3) | |
Bond angles (°) | |||
S1–Ru–N1 | 81.96(5) | 81.28(8) | |
S1–Ru–N3 | 87.61(5) | – | |
N1–Ru–N3 | 86.02(6) | – | |
S1–Ru–Cl | – | 89.81(3) | |
N1–Ru–Cl | – | 83.68(8) | |
Torsion angle (°) | |||
N1–C5–C6–S1 | 10.5(2) | 15.9(4) |
Plecstatin-1 data are from Meier et al.22
Plecstatin-1 exhibits unique behaviour in aqueous solution. It rapidly forms dimers with PCA-S atoms bridging Ru centres (Fig. 3).22 Reversed phase–high-performance liquid chromatography (RP-HPLC) was used to investigate the behaviour of 4 and 6 in comparison to plecstatin-1 in aqueous solution (H2O:acetonitrile = 1:1). The retention times (Rt) of 4 and 6 were slightly different from that found for plecstatin-1 due to the N-heterocycle coordinated to the metal centre (Fig. 3, Supplementary Fig. S13–S17). However, both 4 and 6 underwent rapid dimerisation in aqueous conditions as evidenced by the formation of peaks with larger retention times. Co-injection of plecstatin-1, 4 and 6 revealed that they were forming the same dimeric complex, with the μ-S bridging atom inducing the dissociation of the N-heterocyclic or chlorido ligands from the Ru centres (Fig. 3). The differences in peak ratios for the two compounds may be explained by the ligand donor strengths for 1-methylimidazole as compared to pyridine.41 The former binds more strongly to metal centres than pyridine and accordingly the conversion of the complex into the dimer occurs to a lesser extent.
(a) Dimerisation of plecstatin in aqueous solution and conversion of 4 and 6 into the same dimer. (b) From top to bottom: chromatograms of solutions of plecstatin-1, 4, 6 and a mixture of plecstatin-1, 4 and 6 in MeCN/H2O (1:1).
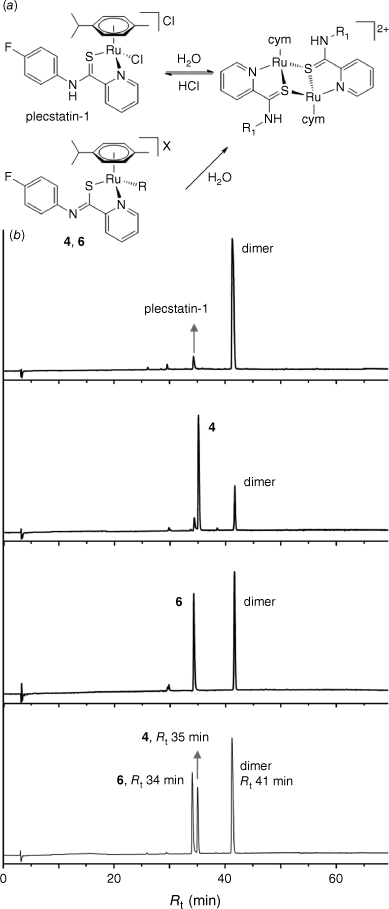
In another experiment complex 6 was incubated in water as well as in an aqueous solution acidified with HCl (60 mM) to give a pH value of 1. Aliquots taken after 0, 4 and 24 h were analysed by RP-HPLC (Supplementary Fig. S16 and S17). The complex showed rapid formation of a dimeric species in both media with a prominent peak with an intensity similar to 6 in the chromatogram at t = 0 h. In H2O, it appears as if an equilibrium is present with both complex 6 and the formed dimeric structure at a ratio of ~1:1. In the acidified solution, the initial dimerisation also occurred and after 4 h both complexes were detected at a similar ratio in the chromatogram. However, after 24 h the equilibrium shifted almost completely back to a monomeric species. Owing to the similarity in Rt it is difficult to say whether 6 is formed or the chlorido complex, i.e. plecstatin-1, due to the high chloride concentration. However, this transformation has relevance in a biological setting given the presence of relatively high chloride concentrations in blood serum and therewith affects the mode of action of the compound class.
The antiproliferative activity of 4–6 was determined against a small panel of human cancer cell lines and half-maximal inhibitory concentration (IC50) values in the low micromolar range were observed (Table 2). Complexes 4 and 6 gave IC50 values similar to plecstatin-1 in the cancer cell lines,21 whereas 5 was the slightly less active. That all three complexes still displayed good anticancer activity exemplifies the low effect the exchange of the leaving chlorido ligand with an N-heterocycle had on these ruthenium piano-stool complexes. Considering the studies in aqueous solution, the active pharmacophores for the compounds may well be the same and this explains IC50 values in a similar range. Alternatively, it is possible that the mode of action is based on non-covalent interactions between the compounds and their biomolecular target.19,22
Compounds | IC50 (μM) | ||||
---|---|---|---|---|---|
HCT 116 | NCI-H460 | SiHa | SW480 | ||
Plecstatin-1 | 6.5 ± 0.3 | 10 ± 2 | 8.3 ± 0.7 | 9.9 ± 0.7 | |
4 | 8.5 ± 1.8 | 10 ± 1 | 10 ± 2 | 9.6 ± 1.2 | |
5 | 16 ± 2 | 22 ± 2 | 18 ± 2 | 16 ± 1 | |
6 | 9.9 ± 0.8 | 12 ± 1 | 12 ± 1 | 11 ± 3 |
Plecstatin-1 data are taken from Arshad et al.21
Conclusions
The plecstatin class of metal complexes has shown promise as chemotherapeutic agents with their potent antiproliferative activity, specific interaction with their cellular target plectin and overall unique biological and physicochemical properties. Here, we expanded the diversity of plecstatin complexes by exchanging the chlorido ligand with the N-heterocycles 1-methylimidazole, 1-methylbenzimidazole and pyridine. Two avenues were explored to synthesise complexes 4–6, one which involved the preparation of the [Ru(cym)Cl2] complexes of the aforementioned N-heterocycles followed by reaction with p-F-PCA and the other in which plecstatin-1 was used as the precursor and the chlorido ligand was substituted for the N-heterocycles. Both approaches proved successful with the latter route deemed more efficient due to the fewer synthetic steps and ease of purification by precipitation. The coordination of the ligand to the metal Ru centres was confirmed through 1H–1H NOESY correlations in the 2-D NMR spectrum, single crystal X-ray diffraction and elemental analysis.
Replacing the chlorido ligand in plecstatin-1 for a more strongly coordinated N-heterocycle could have detrimental effects on the biological properties of such complexes as the chlorido–aqua ligand exchange is a key step for biological activity and considered an activation step. However, the synthesised derivatives still exhibited potent antiproliferative activity in the small panel of cancer cell lines, with IC50 values similar to or only slightly higher than those of plecstatin-1. This may be explained by the fact that the complexes with N-heterocycle co-ligands displayed the same behaviour in aqueous and acidic solutions as observed for their chlorido precursor. It appears as if the complexes undergo dimerisation in aqueous solution, whereas acidification with HCl seems to push the equilibrium towards a monomeric derivative. Therefore, exchanging the chlorido ligand with other ligands can provide opportunities for further functionalisation in the future, such as conjugation to delivery vectors with a functionalised N-heterocyclic co-ligand as a conjugation handle that could be cleaved under physiologically relevant conditions.
Data availability
The data that support this study are available in the article and accompanying online Supplementary material.
Acknowledgements
The authors thank the University of Auckland and the Maurice Wilkins Centre for Molecular Biodiscovery for financial support. They are grateful to Githal Arachchige for collecting ESI-QToF-MS data, Timothy Christopher for recording the single crystal X-ray diffraction data and Natalia Abrego for help with elemental analysis. Prof. Christian G. Hartinger was the recipient of the 2023 Burrows Award of the RACI Inorganic Chemistry Division.
References
1 Aird RE, Cummings J, Ritchie AA, Muir M, Morris RE, Chen H, Sadler PJ, Jodrell DI. In vitro and in vivo activity and cross resistance profiles of novel ruthenium(II) organometallic arene complexes in human ovarian cancer. Br J Cancer 2002; 86: 1652-1657.
| Crossref | Google Scholar | PubMed |
2 Yan YK, Melchart M, Habtemariam A, Sadler PJ. Organometallic chemistry, biology and medicine: ruthenium arene anticancer complexes. Chem Commun 2005; 2005(38): 4764-4776.
| Crossref | Google Scholar | PubMed |
3 Peacock AFA, Habtemariam A, Fernández R, Walland V, Fabbiani FPA, Parsons S, Aird RE, Jodrell DI, Sadler PJ. Tuning the reactivity of osmium(II) and ruthenium(II) arene complexes under physiological conditions. J Am Chem Soc 2006; 128: 1739-1748.
| Crossref | Google Scholar | PubMed |
4 Peacock AFA, Sadler PJ. Medicinal organometallic chemistry: designing metal arene complexes as anticancer agents. Chem Asian J 2008; 3: 1890-1899.
| Crossref | Google Scholar | PubMed |
5 Murray BS, Babak MV, Hartinger CG, Dyson PJ. The development of RAPTA compounds for the treatment of tumors. Coord Chem Rev 2016; 306: 86-114.
| Crossref | Google Scholar |
6 Morris RE, Aird RE, del Socorro Murdoch P, Chen H, Cummings J, Hughes ND, Parsons S, Parkin A, Boyd G, Jodrell DI, Sadler PJ. Inhibition of cancer cell growth by ruthenium(II) arene complexes. J Med Chem 2001; 44: 3616-3621.
| Crossref | Google Scholar | PubMed |
7 Scolaro C, Bergamo A, Brescacin L, Delfino R, Cocchietto M, Laurenczy G, Geldbach TJ, Sava G, Dyson PJ. In vitro and in vivo evaluation of ruthenium(II)−arene PTA complexes. J Med Chem 2005; 48: 4161-4171.
| Crossref | Google Scholar | PubMed |
8 Chen H, Parkinson JA, Morris RE, Sadler PJ. Highly selective binding of organometallic ruthenium ethylenediamine complexes to nucleic acids: novel recognition mechanisms. J Am Chem Soc 2003; 125: 173-186.
| Crossref | Google Scholar | PubMed |
9 Wu B, Ong MS, Groessl M, Adhireksan Z, Hartinger CG, Dyson PJ, Davey CA. A ruthenium antimetastasis agent forms specific histone protein adducts in the nucleosome core. Chem Eur J 2011; 17: 3562-3566.
| Crossref | Google Scholar | PubMed |
10 Nazarov AA, Hartinger CG, Dyson PJ. Opening the lid on piano-stool complexes: An account of ruthenium(II)–arene complexes with medicinal applications. J Organomet Chem 2014; 751: 251-260.
| Crossref | Google Scholar |
11 Adhireksan Z, Davey GE, Campomanes P, Groessl M, Clavel CM, Yu H, Nazarov AA, Yeo CHF, Ang WH, Dröge P, Rothlisberger U, Dyson PJ, Davey CA. Ligand substitutions between ruthenium–cymene compounds can control protein versus DNA targeting and anticancer activity. Nat Commun 2014; 5: 3462.
| Crossref | Google Scholar | PubMed |
12 Nazarov AA, Meier SM, Zava O, Nosova YN, Milaeva ER, Hartinger CG, Dyson PJ. Protein ruthenation and DNA alkylation: chlorambucil-functionalized RAPTA complexes and their anticancer activity. Dalton Trans 2015; 44: 3614-3623.
| Crossref | Google Scholar | PubMed |
13 Artner C, Holtkamp HU, Kandioller W, Hartinger CG, Meier-Menches SM, Keppler BK. DNA or protein? Capillary zone electrophoresis–mass spectrometry rapidly elucidates metallodrug binding selectivity. Chem Commun 2017; 2017(57): 8002-8005.
| Crossref | Google Scholar | PubMed |
14 Artner C, Holtkamp HU, Hartinger CG, Meier-Menches SM. Characterizing activation mechanisms and binding preferences of ruthenium metallo-prodrugs by a competitive binding assay. J Inorg Biochem 2017; 177: 322-327.
| Crossref | Google Scholar | PubMed |
15 Kenny RG, Marmion CJ. Toward multi-targeted platinum and ruthenium drugs – a new paradigm in cancer drug treatment regimens? Chem Rev 2019; 119: 1058-1137.
| Crossref | Google Scholar | PubMed |
16 Steel TR, Walsh F, Wieczorek-Błauż A, Hanif M, Hartinger CG. Monodentately coordinated bioactive moieties in multimodal half-sandwich organoruthenium anticancer agents. Coord Chem Rev 2021; 439: 213890.
| Crossref | Google Scholar |
17 Tremlett WDJ, Goodman DM, Steel TR, Kumar S, Wieczorek-Błauż A, Walsh FP, Sullivan MP, Hanif M, Hartinger CG. Design concepts of half-sandwich organoruthenium anticancer agents based on bidentate bioactive ligands. Coord Chem Rev 2021; 445: 213950.
| Crossref | Google Scholar |
18 Sumithaa C, Ganeshpandian M. Half-sandwich ruthenium arene complexes bearing clinically approved drugs as ligands: the importance of metal–drug synergism in metallodrug design. Mol Pharmaceutics 2023; 20: 1453-1479.
| Crossref | Google Scholar | PubMed |
19 Hartinger CG. A multifaceted approach towards organometallic anticancer agent development. J Organomet Chem 2024; 1012: 123144.
| Crossref | Google Scholar |
20 Kinney WA, Lee NE, Blank RM, Demerson CA, Sarnella CS, Scherer NT, Mir GN, Borella LE, Dijoseph JF, Wells C. N-Phenyl-2-pyridinecarbothioamides as gastric mucosal protectants. J Med Chem 1990; 33: 327-336.
| Crossref | Google Scholar | PubMed |
21 Arshad J, Hanif M, Movassaghi S, Kubanik M, Waseem A, Söhnel T, Jamieson SMF, Hartinger CG. Anticancer Ru(η6-p-cymene) complexes of 2-pyridinecarbothioamides: a structure–activity relationship study. J Inorg Biochem 2017; 177: 395-401.
| Crossref | Google Scholar | PubMed |
22 Meier SM, Hanif M, Adhireksan Z, Pichler V, Novak M, Jirkovsky E, Jakupec MA, Arion VB, Davey CA, Keppler BK, Hartinger CG. Novel metal(II) arene 2-pyridinecarbothioamides: a rationale to orally active organometallic anticancer agents. Chem Sci 2013; 4: 1837-1846.
| Crossref | Google Scholar |
23 Meier SM, Novak M, Kandioller W, Jakupec MA, Arion VB, Metzler-Nolte N, Keppler BK, Hartinger CG. Identification of the structural determinants for anticancer activity of a ruthenium arene peptide conjugate. Chem Eur J 2013; 19: 9297-9307.
| Crossref | Google Scholar | PubMed |
24 Hanif M, Moon S, Sullivan MP, Movassaghi S, Kubanik M, Goldstone DC, Söhnel T, Jamieson SMF, Hartinger CG. Anticancer activity of Ru- and Os(arene) compounds of a maleimide-functionalized bioactive pyridinecarbothioamide ligand. J Inorg Biochem 2016; 165: 100-107.
| Crossref | Google Scholar | PubMed |
25 Arshad J, Hanif M, Zafar A, Movassaghi S, Tong KKH, Reynisson J, Kubanik M, Waseem A, Söhnel T, Jamieson SMF, Hartinger CG. Organoruthenium and organoosmium complexes of 2-pyridinecarbothioamides functionalized with a sulfonamide motif: synthesis, cytotoxicity and biomolecule interactions. ChemPlusChem 2018; 83: 612-619.
| Crossref | Google Scholar | PubMed |
26 Arshad J, Tong KKH, Movassaghi S, Söhnel T, Jamieson SMF, Hanif M, Hartinger CG. Impact of the metal center and leaving group on the anticancer activity of organometallic complexes of pyridine-2-carbothioamide. Molecules 2021; 26: 833.
| Crossref | Google Scholar | PubMed |
27 Iqbal S, Siddiqui WA, Ashraf A, Tong KKH, Aman F, Söhnel T, Jamieson SMF, Hanif M, Hartinger CG. Substitution of the chlorido ligand for PPh3 in anticancer organoruthenium complexes of sulfonamide-functionalized pyridine-2-carbothioamides leads to high cytotoxic activity. Inorg Chim Acta 2022; 536: 120889.
| Crossref | Google Scholar |
28 Riaz Z, Lee BYT, Stjärnhage J, Movassaghi S, Söhnel T, Jamieson SMF, Shaheen MA, Hanif M, Hartinger CG. Anticancer Ru and Os complexes of N-(4-chlorophenyl)pyridine-2-carbothioamide: Substitution of the labile chlorido ligand with phosphines. J Inorg Biochem 2023; 241: 112115.
| Crossref | Google Scholar | PubMed |
29 Meier SM, Kreutz D, Winter L, Klose MHM, Cseh K, Weiss T, Bileck A, Alte B, Mader JC, Jana S, Chatterjee A, Bhattacharyya A, Hejl M, Jakupec MA, Heffeter P, Berger W, Hartinger CG, Keppler BK, Wiche G, Gerner C. An organoruthenium anticancer agent shows unexpected target selectivity for plectin. Angew Chem Int Ed Engl 2017; 56: 8267-8271.
| Crossref | Google Scholar | PubMed |
30 Riisom M, Eade L, Tremlett WDJ, Hartinger CG. The aqueous stability and interactions of organoruthenium compounds with serum proteins, cell culture medium, and human serum. Metallomics 2022; 14: mfac043.
| Crossref | Google Scholar | PubMed |
31 Riisom M, Morrow SJ, Herbert CD, Tremlett WDJ, Astin JW, Jamieson SMF, Hartinger CG. In vitro and in vivo accumulation of the anticancer Ru complexes [RuII(cym)(HQ)Cl] and [RuII(cym)(PCA)Cl]Cl. J Biol Inorg Chem 2023; 28: 767-775.
| Crossref | Google Scholar | PubMed |
32 Steel TR, Hartinger CG. Metalloproteomics for molecular target identification of protein-binding anticancer metallodrugs. Metallomics 2020; 12: 1627-1636.
| Crossref | Google Scholar | PubMed |
33 Kumar S, Riisom M, Jamieson SMF, Kavianinia I, Harris PWR, Metzler-Nolte N, Brimble MA, Hartinger CG. On-resin conjugation of the ruthenium anticancer agent plecstatin-1 to peptide vectors. Inorg Chem 2023; 62: 14310-14317.
| Crossref | Google Scholar | PubMed |
34 Truong D, Lam NYS, Kamalov M, Riisom M, Jamieson SMF, Harris PWR, Brimble MA, Metzler-Nolte N, Hartinger CG. A solid support-based synthetic strategy for the site-selective functionalization of peptides with organometallic half-sandwich moieties. Chem Eur J 2022; 28: e202104049.
| Crossref | Google Scholar | PubMed |
35 Albada B, Metzler-Nolte N. Organometallic–peptide bioconjugates: synthetic strategies and medicinal applications. Chem Rev 2016; 116: 11797-11839.
| Crossref | Google Scholar | PubMed |
37 Parveen S, Hanif M, Movassaghi S, Sullivan MP, Kubanik M, Shaheen MA, Söhnel T, Jamieson SMF, Hartinger CG. Cationic Ru(η6-p-cymene) complexes of 3-hydroxy-4-pyr(id)ones – lipophilic triphenylphosphine as co-ligand is key to highly stable and cytotoxic anticancer agents. Eur J Inorg Chem 2017; 2017: 1721-1727.
| Crossref | Google Scholar |
38 Tremlett W, Tong K, Steel TR, Movassaghi S, Hanif M, Jamieson S, Söhnel T, Hartinger CG. Hydroxyquinoline-derived anticancer organometallics: introduction of amphiphilic PTA as an ancillary ligand increases their aqueous solubility. J Inorg Biochem 2019; 199: 110768.
| Crossref | Google Scholar | PubMed |
39 Baird IR, Rettig SJ, James BR, Skov KA. Synthesis and characterization of homoleptic ruthenium(II) imidazole complexes, and a carbonyl species derived by CO abstraction from DMF. Can J Chem 1998; 76: 1379-1388.
| Crossref | Google Scholar |
40 Matveevskaya VV, Pavlov DI, Samsonenko DG, Ermakova EA, Klyushova LS, Baykov SV, Boyarskiy VP, Potapov AS. Synthesis and structural characterization of half-sandwich arene–ruthenium(II) complexes with bis(imidazol-1-yl)methane, imidazole and benzimidazole. Inorganics 2021; 9: 34.
| Crossref | Google Scholar |
41 Teng Q, Huynh HV. A unified ligand electronic parameter based on 13C NMR spectroscopy of N-heterocyclic carbene complexes. Dalton Trans 2017; 46: 614-627.
| Crossref | Google Scholar | PubMed |