Myrtaceae anther glands: morphology, anatomy and variation in glandular contents
P. G. Ladd
A
Abstract
Most tissues of Myrtaceae plants have oil glands. The anthers of many species have an oil-containing apical gland that is larger than those in other tissues of the plant.
Representative species in the family were examined for the diversity of gland form and their oil contents.
Representative anthers were sectioned for light microscopy and scanning electron microscopy study and anthers from selected species were analysed for oil content.
The most common gland form is globular and narrowly attached to the apex of the connective, but in members of certain tribes, the gland is completely enclosed in the connective. The greatest morphological diversity is in the Chamelaucieae. Anther glands vary from plesiomorphic globular forms to glands that are larger than the anther thecae and almost completely fill the connective.
There are three possible functions for the glands, including the following: (1) protecting the anthers from herbivores, (2) mixing with the pollen to aid adhesion to stylar hairs on many Chamelaucineae, and (3) rewarding pollinators that use the oil–pollen mixture as food.
It is generally considered that the oils in various tissues of the Myrtaceae deter herbivores. In Myrtaceae with abundant anthers, the glands could deter flower visitors from consuming the anthers. Gland oil of the Eucalyptus and Leptospermum species examined contained α pinene as did the leaves of all species examined. The gland oil composition in Chamelaucium uncinatum and Verticordia grandis that have pollen presenters was different from that in the leaves and also different from that in the anthers of the two Verticordia species where bees collect the pollen–oil mixture for food.
Keywords: anther anatomy, anther morphology, Chamelaucieae, floral biology, floral herbivory, Myrtaceae oil, pollen presenter, pollination.
Introduction
The development of the flowering plant reproductive system has resulted, at least in part, from a complex interplay between the need to protect somewhat energetically expensive reproductive structures and the requirement to advertise and attract a desirable go between. In addition, the diversity of floral form seems to be related to specialised relationships with pollinators (Ollerton 1996; Johnson 2010) but flower–animal interactions may be related to chemical traits as well (e.g. Dafni and Bernhardt 1990; Johnson 2016). The trade-off between plants and pollinators is food for the pollinator and transport of pollen for the plant. However, the floral advertisement to attract pollinators will also advertise to animal herbivores that there is a food source available and these florivores may consume the reproductive organs or damage the advertising organs so much that they no longer function to attract legitimate pollinators (Sánchez-Lafuente 2007; Cardel and Koptur 2010). Damage to flowers will affect male and female fitness, particularly if the anthers or gynoecia are consumed (McCall and Irwin 2006).
In most flowers there seems little protection for either sterile or fertile organs, with the plant relying on the abundance of flowers or their ephemeral nature to ensure that damage is manageable and that some will be successful in producing seeds. However, in a number of groups protective mechanisms for some floral organs are provided. In most cases, these are related to the general herbivore protective mechanisms in the vegetative parts of the plant, for example, in Solanaceae (Datura) and Apocynaceae (Nerium), where the flowers contain toxins. In some species, there is even toxic nectar or pollen (e.g. Ranunculus; Wcislo and Cane 1996).
Armbruster et al. (1997) provided a detailed analysis of the floral protective system in Dalechampia (Euphorbiaceae), which they believed had become modified through coevolution with bees into a pollinator-attraction system. The protective resins in inflorescences (oxygenated triterpenes) had been evolutionally modified to become attractant chemicals for specialist pollinators (worker meliponine, Apidae, bees). In an extension of the system, the presence of floral reward chemicals in the inflorescence facilitated the evolution, often by minor biochemical modifications, of resin defences in the leaves of a few advanced species (e.g. Dalechampia websteri). This is a complex, if chemically minor, sequence of changes because the chemical changes in the inflorescence are accompanied by structural changes in the arrangement of the resin-producing glands as well. This is perhaps not unusual because there can be a considerable diversity of secondary plant chemicals even in a single genus of tree (Inga) and the most divergent chemical profiles were in sympatric species, indicating that maintaining unique chemical profiles was important in co-occurring species (Forrester et al. 2023).
One of the characteristics of the family Myrtaceae is the presence of oil glands in the leaves, even in the pith of stems and in various floral parts (Johnson and Briggs 1984). It is generally considered that the oils are unpleasant/poisonous to animals that might be attracted to consume plant parts, and so, they are a protection against herbivory (Rafferty and Lamont 2021; Müller et al. 2022). For example, the monoterpene 1,8 cineole, which is a common component of Eucalyptus oil, was considered a deterrent to hares from eating balsam poplars in Alaska (Reichardt et al. 1990). However, many animals have developed mechanisms to cope with Myrtaceae secondary chemicals (e.g. Koalas). The oil glands in most Myrtaceae primary tissue are small (45–54 μm) lysigenous cavities (e.g. Dörken and Parsons 2018). In Melaleuca alternifolia, leaf glands can be from 80 to 150 μm in diameter (List et al. 1995), and in the pith of two eucalypts from 91 to 200 μm (Carr and Carr 1969). In many members of the family, larger glands are located on the anthers (Johnson and Briggs 1984), either at the apex or within the connective between the loculi and, although little studied, are considered to contain predominantly oil chemicals. Apical extensions of anthers are uncommon, although widely spread through the angiosperms (Bernhardt 1996; Endress 1996). Glands on anthers are even less common. They do occur in some members of the Rutaceae (P. G. Ladd, pers. obs.), and in the Fabaceae are considered to produce substances that assist in pollen transfer via pollinators (Leite et al. 2019). In the Myrtaceae, anthers are often important characters in the taxonomy of different groups (Rye 2009). In the Myrteae, stamen position in bud is important (Vasconcelos et al. 2015) and, in Chamelaucieae, there is considerable diversity in anther morphology both in the way pollen is liberated and in the shape of the anther (Rye 2009). An evolutionary driver towards diversity in anther-gland form in the family may have in part been driven by the fact that in most of the tribes anthers are the primary advertisement to pollinators (e.g. Eucalypteae, Melaleuceae, Myrteae, Syzygieae) and thus there would be benefit in providing them with herbivore deterrents. In contrast to these groups, in the Chamelaucieae the petals are more obvious as an advertisement than are anthers, and anther number is reduced; however, within the tribe the stamen morphology is more varied than in the groups with abundant anthers. There is considerable diversity in the form and size of the glands and in their function (e.g. Ladd et al. 1999; Rye 2009). In the majority of the species, they remain intact at anthesis and if filled with distasteful oil and eaten would, subsequently, presumably, act as a deterrent to protect the male gametes. However, in a number of species in the Chamelaucieae tribe, the oil exudes from the gland to mix with the pollen at or before anthesis and either remains on the anther, or (in five genera) may be transferred to a pollen presenter on the gynoecium for later transfer on a pollinator to other flowers (for summary, see Ladd 1994; Ladd et al. 1999).
In this study, the general morphology, and in some cases, anatomy, of anthers will be summarised to give an overview of anther-gland form in the family, with some concentration on the Chamelaucieae because this tribe has the most diverse anther morphology. As in the study by Armbruster et al. (1997), hypotheses can be proposed to aid investigation of the glandular products in the pollination system and how any changes in function may have evolved. These include the following:
Materials and methods
Anther morphology was determined from various sources, and sketch diagrams from a range of species are included in Supplementary Table S1. Species were collected from the field, particularly in Western Australia (voucher specimens lodged in the Murdoch University Herbarium; MUR), from botanic gardens where plants were labelled, examination of herbarium specimens and from published species’ descriptions. Flowers that were examined were from a range of species over most of the tribes in the family as recognised in Thornhill et al. (2015) (see Table S2). Web links and some images of the forms of the flowers that were specifically examined in this study are listed in Table S3.
Material for histological examination was collected as mature buds fixed in 3% glutaraldehyde in 0.025 phosphate buffer (pH 7.0) and embedded in Spurr’s resin after dehydration through a graded series of acetone solutions. Light-microscope sections were cut on a Leica RM2235 rotary microtome at 10 μm thickness stained with Toluidine blue or 1% methylene blue and 1% azur 11 in 1% sodium tetraborate. For scanning electron microscopy, anthers were initially fixed in formalin–acetic acid–alcohol (FAA) and, subsequently, critical-point dried after dehydrating through a graded series of ethanol–water solutions. Specimens were sputter coated with gold.
Six species in the Myrtaceae were examined for the oil content of leaves and anthers. They were from three of the tribes of the Myrtaceae and included Eucalyptus conferruminata (Eucalypteae), Leptospermum laevigatum (Leptospermeae) and four species from the Chamelaucieae (Chamelaucium uncinatum, Verticordia grandis, V. nitens and V. aurea). Anthers selected from mature, unopened buds and leaves collected from live plants were extracted in absolute ethanol and analysed using a HP 5890 gas chromatograph fitted with HP 6890 autosampler using a 25 m 5% methyl phenyl silicone capillary column and on-column injection. The temperature program commenced isothermally for 5 min at 80°C, rising at 5°C per min to 180°C with a 7.5 min isothermal period to conclude the analysis. Helium was used as the carrier gas and detection was performed by a flame ionisation detector. Compound assignments were initially made by comparison based on retention time and confirmed by analytical-grade external standards where these were available.
Results
Description of anther characteristics
There is a diversity of anther forms in the Myrtaceae and seven types have been recognised here (Table 1). All the Myrtaceae taxa that have stamens as their main floral display (the majority of the genera in the family) have anthers in Categories A, B, C and G (Calytrix) and oil is retained in the anther gland if that is present. The most frequent Myrtaceae anther-gland type has a globular morphology and is narrowly connected at the apex to the connective (Fig. 1c). This is the plesiomorphic form (Rye 2009) and occurs in at least some species of all tribes. A modification of this type is where the gland is more incorporated in the upper part of the connective and may be completely included or bulges from the upper part of the connective on the abaxial side (Fig. 1a, e and f).
Category | Gland type | Oil exudes | Exuded oil mixes with pollen | Dehiscence pore/slit | Taxon | References | |
---|---|---|---|---|---|---|---|
A | Absent | – | – | Slit, some pores | Calothamnus (some), Cheyniana; Hypocalymma (some), Scholtzia (some), Xanthostemon | Observations this study | |
B | Globular at apex of connective, narrow attachment | No | No | Slit or pore | Agonis, Aluta, Astartea, Astus, Austromyrtus, Enekbatus, Ericomyrtus, Euromyrtus, Kunzea, Leptospermum, Lithomyrtus, Melaleuca (some), Micromyrtus (some), Seorsus, Stenostegia, Taxandria, Thryptomene (some), Triplarina | Observations this study; Bean (1995, 1998); Rye et al (2020); Trudgen and Rye (2005); Rye and Trudgen (2000, 2008) | |
C | Globular to elongate at apex of connective, may extend into connective, broad attachment | No | No | Slit, some pores | Angophora, Callistemon, Corymbia, Eucalyptus, Hypocalymma, Lophostemon, Melaleuca (some), Oxymyrrihine, Scholtzia, Rinzia, Syzigium, Tristania, Tristaniopsis | Observations this study; Rye (2009) | |
D | Small (<0.1 mm) and globular at apex of connective | No | No | Slit or pore | Actinodium, Balustion, Beaufortia, Calothamnus (some), Calytrix (some), Conothamnus, Darwinia (some), Eremaea, Homoranthus, Hypocalymma (some), Regelia, Oxymrrhine, Pileanthus, Stenostegia, Syncarpia, Thryptomene (some), Verticordia (some) | Observations this study; Rye (2014) | |
E | Globular at apex of connective with broad attachment | Yes | Yes | Pore | Chamelaucium, Ericomyrtus (some), Darwinia, (some), Verticordia (some) | Observations this study | |
F | Expanded apical modification of connective, sometime with elaborate non-glandular tissue | Yes | In some | Pore | Anticoryne, Austrobaeckea, Babbingtonia, Baeckea Murchison sp., Darwinia (some) Ericomyrtus, Hysterobaeckea, Malleostemon, Micromyrtus, Sannantha, Tetrapora, Thryptomene (some), Verticordia (Chrysoma, and others) | Observations this study; Rye (2015, 2017, 2021); Rye et al (2020); Wilson and Heslewood (2014) | |
G | Stalked or elongate gland at apex of connective | In some | No | Slit or pore | Calytrix (some), Cyathostemon, Thryptomene (some) | Observations this study |
Myrtaceae anthers. (a, b, d, e) Transmission light microscope images, (c, f) dissecting microscope images. (a) Baeckea sp. Longitudinal section (LS) of an anther typical of many in Chamelaucieae; fl, filament; gl, gland; ep, epidermis; en, endothecium; oc, oil cell remains; l, loculus. (b) Verticordia grandis anther LS, showing the network of cells in an immature anther gland (gl). (c) Leptospermum laevigatum abaxial view, showing discrete gland (arrow) attached to the connective above the filament junction. (d) L. laevigatum anther LS, showing discrete stalked gland and two loculi with pollen grains. (e) Eucalyptus leucoxylon anther transverse section (TS), showing the gland (gl) completely internal in the connective (slightly oblique, TS). (f) E. drummondii showing the gland (arrows) only slightly bulging from the connective (left) and LS of an anther showing oil in the gland (right). Scale bars = 50 μm (a–e), 500 μm (f).
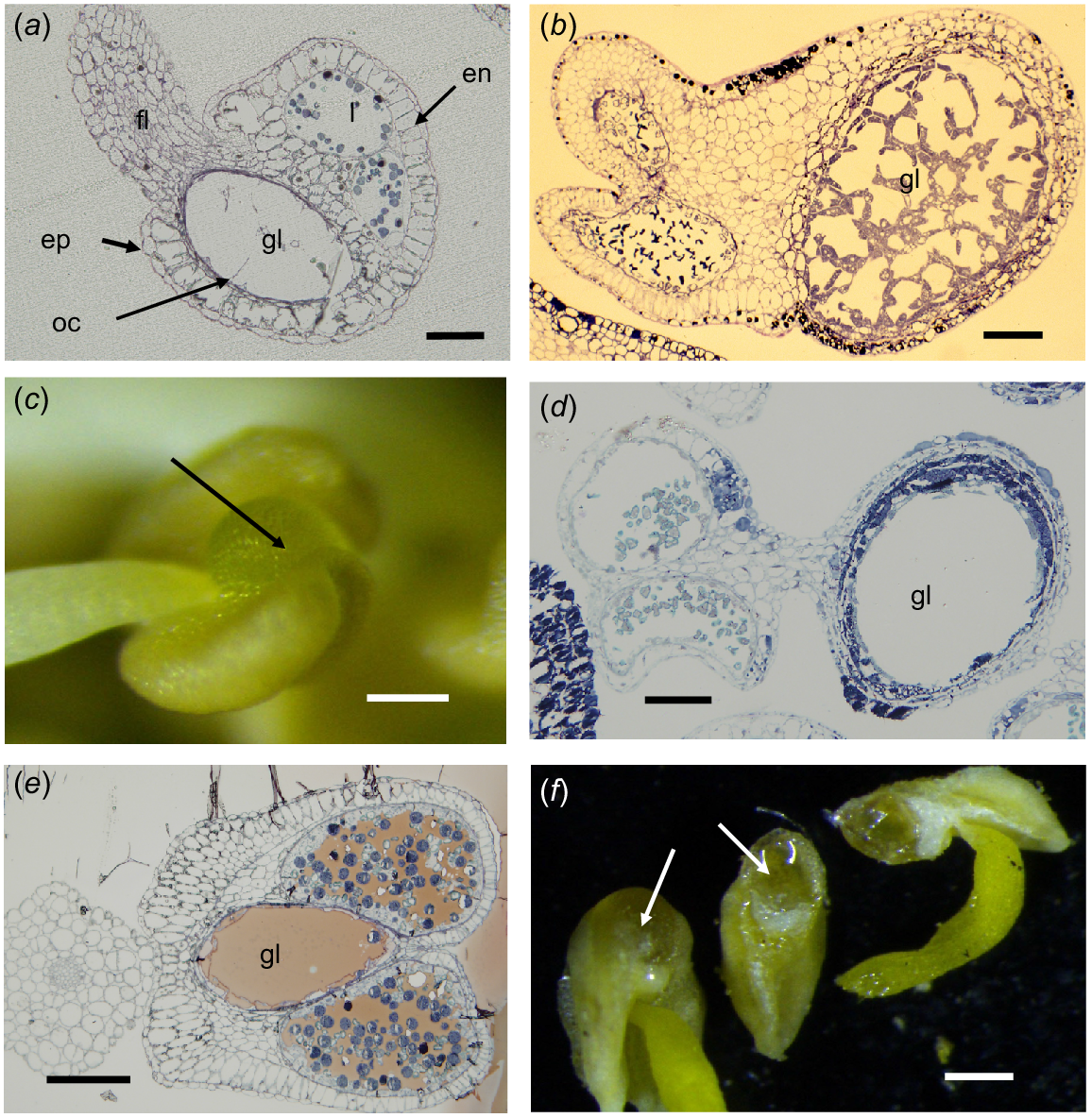
In the Melaleuceae, in some species, a gland is absent (Category A, some Callistemon, Calothamnus), or so small it seems almost absent (<0.1 mm) and is embedded in the apex of the connective (Beaufortia, Category D). There is more diversity in gland types and function in the Chamelaucieae. There is a range from glands that are very small, contained in the top of the connective and do not exude contents (Actinodium, Homoranthus, some Verticordia) to large bulbous apical structures broadly attached to the top of the connective that ooze oil that mixes with the pollen at anthesis (Category E, Fig. 2a, b). Category F anthers occur in flowers that have petals as the main advertisement and glands are often not externally obvious, being incorporated within the top of the connective. Often the anthers all face towards the centre of the flower; some may exude oil to mix with the pollen at anthesis, but this needs more observations to confirm with many of the species.
Chamelaucieae anthers. (a, b, d) Dissecting microscope images, (c, e, f) transmission light microscope images. (a) Chamelaucium uncinatum front view of anther, with four loculi and gland (arrow) bulging from the top of the connective. (b) C. uncinatum showing oil and pollen loading onto the pollen presenter hairs at anthesis. (c) C. uncinatum oblique transverse section (TS) of an anther, showing the network of cells in an immature anther gland (gl). (d) Babingtonia grandiflora external appearance of gland (gl) in side view, completely contained in the connective on the abaxial side of the anther. (e) B. grandiflora TS of anther, showing four loculi and the abaxial gland (gl). (f) B. grandiflora LS of anther, showing the gland (gl) occupying almost the full length of the connective above the loculus. Scale bars = 50 μm.
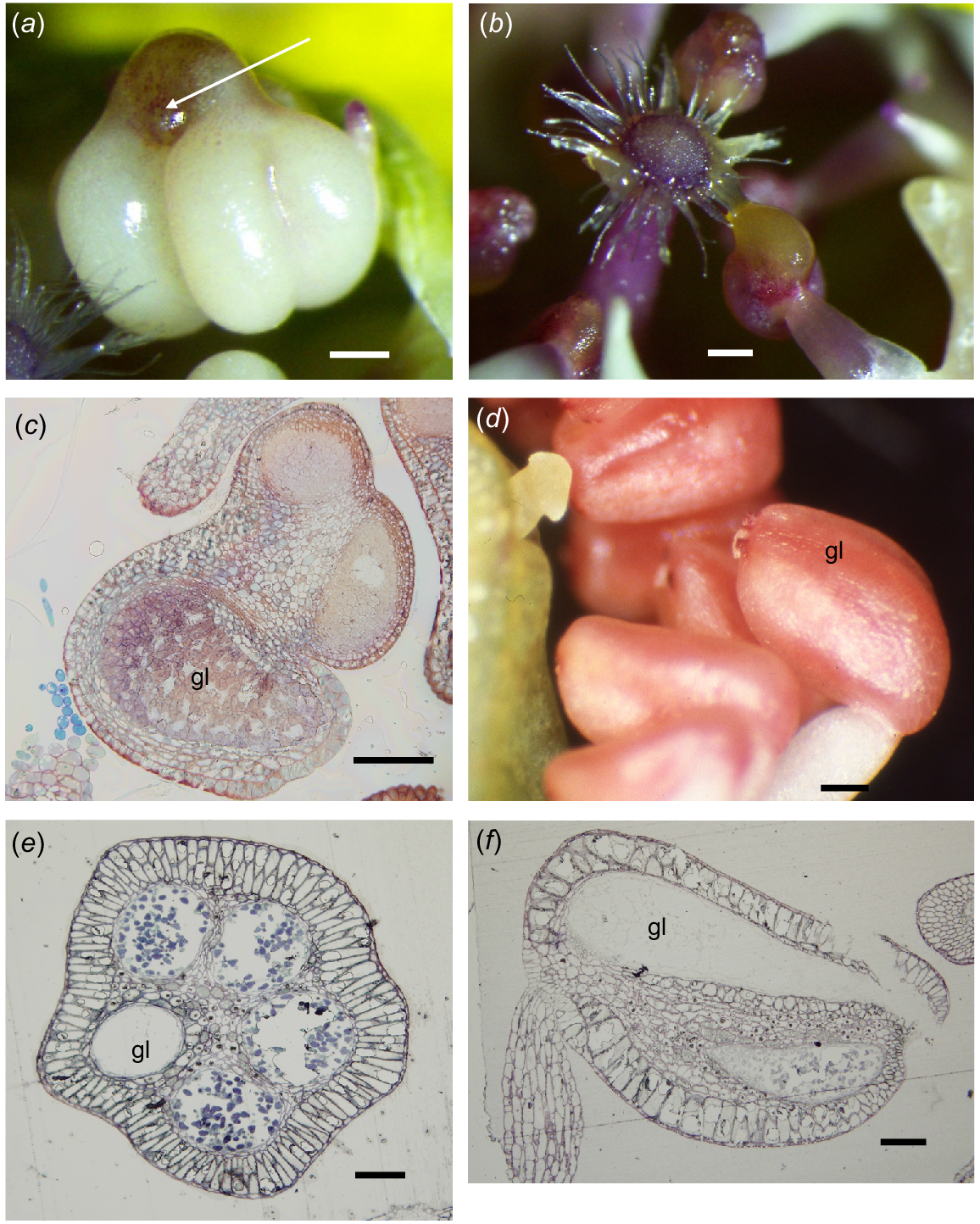
The glands form schizo-lysigenously, with the contents of the mature gland being produced by the cells that completely filled the gland cavity in immature anthers (Figs 1b, 2b). These cells ultimately break down as they mature, releasing their contents so a secretory cavity forms and the cell wall remnants then line the wall of the cavity. The anthers of a number of species with representative morphology of different gland types are described below.
Anther was tetrasporangiate with two thecae, dehiscence introse by slits. Anther was versatile with the filament attached at about the mid-point of the connective that joined the thecae. A globular stalked gland was attached to the upper part of the connective above the filament attachment (Fig. 1c). The epidermis of the thecae and the gland was composed of flattened thin-walled cells (10.4 × 3.5 μm, Fig. 1d). Underlying the epidermis on the thecae there was a single layer of cells (endothecium, 13.5 × 17.3 μm) that were different from the thinner, more elongate cells in several layers around the gland (3.5 × 10.4 μm, Fig. 1d). Within the gland cavity, there were the remains of the original cells lying along the gland wall.
Anthers was tetrasporangiate but at anthesis opening by a slit down the join between each pair of sporangia, versatile. The anther of E. leucoxylon was box-like, the gland being completely contained in the connective, so not easily discernable externally and did not exude oil at anthesis. The epidermis was a single layer of very thin cells (10.4 × 20.7–10.4 × 55.2 μm) underlain by one to two layers of cells around the thecae, but in the connective surrounding the gland, the cells were more elliptical to equidimensional and next to the gland very thin and flattened (34.5 × 3.5 μm, Fig. 1e). Although there was only a very thin layer of cells between the gland and the loculi, there was no evidence that oil mixes with the pollen at anthesis. In E. drummondii, the anther was more elongate and the gland was in the upper part of the connective (Fig. 1f), but did bulge towards the top of the connective, so was more obvious than in the other species. Again oil was not exuded at anthesis.
Anthers were tetrasporangiate with two thecae and at maturity only two loculi. The gland was large and broadly fused to and contained in the top of the connective (Fig. 2a). The epidermis was of thin-walled cells, smaller over the thecae (10.2 × 6.9 μm) than over the gland (17.3 × 13.8–24.2 × 13.8 μm). Underlying the epidermis, the hypodermis consisted of flattened, slightly elongate thin-walled cells (4.4 × 3.5 μm) and the gland was delimited by a single layer of small, more equidimensional cells (Fig. 2c). Anthesis occurred before the flower opened and oil from the gland was exuded to mix with the pollen that was loaded onto the pollen-presenter hairs (Fig. 2b).
Anthers were tetrasporangiate with four loculi. The anther was orientated at 90° to the filament and the apex pointed towards the style in bud and at anthesis (Fig. 2d). The gland was entirely contained within and occupied almost the full length of the connective of the anther (Fig. 2f), so the whole structure was ‘helmet-shaped’ (Rye 2009). In transverse section, the epidermal cells were very thin (24.2 × 3.4 μm) and similar over both the thecae and the gland (Fig. 2e). Underlying the epidermis the hypodermis was of one to two layers of radially elongated thin-walled cells (24.2 × 13.8 μm) that were identical over the gland and the thecae. The cells of the connective were equidimensional and slightly smaller surrounding the thecae than the gland. The gland cavity at maturity was lined by flattened cells and cell-wall remains.
In S. laxiflora, the anthers were tetrasporangiate and had four loculi. The gland was contained within the connective and had a small extension that lies between the thecae (Fig. 3a). Epidermal cells were very narrow and thin-walled over the theca and slightly larger over the gland. The endothecium was a single layer of cells surrounding the thecae. There were several layers of elongate cells around the gland cavity and, in Fig. 3b, a longitudinal section (on the right) and transverse section (on the left) show that the gland was a tube in the connective that ends in the projection shown in Fig. 3a.
Chamelaucieae anthers. (a, c, e, f) Scanning electron microscope (SEM) images, and (b, d) transmission light microscope images. (a) Scholtzia laxiflora frontal view of anther, with four loculi and gland spout (arrow) projecting between the thecae from the more extensively developed cavity in the connective. (b) S. laxiflora anther section showing three of the four loculi (l) and gland cavity (gl) in transverse section (TS, left) and longitudinal section (LS, right) from a semi-mature bud. (c) View of the top of a Micromyrtus obovatus anther, showing four loculi and the external view of the gland (gl) on the upper part of the connective overarching the thecae. (d) M. obovatus slightly oblique TS, showing three cavities in the gland. (e) Baeckea sp. Murchison River, showing the thecae (t) borne on the front of the connective that completely encloses the gland (gl). (f) Front and top views of Rinzia crassifolia anthers showing four loculi attached to a very broad filament (fl) and a small gland at the top of the connective (arrow). Scale bars = 50 μm.
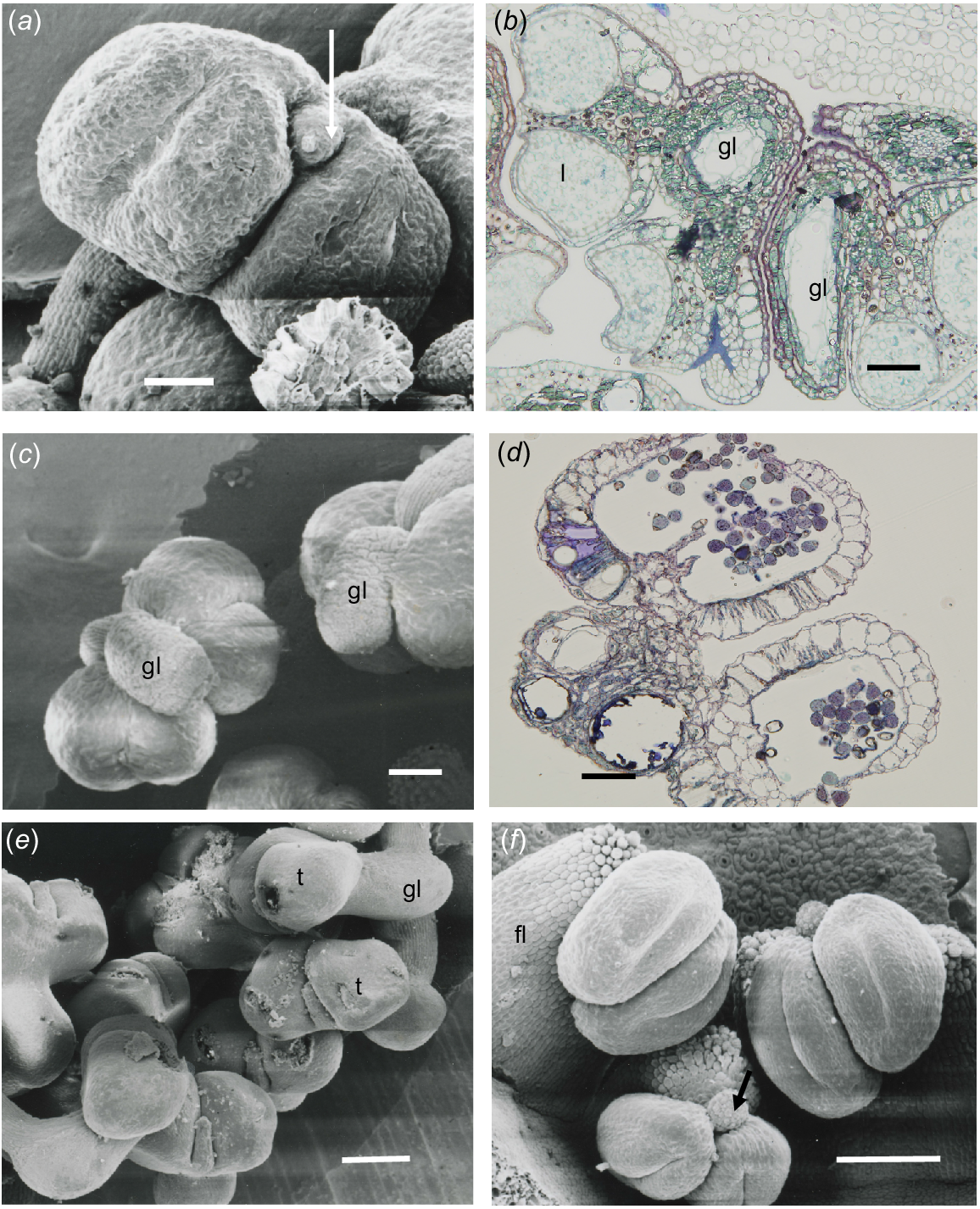
In S. teretifolia, similarly to S. laxiflora, the anthers were tetrasporangiate and have four loculi at anthesis. The filament was attached basally to the connective (Fig. 4a) that contains the gland and the thecae protrude from this towards the centre of the flower. The epidermis was a single layer of very thin cells (13.8–24.2 × 3.5 μm) underlain by one to two layers of cells becoming much reduced as the hypodermis over the gland, compared with the endothecium over the thecae (13.8 × 3.45 μm). The gland cavity at maturity was lined with very thin cells and the remains of the walls of the oil-producing cells.
Chamelaucieae anthers. (a) Transmission light microscope image, (b) scanning electron microscope (SEM) image, and (c, d) dissecting microscope images. (a) Scholtzia teretifolia LS of gland and one loculus, showing an anther structure similar to that in Baeckea in Fig. 3e. (b) Abaxial view of Calytrix aurea urn-shaped gland (gl) narrowly attached to the connective. (c) Calytrix decandra anther in side view, with loculus on the upper side and the sterile anther extension below. (d) Thryptomene baeckeacea showing frontal view of two thecae extruding coherent pollen strands and a gland overarching the thecae (arrows). Scale bars = 50 μm.
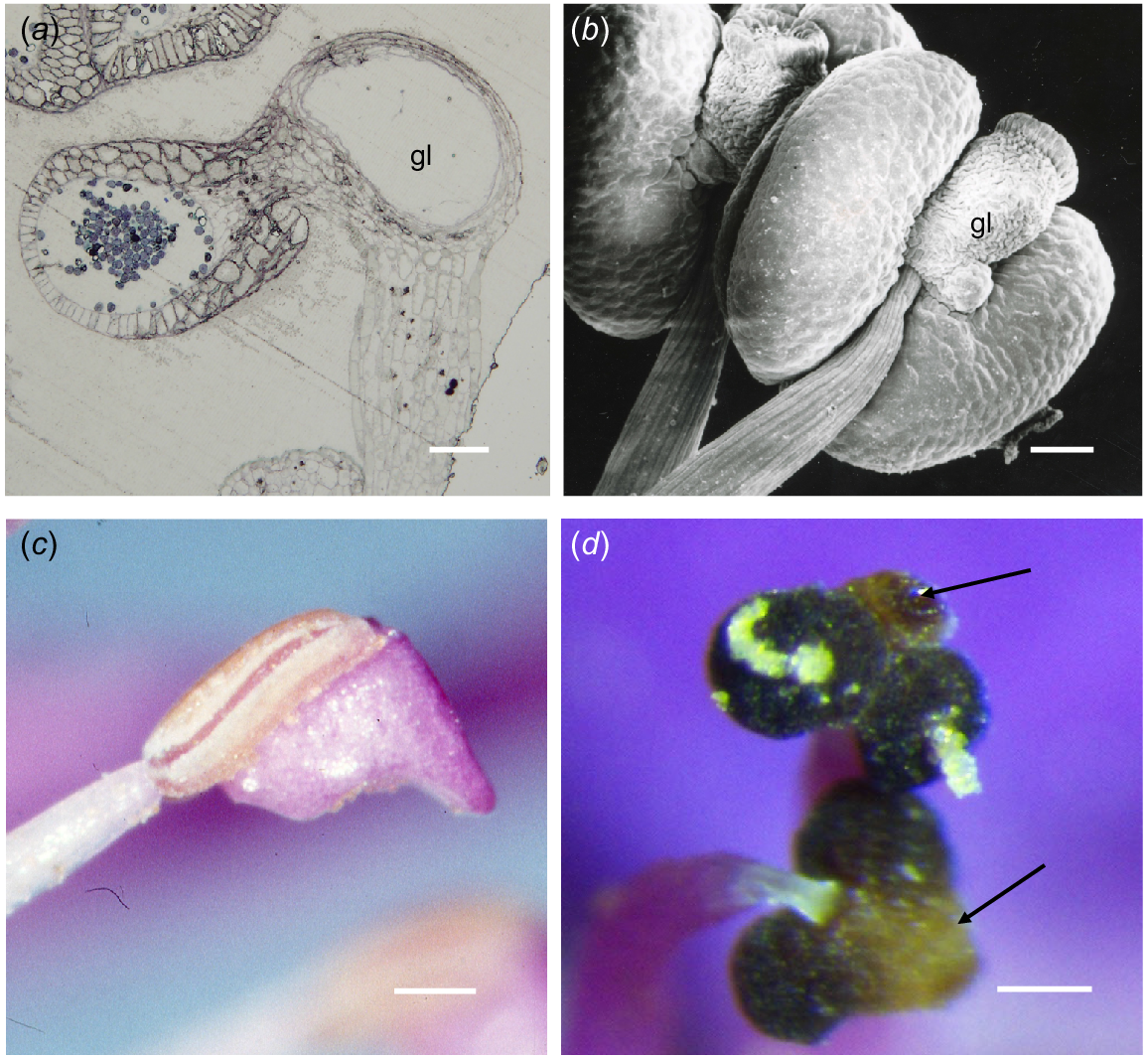
Anthers are tetrasporangiate, but mature as two locular. The glands were attached to the upper part of the connective distal to the point of filament attachment (Fig. 3c). A very thin epidermis (20.7 × 3.5–13.8 × 3.5 μm) covered the thecae and cells become slightly larger over the gland area. Under the epidermis, the endothecium was a single layer of thin-walled cells (17.3 × 27.6–6.9 × 13.8 μm) surrounding the loculi. There were three gland cavities with only epidermal cells (3.45 × 13.8 μm) and no hypodermis enclosing the gland and slightly larger equidimensional cells in the connective (Fig. 3d).
In this species, the anther was orientated at 90° to the filament and the two thecae were borne on the proximally extended connective containing the gland. The gland had a projecting mucro between the thecae (Fig. 3e), which may be where oil is exuded at anthesis.
The stamens of this genus were characterised by the very broad filament that in some species exceeded the anthers, but in R. crassifolia the filament extension was shorter (Fig. 3f). The gland was globular and small, emphasising that it was attached on part of the connective and not the filament.
Calytrix anthers have variably shaped apical projections that in some cases cover a globular gland. In C. aurea, the projection was urn-shaped (Fig. 4b), but in C. duplistipulata and C. decandra (Fig. 4c), there was a ‘phryngian cap-like’ addition to the back of the connective (P. G. Ladd, pers. obs., Table S1).
Gland chemical contents
The details of the six species investigated are shown in Table 2. The oil composition of the leaves from the six species reflects their taxonomic relationships. All species had α pinene, with additional different compounds specific to the different genera. It is notable that the three Verticordia species have eremophilene, despite V. grandis being in a different section from the other two species.
Species | Eucalyptus conferruminata | Leptospermum laevigatum | Chamelaucium uncinatum | Verticordia grandis | Verticordia nitens | Verticordia aurea | |||||||
---|---|---|---|---|---|---|---|---|---|---|---|---|---|
Tribe | Eucalypteae | Leptospermeae | Chamelaucieae | Chamelaucieae | Chamelaucieae | Chamelaucieae | |||||||
With pollen presenter | No | No | Yes | Yes | No | No | |||||||
Oil expressed from anther | No | No | Yes | No | Yes | Yes | |||||||
Plant organ | Anther | Leaf | Anther | Leaf | Anther | Leaf | Anther | Leaf | Anther | Leaf | Anther | Leaf | |
Oil compounds | |||||||||||||
α pinene | 27 | 36 | 78 | 39 | 17 | 78 | 44 | 65 | |||||
β pinene | 14 | ||||||||||||
1-8-cineole | 46 | 39 | |||||||||||
guiacol | 7 | 10 | |||||||||||
Unknown 1 (10,573) | 5 | ||||||||||||
Unknown 2 (10,949) | 21 | ||||||||||||
piperitone | 100 | ||||||||||||
Unknown 3 (18,670) | 50 | ||||||||||||
trans-Caryophyllene | 5 | ||||||||||||
Unknown 4 (19,300) | 5 | ||||||||||||
Unknown 5 (20,740) | 34 | ||||||||||||
Eremophilene | 19 | 41 | 23 | ||||||||||
β bisabolene | 5 | ||||||||||||
Unknown 5 (23,374) | 5 | ||||||||||||
Unknown 6 (23,533) | 7 | 3 | |||||||||||
Unknown 7 (23,880) | 26 | ||||||||||||
Unknown 8 (23,936) | 22 | 58 | |||||||||||
Unknown 9 (24,707) | 9 | ||||||||||||
Isopentyl palmitate* | xxx | ||||||||||||
Isopentyl stearate* | xx | ||||||||||||
Isopentyl eicosenate* | xxx | ||||||||||||
Isopentyl arachidate* | xxxx | ||||||||||||
Isopentyl docosenoate* | xxxx | ||||||||||||
Isopentyl behenate* | xxxx |
Numbers in the table indicate percentage of oil in the extract (only compounds with greater than 5% representation in at least one organ are listed). Unknown compounds have yet to be determined and are listed by an increasing molecular weight. The isopentyl compound records (*) are derived from Houston et al. (1993) and the number of x’s indicates the relative abundance of the compound in the extract.
Both E. conferruminata and L. laevigatum retain oil in the anther glands and the composition is similar between the leaves and the anthers for E. conferruminata, but only α pinene was in both organs for Leptospermum and occurs in leaves but not anthers of the other species. In the other four species, no compounds were shared between the leaves and anthers, although C. uncinatum contained a small percentage of β pinene rather than the α pinene, which occurs in the leaf. Although there was considerable similarity in the leaf compounds between species, there was virtually no similarity in the compounds in the anthers except for one compound shared between E. conferruminata and L. laevigatum and a different one between L. laevigatum and C. uncinatum. The most closely related species (V. aurea and V. nitens) shared no major anther compounds despite very similar leaf chemistry.
Discussion
Anther glands are almost ubiquitous in the Myrtaceae (see Schmid 1976) and are a plesiomorphic character, because Heteropyxis natalensis, which is sister to all Myrtaceae (Thornhill et al. 2015), also has anthers with an apical gland. The main variation in anther-gland form is related to how the gland is attached to the connective or how completely it is contained within the connective. A number of categories of anthers can be recognised and are listed in Table 1. Anther-gland and overall anther morphology in most species is uniform with the ancestral form being tetrasporangiate, which may be reduced to two loculi at maturity and dehisce by two longitudinal slits. The gland is globular and located at the top of the connective distal to where the filament joins the connective or, in some species, the gland is contained within the connective such as in Eucalyptus and associated genera where the gland is more often not narrowly attached but is incorporated in the upper part of the connective and does not exude oil. In E. leucoxylon, the anther is reported to lack a gland (Chippendale 1988), but, as shown in Fig. 1d, the gland is certainly present but completely enclosed in the connective. In all the species with anthers as the main floral advertisement, oil is not released from the secretory cavity and this is also the case in many of the flowers with petals, such a Leptospermum, Tristaniopsis, Taxandria.
There is more structural diversity in the Chamelaucieae anthers than in the other groups and anther form is important taxonomically (Rye 2009). However, the majority still have the plesiomorphic type of anther with a free to relatively free connective gland at the apex of the anther (Rye et al. 2020). Unlike in most other Myrtaceae, the advertising components of the flower in the Chamelaucieae are petals and the anthers are less obvious than in species of most other tribes. Many species have geniculate anthers in a ring facing towards the centre of the flower and anther dehiscence is introse. In general, anther dehiscence is related to desiccation (Schmid 1976) and, in most Myrtaceae, pollen is relatively sticky and exposed by retraction of the edges of anther thecae along a vertical slit. However, in many of the Chamelaucieae species, the pollen is extruded from a pore on the face of each theca in long strands, like paste from a tube. In these anthers the thecae appear to contract as they dry, causing the pollen, held together by tapetal material, to be extruded. Species with Anther types E and F may exude oil from the secretory cavity at anthesis but only in some taxa (Babingtonia, Rye 2015; Thryptomene) has it been noted that the oil and pollen mix. The oil does not seem to enter the loculi of the anther but is exuded separately from the pollen. The gland of Thryptomene is described as large and free (Rye et al. 2020), whereas in some species it is columnar to oval in shape and projects from the apex of the connective between the thecae. In T. calycina, it has been suggested the pollen–oil mixture provides food for pollinators (Beardsell et al. 1989). Because anthers of many of the taxa in the Chamelaucieae face towards the centre of the flower, pollen and oil could be separately applied to flower visitors when they seek nectar from surface of the hypanthium below the ring of stamens. However, in many Thryptomene species there is very little oil exuded, so mixing of oil and pollen is unlikely (Fig. 4d). Rye et al. (2020) described the gland in Baeckea as narrowly attached to the connective (free), whereas in Baeckea sp. Murchison River (Fig. 3e), it is incorporated in the connective and the thecae are borne on the front (dorsal side) of the gland–connective complex.
In the phylogeny of the Chamelaucieae (Rye et al. 2020), Homalocalyx, Calytrix and Ochrosperma are sister to the rest of the tribe. Unlike in others of the tribe, anthers in Calytrix and Homalocalyx are usually abundant and obvious as in most Myrtaceae flowers. Anther glands of Calytrix can be unusually shaped, such as urn-shaped (Fig. 3f), ‘peaked cap’-shaped with non-glandular tissue surrounding a gland at the apex of the connective (e.g. C. duplistipulata) or small and globular depending on the species, but oil is never exuded from the gland.
In Chamelaucium, Darwinia and Verticordia, glands (when present) are usually comparatively large in relation to those in other genera. In species with Anther categories E and F and a pollen presenter, the anthers grow up to surround the apex of the style in bud and the secretory cavity contents and pollen are combined and deposited on the pollen-presenter hairs before the flower opens. In the Verticordia–Chrysoma group, all species lack a pollen presenter (George 1991) and the pollen and oil combine in the apical extension of the connective as the flower opens. In other sections of the genus of the 42 species examined, 43% had a pollen presenter and an anther gland, 45% had a pollen presenter and either no or a very diminutive gland and 12% had neither a gland nor a pollen presenter (Ladd et al. 1999). Most species in Darwinia and Chamelaucium have both a pollen presenter and a large anther gland. In the species with a pollen presenter and anther gland, the combination of oil and pollen aids the adherence of the mixture to the pollen-presenter hairs. However, for most of the species of Verticordia and a number of Darwinia with a pollen presenter and no anther gland, the pollen is still deposited on the pollen presenter hairs in a viscous fluid entirely produced from the anther loculi. V. picta is an exception where pollen is not loaded onto the few sparse pollen-presenter hairs of the style, but is displayed directly on the anthers but is still contained in a liquid on the face of the anther.
Anther morphology is considered to support the monophylly of subtribe Hysterobaeckeinae (Rye et al 2020), but there is considerable variation in the glands of species in the group, from anthers with no apparent gland in Balustion to the anthers of Babingtonia where the gland occupied almost the whole length of the connective. In Hysterobaeckea and some Scholtzia, there is also a large connective containing the gland at the apex of the filament but, unlike in Babingtonia, the thecae are located on the front of the connective, giving the anthers a ‘hammer-like’ morphology. It is unclear whether oil and pollen are combined at anthesis. The group is the most species rich in the tribe and with morphologically diverse flowers. The diversity of anther form may have increased the rate of speciation owing to pollinator specificity (Rye et al. 2020).
Oil-secreting flowers are uncommon in the flowering plants, but flowers offering fatty oils occur in genera from 10 families of angiosperms only distantly related to the Myrtaceae (Buchmann 1987), including, for example, the Solanaceae (Cosacov et al. 2008), Scrophulariaceae (Steiner and Whitehead 2002), Iridaceae (Cocucci and Vogel 2001). In some Dalechampia, the oil products (as resins) are used by pollinating bees as nesting materials. (Armbruster et al. 1997).
Oil was examined in only six species, but the intention was to compare the oil content of leaves with that of anther glands in species from several different Myrtaceae tribes. E. conferruminata contained 1-8 cineole, which is consistent with other studies on eucalypts (Dunlop et al. 1997; King et al. 2006; Müller et al. 2022). This monoterpene was found in both the leaves and anthers but not in any of the other species. All species contained considerable amounts of α pinene in the leaves, which is consistent with other studies (Egerton-Warburton et al. 1998; Brophy et al. 2000), but only the eucalypt and Leptospermum also had it in the anthers, whereas β pinene occurred in C. uncinatum. It is notable that V. grandis anthers contained only piperitone and this compound has been found to have antimicrobial activity (Sivropoulou et al. 1995), so may protect the pollen from microbial degradation while it is on the pollen presenter.
The similarity and disparity in anther-gland contents in the different species indicates that oil glands in different parts of the plant of some species can produce different compounds. Oil composition varies among species (Dunlop et al. 1997; Brophy et al. 2000) and in leaves of Eucalyptus polybractea varied among plants and, within a plant, there was variation in oil composition among glands (King et al 2006). Thus, variation in oil composition is common in the Myrtaceae and could be influenced by selective forces related to protection from herbivory and, in the case of flowers, selective forces related to pollination.
Although it has not been specifically shown in this study, namely, that the contents of the anther glands, which retain their oil at maturity, deter herbivores, it seems likely they would from their similarity to the leaf oils. Thus the first hypothesis seems to be supported by the data for Eucalyptus and Leptospermum in this small sample of species. The brush blossom-type of flower, such as is common in most Myrtaceae, is considered to be part of a generalist pollination system. Beetles could be common visitors and many come primarily to eat flower parts (Armstrong 1979 illustrated the beetle Eupoecila australasiae at the cup-like flower of Angophora hispida). The anther glands would be useful for protection in taxa with brush blossoms. However, in more specialised pollination syndromes, such as melitophilly, the pollinators are unlikely to consume plant parts, so that the protective function of anther glands against herbivores would be redundant.
The second hypothesis was partially supported by the plant chemical data for the other species. Unfortunately, there is a lack of detail available for the pollinators of C. uncinatum and V. grandis. In C. uncinatum, the gland oil mixes with the pollen to aid adhesion to the pollen presenter; however, it is not known whether the pollen–oil mixture is a food source for pollinators, could be distasteful or is simply ignored. Flower visitors include a number of fly taxa, six bee species and a thynnid wasp at C. uncinatum (Lochman n.d.; Houston 2000), whereas honeyeater birds (Family Meliphagidae) and the honey possum (Tarsipes rostratus) have been recorded at the red flowers of V. grandis (McNee et al. 1994; Barret 1995). Both flower types have copious nectar and it is assumed this is the main reward for visitors.
There is more detailed information on Verticordia aurea and V. nitens (Houston et al. 1993). The most prevalent visitors to these species are solitary bees in the Colletidae. Euryglossa morrisoni forages exclusively on V. nitens, and E. aureophila exclusively on V. aurea. Both males and females visit the flowers. Pollen (from the thecae) and oil (from the gland) is combined in a spout-like structure at the apex of the anther. Only the females harvest oil and pollen from these apical processes to feed brood (Houston et al. 1993). In this the system differs from that in Dalechampia as the chemicals must be sufficiently modified to be a food rather than a poison. In euphorbiaceous vines the triterpenes are not ingested and are little modified from their protective resin function which allows them to be used as nest cement but not for food (Armbruster et al. 1997).
The change of gland function from a protective role to production of a food source for pollinators may be advantageous to the plant because it allows a stronger tie of the pollinator to the flower type. High specificity could be attained by production of particular chemicals for particular pollinating species such as seems to be the case in the very close association between Verticordia aurea, V. nitens and their pollinators.
It is possible that there is an intermediate step between the gland as protection for the anther and the gland as producer of reward oil. In taxa with a pollen presenter the oil may be a protection for the pollen if it is distasteful to pollinators or an antibacterial agent. There is some possibility that this may be the case in C. uncinatum in which the anther-gland oil contains a proportion of β pinene and shares the compound unknown eight with L. laevigatum. Further analysis is needed to clarify this possibility.
Armbruster (1993) was cautious about extending his ideas about biochemical exaptations preparing the way for changes in pollination systems in other plant groups. However the Myrtaceae seems to be another family in which protective biochemicals have been modified into either pollinator reward chemicals or at least have had their role changed to facilitate pollination efficiency. The Chamelaucieae is particularly species rich in Western Australia in the mediterranean to arid climatic region. For instance Verticordia has over 90 species in the south-west of Western Australia (George 1991), with a diversity of anther- and pollen-presenter forms (Ladd et al. 1999, 2000). Studies such as those of Houston et al. (1993) demonstrate very tight relationships between pollinators and plant species (oligolectic relationships) similar to those in Dalechampia. It is informative that similar processes seem to be operating in two highly species-rich areas, namely, the tropics and the Mediterranean climatic region, despite the very different environments.
Data availability
All data are included in the paper and supplementary information or from already published sources.
Acknowledgements
The author thanks R. Greening who chemically analysed the plant material, G. Thomson who cut the anatomical sections and M. Trudgen for identification of difficult Chamelaucieae species.
References
Armbruster WS (1993) Evolution of plant pollination systems: hypotheses and tests with the neotropical vine Dalechampia. Evolution 47, 1480-1505.
| Crossref | Google Scholar | PubMed |
Armbruster WS, Howard JJ, Clausen TP, Debevec EM, Loquvam JC, Matsuki M, Cerendolo B, Andel F (1997) Do biochemical exaptations link evolution of plant defence and pollination systems? Historical hypotheses and experimental tests with Dalechampia vines. The American Naturalist 149, 461-484.
| Crossref | Google Scholar |
Armstrong JA (1979) Biotic pollination mechanisms in the Australian flora: a review. New Zealand Journal of Botany 17, 467-508.
| Crossref | Google Scholar |
Barret G (1995) Pollen loads of vertebrates in heath vegetation during autumn at Eneabba. Western Australian Naturalist 20, 155-158.
| Google Scholar |
Bean AR (1995) Reinstatement and revision of Triplarina Raf. (Myrtaceae). Austrobaileya 4, 353-367.
| Google Scholar |
Beardsell DV, Williams EG, Knox RB (1989) The structure and histochemistry of the nectary and anther secretory tissue of the flowers of Thyryptome calycina (Lindl.) Stapf (Myrtaceae). Australian Journal of Botany 37, 65-80.
| Crossref | Google Scholar |
Brophy JJ, Goldsack RJ, Punruckvong A, Bean AR, Forster PI, Lepschi BJ, Doran JC, Rozefelds AC (2000) Leaf essential oils of the genus Leptospermum (Myrtaceae) in eastern Australia. Part 7. Leptospermum petersonii, L. liversidgei and allies. Flavour and Fragrence Journal 15, 342-351.
| Crossref | Google Scholar |
Buchmann SL (1987) The ecology of oil flowers and their bees. Annual Review of Ecology and Systematics 18, 343-369.
| Crossref | Google Scholar |
Cardel YJ, Koptur S (2010) Effects of florivory on the pollination of flowers: an experimental field study with a perennial plant. International Journal of Plant Science 171, 283-292.
| Crossref | Google Scholar |
Carr SGM, Carr DJ (1969) Oil glands and ducts in Eucalyptus L’Hérit. I. The phloem and the pith. Australian Journal of Botany 17, 471-513.
| Crossref | Google Scholar |
Cocucci AA, Vogel S (2001) Oil-producing flowers of Sisyrinchium species (Iridaceae) and their pollinators in southern South America. Flora 196, 26-46.
| Crossref | Google Scholar |
Cosacov A, Nattero J, Cocucci AA (2008) Variation of pollinator assemblages and pollen limitation in a locally specialised system: the oil-producing Nierembergia linariifolia (Solanaceae). Annals of Botany 102, 723-734.
| Crossref | Google Scholar | PubMed |
Dafni A, Bernhardt P (1990) Pollination of terrestrial orchids of southern Australia and the Mediterranean region, systematic, ecological and evolutionary implications. Evolutionary Biology 24, 193-252.
| Google Scholar |
Dunlop PJ, Bignell CM, Hibbert DB (1997) Use of gas chromatograms of the essential leaf oils of the genus Eucalyptus for taxonomic purposes. Australian Journal of Botany 45, 1-13.
| Crossref | Google Scholar |
Dörken VM, Parsons RF (2018) The foliar change in two species of Melaleuca (Myrtaceae): a morphological anatomic and ontogenetic approach. Trees 32, 1013-1028.
| Crossref | Google Scholar |
Egerton-Warburton LM, Ghisalberti EL, Burton NC (1998) Intergeneric hybridism between Chamelaucium and Verticordia (Myrtaceae) based on analysis of essential oils and morphology. Australian Journal of Botany 46, 201-208.
| Google Scholar |
Forrester DL, Endara M-J, Soule AJ, Younkin GC, Mills AG, Lokvam J, Dexter KG, Pennington RT, Kidner CA, Nicholls JA, Loiseau O, Kursari TA, Coley PD (2023) Diversity and divergence: evolution of secondary metabolism in the tropical tree genus Inga. New Phytologist 237, 631-642.
| Crossref | Google Scholar |
George AS (1991) New taxa, combinations and typifications in Verticordia (Myrtaceae: Chamelaucieae). Nuytsia 7, 231-393.
| Google Scholar |
Houston TF, Lamont BB, Radford S, Errington SG (1993) Apparent mutualism between Verticordia nitens and V. aurea (Myrtaceae) and their oil-ingesting bee pollinators (Hymenoptera, Colletidae). Australian Journal of Botany 41, 369-380.
| Crossref | Google Scholar |
Johnson SD (2010) The pollination niche and its role in the diversification and maintenance of the southern African flora. Philosophical Transactions of the Royal Society B: Biological Sciences 365, 499-516.
| Crossref | Google Scholar | PubMed |
Johnson SD (2016) Carrion flowers. Current Biology 26, R543-R576.
| Crossref | Google Scholar | PubMed |
Johnson LAS, Briggs BG (1984) Myrtales and Myrtaceae: a phylogenetic analysis. Annals of the Missouri Botanical Gardens 71, 700-756.
| Crossref | Google Scholar |
King DJ, Gleadow RM, Woodrow IE (2006) Regulation of oil accumulation in single glands of Eucalyptus polybractea. New Phytologist 172, 440-451.
| Crossref | Google Scholar | PubMed |
Ladd PG (1994) Pollen presenters in the flowering plant-form and function. Botanical Journal of the Linnean Society 115, 165-195.
| Crossref | Google Scholar |
Ladd PG, Parnell JAN, Thomson G (1999) Anther diversity and function in Verticordia DC. (Myrtaceae). Plant Systematics and Evolution 219, 79-97.
| Crossref | Google Scholar |
Leite VG, Mansano VF, Pansarin ER (2019) Presence of the anther gland is a key feature in pollination of the early-branching papilionoids Dipteryx alata and Pterodon pubescens (Leguminosae). Plant Biology 21, 1016-1023.
| Crossref | Google Scholar | PubMed |
List S, Brown PH, Walsh KB (1995) Functional anatomy of the oil glands of Melaleuca alternifolia (Myrtaceae. Australian Journal of Botany 43, 629-641.
| Crossref | Google Scholar |
Lochman J (n.d.) Lochman transparencies. Available at https://www.lochmantransparencies.com/
McCall AC, Irwin RE (2006) Florivory: the intersection of pollination and herbivory. Ecology Letters 9, 1351-1365.
| Crossref | Google Scholar | PubMed |
Müller ES, Hohn C, Pinheiro de Almeida MO, Patussi P, Calisto JFF, Floss P, Dias de Oliveira A, Simoes DA, de Sousa RR, Oliveira JV, Magro JD (2022) Influence of Eucalyptus spp. essential oils and tannins on herbivory. Research Society and Development 11(6), e14611628903.
| Crossref | Google Scholar |
Ollerton J (1996) Reconciling ecological processes with phylogenetic patterns: the apparent paradox of plant pollinator systems. Journal of Ecology 84, 767-769.
| Crossref | Google Scholar |
Rafferty C, Lamont BB (2021) Plant tannins and essential oils have an additive deterrent effect on diet choice by kangaroos. Forests 12, 1639.
| Crossref | Google Scholar |
Reichardt PB, Bryant JP, Mattes BR, Clausen TP, Chapin FS, III, Meyer M (1990) Winter chemical defense of Alaskan balsam poplar against snowshoe hares. Journal of Chemical Ecology 16, 1941-1959.
| Crossref | Google Scholar | PubMed |
Rye BL (2009) An interim key to the Western Australian tribes and genera of the Myrtaceae. Nuytsia 19, 313-323.
| Crossref | Google Scholar |
Rye BL (2014) An update to the taxonomy of some Western Australian genera of Myrtaceae tribe Chamelaucieae. 3. Thryptomene. Nuytsia 24, 269-306.
| Crossref | Google Scholar |
Rye BL (2015) A revision of the south-western Australian genus Babingtonia (Myrtaceae: Chamelaucieae). Nuytsia 25, 219-250.
| Crossref | Google Scholar |
Rye BL (2017) Revision of the south-western Australian genus Anticoryne (Myrtaceae: Chamelaucieae). Nuytsia 28, 205-215.
| Google Scholar |
Rye BL (2021) Austrobaeckea, a new south-western Australian genus of Myrtaceae (Chamelaucieae: Hysterobaeckeinae). Nuytsia 32, 173-197.
| Google Scholar |
Rye BL, Trudgen ME (2000) Aluta, a new Australian genus of Myrtaceae. Nuytsia 13, 345-366.
| Google Scholar |
Rye BL, Trudgen ME (2008) Seorsus, a new Gondwanan genus of Myrtaceae with a disjunct distribution in Borneo and Australia. Nuytsia 18, 235-257.
| Google Scholar |
Rye BL, Wilson PG, Heslewood MM, Perkins AJ, Thiele KR (2020) A new subtribal classification of the Myrtaceae tribe Chamelaucieae. Australian Systematic Botany 33, 191-206.
| Crossref | Google Scholar |
Schmid R (1976) Filament histology and anther dehiscence. Botanical Journal of the Linnean Society 73, 303-315.
| Crossref | Google Scholar |
Sivropoulou A, Kokkini S, Lanaras T, Arsenakis M (1995) Antimicrobial activity of mint essential oils. Journal of Agriculture and Food Chemistry 43, 2384-2388.
| Crossref | Google Scholar |
Steiner KE, Whitehead VB (2002) Oil secretion and the pollination of Colpias mollis (Scrophulariaceae). Plant Systematics and Evolution 235, 53-66.
| Crossref | Google Scholar |
Sánchez-Lafuente AM (2007) Corolla herbivory, pollination success and fruit predation in complex flowers: an experimental study with Linaria lilacina (Scrophulariaceae). Annals of Botany 99, 355-364.
| Crossref | Google Scholar | PubMed |
Thornhill AH, Ho SYW, Külheim C, Crisp MD (2015) Interpreting the modern distribution of Myrtaceae using a dated molecular phylogeny. Molecular Phylogenetics and Evolution 93, 29-43.
| Crossref | Google Scholar | PubMed |
Trudgen ME, Rye BL (2005) Astus, a new Western australian genus of Myrtaceae with heterocarpic fruits. Nuytsia 15, 495-512.
| Google Scholar |
Vasconcelos TNC, Prenner G, Bunger MO, de-Carvalho PS, Wingler A, Lucas EJ (2015) Systematic and evolutionary implications of stamen position in Myrteae (Myrtaceae). Botanical Journal of the Linnean Society 179, 388-402.
| Crossref | Google Scholar |
Wcislo WT, Cane JH (1996) Floral resource utilization by solitary bees (Hymenoptera: Apoidea) and exploitation of their stored foods by natural enemies. Annual Review of Entomology 41, 257-286.
| Crossref | Google Scholar | PubMed |
Wilson PG, Heslewood MM (2014) An expanded phylogenetic analysis of Sannantha (Myrtaceae) and description of a new species. Australian Systematic Botany 27, 78-84.
| Crossref | Google Scholar |