Plant life-history data as evidence of an historical mixed-severity fire regime in Banksia Woodlands
Russell G. Miller




A
B
C
Abstract
The concept of the fire regime is central to understanding and managing fire-prone ecosystems globally, and information on past regimes can provide useful insights into species disturbance adaptations. Although observations from satellite imagery or palaeoecological proxy data can provide direct evidence of past, pre-colonial fire regimes, they may be limited in temporal or spatial resolution and are not available for all ecosystems. However, fire-related plant-trait and demographic data offer an alternative approach to understand species–fire regime associations at the ecosystem scale.
We aimed to quantify the life-history strategies and associated fire regimes for six co-occurring shrub and tree species from fire-prone, Mediterranean-climate Banksia Woodlands in south-western Australia.
We collected static demographic data on size structure, seedling recruitment, and plant mortality across sites of varying time since last fire. We combined demographic data with key fire-related species traits to define plant life-history strategies. We then compared observed life histories with a priori expectations for surface, stand-replacing, and mixed-severity fire-regime types to infer historical fire-regime associations.
Fire-killed shrubs and weakly serotinous trees had abundant post-fire seedling recruitment, but also developed multi-cohort populations during fire-free periods via inter-fire seedling recruitment. Resprouting shrubs had little seedling recruitment at any time, even following fire, and showed no signs of decline in the long absence of fire, likely owing to their very long lifespans.
The variation in life-history strategies for these six co-occurring species is consistent with known ecological strategies to cope with high variation in fire intervals in a mixed-severity fire regime. Whereas resprouting and strong post-fire seedling recruitment indicate a tolerance of shorter fire intervals, inter-fire recruitment and weak serotiny are interpreted as a bet-hedging strategy to cope with occasional long fire-free periods that may otherwise exceed adult and seed-bank lifespans.
Our findings suggested that Banksia Woodlands have evolved with highly variable fire intervals in a mixed-severity fire regime. Further investigations of species adaptations to varying fire size and patchiness can help extend our understanding of fire-regime tolerances.
Keywords: ecological disturbance, ecosystem management, fire interval, life-history strategy, resprouting, seedling recruitment, serotiny, soil seed bank, species traits.
Introduction
Patterns of ecological disturbance, such as by fire, have shaped species traits and ecosystem structure for millions of years (Lamont et al. 2019; Keeley and Pausas 2022). Species population dynamics and coexistence are often contingent on disturbance dynamics that reflect historical patterns (Johnstone et al. 2016; Quintana-Ascencio et al. 2019; Miller and Safford 2020), and therefore, understanding the characteristic fire regime of an ecosystem is crucial for its management and conservation. Altered or ‘inappropriate’ fire regimes are listed as a threatening process for many endangered species and ecosystems (Shedley et al. 2018; Monks et al. 2019), but baseline data on historical fire regimes are often poorly quantified. Reconstructing attributes of past fire regimes, such as frequency, intervals, and severity, can help improve our understanding of the role of fire in the landscape and provide a reference that can help guide contemporary ecosystem management (Swetnam et al. 1999; McLauchlan et al. 2020).
Fire regimes are often defined by a broadly inverse relationship between fire frequency and severity, which is driven by interactions among climate, vegetation structure, and topography (Murphy et al. 2013; Miller and Safford 2020). Major fire-regime types range from frequent, surface-fire regimes in open ecosystems (e.g. grasslands, tropical savannas, and open forests) to infrequent, high-severity stand-replacing crown-fire regimes in closed ecosystems (e.g. shrublands and closed forests) (Enright et al. 2012; Archibald et al. 2013; Murphy et al. 2013). Mixed-severity fire regimes are another important regime type, comprising a mosaic of unburnt, low-, moderate-, and high-severity fire effects within and between fires and with higher variance in frequency occurring in some dry and open woodlands and forest types with complex spatial or successional patterns (Agee 2005; Halofsky et al. 2011; Perry et al. 2011). Agee (2005) noted that mixed-severity fire regimes may elicit a unique ecosystem and species response that is not a simple intermediate between surface- and crown-fire regimes, often owing to the complex fine-grained mosaic of fire effects, and patterns of variation through time.
Historical fire regimes can be difficult to document, given the transient and complex nature of fire effects, limitations of data sources, colonial exclusion of Indigenous management and knowledge, and modern anthropogenic disruptions to historical fire patterns (Miller and Murphy 2017; McLauchlan et al. 2020). Satellite imagery has become a powerful tool for mapping past fires, but even the most extensive records cover only the past ~50 years, limiting the capacity to assess long-term fire regimes driving species evolution (Archibald et al. 2013; Callister et al. 2016). Dendrochronological (tree-ring dating) and other palaeoecological proxy archives (e.g. sedimentary charcoal) provide longer-term fire-history data, but are often limited in their spatial or temporal resolution and are not equally available in all ecosystems (Conedera et al. 2009; McLauchlan et al. 2020).
In this paper, we instead use contemporary plant-trait and demographic data to infer historical fire-regime type (Armstrong and Phillips 2012; de Gouvenain and Delgadillo 2012). We leverage the understanding that fire regimes impose a strong evolutionary pressure on plant species, often limiting the range of compatible life-history strategies and leading to the development of characteristic adaptation syndromes involving suites of correlated traits (Schwilk and Ackerly 2001; Keeley 2012; Pausas 2015; Varner et al. 2016). This approach also has the benefit of being broadly applicable, because all species have traits and exhibit demographic rates of recruitment, growth, survival, and reproduction, and findings can directly inform management for each species.
Plant species’ adaptations to different fire regimes usually involve a trade-off between traits that enhance either adult survival (‘fire-tolerator’ syndrome) or post-fire regeneration via seedling recruitment (‘fire-embracer’ syndrome) (Schwilk and Ackerly 2001; Pausas 2015; Treurnicht et al. 2016). These necessary trade-offs are consistent with those recognised for broader life-history strategies for plants and animals that describe the speed at which biota live (Franco and Silvertown 1996; Salguero-Gómez et al. 2016). Under this framework, plants can be separated into fast-living species with rapid growth, high reproduction, short lifespan, and rapid generation turnover (analogous to fire embracers) versus slow-living species with slow growth, low fecundity, long lifespan, and slow generation turnover (analogous to fire tolerators).
Fire-tolerating species can be linked to low-severity, surface-fire regimes and benefit from traits such as thick bark and low flammability that enhance survival, allow retention of aboveground biomass, and allow growth and reproduction to continue largely uninterrupted following fire (Schwilk and Ackerly 2001; Pausas et al. 2017). In contrast, fire-embracing species are linked to high-severity, stand-replacing fire regimes and may be fire-killed (also called post-fire obligate seeders) but benefit from traits such as canopy or soil seed storage and fire-cued germination (Pausas and Keeley 2014). These traits maximise seed accumulation during the inter-fire period and trigger mass seedling regeneration in the post-fire environment where competition with mature plants for limited resources is reduced (Pausas 2015).
The limitations that fire regimes impose on compatible plant traits and demographic parameters can be used to derive a set of indicators of characteristic fire regimes (Table 1). For example, the distribution of different plant-size or age cohorts in a population reflect past recruitment and mortality dynamics, and an understanding of how fire affects these processes can provide an indication of the type of fire regime to which a species is adapted (Wiegand et al. 2000; Lehmann et al. 2009). Single-cohort (even-aged) stands are common in dominant shrub or tree canopy species where stand-replacing fire regimes occur and where established plants are killed by fire and seedlings are intolerant of competition with mature individuals, such that seedling regeneration is usually restricted to the post-fire environment (Keeley et al. 2006; Schoennagel et al. 2006; Pausas and Keeley 2014). Some species that resprout from basal or belowground structures may also resemble single-sized cohorts (unimodal distribution) where fire kills aboveground biomass and plants simultaneously resprout in the post-fire environment, along with typically low levels of post-fire seedling recruitment (Enright and Goldblum 1999; Pausas and Keeley 2014). Single-cohort stands are common in wet forests and shrublands where high tree- or shrub-canopy cover often precludes seedling establishment during the inter-fire period (Ashton 1976; Enright et al. 1996). Single-cohort stands indicate that species are adapted to stand-replacing crown-fire regimes and are also often associated with prolonged seed-bank storage (e.g. strong serotiny or long-lived, dormant soil seed bank) to ensure adequate seed supply at the time of fire (Gauthier et al. 1996; Auld et al. 2007; Clarke et al. 2015; Agne et al. 2022).
Demographic parameter | Fire-regime type | |||
---|---|---|---|---|
Stand-replacing (low frequency, high severity) | Surface (high frequency, low severity) | Mixed severity (variable frequency, variable severity) | ||
Cohort structure | Single post-fire cohortA,B | Understorey: single post-fire cohort Canopy: multi-cohortC | Understorey: single post-fire cohort, developing into multi-cohort without fire Canopy: single or multi post-fire cohort depending on recent fire severity, developing into multi-cohort without fireA | |
Seedling recruitment | Post-fire onlyD | Semi-continuousE,F | Short-lived species: post- and inter-fireG Long-lived species: likely post-fire onlyG | |
Adult lifespan | One fire cycleH | Understorey: one fire cycleH Canopy: multiple fire cyclesH | Understorey: less than or equal to one fire cycle depending on interval Canopy: less than, equal to, or greater than one fire cycle depending on interval and severity | |
Canopy seed storage | Prolonged (e.g. strong serotiny)I | None–transientJ,K | TransientG,I | |
Example ecosystems | Closed conifer forest, shrubland | Grassland, savanna | Dry open forest, woodland |
Demographic parameters refer to those for perennial species at the scale of individuals or groups of individuals subject to the same fire interval and severity. For species that can resprout from basal shoots, cohort structures and adult lifespans refer to those of aboveground living biomass, acknowledging that underground organs (e.g. lignotubers, bulbs, rhizomes) that enable resprouting may persist through multiple fire cycles.
Short-lived species, lifespan less than median fire interval; long-lived species, lifespan greater than median fire interval.
Multi-cohort or mixed-aged stands indicate an alternative life-history strategy where adult mortality and seedling recruitment are not necessarily tied to fire, or where fire effects vary widely among fires and between surface and canopy vegetation layers. Multi-cohort stands can arise from incomplete fire mortality or fire-independent recruitment in vegetation gaps (Gauthier et al. 1993; Campbell et al. 2012). Incomplete fire mortality is common for resprouters but may also occur in fire-killed trees or tall shrubs under a surface or mixed-severity fire regime (Lindenmayer et al. 2000). Seedling recruitment in fire-prone ecosystems may be more successful in the post-fire environment, especially where fire causes a substantial change in the competition environment (Cowling and Lamont 1987). Where fire intervals are regular, a post-fire recruitment strategy maximises the chance that seedlings will reach maturity prior to subsequent fire (Enright et al. 1998; Lamont et al. 2020). Fire-independent recruitment may be part of a bet-hedging strategy to avoid local extinction in the long absence of fire, or in the absence of reliable or strong fire cues from surface or low-severity fire (Lamont et al. 2020). Under a mixed-severity regime, individual plants (or groups of plants) may not receive crown scorch for long periods if fire simply does not occur, or if they escape the effects of fire in unburnt or low-severity patches, as would especially be the case for tree species.
Variation in canopy seed storage (serotiny) in relation to fire regimes has been well studied, with evidence that the degree of serotiny (i.e. the length of time that seeds are retained in woody fruits) (Lamont 2021) is lowest where crown fires are rare (Gauthier et al. 1996; Radeloff et al. 2004; Hernández-Serrano et al. 2013; de Gouvenain et al. 2019). Strongly serotinous species that retain seeds for long periods of time (>10 years) typically rely on the heat of fire as a cue to release seeds into the resource-rich post-fire environment. However, under a fire regime with rare or inconsistent crown-fire cues, strong serotiny may be detrimental for population persistence, and therefore some species or populations have developed weak to moderate degrees of serotiny where seeds may be retained for only a few years and are spontaneously released without fire (Lamont et al. 2020). Some species with soil seed storage may also possess a similar bet-hedging strategy where physical seed-dormancy break can be triggered by both fire and summer soil temperatures, termed facultative pyrogenic seed dormancy, or may have lower dormancy-breaking temperature thresholds as seeds age (Ooi et al. 2014; Liyanage and Ooi 2015; Liyanage and Ooi 2017). Variation in dormancy-breaking thresholds would allow at least some germination following a range of low to high fire severities, but also in the long absence of fire, all of which are possible scenarios in a mixed-severity fire regime.
In this study, we investigated the life-history characteristics and historical fire-regime associations of six co-occurring shrub and tree species from low, open Banksia Woodlands in south-western Australia (Ritchie et al. 2021). We explored the idea that contemporary plant traits and demographic parameters provide a signature of past fire regimes experienced during a species’ evolutionary history. We collected static demographic data to quantify patterns of recruitment, mortality, and population-cohort structures along a fire-age chronosequence across 34 sites and compared to a priori expectations for different fire-regime types (i.e. surface, stand-replacing, and mixed-severity; Table 1). Species varied in their growth form (shrub vs tree), fire-response type (fire-killed vs resprouter), lifespan (short vs long-lived), and seed-bank storage strategy (canopy vs soil stored seed).
We expected that fire effects and associated life-history strategies could vary between growth forms and fire-response types and suggest adaptations to a range of low to high fire frequencies and severities, overall indicating an historical mixed-severity fire regime. Previous work has suggested that these low, open woodlands with discontinuous fuels can experience a wide variety of fire frequencies and severities (Densmore and Clingan 2019), with evidence for rapid post-fire surface and understorey fuel accumulation (Burrows and McCaw 1990; Tangney et al. 2022), rapid post-fire species recovery (Wilson et al. 2014), a wide range of plausible fire ages (up to 50–60 years) (Wilson et al. 2014), and plant traits associated with adaptations to high severity (e.g. resprouting, fire-cued germination) or variable severity (e.g. weak serotiny) (Cowling and Lamont 1985).
Materials and methods
Study location
Banksia Woodlands occur on nutrient-poor, deep sandy soils of the Swan Coastal Plain and smaller sandy pockets elsewhere in south-western Australia. They are characterised by a low (4–8 m height), open canopy dominated by Banksia spp. (Proteaceae) trees (particularly Banksia attenuata, B. prionotes, and B. menziesii) over a species-rich understorey dominated by sclerophyllous shrubs largely comprising the plant families Dilleniaceae, Ericaceae, Fabaceae, Myrtaceae, and Proteaceae (Dodd and Griffin 1989; Ritchie et al. 2021). Soils of the Swan Coastal Plain consist of two main Pleistocene dune complexes distinguished by their age of origin (Spearwood sands: 120,000–500,000 years old, Bassendean sands: ~2 million years old) and nutrient content (particularly nitrogen and phosphorus, declining with age) (Turner et al. 2018).
The region experiences a Mediterranean-type climate, with cool, wet winters, and hot, dry summers, making vegetation highly fire-prone during the dry season (austral summer and autumn; December–May). Average annual precipitation ranges from approximately 800 mm in the south, to 550 mm in the north, 80% of which falls between May and October (Tangney et al. 2022). Much of the original extent of Banksia Woodlands has been cleared in recent decades to accommodate the rapid urbanisation of Western Australia’s capital city of Perth. As a result, Banksia Woodlands are listed as a federally recognised Threatened Ecological Community, with smaller portions listed at the state level (Ramalho et al. 2014; Ritchie et al. 2021). Remnant Banksia Woodlands persist in a few large conservation areas on the city margins, and in small–medium-sized urban and peri-urban reserves; however, even in these areas, there is projected ongoing species loss associated with time-delayed effects of habitat loss and fragmentation (Fowler et al. 2023).
Human-caused ignitions are the major source of wildfires during summer and autumn (Plucinski 2014). Prescribed burning is a widespread management practice on the Swan Coastal Plain, with most prescribed burning being conducted in spring (September–November) for risk reduction and conservation purposes (Plucinski 2014; Howard et al. 2020). The proximity of urban and peri-urban Banksia Woodlands to suburbs and other infrastructure underscores the importance of fire management in this ecosystem. Although there is little evidence of historical fire intervals in Banksia Woodlands, they share attributes (e.g. key plant functional types, and surface and elevated fuel types) with surrounding vegetation types, including shrublands to the north and eucalypt forests to the east and south, where fire intervals have been estimated at 10–40+ years (shrublands) and 5–20 years (low severity, forest) or 20–100 years (high severity, forest) (Enright and Thomas 2008; Enright et al. 2012; Murphy et al. 2013). Indigenous use of fire was widespread in the region prior to European colonisation; however, specific information regarding burning in Banksia Woodlands is not clear (Lullfitz et al. 2021; Rodrigues et al. 2022).
Study species
We selected six woody plant species that are common and widespread in Banksia Woodlands (Table 2). These species represent different plant fire-response types (resprouting, fire-killed), life forms (shrub, tree), lifespans (short, long), and seed-bank storage modes (canopy, soil) and four common plant families (Table 2). In the absence of detailed longevity data, individual plant lifespans were estimated on the basis of field observations of species-growth and senescence patterns. Short-lived shrubs show rapid post-fire growth, followed by clear signs of senescence a few to several years later, whereas long-lived species (especially resprouting shrubs) show little to no signs of senescence even in long-unburnt vegetation. The two selected tree Banksia species are known to be weakly serotinous in the study area, with most seeds being released from woody fruits (hereafter cones) within 1–2 years of cone maturity (Cowling and Lamont 1985). The shrub species Allocasuarina humilis is moderately serotinous, retaining seeds for up to 5 years (R. Miller, pers. obs.). Germination and seedling emergence for all species is restricted to the cool, wet winter–spring period (Miller et al. 2021).
Species | Growth form A | Fire response type | Resprouting | Estimated lifespan | Seed bank | Seed dormancy type | N | |
---|---|---|---|---|---|---|---|---|
Gompholobium tomentosum (Fabaceae) | Shrub (single-stemmed; 0.3–1 m) | Fire-killed | None | Short (~5 years) | Soil | PY | 17 | |
Acacia pulchella (Fabaceae) | Shrub (single-stemmed; 0.5–2 m) | Fire-killed | None | Short (10–15 years) | Soil | PY | 16 | |
Banksia prionotes (Proteaceae) | Tree (up to 10 m) | Fire-killed | None | Long (40–60 years) | Canopy (weak) | ND | 11 | |
Hibbertia hypericoides (Dilleniaceae) | Subshrub (multi-stemmed; 0.2–1 m) | Resprouter | Basal | Long (80–100 years) | Soil | MPD B | 17 | |
Allocasuarina humilis (Casuarinaceae) | Shrub (multi-stemmed; 0.2–2 m) | Resprouter | Usually basal | Long (80–100 years) | Canopy (moderate) | ND | 11 | |
Banksia attenuata (Proteaceae) | Tree (up to 10 m) | Resprouter | Epicormic and/or basal | Long (>100 years) | Canopy (weak) | ND | 16 |
Seed dormancy type: PY, physical dormancy; ND, no dormancy; MPD, morphophysiological dormancy.
Study sites
To establish a space-for-time chronosequence of fire intervals (times since fire), we first interrogated fire-history data for remnant vegetation in urban and peri-urban reserves across the study region to identify a set of candidate sites with a wide range of fire ages, suitable access (with permission from land managers), and appropriate vegetation type (i.e. not wetland, riparian, or limestone vegetation). Year of last fire for each candidate site was determined from spatial data layers maintained by the Western Australian Department of Biodiversity, Conservation and Attractions (DBCA-060, https://catalogue.data.wa.gov.au/dataset/dbca-fire-history); historical satellite and aerial imagery (Landgate, https://map-viewer-plus.app.landgate.wa.gov.au/index.html), and local government records. Given limitations in fire-history records and satellite and aerial imagery, we were unable to confirm exact ages for some sites beyond 35 years (occurring before approximately 1986). Sites with no assignable fire age are referred to here as long-unburnt (LUB), and for analysis are given a notional age of 35 years.
We visited candidate sites to confirm the above criteria as well as the presence of the study species and the absence of human-caused soil or vegetation disturbances that could otherwise alter demographic attributes. This produced a total of 34 sites across a fire-interval chronosequence from 1 to 35 years (or more), with each site containing one to five study species. The number of sites surveyed for each species ranged from 11 to 17, all spanning a similar post-fire chronosequence (Table 2). The most recent fire at most sites were wildfires, although a few were burnt by prescribed fires. Prescribed burns occurred in spring or autumn and wildfires mostly occurred in summer, with a few in late spring or early autumn. All sites containing B. prionotes were last burnt by wildfires. Sites were mostly located on Spearwood sands across a 100-km extent of the Swan Coastal Plain following the north–south rainfall gradient (Fig. 1), with sites of varying fire age spread as evenly as possible across this rainfall gradient (Supplementary Fig. S1). Mean annual rainfall ranged from 719 mm at the northern-most sites (Station #009105 Wanneroo, 1971–2000), to 812 mm in the southern-most sites (Station #009572 Halls Head, 1971–2000) (http://www.bom.gov.au/climate/data/).
Locations of the 34 study sites in urban and peri-urban nature reserves on the Swan Coastal Plain around Perth, Western Australia. Some reserves contained multiple sites of various fire ages (some points obscured by other points). Mean annual rainfall follows a north–south (low–high) gradient indicated by the colour (light blue–dark blue) of site location points (circles). The distribution of sites with varying rainfall across the sampled fire-age chronosequence is shown in Fig. S1. Green shading indicates extant native vegetation, grey shading indicates urban areas, and the solid red line indicates the boundary of the Swan Coastal Plain region (also shown on inset map).
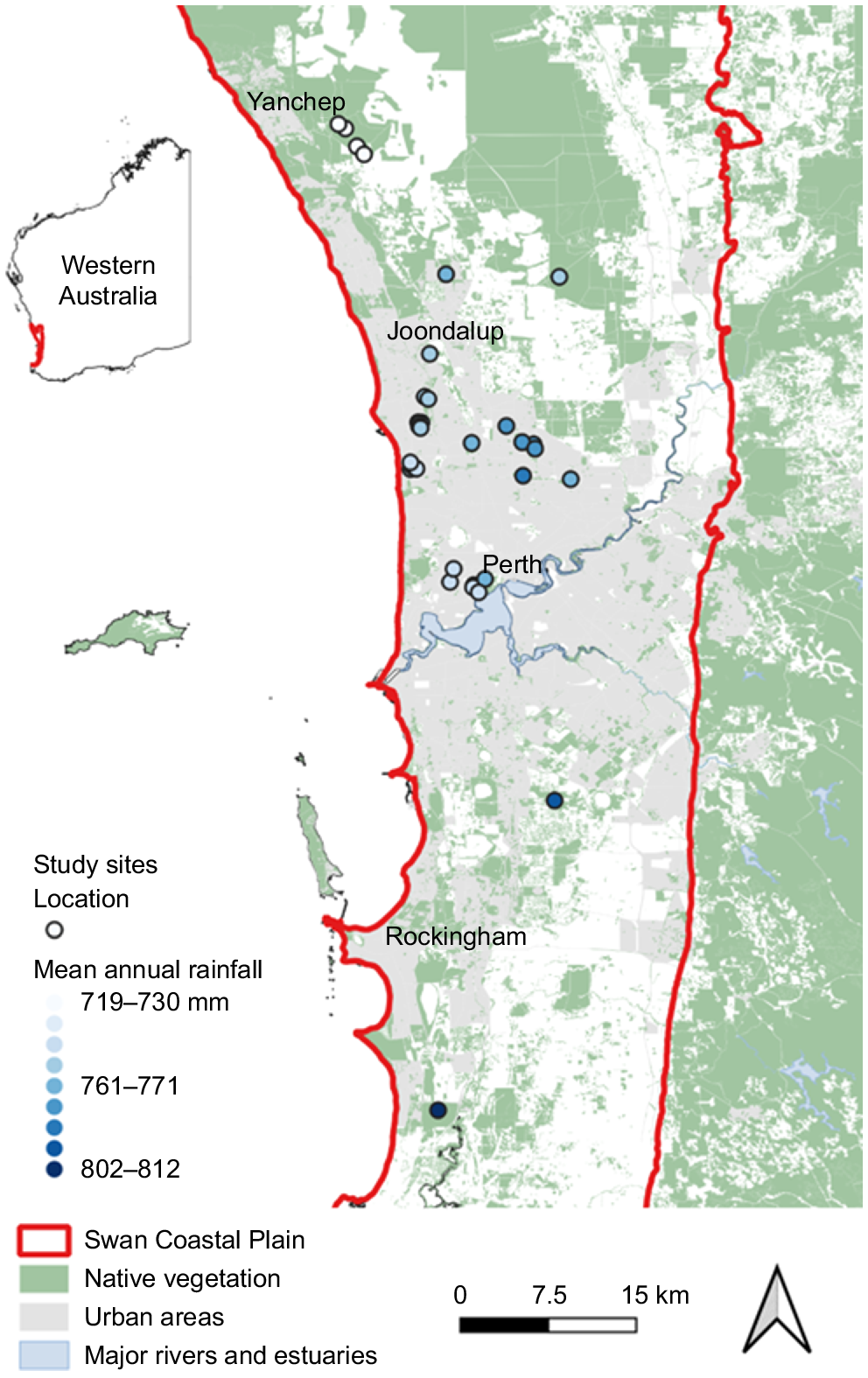
Demographic sampling
At each site, we recorded the size of all plants (including seedlings, juveniles, adults, and dead standing plants) of each species in plots. Plots ranged in size from 25 to 150 m2 to suit species of different growth forms. Smaller plots were used for shrub species (0.1–4.6 plants m−2), and larger plots for tree species (0.03–1.58 plants m−2). At least two plots were established at each site, and at least 30 individuals were sampled in each plot. However, where plant density was low, measurements were taken from an extended area based on a nearest-neighbour search until a minimum of 30 plants were recorded. For some plot-based surveys, additional nearest plants outside of plots were measured to fulfil the sample-size criterion.
Individual plants were designated as seedlings on the basis of their size and lack of reproduction (i.e. lack of flowering or evidence of past flowering), and were estimated to be 1 or 2 years old for shrub species (<30 cm tall) and up to 3 years old for tree species (<60 cm tall), on the basis of observations of seedlings of known age in post-fire sites elsewhere. Standing dead plants were recorded as a measure of recent mortality. Dead plants are likely to remain standing and easily detectable for varying times on the basis of growth form, as follows: small shrubs (Acacia pulchella, Gompholobium tomentosum, and Hibbertia hypericoides) up to 1 year; large shrubs (A. humilis) 2 or 3 years; and trees (B. attenuata and B. prionotes) at least 3–5 years.
For all species except the resprouting shrubs (H. hypericoides and A. humilis), maximum plant height was deemed the most suitable and representative measurement of size, given their single-stemmed, shrub or tree growth form. Canopy volume was used as a measure of size for both resprouting shrub species, given their multi-stemmed and spreading growth forms. Canopy volume (V, m3) was calculated from maximum plant-height (H, m) and perpendicular canopy-width measurements (W1 and W2, m), by using an ellipsoid form, as follows:
Data analysis
In total, 3742 live and dead plants were recorded across the six species in 34 sites. Sample sizes for each species ranged from 387 (A. humilis) to 805 (G. tomentosum) individuals. To test our a priori hypothesis that demographic patterns vary among species according to growth form and fire-response type, we visually assessed population-size distributions to identify cohort structures, and modelled changes in size-distribution metrics (mean size, Gini coefficient, permutation index), seedling recruitment, mortality, and plant density relative to time since fire. These analyses allowed us to understand the life-history strategies that plant populations employ to persist under different fire regimes, specifically varying patterns of fire interval and severity, and to infer the historical fire-regime type that is compatible with strategies of these co-occurring species.
Size distributions of live plants (seedlings, juveniles, adults) were constructed according to time-since-fire classes along the sampled fire-age chronosequence. Time-since-fire classes were chosen to encompass multiple sites (with data pooled for analysis) with approximately the same number of sites per grouping, and to represent different post-fire stand-development stages, ranging from initial post-fire recovery to reproductive maturity, and potentially population senescence. To help provide context for the visual assessment of size distributions, we compare patterns here to those observed for Hakea decurrens (fire-killed) and Hakea rostrata (resprouter), two model shrub species from fire-prone eucalypt woodlands in south-eastern Australia (data reproduced from Enright and Goldblum 1999). These model species represent the population dynamics expected in a stand-replacing fire regime in a similar vegetation type for closely related shrub species with comparable lifespans and fire-related traits. H. decurrens represents a typical post-disturbance, fire-killed species with mass post-fire seedling recruitment and gradual size increase with increasing time since fire until plants begin to senesce in the long absence of fire (>25 years). H. rostrata represents a typical resprouting species that rapidly returns to pre-fire size shortly after fire and remains stable in size thereafter. Both species show little evidence of inter-fire recruitment, except in the oldest stands of H. decurrens where an increasing rate of adult plant mortality instigates seed release.
To further explore patterns in population-size distributions, we also analysed the following metrics of the size distributions for individual populations by using data for each site separately: mean size, Gini coefficient, permutation index (see below for more detail). We used generalised additive models (GAMs) by using the mgcv package in R (Wood 2017; R Core Team 2020) because data exploration indicated non-linear relationships with time since fire. Mean size (height or volume) was modelled using a gamma error distribution (log link), Gini coefficient with a beta error distribution (logit link), and permutation index with a quasi-Poisson error distribution (log link) to account for overdispersion. All models were fit with k = 3 to prevent overfitting and to create conservative, ecologically interpretable models. The time-since-fire response variable was square-root transformed because responses were expected to change most rapidly in the first few years after fire, and to optimise the distribution of values across the predictor range. Model assumptions were checked using Q–Q plots and residual plots, and no violations were observed. Mean height, Gini coefficient, and permutation index were also calculated for the model Hakea species for comparison. Interpretation of the Gini coefficient and permutation index are outlined below. All visualisations were created using the ggplot2 package in R (Wickham 2016).
The Gini coefficient provides a measure of the spread or inequality of frequency distributions (Weiner and Solbrig 1984). Values range from 0 to 1, where 0 indicates that all size classes have equal frequency (flat distribution) and 1 indicates that all observations fall within a single size class. Even-sized populations with a single post-fire cohort are expected to have higher Gini coefficient values than are mixed-sized populations with multiple cohorts. Increasing variation in size over time for a single cohort stand may also be reflected in a declining Gini coefficient over time.
The permutation index is a measure of the deviation from a reverse J-shaped (monotonically declining) distribution where the smallest size class has the highest frequency and frequency declines for each successively larger size class (Venter and Witkowski 2010). Values have a lower limit of zero, which indicates a perfect reverse J-shaped (negative exponential) distribution representing continuous recruitment and mortality. Values of >0 represent an increasing deviation from a reverse J-shaped distribution. Populations with fire-independent recruitment and mortality are expected to have low permutation index values across a range of times since fire, given continual seedling input. Populations with strongly fire-dependent recruitment and mortality (or top-kill) are instead expected to show low permutation index post-fire values (reflecting a single cohort), followed by a steady increase with increasing time since fire.
In each population, seedling recruitment was calculated as the frequency of individuals attributed as seedlings, and mortality as the frequency of standing dead plants (see definition above). Where plants were recorded in a defined area within plots (83% of populations), plant density (plants per m2) was also calculated. To assess changes in recruitment, mortality, and density relative to time since fire, we constructed GAMs as described above. Seedling recruitment and mortality were modelled using binomial error distributions (logit link), and plant density was modelled using a quasi-Poisson error distribution (log link) to account for overdispersion. Data on seedling frequency for the model Hakea species (Enright and Goldblum 1999) are presented for comparison, where seedlings are virtually absent during the inter-fire period, except for a few in the oldest sites for H. decurrens (fire-killed), which emerged from seeds released from the woody fruits of senescing adults.
Results
Size-class distributions: fire-killed species
Of the three fire-killed species, size-class distributions of the two shrubs (G. tomentosum, A. pulchella) reflected a multi-cohort pattern, whereas the tree species (B. prionotes) was largely consistent with a single-cohort pattern (Fig. 2). Both fire-killed shrub species had mostly continuous seedling recruitment during the inter-fire period, as evidenced by the high frequency of seedlings in almost all time-since-fire classes (Fig. 2a, b). Size distributions for these fire-killed shrubs were not consistent with a known reference type (H. decurrens) that exhibits generally even-sized, single-cohort, post-disturbance populations (Fig. 2d). Size-class distributions for B. prionotes (fire-killed, weakly serotinous tree) largely reflect even-sized (single, post-fire cohort) populations up to ~20 years post-fire, after which there is evidence of inter-fire recruitment cohorts and mixed-sized structures (Fig. 2c).
Size (height)-class distributions for (a, b) two fire-killed shrubs (Gompholobium tomentosum, Acacia pulchella) and (c) a fire-killed, serotinous tree (Banksia prionotes) along a fire-age chronosequence (LUB, long-unburnt, >35 years). (d) Size-class distributions for Hakea decurrens, a model fire-killed shrub with known single-cohort populations. Size-class distributions in a–c were constructed using pooled data from multiple sites (n = 2–4) in each fire-age class (except B. prionotes, 2 years, n = 1 site). White bars represent individuals identified as seedlings, and grey bars represent juvenile and adult plants. Solid lines depict size-class density distributions for the study species (blue) and the model fire-killed shrub (grey). Study species (a–c) are ordered by estimated adult lifespan, increasing from left to right. Number of individuals (n) in each distribution is given.
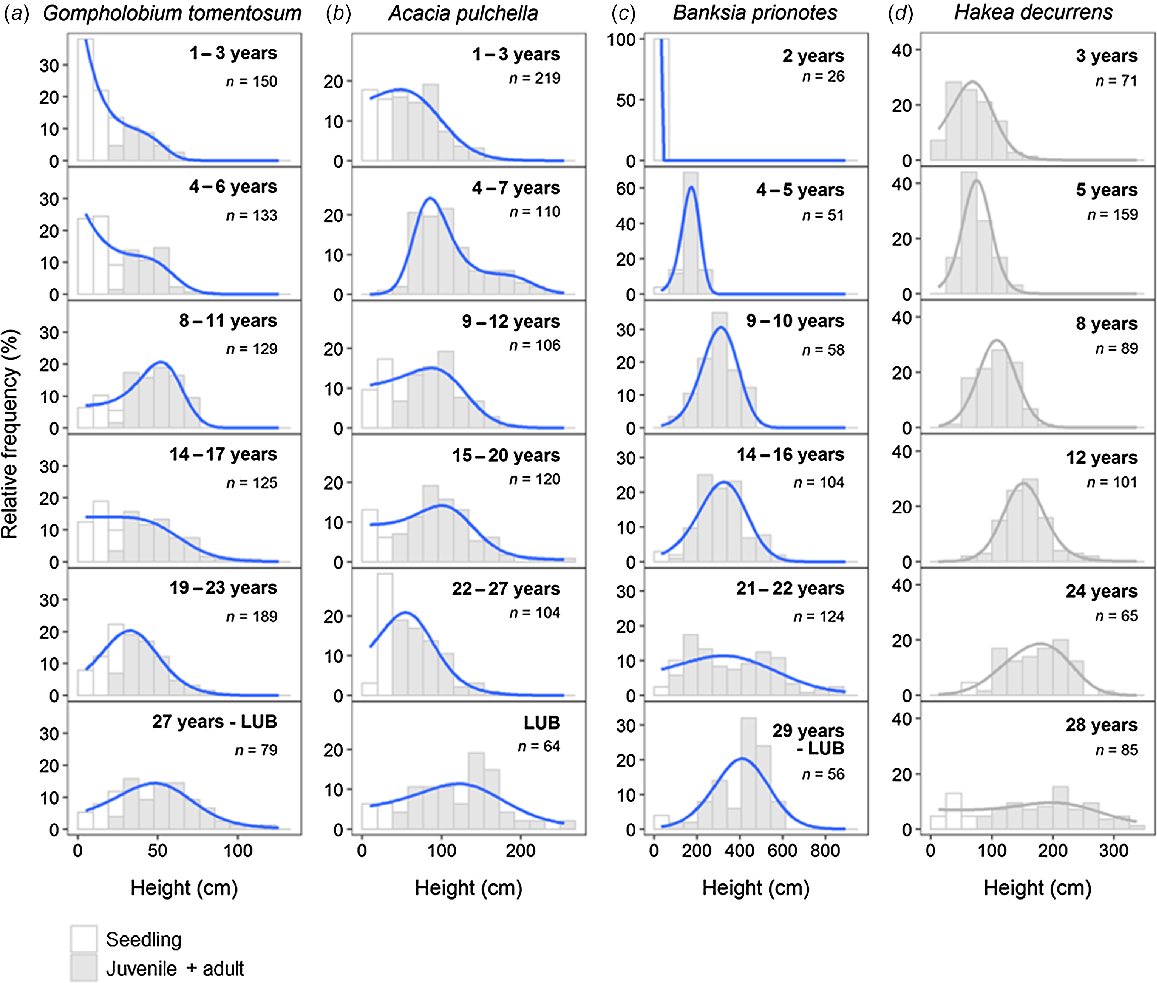
Size-class distributions: resprouters
Size-class distributions for the resprouting shrubs (H. hypericoides, A. humilis) (Fig. 3a, b) are consistent with a known resprouter reference type (H. rostrata) that illustrates a dominant post-fire resprouting cohort (Fig. 3d). Size distributions exhibit little change with an increasing time since fire, indicating that they rapidly regain their pre-fire size following fire, and have very low levels of new seedling recruitment. Resprouting shrubs showed no substantial evidence of inter-fire recruitment. In comparison, size-class distributions for B. attenuata (resprouting, weakly serotinous tree) illustrate mixed-sized (multi-cohort) stands with abundant post-fire recruitment and continuous but lower levels of inter-fire recruitment indicated by the presence of seedlings and small individuals in all fire-age classes (Fig. 3c).
Size (height or volume)-class distributions for (a, b) two resprouting shrub species (Hibbertia hypericoides, Allocasuarina humilis) and (c) a resprouting, serotinous tree species (Banksia attenuata) along a fire-age chronosequence. (d) Size-class distributions for Hakea rostrata, a model resprouting shrub (data from Enright and Goldblum 1999). Note square-root transformation of x-axes for canopy-volume distributions (H. hypericoides and A. humilis). Size-class distributions in a–c were constructed using pooled data from multiple sites (n = 2–4) in each fire-age class. White bars represent individuals identified as seedlings, and grey bars represent juvenile and adult plants. Solid lines depict size-class density distributions for the study species (blue) and the model resprouter shrub (grey). Study species (a–c) are ordered by estimated adult lifespan, increasing from left to right. Number of individuals (n) in each distribution is given.
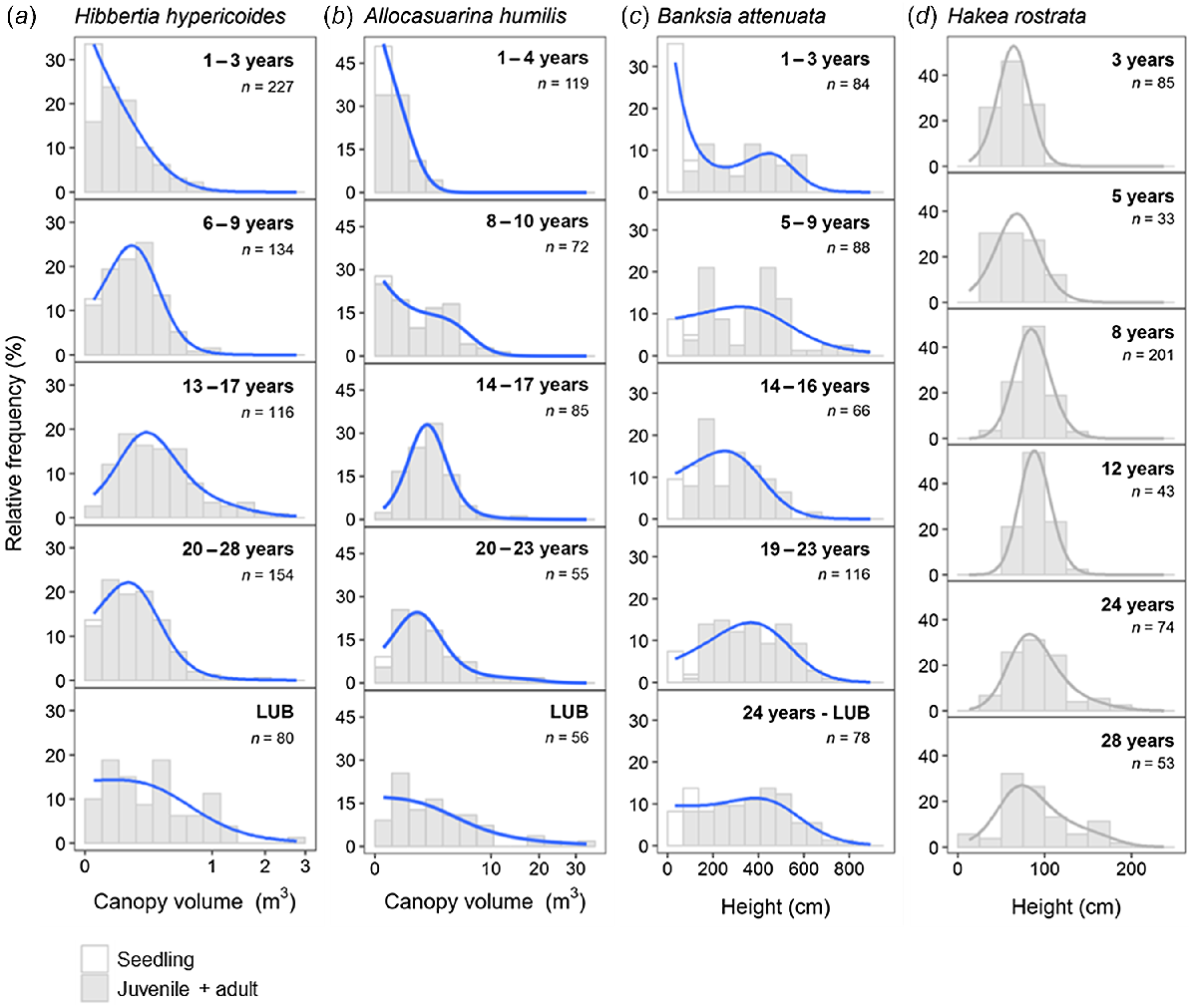
Size-distribution metrics: fire-killed species
For the fire-killed shrubs (G. tomentosum, A. pulchella), mean plant height had a weakly positive, non-significant relationship with an increasing time since fire (Fig. 4a). Mean plant height of the fire-killed tree (B. prionotes) showed a stronger, significant relationship with an increasing time since fire where mean height increased rapidly until ~15 years, stabilising at ~3.5 m thereafter, representing a similar pattern to the model fire-killed shrub (H. decurrens) (Fig. 4a).
Relationships between (a) mean plant height, (b) Gini coefficient, and (c) permutation index of size-class distributions and time (years) since fire for two fire-killed shrub species (Gompholobium tomentosum, Acacia pulchella) and a serotinous, fire-killed tree species (Banksia prionotes). Relationships for Hakea decurrens are presented as a model for fire-killed shrubs with even-sized, post-disturbance populations (data from Enright and Goldblum 1999). Data points represent values for individual sites. Coloured solid lines show generalised additive model (GAM) fits (k = 3) for (a) mean plant height (green), (b) Gini coefficient (blue), and (c) permutation index (red). Grey solid lines show respective GAM fits for the model fire-killed shrub. Shaded ribbons indicate 95% confidence intervals for each GAM fit. Significance of smooth term (P) is given for each GAM. Note different y-axis scale for mean height of each species.
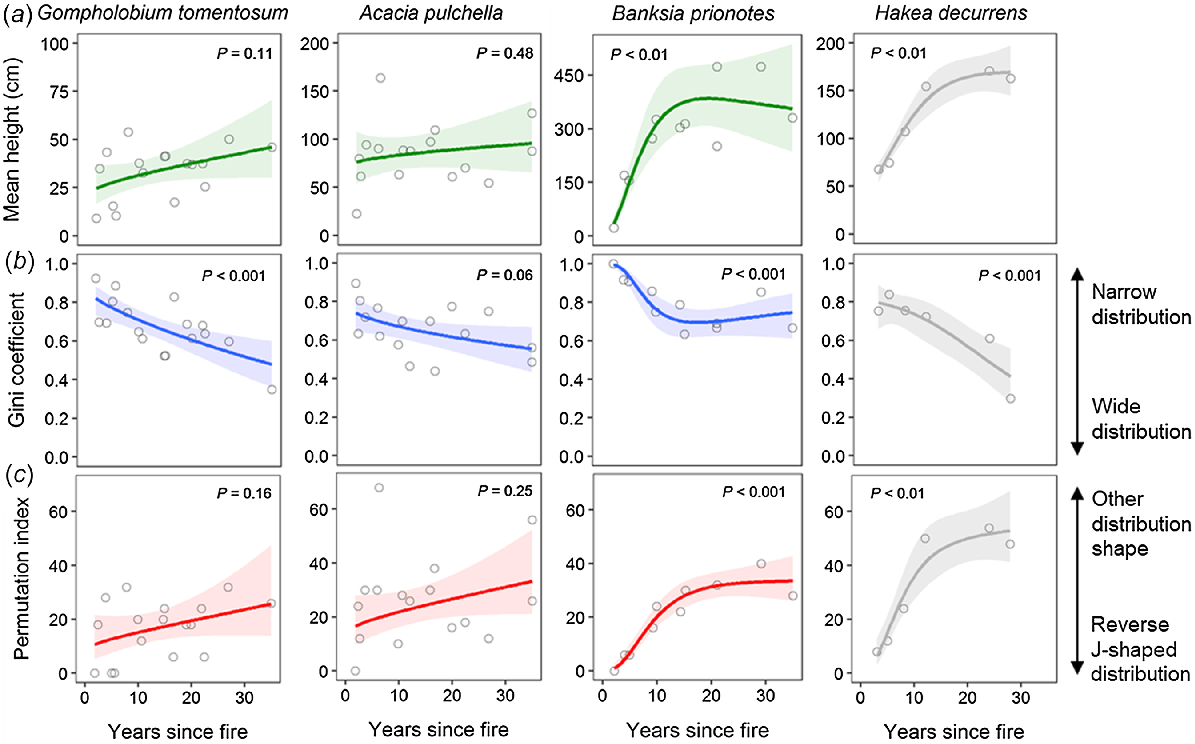
The Gini coefficient, a metric of the spread of size classes, showed a significant (or close to significant) negative relationship with an increasing time since fire for all fire-killed species (Fig. 4b). For the fire-killed shrubs, the Gini coefficient declined with time since fire, indicating a greater spread of size classes with an increasing time since fire, a pattern similar to that for H. decurrens (Fig. 4b). For B. prionotes, the Gini coefficient decreased until ~15 years since fire, before stabilising.
The permutation index, a metric of how closely a size distribution represents a reverse J-shape, showed a weakly positive, non-significant relationship with an increasing time since fire for the fire-killed shrubs (Fig. 4c). The permutation index for B. prionotes populations showed a significant relationship with time since fire, increasing up to ~15 years, then stabilising, a pattern similar to that for the model fire-killed shrub, although the permutation index for B. prionotes remained lower, indicating a distribution somewhat closer to a reverse J-shape (Fig. 4c).
Size-distribution metrics: resprouters
Mean plant canopy volume for the resprouting shrubs (H. hypericoides, A. humilis) showed a significant, positive relationship with an increasing time since fire, somewhat similar to the pattern shown by the model resprouter (H. rostrata) for plant height (Fig. 5a). Mean height of B. attenuata showed no significant relationship with time since fire (Fig. 5a).
Relationships between (a) mean plant canopy volume or height, (b) Gini coefficient, and (c) permutation index of size-class distributions and time (years) since fire for two resprouting shrub species (Hibbertia hypericoides, Allocasuarina humilis) and a resprouting, serotinous tree species (Banksia attenuata). Relationships for Hakea rostrata are presented as a model for resprouting shrubs (data from Enright and Goldblum 1999). Data points represent values for individual sites. Coloured solid lines show generalised additive model (GAM) fits (k = 3) for (a) mean plant canopy volume or height (green), (b) Gini coefficient (blue), and (c) permutation index (red). Grey solid lines show respective GAM fits for the model resprouter. Shaded ribbons indicate 95% confidence intervals for each GAM fit. Significance of smooth term (P) is given for each GAM. Note different y-axis scale for mean canopy volume or height of each species.
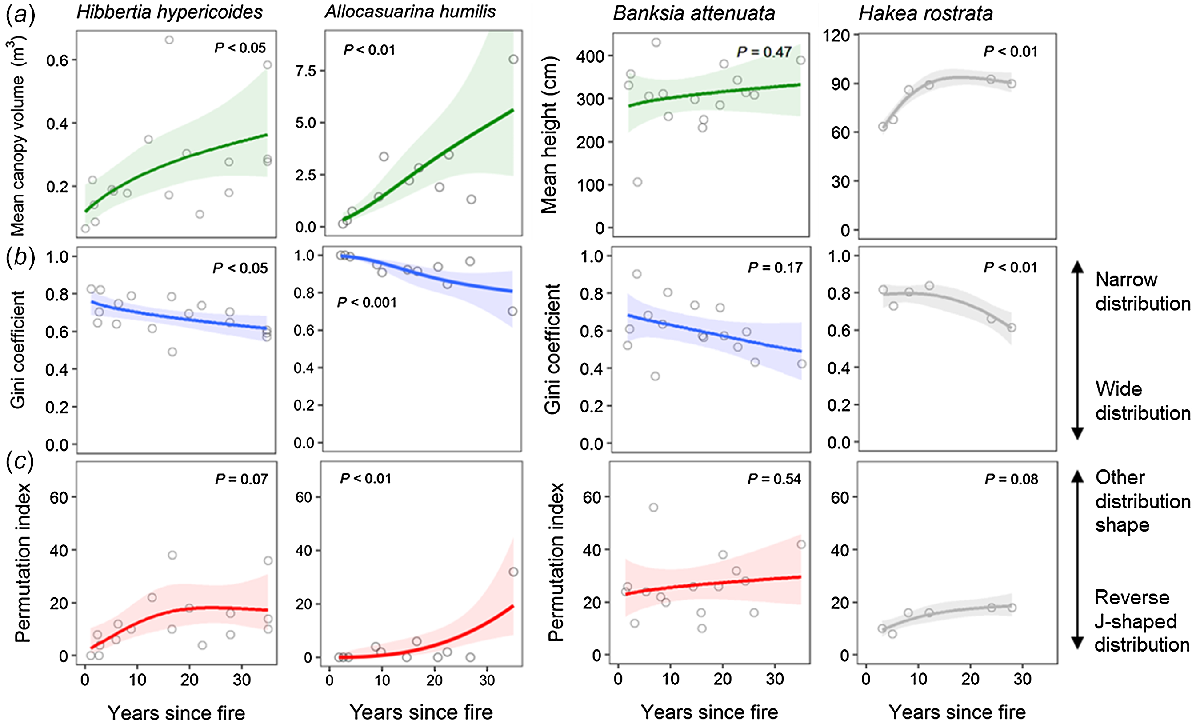
There was a weakly negative relationship between the Gini coefficient and time since fire for all resprouter species, indicating a greater spread of size classes with an increasing time since fire, a pattern similar to that for the model resprouter (Fig. 5b). Both resprouting shrubs showed a significant (or close to significant) positive relationships between the permutation index and time since fire, similar to the model resprouter (Fig. 5c). The relationship for A. humilis is dominated by a high permutation index value in the oldest site (Fig. 5c). The resprouting tree (B. attenuata) showed no significant relationship between the permutation index and time since fire, indicating little change in size distribution with time since fire (Fig. 5c).
Seedling recruitment
Seedling frequency was highest in young post-fire sites for all species, reflecting abundant post-fire recruitment (Fig. 6). However, seedlings were also present in surveyed populations at other fire ages for both fire-killed shrubs (G. tomentosum and A. pulchella) and both Banksia tree species, indicating that inter-fire recruitment is also present, and it differs from expected recruitment patterns illustrated by the model Hakea species (Fig. 6). These patterns are consistent with the evidence presented from size-class distributions. Generalised additive models for the fire-killed shrubs suggest that G. tomentosum has a continuous inter-fire recruitment, whereas A. pulchella experiences a gap in recruitment (from approximately 2 to 8 years post-fire) following the immediate post-fire recruitment pulse (Fig. 6). B. prionotes shows a similar recruitment gap for ~15 years post-fire, after which low levels of inter-fire recruitment are apparent (Fig. 6).
Relationships between seedling frequency and time since fire for three fire-killed (top row, blue) and three resprouting (bottom row, green) species. Data for Hakea decurrens (fire-killed) and H. rostrata (resprouter) are presented as expectations of recruitment patterns for these fire-response types (data from Enright and Goldblum 1999). Data points represent values for individual sites. Coloured solid lines show generalised additive model fits (k = 4), and shaded ribbons indicate 95% confidence intervals. Estimated degrees of freedom (ed.f.) and significance of smooth terms (P) are given for each generalised additive model.
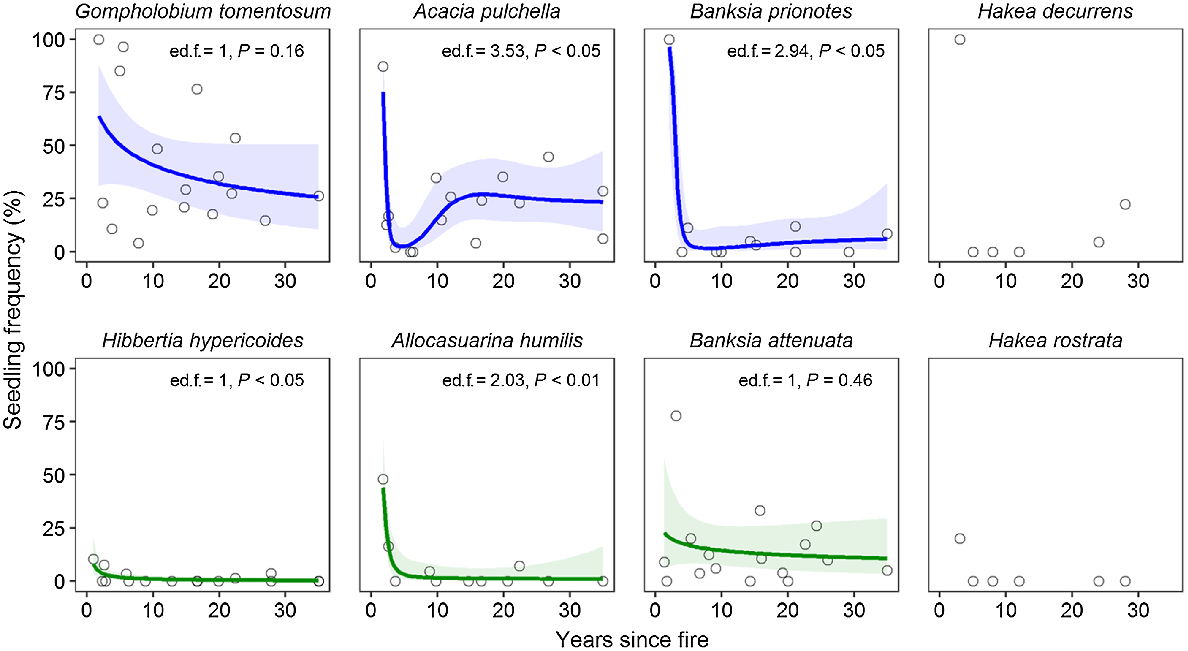
Although populations of the resprouting tree (B. attenuata) show evidence for a pulse of post-fire seedling recruitment, regeneration occurs largely through resprouting, and, so, seedling frequency is low compared with the fire-killed species (Fig. 6). However, like the fire-killed tree (B. prionotes), B. attenuata also has low to intermediate levels of recruitment during the inter-fire period (Fig. 6). The two resprouting shrubs also readily survive and resprout after fire and appear to have low to intermediate levels of post-fire recruitment; however, both species lack evidence for ongoing inter-fire recruitment (Fig. 6).
Plant mortality
Recent mortality, as indicated by the frequency of standing dead plants, remains low in most species regardless of time since fire, although there was weak evidence for an increase in mortality in longer-unburnt sites for A. pulchella and B. prionotes (Fig. 7). No inter-fire mortality was recorded for H. hypericoides.
Relationships between the frequency of standing dead plants (recent mortality) and time since fire for three fire-killed (top row, blue) and three resprouting (bottom row, green) species. Data points represent values for individual sites. Coloured solid lines show generalised additive model fits (k = 3), and shaded ribbons indicate 95% confidence intervals. Estimated degrees of freedom (ed.f.) and significance of smooth terms (P) are given for each generalised additive model.
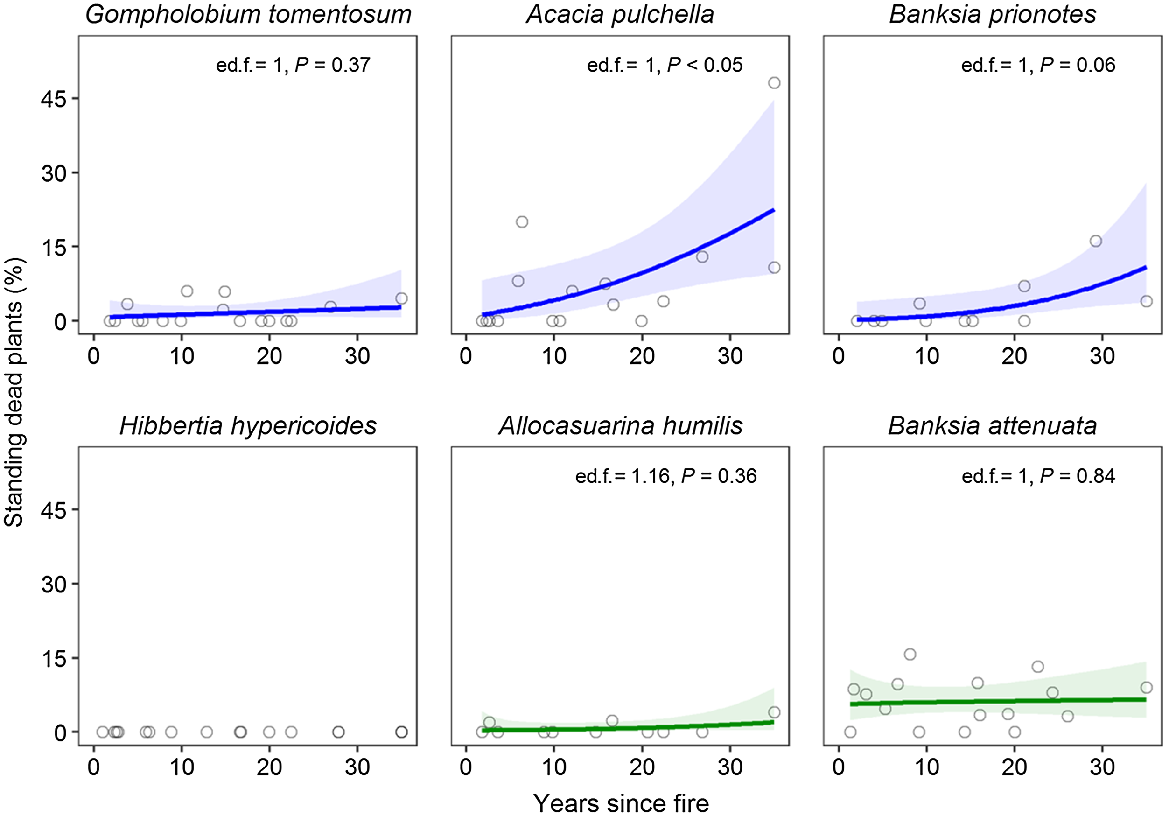
Plant density
The relationship between plant density and time since fire was strongest for G. tomentosum where density declined rapidly up to 10 years since fire, remaining stable thereafter (Fig. 8). There was weak evidence that populations of the other fire-killed shrub (A. pulchella) follow a similar pattern, although there was greater variation at younger sites (Fig. 8). Plant density in B. prionotes populations appeared to decline in longer-unburnt sites, even when disregarding outlying, high-density sites approximately 15–20 years since fire (Fig. 8). There was little evidence for substantial density decline in long-unburnt populations of resprouter species (Fig. 8).
Relationships between plant density (plants m−2) and time since fire for three fire-killed (top row, blue) and three resprouting (bottom row, green) species. Data points represent values for individual sites. Coloured solid lines show generalised additive model fits (k = 3), and shaded ribbons indicate 95% confidence intervals. Estimated degrees of freedom (ed.f.) and significance of smooth terms (P) are given for each generalised additive model. For Allocasuarina humilis, only a few sites had plot-based density measurements and hence the relationship is not modelled.
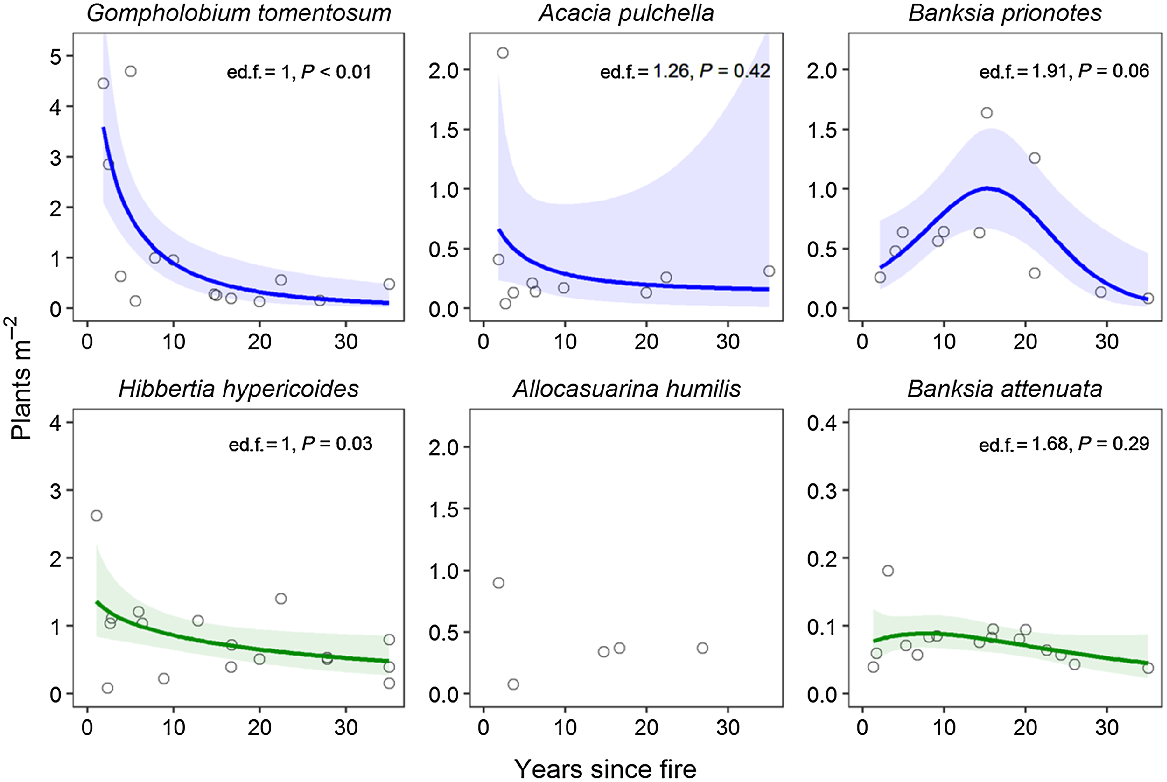
Discussion
We used static demographic data to quantify fire-related patterns in cohort structure, seedling recruitment, and plant mortality across six co-occurring woody plant species differing in life-history traits from fire-prone Banksia Woodlands in south-western Australia and identified the following life-history types: (1) short-lived, fire-killed shrubs with abundant post-fire and inter-fire seedling recruitment; (2) long-lived, resprouting shrubs with little seedling recruitment; and (3) trees (fire-killed or resprouting) with elevated post-fire seedling recruitment and low to moderate levels of inter-fire recruitment. The co-occurrence of these three broad life-history strategies, particularly the combination of strong post-fire responses (resprouting or seedling recruitment) with inter-fire seedling recruitment and multi-cohort populations, is incompatible with surface or stand-replacing fire regimes in a Mediterranean-type climate region, and instead reflects a historical mixed-severity fire regime across our study sites characterised by high variance in fire interval and severity within and between fires. In addition to gaining an insight into historical fire-regime adaptations, classifications of life-history strategies based on empirical demographic data and species traits can enable better prediction of species responses under future fire-regime scenarios, including changes in fire interval and severity.
Life-history strategies
Cohort structures and seedling recruitment patterns varied across the six species studied here, with a broad distinction between life forms (shrub vs tree) and fire-response types (fire-killed vs resprouting). Short-lived, fire-killed shrubs (A. pulchella and G. tomentosum) had abundant post-fire recruitment developing into strongly multi-cohort populations with mostly continuous recruitment throughout the inter-fire period. In contrast, long-lived, resprouting shrubs (A. humilis and H. hypericoides) had little evidence of recruitment or mortality at any time and cohort structure varied little after initial post-fire recovery (Baird 1977). Both weakly serotinous Banksia tree species had low to moderate levels of recruitment in the absence of fire, and cohort structures were somewhat distinguished by fire response; post-fire, single-cohort populations of B. prionotes (fire-killed) developed into multi-cohort populations in the longer absence of fire (>20 years), whereas the resprouting B. attenuata maintained multi-cohort populations regardless of time since fire.
Estimates of plant longevity (Table 2) were consistent with observed population dynamics and appear to be broadly accurate in the absence of more detailed investigations. Fire-killed shrubs reached their maximum size in the first few years after fire and plant density declined rapidly over this time as well. Monk et al. (1981) estimated that most individuals of A. pulchella live for less than 6–7 years and only the longest-lived individuals could exceed 13 years of age. Intense competition among young plants in the first few years after fire is likely to limit the lifespan of most individuals and our estimate of a 10–15-year lifespan for A. pulchella may, therefore, represent the longest-lived plants, with most individuals living for only 5–10 years. Mortality was difficult to assess for G. tomentosum, with skeletons of dead plants likely to be degrading rapidly after death and largely avoiding detection during our surveys. Regardless, our initial estimate of a ~5-year lifespan for G. tomentosum appears to be broadly accurate. B. prionotes showed some evidence for a slight increase in mortality in the oldest sites (>35 years), and, therefore, our initial estimate of a 40–60-year lifespan appears reasonable and is consistent with estimates for other fire-killed Banksia spp. in the region (Enright et al. 1998; Wooller et al. 2002). There was little evidence of age-related mortality for the resprouting species studied here, and our estimates of longevity (80–100 years for A. humilis and H. hypericoides, and >100 years for B. attenuata) are perhaps an underestimate because comparable resprouting shrubs may live up to 300 years (Enright and Lamont 1992).
Whereas seedling recruitment was found to be most abundant during the immediate post-fire period, inter-fire recruitment appears to be important for the persistence of short-lived shrubs and weakly serotinous trees under fire intervals approaching or beyond adult lifespans. In sites older than 15–20 years since fire, populations of short-lived, fire-killed shrubs may comprise entirely inter-fire recruits because these ages exceed their estimated post-fire adult lifespan of 5–15 years. Although inter-fire recruitment is also evident in both weakly serotinous Banksia tree species, even the oldest sites sampled here (>35 years old) probably comprise mostly individuals from the post-fire resprouting or seedling cohorts, given that these ages are within estimated adult lifespans of 40–100+ years. In some ecosystems, the long absence of fire may correlate with canopy closure, or the invasion of fire-sensitive species that may fill vegetation gaps and preclude inter-fire recruitment opportunities for gap-recruiting species (Ooi et al. 2006). The woodlands studied here generally remain open (low canopy cover) even in the long absence of fire (Tangney et al. 2022), but local variations in canopy cover have the potential to alter inter-fire recruitment success and therefore the aboveground persistence of short-lived species in the long absence of fire.
Multi-cohort populations and inter-fire recruitment are not often documented in fire-prone vegetation in Australia (Zammit and Westoby 1987; Witkowski et al. 1991; Ooi et al. 2006; Barrett and Cochrane 2007). However, such patterns of recruitment and population structure are consistent with the weakly serotinous nature of both Banksia tree species studied here (Cowling and Lamont 1985; Hobbs and Atkins 1990). Cowling and Lamont (1985) showed that populations of serotinous Banksia species at the arid end of a climate gradient in south-western Australia grow as shrubs and are strongly serotinous (i.e. they retain seeds within woody fruits for 5–10 years), whereas populations of the same species at the mesic end of this gradient, corresponding to the woodlands studied here, grow as trees and are weakly serotinous (i.e. they retain seeds for only 1–3 years). Similar patterns in cohort structure and inter-fire recruitment have been shown for other weakly serotinous tree populations in fire-prone ecosystems in Australia (Whelan et al. 1998; Ladd et al. 2022). Further work is required to quantify co-variation in recruitment and serotiny within fire-prone woodland vegetation types.
Whereas inter-fire recruitment in weakly serotinous species is consistent with expectations, inter-fire recruitment in species with soil seed banks of physically dormant seeds, such as our study species A. pulchella and G. tomentosum, is rare and largely unexpected in fire-prone ecosystems. Seeds with physical dormancy often require a heat shock to alleviate dormancy and allow germination (Auld and O’Connell 1991), and some species (especially Acacia sp.) are considered to have obligate pyrogenic dormancy release where the temperature threshold required for dormancy break (generally 80–100°C) is experienced only during fire (Ooi et al. 2014). However, other species with facultative pyrogenic dormancy release have lower dormancy-break temperature thresholds, which may be experienced on the soil surface over summer, facilitating germination for at least part of the seed bank in the absence of fire (Orscheg and Enright 2011; Liyanage and Ooi 2015). Some seed batches may also produce non-dormant seeds, or seeds may lose dormancy or have reduced dormancy-break temperature thresholds as they age (Liyanage and Ooi 2017). Species with soil-stored seed banks occur across a wide range of environments with potentially different fire regimes, and there is some evidence that dormancy-alleviating thresholds vary within and among populations, analogous to varying degrees of serotiny (Hudson et al. 2015; Liyanage and Ooi 2015; Zomer et al. 2022). Inter-fire recruitment in Leucopogon (Ericaceae) and Acacia (Fabaceae) shrubs with soil-stored seed has been documented in fire-prone woodlands and forests in south-eastern Australia, but is largely undocumented for other species groups (Hazard and Parsons 1977; Ooi et al. 2006; Franco and Morgan 2007).
Fire-regime adaptations
The combination of life-history strategies observed for shrub and tree species align with our expectations for a mixed-severity fire regime and reflect adaptations to high variance in fire intervals (Table 1). Multi-cohort populations indicate that recruitment is not limited to the post-fire environment, as would be expected in crown-fire (stand-replacing) ecosystems (Clarke et al. 2015). Whereas multi-cohort tree populations may indicate an adaptation to frequent, surface or low-severity fire regimes where fire cues rarely reach the canopy, fuel-accumulation patterns in the study area do not reflect a regime of frequent fires, and fire intervals less than 10 years are rare in the study area (Wilson et al. 2014; Tangney et al. 2022). Personal observations of the fire-killed tree species studied here (B. prionotes) indicated that mature trees can survive low-severity fire, although this is uncommon and unlikely to be a dominant mode of persistence. Indeed, all post-fire (post-wildfire) populations of B. prionotes were single-cohort, indicating a strong response to canopy fire. In addition, shrub populations in frequent, surface-fire regimes would be expected to be single-cohort, given short fire-return intervals; however, shrub populations studied here were also observed to be multi-cohort. The logical conclusion from these multiple lines of evidence is that the historical fire regime was mixed-severity with high variance in patch-scale lethal fire intervals, including intervals that exceeded the lifespan of post-fire cohorts and their seed banks, particularly for shrubs.
High variance in lethal fire intervals is considered a key selective pressure resulting in weak serotiny, inter-fire recruitment, and multi-cohort populations (Tonnabel et al. 2012; Hernández-Serrano et al. 2013; Lamont et al. 2020). These linked demographic behaviours reflect a bet-hedging strategy of fire-independent recruitment to spread the risk of senescence and recruitment failure posed by fire regimes in which suitable post-fire germination and recruitment opportunities may be absent or insufficient within adult and seed-bank lifespans (Enright et al. 1998; Lamont et al. 2020). Senescence generally poses a greater risk for fire-killed species, which are, by definition, shorter-lived than are co-occurring resprouting species. Adult lifespans for the species studied here may range from 5 to 10 years for fire-killed shrubs (Monk et al. 1981) and potentially 40–60 years for fire-killed trees, whereas resprouting shrubs and trees may live for 80–100 years or longer, reducing their reliance on regular recruitment. Fire-killed shrub populations were recorded well beyond 10 years since fire, and these fire ages are not uncommon in Banksia Woodlands (Wilson et al. 2014; Tangney et al. 2022). While we could not confirm that any sites exceeded the estimated lifespan for the focal fire-killed tree species (B. prionotes), there was weak evidence of increased mortality in the oldest sites (30+ years) and ongoing recruitment in the absence of fire, albeit at lower rates than post-fire.
Patterns of recruitment and estimated adult lifespans provide a basis for estimating the range of historical fire intervals that are compatible with observed demographic behaviour (Gosper et al. 2013; Gosper et al. 2022). Inter-fire recruitment was recorded for shrub and tree species, and for this strategy to be adaptive, an evolutionarily significant proportion of fire intervals must exceed adult and seed-bank longevity (Lamont et al. 2020). Given that fire-killed shrubs can be readily killed by even low severity fire, it is likely that median historical understorey-fire intervals exceeded the lifespan of fire-killed shrubs (5–10 years). An upper limit of understorey-fire intervals is likely to correspond to the lifespan of resprouting shrubs (80–100 years or more) because a lack of inter-fire recruitment in these species limits their capacity to persist under longer fire intervals. Upper limits are indeed probably much shorter, given that lignotuber vigour may decline with age such that even long fire intervals within resprouter shrub lifespan may increase resprouter plant mortality (Enright et al. 2011). Inter-fire recruitment for both Banksia tree species suggests that median historical intervals of canopy fire (as part of a mixed severity fire regime) exceeded fire-killed adult lifespans (40–60 years) and potentially resprouting adult lifespans (>100 years) for such a strategy to be adaptive. In the adjacent northern jarrah forest (dry sclerophyll forest), mean intervals between high-severity (stand-replacing) fires have been estimated at approximately 80 years (Burrows et al. 1995). Most of our study species also occur in these forests, although they are concentrated in discrete patches of sandy soils, potentially showing varying dynamics to the forest matrix they are embedded within. The historical fire intervals suggested by the demographic evidence presented here reflect lethal fire intervals, given the strong evolutionary pressures involved; however, this does not necessarily preclude the historical occurrence of frequent, non-lethal (very low intensity) or patchy fires. In fact, spatial fuel patterns in these woodlands (Tangney et al. 2022) could facilitate such fire regimes where non-lethal, patchy fires could lead to longer fire-free periods in unburnt patches and the development of the life-history strategies observed here.
An apparent gap in recruitment following the post-fire recruitment phase for fire-killed species may provide an insight into minimum tolerable fire intervals for the persistence of populations. The lower bounds of tolerable lethal fire intervals are commonly defined as the time required for populations to gain reproductive maturity (Burrows et al. 2008), but in the absence of reproductive data, the minimum fire age at which fire-killed shrubs have evidence of inter-fire recruitment may indicate maturity. Following a post-fire recruitment pulse, both G. tomentosum and A. pulchella appear to have a gap in recruitment of approximately 4–8 years, which is especially evident in the generalised additive model for A. pulchella (Fig. 6). If a lack of recruitment at this time reflects a lack of a viable seed bank, then lethal fire intervals shorter than 8 years may cause population decline. However, soil seed banks, such as those possessed by G. tomentosum and A. pulchella, may not be completely depleted by fire, and a residual seed bank may buffer populations from local extinction in the first few years after fire (Auld and Denham 2006). Residual seed banks are also dependent on fire intensity such that high fire intensity can greatly reduce residual seed-bank size and potentially expose populations to immaturity risk (Palmer et al. 2018). Alternatively, this gap in recruitment of approximately 4–8 years could reflect that all seeds were exhausted (germinated or decayed) from the previous fire event, and then the new seed bank deposited by plants that have grown since the last fire mostly comprises young seeds that have a high dormancy rate and high dormancy-breaking temperature thresholds. Until these seed banks age further, the young seeds would be likely to germinate only following another fire event and not summer soil temperatures, which can trigger germination in older seeds in the absence of fire (Liyanage and Ooi 2017).
Management implications
The evidence presented here suggests that historical fire regimes to which a set of dominant banksia woodland plant species are adapted were of mixed severity with highly variable fire intervals. All species showed a strong fire response via resprouting or post-fire seedling recruitment, with the shrub species displaying little difference in size distributions with longer-unburnt populations, suggesting rapid post-fire growth and return to pre-fire size. Our observations are consistent with studies of post-fire juvenile periods in Banksia Woodlands and adjacent forests, which show that many species gain (or re-gain) reproductive maturity within 3–5 years after fire (Burrows et al. 2008; Wilson et al. 2014). Fuels also rapidly accumulate in the first 5 years after fire in Banksia Woodlands, where total fine fuels in 5-year-old vegetation (5–7 t ha−1) are sufficient to support fire spread (Tangney et al. 2022). Together, these multiple lines of evidence suggest that an historical mixed-severity fire regime could have included occasional short 5–10-year understorey-fire intervals, and that many common banksia woodland plants are likely to be tolerant of such intervals. Rare, threatened, or restricted species may require longer fire intervals for persistence, and species-specific fire-regime requirements should be quantified in these cases.
Our data also suggested that historical fire regimes included a range of longer fire intervals, specifically intermediate understorey-fire intervals that exceeded the lifespan of fire-killed shrubs (~5–10 years) and long crown-fire intervals that exceeded the lifespan of fire-killed trees (40–60 years). The absence of fire beyond the lifespan of fire-dependent species would normally be a cause for concern; however, the inter-fire recruitment documented for our study species, and longer-lived seed banks for some, may allow populations to persist for longer than expected where open vegetation structure allows inter-fire recruitment opportunities. As part of a mixed-severity fire regime, long intervals between canopy fires may have been common historically and may be critical for the development of slow-maturing canopy species and faunal food resources (Valentine et al. 2014). Long canopy (crown) fire-free intervals (>20–25 years) are known to be important for canopy seed-bank dynamics of dominant Banksia tree species in the region, providing critical food resources for the endangered Carnaby’s cockatoo (Calyptorhynchus (Zanda) latirostris) (Valentine et al. 2014). Current management objectives of applying low-intensity prescribed burning in Banksia Woodlands that largely affects only the understorey provides an important tool to prolong intervals between canopy fires (Densmore and Clingan 2019).
Long fire-free periods also appear to be significant in evolutionary terms for plant and animal species in the understorey. The shrub populations studied here show an ability to persist in the absence of fire beyond 20 years, and previous work on reptiles and amphibians has indicated that some species prefer longer-unburnt vegetation (>12 years) (Bamford 1992; Valentine et al. 2012; Davis and Doherty 2015). For any patch of vegetation, long fire-free periods in the understorey could arise through the absence of fire at a broad scale, or by being located within a matrix of more frequent, patchy burns and therefore potentially leaving other patches unburnt over multiple fire cycles. Banksia woodland fuels rapidly accumulate in the first 10–20 years after fire but remain generally stable thereafter (Tangney et al. 2022), meaning that fire risk does not necessarily continue to increase in the long absence of fire. Instead, occasional areas of long-unburnt understory vegetation within patchy burns or at a larger scale can be retained to provide benefits for fire-sensitive plant or animal species, without posing an unreasonably high fire or plant-senescence risk. The demographic data and sampling design used in this study targeted fire-interval responses, but further investigations of species responses to varying fire size and patchiness are also needed to extend our understanding of fire-regime tolerances. Fire size and patchiness also have important implications for understanding pre-colonial Indigenous burning and informing efforts to reinstate Indigenous-led fire management.
Conclusions
We identified three varied plant life-history strategies in co-occurring shrub and tree species, the combination of which follows expectations of a mixed-severity fire regime. In particular, the combination of strong post-fire resprouting and seedling recruitment with weak serotiny, inter-fire recruitment, and multi-cohort populations is associated with high variance in fire interval and severity over evolutionary timescales. It is likely that historical fire regimes involved a mix of short to long fire intervals and mixed fire severity where understorey-fire intervals were shorter than canopy-fire intervals. Varying fire severity within and among fires appears to be an important factor in the fire-regime adaptations of our study species. Inter-fire recruitment was common among fire-killed and weakly serotinous species, providing an insurance against recruitment failure when lethal fire intervals exceed adult and seed-bank lifespans. This study demonstrated the use of demographic and trait data to explore life-history adaptations as a signature of past fire regimes.
Declaration of funding
This research was supported by an Australian Research Council Linkage grant (LP160100996) to NJE, JBF, and BPM.
Acknowledgements
We thank the undergraduate students who assisted with fieldwork. We acknowledge the Traditional Owners of country throughout Western Australia and their continuing connection to land, sea, and community. We pay our respects to them, their culture, and to their Elders past and present.
References
Agee JK (2005) The complex nature of mixed severity fire regimes. In ‘Mixed severity fire regimes: ecology and management, Vol. AFE MISC03’. (Eds L Lagene, J Zelnik, S Cadwallader, B Hughes) pp. 1–10. (Washington State University Cooperative Extension Service/The Association for Fire Ecology: Spokane, WA, USA)
Agne MC, Fontaine JB, Enright NJ, Bisbing SM, Harvey BJ (2022) Demographic processes underpinning post-fire resilience in California closed-cone pine forests: the importance of fire interval, stand structure, and climate. Plant Ecology 223, 751-767.
| Crossref | Google Scholar |
Archibald S, Lehmann CER, Gómez-Dans JL, Bradstock RA (2013) Defining pyromes and global syndromes of fire regimes. Proceedings of the National Academy of Sciences 110, 6442-6447.
| Crossref | Google Scholar |
Armstrong G, Phillips B (2012) Fire history from life-history: determining the fire regime that a plant community is adapted using life-histories. PLoS ONE 7, e31544.
| Crossref | Google Scholar | PubMed |
Ashton DH (1976) The development of even-aged stands of Eucalyptus regnans F.Muell. in central Victoria. Australian Journal of Botany 24, 397-414.
| Crossref | Google Scholar |
Auld TD, Denham AJ (2006) How much seed remains in the soil after a fire? Plant Ecology 187, 15-24.
| Crossref | Google Scholar |
Auld TD, O’Connell MA (1991) Predicting patterns of post-fire germination in 35 eastern Australian Fabaceae. Australian Journal of Ecology 16, 53-70.
| Crossref | Google Scholar |
Auld TD, Denham AJ, Turner K (2007) Dispersal and recruitment dynamics in the fleshy-fruited Persoonia lanceolata (Proteaceae). Journal of Vegetation Science 18, 903-910.
| Crossref | Google Scholar |
Baird AM (1977) Regeneration after fire in King’s Park, Perth, Western Australia. Journal of the Royal Society of Western Australia 60, 1-22.
| Google Scholar |
Bamford MJ (1992) The impact of fire and increasing time after fire upon Heleioporus eyrei, Limnodynastes dorsalis and Myobatrachus gouldii (Anura: Leptodactylidae) in Banksia Woodland near Perth, Western Australia. Wildlife Research 19, 169-178.
| Crossref | Google Scholar |
Barrett S, Cochrane A (2007) Population demography and seed bank dynamics of the threatened obligate seeding shrub Grevillea maxwellii McGill (Proteaceae). Journal of the Royal Society of Western Australia 90, 165-174.
| Google Scholar |
Burrows ND, McCaw WL (1990) Fuel characteristics and bushfire control in banksia low woodlands in Western Australia. Journal of Environmental Management 31, 229-236.
| Crossref | Google Scholar |
Burrows ND, Ward B, Robinson AD (1995) Jarrah forest fire history from stem analysis and anthropological evidence. Australian Forestry 58, 7-16.
| Crossref | Google Scholar |
Burrows ND, Wardell-Johnson G, Ward B (2008) Post-fire juvenile period of plants in south-west Australia forests and implications for fire management. Journal of the Royal Society of Western Australia 91, 163-174.
| Google Scholar |
Callister KE, Griffioen PA, Avitabile SC, et al. (2016) Historical maps from modern images: using remote sensing to model and map century-long vegetation change in a fire-prone region. PLoS ONE 11, e0150808.
| Crossref | Google Scholar | PubMed |
Campbell ML, Clarke PJ, Keith DA (2012) Seed traits and seed bank longevity of wet sclerophyll forest shrubs. Australian Journal of Botany 60, 96-103.
| Crossref | Google Scholar |
Clarke PJ, Lawes MJ, Murphy BP, et al. (2015) A synthesis of postfire recovery traits of woody plants in Australian ecosystems. Science of The Total Environment 534, 31-42.
| Crossref | Google Scholar | PubMed |
Conedera M, Tinner W, Neff C, Meurer M, Dickens AF, Krebs P (2009) Reconstructing past fire regimes: methods, applications, and relevance to fire management and conservation. Quaternary Science Reviews 28, 555-576.
| Crossref | Google Scholar |
Cowling RM, Lamont BB (1985) Variation in serotiny of three Banksia species along a climatic gradient. Australian Journal of Ecology 10, 345-350.
| Crossref | Google Scholar |
Cowling RM, Lamont BB (1987) Post-fire recruitment of four co-occurring Banksia species. Journal of Applied Ecology 24, 645-658.
| Crossref | Google Scholar |
Davis RA, Doherty TS (2015) Rapid recovery of an urban remnant reptile community following summer wildfire. PLoS ONE 10, e0127925.
| Crossref | Google Scholar | PubMed |
de Gouvenain RC, Delgadillo J (2012) Geographical variation in population demography and life history traits of Tecate cypress (Hesperocyparis forbesii) suggests a fire regime gradient across the USA-Mexico border. Plant Ecology 213, 723-733.
| Crossref | Google Scholar |
de Gouvenain RC, Midgley JJ, Merow C (2019) Serotiny in the South African shrub Protea repens is associated with gradients of precipitation, temperature, and fire intensity. Plant Ecology 220, 97-109.
| Crossref | Google Scholar |
Densmore VS, Clingan ES (2019) Prescribed burning in a mediterranean-climate region mitigates the disturbance by bushfire to a critical food resource for an endangered bird, the Carnaby’s cockatoo. Fire Ecology 15, 36.
| Crossref | Google Scholar |
Dodd J, Griffin EA (1989) Floristics of the Banksia woodlands. Journal of the Royal Society of Western Australia 71, 89-90.
| Google Scholar |
Enright NJ, Goldblum D (1999) Demography of a non-sprouting and resprouting Hakea species (Proteaceae) in fire-prone Eucalyptus woodlands of southeastern Australia in relation to stand age, drought and disease. Plant Ecology 144, 71-82.
| Crossref | Google Scholar |
Enright NJ, Lamont BB (1992) Recruitment variability in the resprouting shrub Banksia attenuata and non-sprouting congeners in the northern sandplain heaths of southwestern Australia. Acta Oecologica 13, 727-741.
| Google Scholar |
Enright NJ, Thomas I (2008) Pre-European fire regimes in Australian ecosystems. Geography Compass 2, 979-1011.
| Crossref | Google Scholar |
Enright NJ, Lamont BB, Marsula R (1996) Canopy seed bank dynamics and optimum fire regime for the highly serotinous shrub, Banksia hookeriana. Journal of Ecology 84, 9-17.
| Crossref | Google Scholar |
Enright NJ, Marsula R, Lamont BB, Wissel C (1998) The ecological significance of canopy seed storage in fire-prone environments: a model for non-sprouting shrubs. Journal of Ecology 86, 946-959.
| Crossref | Google Scholar |
Enright NJ, Fontaine JB, Westcott VC, Lade JC, Miller BP (2011) Fire interval effects on persistence of resprouter species in Mediterranean-type shrublands. Plant Ecology 212, 2071-2083.
| Crossref | Google Scholar |
Enright NJ, Keith DA, Clarke MF, Miller BP (2012) Fire regimes in Australian sclerophyllous shrubby ecosystems: heathlands, heathy woodlands and mallee woodlands. In ‘Flammable Australia: fire regimes, biodiversity and ecosystems in a changing world’. (Eds RA Bradstock, AM Gill, RJ Williams) pp. 215–234. (CSIRO Publishing: Melbourne, Vic, Australia)
Fowler WM, Standish RJ, Enright NJ, Fontaine JB (2023) Extinction debt varies in two threatened Mediterranean-type woodland communities undergoing rapid urbanisation. Australian Journal of Botany 71, 421-433.
| Crossref | Google Scholar |
Franco JA, Morgan JW (2007) Using historical records, aerial photography and dendroecological methods to determine vegetation changes in a grassy woodland since European settlement. Australian Journal of Botany 55, 1-9.
| Crossref | Google Scholar |
Franco M, Silvertown J (1996) Life history variation in plants: an exploration of the fast-slow continuum hypothesis. Philosophical Transactions of the Royal Society of London. Series B: Biological Sciences 351, 1341-1348.
| Crossref | Google Scholar |
Gauthier S, Gagnon J, Bergeron Y (1993) Population age structure of Pinus banksiana at the southern edge of the Canadian boreal forest. Journal of Vegetation Science 4, 783-790.
| Crossref | Google Scholar |
Gauthier S, Bergeron Y, Simon J-P (1996) Effects of fire regime on the serotiny level of jack pine. Journal of Ecology 84, 539-548.
| Crossref | Google Scholar |
Gosper CR, Prober SM, Yates CJ (2013) Estimating fire interval bounds using vital attributes: implications of uncertainty and among-population variability. Ecological Applications 23, 924-935.
| Crossref | Google Scholar | PubMed |
Gosper CR, Miller BP, Gallagher RV, et al. (2022) Mapping risk to plant populations from short fire intervals via relationships between maturation period and environmental productivity. Plant Ecology 223, 769-787.
| Crossref | Google Scholar |
Halofsky JE, Donato DC, Hibbs DE, et al. (2011) Mixed-severity fire regimes: lessons and hypotheses from the Klamath–Siskiyou Ecoregion. Ecosphere 2, 1-19.
| Crossref | Google Scholar |
Hazard J, Parsons RF (1977) Size-class analysis of coastal scrub and woodland, Western Port, southern Australia. Australian Journal of Ecology 2, 187-197.
| Crossref | Google Scholar |
Hernández-Serrano A, Verdú M, González-Martínez SC, Pausas JG (2013) Fire structures pine serotiny at different scales. American Journal of Botany 100, 2349-2356.
| Crossref | Google Scholar | PubMed |
Hidayati SN, Walck JL, Merritt DJ, Turner SR, Turner DW, Dixon KW (2012) Sympatric species of Hibbertia (Dilleniaceae) vary in dormancy break and germination requirements: implications for classifying morphophysiological dormancy in Mediterranean biomes. Annals of Botany 109, 1111-1123.
| Crossref | Google Scholar | PubMed |
Hobbs RJ, Atkins L (1990) Fire related dynamics of a Banksia woodland in south-western Western Australia. Australian Journal of Botany 38, 97-110.
| Crossref | Google Scholar |
Howard T, Burrows N, Smith T, Daniel G, McCaw L (2020) A framework for prioritising prescribed burning on public land in Western Australia. International Journal of Wildland Fire 29, 314-325.
| Crossref | Google Scholar |
Hudson AR, Ayre DJ, Ooi MKJ (2015) Physical dormancy in a changing climate. Seed Science Research 25, 66-81.
| Crossref | Google Scholar |
Johnstone JF, Allen CD, Franklin JF, et al. (2016) Changing disturbance regimes, ecological memory, and forest resilience. Frontiers in Ecology and the Environment 14, 369-378.
| Crossref | Google Scholar |
Keeley JE (2012) Ecology and evolution of pine life histories. Annals of Forest Science 69, 445-453.
| Crossref | Google Scholar |
Keeley JE, Pausas JG (2022) Evolutionary ecology of fire. Annual Review of Ecology, Evolution, and Systematics 53, 203-225.
| Crossref | Google Scholar |
Keeley JE, Fotheringham CJ, Baer-Keeley M (2006) Demographic patterns of postfire regeneration in Mediterranean-climate shrublands of California. Ecological Monographs 76, 235-255.
| Crossref | Google Scholar |
Ladd PG, Zhao X, Enright NJ (2022) Fire regime and climate determine spatial variation in level of serotiny and population structure in a fire-killed conifer. Plant Ecology 223, 849-862.
| Crossref | Google Scholar |
Lamont BB (2021) Evaluation of seven indices of on-plant seed storage (serotiny) shows that the linear slope is best. Journal of Ecology 109, 4-18.
| Crossref | Google Scholar |
Lamont BB, He T, Yan Z (2019) Evolutionary history of fire-stimulated resprouting, flowering, seed release and germination. Biological Reviews 94, 903-928.
| Crossref | Google Scholar | PubMed |
Lamont BB, Pausas JG, He T, Witkowski ETF, Hanley ME (2020) Fire as a selective agent for both serotiny and nonserotiny over space and time. Critical Reviews in Plant Sciences 39, 140-172.
| Crossref | Google Scholar |
Lehmann CER, Prior LD, Bowman DMJS (2009) Fire controls population structure in four dominant tree species in a tropical savanna. Oecologia 161, 505-515.
| Crossref | Google Scholar | PubMed |
Lindenmayer DB, Cunningham RB, Donnelly CF, Franklin JF (2000) Structural features of old-growth Australian montane ash forests. Forest Ecology and Management 134, 189-204.
| Crossref | Google Scholar |
Liyanage GS, Ooi MKJ (2015) Intra-population level variation in thresholds for physical dormancy-breaking temperature. Annals of Botany 116, 123-131.
| Crossref | Google Scholar | PubMed |
Liyanage GS, Ooi MKJ (2017) Do dormancy-breaking temperature thresholds change as seeds age in the soil seed bank? Seed Science Research 27, 1-11.
| Crossref | Google Scholar |
Lullfitz A, Pettersen C, Reynolds R(Doc), et al. (2021) The Noongar of south-western Australia: a case study of long-term biodiversity conservation in a matrix of old and young landscapes. Biological Journal of the Linnean Society 133, 432-448.
| Crossref | Google Scholar |
McLauchlan KK, Higuera PE, Miesel J, et al. (2020) Fire as a fundamental ecological process: research advances and frontiers. Journal of Ecology 108, 2047-2069.
| Crossref | Google Scholar |
Merritt DJ, Turner SR, Clarke S, Dixon KW (2007) Seed dormancy and germination stimulation syndromes for Australian temperate species. Australian Journal of Botany 55, 336-344.
| Crossref | Google Scholar |
Miller JED, Safford HD (2020) Are plant community responses to wildfire contingent upon historical disturbance regimes? Global Ecology and Biogeography 29, 1621-1633.
| Crossref | Google Scholar |
Miller RG, Fontaine JB, Merritt DJ, Miller BP, Enright NJ (2021) Experimental seed sowing reveals seedling recruitment vulnerability to unseasonal fire. Ecological Applications 31, e02411.
| Crossref | Google Scholar | PubMed |
Miller R, Enright N, Fontaine J, Merritt D, Miller B (2023) Plant life history data as evidence of an historical mixed-severity fire regime in Banksia woodlands [Dataset]. Zenodo. https://doi.org/10.5281/zenodo.10046435
Monk D, Pate JS, Loneragan WA (1981) Biology of Acacia pulchella R.Br. with special reference to symbiotic nitrogen fixation. Australian Journal of Botany 29, 579-592.
| Crossref | Google Scholar |
Monks L, Barrett S, Beecham B, et al. (2019) Recovery of threatened plant species and their habitats in the biodiversity hotspot of the Southwest Australian Floristic Region. Plant Diversity 41, 59-74.
| Crossref | Google Scholar | PubMed |
Murphy BP, Bradstock RA, Boer MM, Carter J, Cary GJ, Cochrane MA, Fensham RJ, Russell-Smith J, Williamson GJ, Bowman DMJS (2013) Fire regimes of Australia: a pyrogeographic model system. Journal of Biogeography 40, 1048-1058.
| Crossref | Google Scholar |
Ooi MKJ, Whelan RJ, Auld TD (2006) Persistence of obligate-seeding species at the population scale: effects of fire intensity, fire patchiness and long fire-free intervals. International Journal of Wildland Fire 15, 261-269.
| Crossref | Google Scholar |
Ooi MKJ, Denham AJ, Santana VM, Auld TD (2014) Temperature thresholds of physically dormant seeds and plant functional response to fire: variation among species and relative impact of climate change. Ecology and Evolution 4, 656-671.
| Crossref | Google Scholar | PubMed |
Orscheg CK, Enright NJ (2011) Patterns of seed longevity and dormancy in obligate seeding legumes of box-ironbark forests, south-eastern Australia. Austral Ecology 36, 185-194.
| Crossref | Google Scholar |
Palmer HD, Denham AJ, Ooi MKJ (2018) Fire severity drives variation in post-fire recruitment and residual seed bank size of Acacia species. Plant Ecology 219, 527-537.
| Crossref | Google Scholar |
Pausas JG (2015) Evolutionary fire ecology: lessons learned from pines. Trends in Plant Science 20, 318-324.
| Crossref | Google Scholar | PubMed |
Pausas JG, Keeley JE (2014) Evolutionary ecology of resprouting and seeding in fire-prone ecosystems. New Phytologist 204, 55-65.
| Crossref | Google Scholar | PubMed |
Pausas JG, Keeley JE, Schwilk DW (2017) Flammability as an ecological and evolutionary driver. Journal of Ecology 105, 289-297.
| Crossref | Google Scholar |
Perry DA, Hessburg PF, Skinner CN, Spies TA, Stephens SL, Taylor AH, Franklin JF, McComb B, Riegel G (2011) The ecology of mixed severity fire regimes in Washington, Oregon, and northern California. Forest Ecology and Management 262, 703-717.
| Crossref | Google Scholar |
Plucinski MP (2014) The timing of vegetation fire occurrence in a human landscape. Fire Safety Journal 67, 42-52.
| Crossref | Google Scholar |
Quintana-Ascencio PF, Koontz SM, Ochocki BM, Sclater VL, López-Borghesi F, Li H, Menges ES (2019) Assessing the roles of seed bank, seed dispersal and historical disturbances for metapopulation persistence of a pyrogenic herb. Journal of Ecology 107, 2760-2771.
| Crossref | Google Scholar |
Radeloff VC, Mladenoff DJ, Guries RP, Boyce MS (2004) Spatial patterns of cone serotiny in Pinus banksiana in relation to fire disturbance. Forest Ecology and Management 189, 133-141.
| Crossref | Google Scholar |
Ramalho CE, Laliberté E, Poot P, Hobbs RJ (2014) Complex effects of fragmentation on remnant woodland plant communities of a rapidly urbanizing biodiversity hotspot. Ecology 95, 2466-2478.
| Crossref | Google Scholar |
R Core Team (2020) R: a language and environment for statistical computing. Available at https://www.R-project.org/
Ritchie AL, Svejcar LN, Ayre BM, et al. (2021) A threatened ecological community: research advances and priorities for Banksia woodlands. Australian Journal of Botany 69, 53-84.
| Crossref | Google Scholar |
Rodrigues U, Lullfitz A, Coyne L, et al. (2022) Indigenous knowledge, aspiration, and potential application in contemporary fire mitigation in southwest Australia. Human Ecology 50, 963-980.
| Crossref | Google Scholar |
Salguero-Gómez R, Jones OR, Jongejans E, Blomberg SP, Hodgson DJ, Mbeau-Ache C, Zuidema PA, de Kroon H, Buckley YM (2016) Fast–slow continuum and reproductive strategies structure plant life-history variation worldwide. Proceedings of the National Academy of Sciences 113, 230-235.
| Crossref | Google Scholar |
Schoennagel T, Turner MG, Kashian DM, Fall A (2006) Influence of fire regimes on lodgepole pine stand age and density across the Yellowstone National Park (USA) landscape. Landscape Ecology 21, 1281-1296.
| Crossref | Google Scholar |
Schwilk DW, Ackerly DD (2001) Flammability and serotiny as strategies: correlated evolution in pines. Oikos 94, 326-336.
| Crossref | Google Scholar |
Setterfield SA (2002) Seedling establishment in an Australian tropical savanna: effects of seed supply, soil disturbance and fire. Journal of Applied Ecology 39, 949-959.
| Crossref | Google Scholar |
Shedley E, Burrows N, Yates CJ, Coates DJ (2018) Using bioregional variation in fire history and fire response attributes as a basis for managing threatened flora in a fire-prone Mediterranean climate biodiversity hotspot. Australian Journal of Botany 66, 134-143.
| Crossref | Google Scholar |
Swetnam TW, Allen CD, Betancourt JL (1999) Applied historical ecology: using the past to manage for the future. Ecological Applications 9, 1189-1206.
| Crossref | Google Scholar |
Tangney R, Miller RG, Fontaine JB, Veber WP, Ruthrof KX, Miller BP (2022) Vegetation structure and fuel dynamics in fire-prone, Mediterranean-type Banksia woodlands. Forest Ecology and Management 505, 119891.
| Crossref | Google Scholar |
Tonnabel J, Van Dooren TJM, Midgley J, Haccou P, Mignot A, Ronce O, Olivieri I (2012) Optimal resource allocation in a serotinous non-resprouting plant species under different fire regimes. Journal of Ecology 100, 1464-1474.
| Crossref | Google Scholar |
Treurnicht M, Pagel J, Esler KJ, Schutte-Vlok A, Nottebrock H, Kraaij T, Rebelo AG, Schurr FM (2016) Environmental drivers of demographic variation across the global geographical range of 26 plant species. Journal of Ecology 104, 331-342.
| Crossref | Google Scholar |
Turner BL, Hayes PE, Laliberté E (2018) A climosequence of chronosequences in southwestern Australia. European Journal of Soil Science 69, 69-85.
| Crossref | Google Scholar |
Valentine LE, Reaveley A, Johnson B, Fisher R, Wilson BA (2012) Burning in Banksia Woodlands: how does the fire-free period influence reptile communities? PLoS ONE 7, e34448.
| Crossref | Google Scholar | PubMed |
Valentine LE, Fisher R, Wilson BA, Sonneman T, Stock WD, Fleming PA, Hobbs RJ (2014) Time since fire influences food resources for an endangered species, Carnaby’s cockatoo, in a fire-prone landscape. Biological Conservation 175, 1-9.
| Crossref | Google Scholar |
Varner JM, Kane JM, Hiers JK, Kreye JK, Veldman JW (2016) Suites of fire-adapted traits of oaks in the southeastern USA: multiple strategies for persistence. Fire Ecology 12, 48-64.
| Crossref | Google Scholar |
Venter SM, Witkowski ETF (2010) Baobab (Adansonia digitata L.) density, size-class distribution and population trends between four land-use types in northern Venda, South Africa. Forest Ecology and Management 259, 294-300.
| Crossref | Google Scholar |
Weiner J, Solbrig OT (1984) The meaning and measurement of size hierarchies in plant populations. Oecologia 61, 334-336.
| Crossref | Google Scholar | PubMed |
Werner PA, Peacock SJ (2019) Savanna canopy trees under fire: long-term persistence and transient dynamics from a stage-based matrix population model. Ecosphere 10, e02706.
| Crossref | Google Scholar |
Western Australian Herbarium (1998-) Florabase – the Western Australian Flora. Department of Biodiversity, Conservation and Attractions. Available at https://florabase.dbca.wa.gov.au/
Whelan RJ, De Jong NH, Von Der Burg S (1998) Variation in bradyspory and seedling recruitment without fire among populations of Banksia serrata (Proteaceae). Australian Journal of Ecology 23, 121-128.
| Crossref | Google Scholar |
Wiegand K, Ward D, Thulke H-H, Jeltsch F (2000) From snapshot information to long-term population dynamics of acacias by a simulation model. Plant Ecology 150, 97-114.
| Crossref | Google Scholar |
Williams PR (2009) Contrasting demographics of tropical savanna and temperate forest eucalypts provide insight into how savannas and forests function. A case study using Corymbia clarksoniana from north-eastern Australia. Austral Ecology 34, 120-131.
| Crossref | Google Scholar |
Wilson BA, Kuehs J, Valentine LE, Sonneman T, Wolfe KM (2014) Guidelines for ecological burning regimes in Mediterranean ecosystems: a case study in Banksia woodlands in Western Australia. Pacific Conservation Biology 20, 57-74.
| Crossref | Google Scholar |
Witkowski ETF, Lamont BB, Connell SJ (1991) Seed bank dynamics of three co-occurring banksias in south coastal Western Australia: the role of plant age, cockatoos, senescence and interfire establishment. Australian Journal of Botany 39, 385-397.
| Crossref | Google Scholar |
Wooller SJ, Wooller RD, Brown KL (2002) Regeneration by three species of Banksia on the south coast of Western Australia in relation to fire interval. Australian Journal of Botany 50, 311-317.
| Crossref | Google Scholar |
Zammit C, Westoby M (1987) Seedling recruitment strategies in obligate-seeding and resprouting Banksia shrubs. Ecology 68, 1984-1992.
| Crossref | Google Scholar | PubMed |
Zomer M, Moreira B, Pausas JG (2022) Fire and summer temperatures interact to shape seed dormancy thresholds. Annals of Botany 129, 809-816.
| Crossref | Google Scholar | PubMed |