Long-term surveillance of Phytophthora cinnamomi reveals no evidence of increased detections and new insights for monitoring and management
T. J. Mason
A
B
C
D
E
Abstract
Phytophthora cinnamomi Rands is an introduced soil-borne pathogen that causes population decline in susceptible species and changes native vegetation compositions. Land managers require information about changing disease incidence, predisposing site factors and potential efficiencies in field identification of the disease. Previous sampling for P. cinnamomi in southern Sydney conservation reserves has provided a framework to monitor pathogen incidence.
We specifically asked: (1) Is the incidence of P. cinnamomi disease intensifying, decreasing or stable? (2) Is visual assessment of disease symptoms at the community or sentinel species level a good predictor of positive P. cinnamomi laboratory assays? (3) Is the incidence of P. cinnamomi related to access, vegetation type, human visitation or landscape position?
We assembled data from historic surveys in Dharawal Reserves in 2008 (n = 26) and 2014 (n = 110), and undertook additional surveys in 2022 (n = 110). We supplemented detections in 2008 with 2006–2008 records from other southern Sydney conservation reserves (n = 147). We used laboratory detection results, field observations of plant symptoms and environmental data to assess trends.
We did not find evidence of increased pathogen incidence over time; however, detection was spatially and temporally variable among sites. Proximity to waterways appeared to increase the likelihood of P. cinnamomi presence. We found differences between visual field assessment of disease expression and laboratory assay of pathogen detection. We observed marginal improvement in detection agreement when we subsetted the data to sites that explicitly identified Xanthorrhoea sp. as potential host species. A sample size analysis indicated that considerable increase in sampling effort would be required to detect consequential changes in the long-term P. cinnamomi status.
Landscape-scale monitoring of P. cinnamomi incidence requires greater sampling intensity and duration to support reliable inferences about trends. Locally focused management and monitoring efforts that consider susceptible sentinel species dynamics may inform the protection of specific assets at risk from infection by P. cinnamomi.
Keywords: diffuse invasion front, Hawkesbury sandstone vegetation, long-term monitoring, pathogen detection, sample size analysis, sentinel species, temporal study, water mould.
Introduction
Phytophthora cinnamomi Rands is a soil-borne water mould that is pathogenic to many native plant species in Australian vegetation communities (Garkaklis et al. 2004). McDougall et al. (2024) estimated that over 1580 Australian species may be at moderate to very high risk of extinction from disease caused by P. cinnamomi infection. The pathogen infects stem and root tissue of the host plant, and destroys host cell walls (Hardham 2005). Species within the families Ericaceae, Fabaceae, Proteaceae, Dilleniaceae and Asphodelaceae are particularly susceptible to pathogenic expression once infected (Cahill et al. 2008) and present drought-like symptoms such as foliar chlorosis, branch death and often plant death (Podger 1972). Advance of the disease front or infilling of contagion sites may occur slowly via (1) autonomous spread of motile zoospores that are attracted to plant roots (Hill et al. 1994; Garkaklis et al. 2004) or (2) passive spread via water transport in the soil (Garkaklis et al. 2004). Transport may also occur rapidly when humans introduce contaminated soil or plant material to new areas. Road and track construction, off-road vehicle activity, mining and poor soil hygiene in nursery stock have been implicated in rapid P. cinnamomi spread (Cahill et al. 2008; McDougall and Liew 2024). Bushwalkers and vertebrates such as native swamp wallabies and feral deer, may further spread the pathogen along the track network (Walsh et al. 2006). A subset of flora (e.g. graminoids) appears resistant to or unaffected by the pathogen (Podger 1972; Shearer et al. 2004a). The susceptibility of many abundant, rare or threatened native species to P. cinnamomi infection has potential to drive long-term changes in community composition (e.g. Wilson et al. 2024). Phytophthora cinnamomi may also alter faunal diversity by affecting shelter, forage and litterfall quality (Garkaklis et al. 2004), and impair pollination services, stream flow and water quality ecosystem functions (Dell et al. 2007). The seriousness of P. cinnamomi infection in Australian ecosystems has been recognised by its inclusion as a key threatening process under national (Commonwealth Environmental Protection and Biodiversity Conservation (EPBC) Act 1999) and state (NSW Biodiversity Conservation Act 2016) legislation. Invasive species represent the most commonly attributed threat type for threatened taxa in Australia (82% of threatened taxa affected) and P. cinnamomi infection is second only to the European rabbit (Oryctolagus cuniculus) in terms of the number of EPBC Act-listed threatened taxa affected (Kearney et al. 2019).
Phytophthora cinnamomi was likely introduced to Australia in the 19th century (Newhook and Podger 1972) and disease effects attributed to P. cinnamomi were identified in undisturbed bushland of the New South Wales (NSW) central coast region in the 1950s (Fraser 1956). Infection patterns and vegetation response across the continent appear location-specific. In the South-West Botanical Province of Western Australia, P. cinnamomi infection has been termed a ‘biological bulldozer’ (Carter 2004) with approximately 40% of described species susceptible to P. cinnamomi infection (Dundas et al. 2013 and references therein) and distinct invasion fronts observed (e.g. Shearer et al. 2004b). In Victoria, similar invasion fronts have been reported, followed by some evidence of community recovery (Weste et al. 2002). In Tasmania, the pathogen can affect broad areas of forest and heathland (Anon 2022a). However, on the temperate east coast mainland, infection appears comparatively diffuse, selective and patchy (McDougall and Summerell 2003). The pattern and rate of spread may depend on climatic and edaphic conditions, landscape position, disturbance regime, resident community and incidence of susceptible species (Cahill et al. 2008).
Periodic monitoring of an invasion threat allows conservation managers to identify invasion phases and allocate resources for control accordingly. If managers can identify cascading priorities of prevention, eradication, containment and protection (see Fleming et al. 2017), and identify the infection rate, scarce control resources may be expended efficiently. An understanding of spatio-temporal disease trends at the reserve- and landscape-scale will support decisions on management priorities and resourcing. Unfortunately, variability in P. cinnamomi spatial and temporal incidence, and impact contribute to uncertainty about the threat status of the pathogen in many parts of Australia. It is through ongoing monitoring at sites stratified across the national extent that changes in the conservation threat may be detected (O’Gara et al. 2005; Pyšek and Hulme 2005). Leveraging against existing survey effort improves monitoring efficiency and allows ongoing threat assessment. Yet monitoring of incidence and evaluation of threat status within conservation reserves have rarely been reported (Wilson et al. 2020). An additional problem is that requisite statistical power to detect trends is frequently omitted from ecological monitoring programs (Lindenmayer and Likens 2010): inadequate sample sizes undermine the capacity of monitoring programs to detect trends (e.g. Magurran et al. 2010).
Visual symptoms of plant damage or death frequently alert conservation managers to potential P. cinnamomi infection and disease expression. However, correspondence between detection in field soil samples and visual symptoms may be lagged: with increasing time since infection, the relationship between visual assessment and pathogen detection may weaken. In addition, visual indicators of plant damage or death may be attributable to other disease, drought or senescence (Newby 2014). Furthermore, different laboratory techniques have different detection performances (O’Brien et al. 2009). Dedicated monitoring for symptoms in sentinel species that are sensitive to disease expression may expedite detection (Summerell and Liew 2020) and increase visual assessment sensitivity. We seek to explore these expectations of complementarity between laboratory assay detection results and sentinel species approaches to disease monitoring.
Dharawal National Park, Nature Reserve and State Conservation Area (hereafter termed Dharawal Reserves) conserve 7248 ha of Hawkesbury Sandstone vegetation forming a mosaic of Eucalyptus woodland and forest, upland swamp, heathland and riparian scrub. Dharawal Reserves contribute to a network of conservation reserves in the Sydney Basin in eastern NSW. Phytophthora cinnamomi was sampled in Dharawal Reserves in 2008 (Suddaby 2008; Burgess et al. 2017) and 2014 (Craven and McDougall 2015). The pathogen was also surveyed in nearby Royal/Heathcote/Garawarra and Georges River/Kamay Botany Bay Reserves between 2006 and 2008 (Suddaby 2008). These studies demonstrated that the pathogen is widespread in the Sydney region. Geolocated plots in Dharawal Reserves from the 2014 study enabled us to conduct repeat sampling. Survey data from Reserves proximate to Dharawal Reserves increased sample size to elucidate both the status of the P. cinnamomi threat and how detection related to roadways, waterways, human visitation and landform position. All available data were used to investigate whether visual assessment of disease was a suitable proxy for laboratory soil analysis. We specifically asked: (1) Is the incidence of P. cinnamomi infection intensifying, decreasing or stable? (2) Are visual assessments of disease symptoms at the community level or where sentinel species are present, predictive of positive P. cinnamomi laboratory assays? (3) Is the incidence of P. cinnamomi related to roads or streams, vegetation type, human visitation rates or landscape position?
Methods
Study area
We used P. cinnamomi detection data from southern Sydney conservation reserves for this study (Table 1). The reserves were grouped geographically into (A) Dharawal Reserves; (B) Royal/Heathcote/Garawarra Reserves; and (C) Georges River/Kamay Botany Bay Reserves (Fig. 1e, Table 1). The study area is within the Sydney Basin and site geological characteristics relate to topographic elements: elevated plateaux support infertile sandy loams derived from Hawkesbury sandstones, Quaternary alluvium occurs in the headwaters of streams while unconsolidated sand or silt sediments are respectively associated with aeolian deposits or floodplain streams (Tozer et al. 2010). Vegetation structural categories were generalised as Sclerophyll Forest, Sclerophyll Woodland, Dry Heath, Scrub, Upland Swamp or Cleared land. The climate is temperate, and features warm summers and cool winters with mean annual rainfall of at least 1000 mm (http://www.bom.gov.au/climate/australia/cities).
Reserve group | Year sampled | Number of soil sampling sites | Visitation in 2008 | Environmental variable (vegetation type, distance to road, waterway and landform type) measured? | Visual health assessment made? | |
---|---|---|---|---|---|---|
Dharawal | 2008 A, 2014, 2022 | 147 | Low | Yes | Yes | |
GRKBB | 2008 | 110 | Medium | Yes | Yes | |
RHG | 2008 | 110 | High | Yes | Yes |
Detection of Phytophthora cinnamomi in Dharawal Reserves in (a) 2008, (b) 2014, (c) 2022 (labelled A). Detection of P. cinnamomi in (d) 2006–2008 for Royal, Heathcote, Garawarra Reserves (labelled B) and Georges River and Kamay Botany Bay National Parks (labelled C). All southern Sydney conservation reserves are shown in (e) with an inset map showing the study location in Australia.
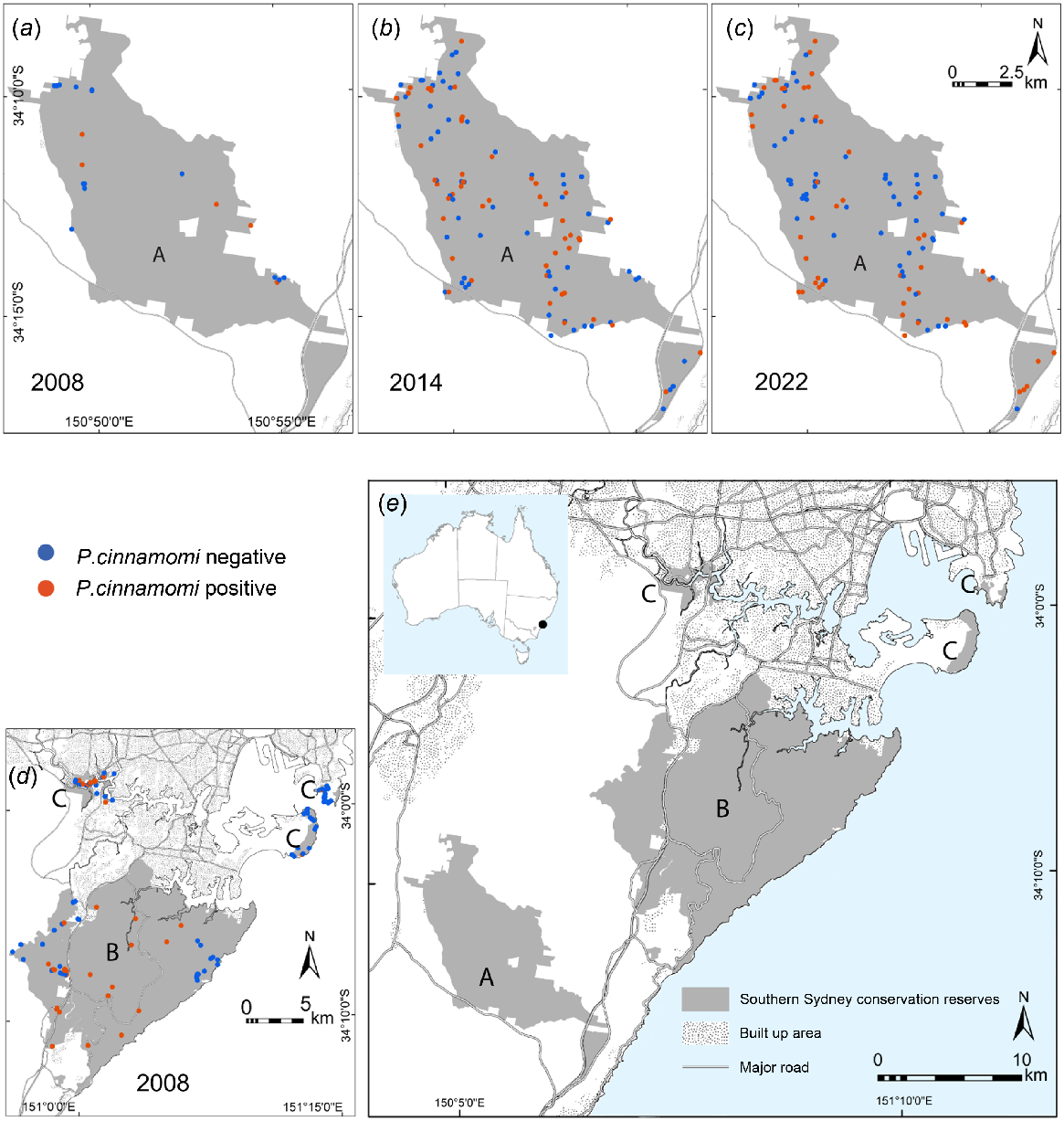
All of the conservation reserves are proximate to metropolitan Sydney. However, they experience different levels of human visitation. Estimated visitation to Royal National Park in 2008 was five-fold greater (3.6 million) than Kamay Botany Bay National Park (0.7 million). All other reserves in our study area were outside the top 20 visited conservation reserves (Anon 2019). We therefore categorised visitation as ‘high’ for Royal/Heathcote/Garawarra, ‘medium’ for Georges River/Kamay Botany Bay and ‘low’ for Dharawal Reserves.
Data sources and field collection
We compiled P. cinnamomi detection and field visual assessment data along with explanatory environmental variables from surveys undertaken in southern Sydney reserves during 2006–2008 (referred to as the ‘2008’ timepoint; n = 147; Burgess et al. (2017) and E. Liew unpubl. data). A new cohort of sites (n = 110) was sampled in a separate 2014 study focused on Dharawal Reserves (Craven and McDougall 2015). We subsequently resurveyed the 2014 sites (n = 110) between October 2021 and February 2022 (referred to as the ‘2022’ timepoint) to assess changes in disease incidence. Soil sampling followed Botanic Gardens of Sydney PlantClinic protocols (Suddaby and Liew 2008). When we resampled Dharawal Reserves in 2022, we navigated to site coordinates and attempted to sample beneath individuals identified in the 2014 survey. However, if the individual was dead or absent, we sampled to match the genus or family. We collected four to eight subsamples around the dripline of an identified plant individual. We removed leaf litter and sampled soil and root material to a depth of 10–15 cm. Soil collection was via a trowel in 2014 and via aluminium corers (2 cm diameter) in 2022. With both implements, sampling aimed to collect fresh root material to maximise P. cinnamomi detection (Newell 1998). Subsamples were bulked and mixed in a labelled clip seal bag. The bulked sample volume was approximated at ‘two cups of soil’ (Suddaby and Liew 2008). Samples were kept cool and delivered within 7 days (or 48 h in 2022) to the PlantClinic Laboratory at the Botanic Gardens of Sydney. Our field sampling followed strict hygiene protocols. Footwear and sampling equipment were treated with 70% ethanol (methylated spirits) after collection at each site to minimise contamination risk at subsequent sites. In 2022, we used a separate corer for each site with corers washed on a hot dishwasher setting and treated with 70% ethanol prior to reuse.
Visual site health assessments followed an ordinal scale at each site: healthy = 0–4% of all plants with symptoms of foliage senescence, branch or individual death; slight = 5–25% plants with symptoms; moderate = 26–50% plants with symptoms; severe = more than 50% plants with symptoms. Sites with slight to severe symptoms (>5%) were pooled and identified as displaying visual signs consistent with P. cinnamomi infection for our analyses. Sites were visually assessed at all sampling epochs and observers used the same visual assessment scale across surveys. The Dharawal Reserves sites sampled for P. cinnamomi presence in 2008 differed from those used in 2014 and 2022.
Laboratory techniques
Laboratory techniques used to detect P. cinnamomi at the Botanic Gardens of Sydney PlantClinic have evolved over the study period. The 2006–2008 and 2014 samples were processed by baiting samples with blue lupin (Lupinus angustifolius) seedlings (Pratt and Heather 1972) on Phytophthora-selective agar medium (PSM) followed by morphological identification by microscopy (Suddaby 2008). The 2021–2022 sampling period spanned a changeover in laboratory procedures at the PlantClinic: 32 samples were processed using a community DNA extraction approach from samples baited with blue lupin seedlings followed by Phytophthora specific Polymerase Chain Reaction (PCR) of the Ypt1 gene region (Schena et al. 2008). A total of 78 samples were processed using PARP 10 agar baits (Nakova 2010) that were modified by using clarified V8 as the basal medium. Detection relied on PCR using a Phytophthora genus specific Taqman™ assay (Laurence et al. 2023). We did not find any documentation comparing detection sensitivities for morphological and PCR identification at the PlantClinic.
Data analysis
We analysed P. cinnamomi incidence over time using the glmmTMB package (Brooks et al. 2017). We fitted a generalised linear mixed model with P. cinnamomi detection as the dependent variable. We used a spatially structured random effect to account for spatial correlation and a random effect for site to account for planned repeated measurements in Dharawal Reserves. We included vegetation type (Forest, Woodland, Heath, Scrub, Swamp, Cleared) as a random effect because the fixed effect was not computationally stable (Oberpriller et al. 2022). Year (2008, 2014, 2022) was a fixed effect, however only Dharawal Reserves were sampled at every time point. Other reserves were sampled at the first time point only. Further fixed effects were Visitation rate, Distance to road (m), Distance to waterway (m) and Landform type ((i) ridgetops, (ii) upper and mid-slopes, (iii) lower-slopes and in-filled valleys and (iv) rises or long gentle foot slopes and alluvial deposits) (Landform (LF7) modelling of NSW – ver. 1 Draft (Murphy et al. 2005)). We report estimated marginal means with 95% confidence intervals for probability of P. cinnamomi incidence using the emmeans package (Lenth 2022) — that averages across the categorical fixed effects in the model — and likelihood ratio tests. We conducted a sensitivity analysis by fitting a model without a spatial term to assess the degree to which effects attributable to these covariates were confounded by spatial proximity (Hodges and Reich 2010).
To evaluate agreement of laboratory soil sample results with the field visual assessment of standing vegetation, we used the receiver-operating characteristic (ROC) curve and the area under the ROC curve (AUC) using the ‘pROC’ package (Robin et al. 2011). A perfect ROC curve is denoted by AUC = 1 and a non-discriminant ROC curve is denoted by AUC = 0.5 (Robin et al. 2011). Xanthorrhoea species are particularly susceptible to infection damage (Keith et al. 2012; Summerell and Liew 2020) therefore we also subsetted the data to sites where Xanthorrhoea sp. was an identified site component to explore the utility of Xanthorrhoea species as disease indicators. All these analyses were undertaken using R ver. 4.1.2 (R Core Team 2021).
We performed a sample size analysis to inform future sampling effort for P. cinnamomi detection. We sought to understand the detectable change using a recent sample size (n = 110) and a larger but plausible sample size (n = 300) in Dharawal Reserves. We applied the 2022 estimated prevalence as the baseline. The test statistic used a two-sided Z-Test with unpooled variance, a significance level of 0.05 and power of 90%. The analysis used the PASS 2020 v 20.0.10 software.
Results
Phytophthora cinnamomi detection over time
Sites within the Dharawal Reserves that were subject to repeat surveys transitioned in detection status over time (Fig. 1a–c). In 2014, P. cinnamomi presence was detected at 50 of 110 sites (0.45 as a proportion) with a further five sites positive for the Phytophthora genus. In 2022, P. cinnamomi presence was detected at 51 of the same 110 sites (0.46 as a proportion) with a further site positive for the Phytophthora genus. Overall detection appeared stable (, P = 0.330; Fig. 2), however a positive status at individual sites changed over time reflecting either the passage of a disease front or variable detection. In 2008, a separate cohort of sites in Dharawal Reserves were positive for P. cinnamomi at 6 of 26 locations (0.23 as a proportion).
Estimated probability of Phytophthora cinnamomi presence (±95% CI) over time in Dharawal Reserves averaged over landform type. Presence probabilities for Georges River/Kamay Botany Bay National Parks and Royal/Heathcote/Garawarra Reserves are available for the first time point only.
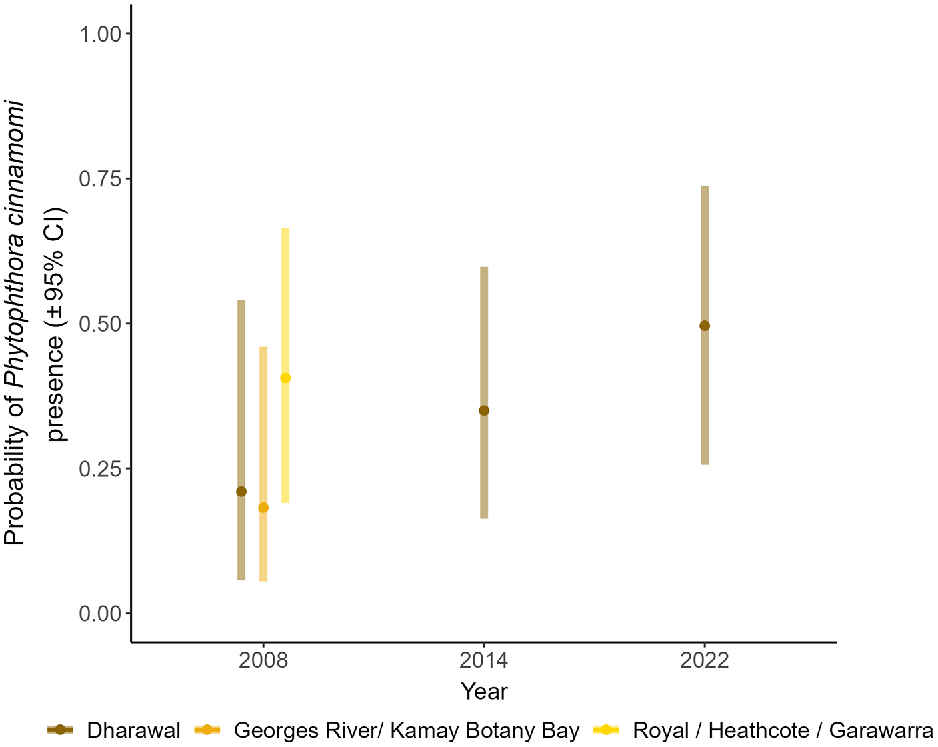
We assumed laboratory detection methods were comparable across the longitudinal study, as has been found in a related formal comparative study (Sena et al. 2018a). Both raw and estimated means notably appeared to increase between 2008 and 2022 but the large confidence intervals in Fig. 2 indicate considerable trend uncertainty.
Inconsistent pathogen detection and the role of sentinel species
Across 2008, 2014 and 2022, we found discrepancies between field observations of site-level P. cinnamomi symptoms and detection via laboratory analyses. Agreement between detection methods was 38% when laboratory analyses were negative and 57% when laboratory analyses were positive for P. cinnamomi. In exploratory analyses, we found that performance of field visual assessment was poor (AUC = 0.46 (95% CI: 0.41–0.52)). When we focused only on sites where Xanthorrhoea sp. was identified as a host species, performance of visual assessment improved (AUC = 0.61 (95% CI: 0.47–0.75)). Agreement between detection methods increased to 52% when laboratory analyses were negative and 64% when laboratory analyses were positive for P. cinnamomi.
Environmental variables as predictors of P. cinnamomi detection
Based on our primary model that contained a spatially structured random effect, we found no evidence of a relationship between P. cinnamomi detection and landform, vegetation type, distance to waterway, distance to roadway or visitation (based on conservation reserve clusters) (P > 0.10). We also fitted a model without a spatially structured random effect as a sensitivity analysis that explored spatial confounding. This model had larger AIC than the primary model. The sensitivity analysis yielded evidence of a relationship between distance from waterway and detection probability: the odds of P. cinnamomi detection increased by 19% (95% CI: 2–39%) for every 100 m towards a waterway (; P = 0.010). Confounding between spatially structured covariates and the spatial random effect may obscure the effect of covariates (Hodges and Reich 2010) and we suspect this has occurred in our primary analysis. Distance to waterway was the only effect that differed qualitatively between models.
The primary model did not provide evidence of human visitation effects across the reserve categories (, P = 0.416). The mean estimated probability of P. cinnamomi detection in Dharawal Reserves (with the lowest visitation) was 0.21 (±95% CI: 0.06–0.54) in 2008, 0.35 (±95% CI: 0.16–0.60) in 2014 and 0.50 (±95% CI: 0.26–0.74) in 2022. These estimates were averaged across landform type. In 2008 the infected proportions were 0.18 (±95% CI: 0.06–0.46) for the medium visitation reserves (Georges River/Kamay Botany Bay National Parks) and 0.41 (±95% CI: 0.19–0.67) for the highest visitation reserves (Royal/Heathcote/Garawarra Reserves) (Figs 1d and 2). A trend of positive detection prevalence and Reserve visitation may be obscured by large confidence intervals.
Estimating future sampling requirements to improve future monitoring
Given the uncertainty of trend estimates in the initial monitoring, we explored requirements of future monitoring to detect changes in P. cinnamomi presence, should these occur. Using the raw detection proportion of 0.46 in 2022, we found that a repeat of 110 samples at the next timepoint would only be able to detect an increase in pathogen presence of ≥47%. More intense sampling of size n = 300 — in each of two future sampling periods — would be more sensitive to increasing P. cinnamomi levels and could detect when pathogen incidence increased by ≥29%.
Discussion
Phytophthora cinnamomi detection over time
Infection by P. cinnamomi represents an ongoing threat within southern Sydney conservation reserves. There is uncertainty as to whether the threat is intensifying. The estimated marginal means for the proportion of P. cinnamomi positive sites in Dharawal Reserves suggest that prevalence may be increasing over time. However, this trend was statistically undetected and characterised by large confidence intervals likely reflecting limited sample size. Detection appeared spatially and temporally heterogeneous without evidence of clear disease fronts. Such patterns conform with other observations for NSW (McDougall and Summerell 2003). We did not find evidence of increasing P. cinnamomi incidence despite greater fluctuations in climatic moisture prior to the 2022 survey (e.g. below average annual rainfall in 2019 and above average annual rainfall in 2021), recent prescribed fire and wildfire activity (during the 2001–2002; 2005–2006; 2015–2016; 2018–2019; 2020–2021 fire seasons), and ongoing human visitation and feral animal activity.
Baseline monitoring of disease threat status is imperative for local, state and national conservation management. Reserve-level monitoring directs local suppression effort (e.g. Wilson et al. 2020) and contributes to state and national threat abatement planning and monitoring. A review of the Commonwealth Environmental Protection and Biodiversity Conservation Act 1999 found inadequate monitoring of key threatening processes (Samuel 2020) and therefore an incapacity to demonstrate protection of threatened species and communities. Ongoing monitoring of pathogen detections and consideration of spatial patterns in multiple reserves across the potential disease distribution would directly address the national threat status. However, our study has demonstrated that substantial within-reserve sampling effort is required to confidently observe changes in P. cinnamomi prevalence. Furthermore, sites must be revisited at ecologically relevant intervals: Botanic Gardens of Sydney protocols recommend revisits every 2 years or after high-risk disturbance such as fire or flood (Suddaby and Liew 2008). In addition, monitoring activities should be timed to maximise detection capacity. For example, teams should mobilize after wet periods — when zoospore activity is more likely — and avoid soil collection during drought conditions (Drenth and Sendall 2001).
Governance of monitoring programs must include evaluation, adaptation and calibration of methodological advancements to improve reporting outcomes (Lindenmayer et al. 2012). In our case, formal comparison of P. cinnamomi detection sensitivity when laboratory assay methodologies change will aid future interpretation of pathogen trend analysis.
Importantly, a P. cinnamomi threat status that is not clearly intensifying does not imply negligible or minimal ecosystem effects. Selective and cumulative elimination or damage of susceptible plant species has implications for biodiversity value, habitat provision and ecosystem function (Weste et al. 2002; Cahill et al. 2008). In addition, disease expression in vegetation communities may be compounded by multiple disturbances such as prescribed fire or wildfire (Metz et al. 2013; Moore et al. 2014). Interactions between disease incidence and climate change may disproportionately affect symptomless or moderately susceptible host species if climatic conditions become more favourable for P. cinnamomi (Thompson et al. 2014; Burgess et al. 2017).
Inconsistent pathogen detection and the role of sentinel species
Monitoring for P. cinnamomi disease generally involves both visual assessment of susceptible individuals for dieback symptoms and laboratory testing of soil samples (Summerell and Liew 2020). However, we found low congruence between field visual assessment and laboratory soil testing. Inconsistencies between detection methods may be explained by combinations of several factors. Firstly, host susceptibility or disease expression may be variable: the host may be infected by P. cinnamomi but appear asymptomatic (e.g. McDougall and Summerell 2003; Wan et al. 2019). While a suite of species has been identified as susceptible to dieback expression following P. cinnamomi infection (Cahill et al. 2008), researchers have increasingly reported susceptibility spectra within families, genera and species (Shearer et al. 2013). Alternatively, low congruence between field and laboratory findings may be explained by erroneous attribution of dieback to P. cinnamomi disease expression when water stress or other stressors may be the cause of symptoms (Jurskis 2005; Anon 2018). And where symptoms appear severe, P. cinnamomi infection may be the cause, however the pathogen may have migrated elsewhere and evade detection in soil samples (Weste and Marks 1987). Furthermore, P. cinnamomi is difficult to isolate from soil samples and may evade detection due to insufficient sampling (Pryce et al. 2002). Finally, laboratory detection may be imperfect with the testing process returning false negative (low specificity) or false positive (low sensitivity) results (Davison and Tay 2005; O’Brien et al. 2009).
While direct sampling of soil or plant material remains a centerpiece of monitoring P. cinnamomi incidence, this is expensive and difficult (Newby et al. 2019) and detection is uncertain under conditions of patchy disease expression. Indirect detection methods using remote sensing (Landsat satellite and orthorectified aerial imagery) have tracked temporal trends in Phytophthora dieback (Cardillo et al. 2018). However, low congruence between laboratory and on-ground visual assessment in our study suggests that aerial interpretation would be unreliable as a rapid detection approach for our coastal NSW study area. Multispectral and hyperspectral imagery have also successfully identified symptomatic vegetation. However identification of asymptomatic or marginally affected vegetation and separation of water stress and disease expression have proved challenging (Newby et al. 2019).
Sentinel species may differ across regions: in Sydney, members of the genus Xanthorrhoea may be candidates (Keith et al. 2012; Summerell and Liew 2020). These are potentially useful sentinels because they are long lived, conspicuous and susceptible. We found marginally greater alignment between pathogen detection and disease expression when we opportunistically analysed a subset of sites where Xanthorrhoea were identified as potential hosts (observers frequently did not distinguish among species within the genus). Xanthorrhoea hosts were often among several identified hosts and field observers did not attribute health response to single hosts. At present, we conclude that visual assessment is insufficiently informative to justify this as a sole monitoring approach. However, further research that explicitly links individual hosts and field health observations may clarify the efficacy of sentinel species in site selection for disease monitoring. We advocate a dual surveillance strategy for P. cinnamomi that explicitly measures the field condition of sentinel species to estimate disease expression and undertakes laboratory assay of the soil pathogen to identify disease potential. This dual approach will produce complementary insights into the dynamic status of the disease in the landscape and inform disease management.
Environmental variables as predictors of P. cinnamomi incidence
We found evidence that detection was higher proximate to waterways (in the non-spatial model) but was unrelated to road and track formation, vegetation type or landscape position. We postulate that, in the absence of active suppression, P. cinnamomi incidence may continue to migrate upslope from creek and drainage lines in southern Sydney conservation reserves. Other studies have demonstrated that waterways facilitate pathogen transport (von Broembsen 1984; Smith et al. 2009; Hüberli et al. 2013) and disease transmission may subsequently occur via direct root contact in the soil matrix (Hill et al. 1994). In light of our findings, water used for firefighting and road-building within uninfected catchments should be sourced from within the catchment (Hüberli et al. 2013) or from uninfected water sources (e.g. chlorinated mains water (Anon 2022b)). Additionally, creation of new vehicular and walking tracks should be avoided and unnecessary extant tracks should be closed where the pathogen is believed to be absent (e.g. Wilson et al. 2000).
Comparison of raw disease detection among low, medium and high visitation reserves (respectively Dharawal Reserves; Georges River/Kamay Botany Bay Reserves and Royal/Heathcote/Garawarra Reserves) suggests that disease incidence may increase with increasing human access. Consequently, areas considered disease-free should be subject to catchment-scale quarantine and hygiene measures.
A management strategy for Dharawal Reserves
Our study has found that P. cinnamomi is widely distributed in southern Sydney conservation reserves and that new detections within Dharawal Reserves have occurred within the monitoring period (2008–2022). Long-term studies have also indicated that P. cinnamomi infection causes elevated mortality rates among susceptible plant species (e.g. Weste 2003). However, there is considerable uncertainty about change in disease incidence and detection confidence at the site scale. This uncertainty makes management of the disease a challenge in eastern Australia where disease expression is typically diffuse (McDougall and Summerell 2003). Due to widespread and long-term detection of P. cinnamomi in southern Sydney and the limitations of current control methods (Sena et al. 2018b), we surmise that invasion has progressed beyond prevention, eradication and containment to the asset protection phase for management actions (Fleming et al. 2017). Nonetheless, ongoing monitoring is essential to firstly inform future management and containment of P. cinnamomi as a key threatening process and secondly protect natural assets. The outcomes of this study suggest how managers may improve confidence in threat status monitoring and provide indicative costs. A sample size analysis indicated that managers would need to increase the sample size from 2022 (n = 110) nearly three-fold (n = 300) — and over two time periods — to detect a 29% increase in Reserve-level P. cinnamomi presence. Such an increase in sampling effort has considerable resourcing implications. In 2023, the Botanic Gardens of Sydney PlantClinic laboratory costs were AUD175 per sample (https://www.botanicgardens.org.au/our-science/our-services/plantclinic-plant-disease-diagnostics). In addition, a two-person team budgeted nine field days in 2014 and 11 field days in 2022 to collect 110 samples across Dharawal Reserves.
The study has identified several pertinent management issues at the reserve level. A monitoring program that resamples sites on a 2–5 year rotation would detect changes in threat status more effectively than sampling at the 6–8 year interval of this study. In any case, escalating P. cinnamomi detection should trigger a reassessment of the prevailing management approach. Where the pathogen has been detected recently, managers should monitor the impacts and protect natural assets. Chemical control of P. cinnamomi, using phosphorous acid (phosphite), has been effective in mitigating population- and community-level impacts in Western Australia (Barrett and Rathbone 2018; Keighery et al. 2023). Phosphite can be sprayed or injected and induces defense responses in infected plants (Garkaklis et al. 2004). Barrett and Rathbone (2018) found that species assemblages and vegetation structure in phosphite-treated infested communities were more similar to healthy than infested non-treated communities. Keighery et al. (2023) found that Banksia anatona survival after fire was greater when seedlings were recurrently sprayed with phosphite compared with untreated seedlings. While phosphite application may improve ecosystem function in infected areas, its effectiveness under NSW climatic conditions and the optimal timing of application require further research. There may also be cumulative collateral impacts from repeated addition of phosphite to low-fertility soils such as Hawkesbury sandstone derived soils of the Sydney region (Lambers et al. 2013).
We suggest that control methods in disease-affected high-priority conservation areas could focus on discrete populations of slow-turnover susceptible species such as Xanthorrhoea resinosa. Xanthorrhoea species are ‘old growth’ components of forest, woodland and heath communities in Sydney conservation reserves (Regan et al. 2011; Wilson et al. 2020). Xanthorrhoea species may also be considered umbrella species (Noss 1990), providing habitat (e.g. Wilson et al. 2020) and forage — especially in the immediate post-fire environment (e.g. Brown and York 2017) — and ameliorating conditions (e.g. Petit and Dickson 2005) for many co-occurring species. The susceptibility of Xanthorrhoea species to P. cinnamomi damage and their conservation multiplier effects, qualify these as focal species (refer to Lambeck (1997) for a related discussion) for pathogen control and monitoring. Given the difficulty and expense of land-extensive pathogen detection and management, a monitoring and control approach that focuses on sentinel and umbrella species may be appropriate. Furthermore, phosphite trials (with careful observations of off-target damage) in discrete areas could prioritise Xanthorrhoea populations where P. cinnamomi is an active threat (Wilson et al. 2024).
Conclusion
Reliably detecting and monitoring changes in P. cinnamomi across reserves in eastern Australia is an enormous challenge. An increase in monitoring budgets with concomitant increases in sampling effort may allow conservation managers to better understand how this threatening process is changing over time. However, monitoring to date indicates that P. cinnamomi is widespread in southern Sydney. Therefore, in the absence of increased resource allocation, managers should focus on asset identification and protection actions. We believe that monitoring and control activities, undertaken in discrete areas and targeting populations of susceptible umbrella or threatened species, will focus P. cinnamomi detection and threat minimisation.
Data availability
Data are archived at https://doi.org/10.5281/zenodo.14399365 and R code is available at https://github.com/gordy2x/Mason_2024.
Declaration of funding
This project has been assisted by the NSW Government through the Environmental Trust (2018/SSC/0049) Program.
Acknowledgements
We acknowledge the Dharawal, Gadigal, Bidjigal and Darug people, who are the traditional custodians of the land on which this study was conducted. Fieldwork was conducted under Department of Planning and Environment Scientific Licence SL101124. Edward Liew, Rosalie Daniel and Theresa Suddaby contributed to soil sampling and data collation. We thank Matthew Laurence and the Botanic Gardens of Sydney PlantClinic for undertaking soil analyses. The manuscript draft was substantially improved with assistance from the Associate Editor and two anonymous reviewers.
References
Anon (2019) Annual visits to NPWS Managed Parks in New South Wales. Roy Morgan Research, Melbourne. Available at https://www.environment.nsw.gov.au/-/media/OEH/Corporate-Site/Documents/Research/Our-science-and-research/annual-visits-npws-managed-parks-nsw-state-final-report-august-2019.pdf
Anon (2022a) State of the forests Tasmania 2022 data report. Forest Practices Authority, Hobart, Tasmania. Available at https://fpa.tas.gov.au/__data/assets/pdf_file/0007/521548/Tas_SOF_data_report_2022_final_28_March_2023.pdf
Anon (2022b) Phytophthora (dieback) control environmental instruction. South Australia Department for Infrastructure and Transport, SADfIa Transport. Available at https://www.dit.sa.gov.au/documents
Barrett S, Rathbone D (2018) Long-term phosphite application maintains species assemblages, richness and structure of plant communities invaded by Phytophthora cinnamomi. Austral Ecology 43, 360-374.
| Crossref | Google Scholar |
Brooks ME, Kristensen K, van Benthem KJ, Magnusson A, Berg CW, Nielsen A, Skaug HJ, Mächler M, Bolker BM (2017) glmmTMB balances speed and flexibility among packages for zero-inflated generalized linear mixed modeling. The R Journal 9(2), 378-400.
| Crossref | Google Scholar |
Brown J, York A (2017) Fire, food and sexual deception in the neighbourhood of some Australian orchids. Austral Ecology 42, 468-478.
| Crossref | Google Scholar |
Burgess TI, Scott JK, McDougall KL, Stukely MJC, Crane C, Dunstan WA, Brigg F, Andjic V, White D, Rudman T, Arentz F, Ota N, Hardy GESJ (2017) Current and projected global distribution of Phytophthora cinnamomi, one of the world’s worst plant pathogens. Global Change Biology 23, 1661-1674.
| Crossref | Google Scholar | PubMed |
Cahill DM, Rookes JE, Wilson BA, Gibson L, McDougall KL (2008) Phytophthora cinnamomi and Australia’s biodiversity: impacts, predictions and progress towards control. Australian Journal of Botany 56, 279-310.
| Crossref | Google Scholar |
Cardillo E, Acedo A, Abad E (2018) Topographic effects on dispersal patterns of Phytophthora cinnamomi at a stand scale in a Spanish heathland. PLoS ONE 13(3), e0195060.
| Crossref | Google Scholar | PubMed |
Carter R (2004) Arresting Phytophthora dieback: the biological bulldozer. WWF and Dieback Consultative Council. Available at https://forestphytophthoras.org/sites/default/files/educational_materials/dieback_report.pdf
Craven P, McDougall K (2015) Occurrence of the plant pathogen Phytophthora cinnamomi at Dharawal National Park. Office of Environment and Heritage, Nowra. Available at https://www.ipcn.nsw.gov.au/resources/pac/media/files/pac/project-submissions/2020/10/dendrobium-extension-project-ssd-8194/20201215t170337/occurrence-of-phytophthora-cinnamomi-at-dharawal-np6271.pdf
Davison EM, Tay FCS (2005) How many soil samples are needed to show that Phytophthora is absent from sites in the south-west of Western Australia? Australasian Plant Pathology 34, 293-297.
| Crossref | Google Scholar |
Dundas SJ, Fleming PA, Hardy GESJ (2013) Flower visitation by honey possums (Tarsipes rostratus) in a coastal banksia heathland infested with the plant pathogen Phytophthora cinnamomi. Australian Mammalogy 35, 166-174.
| Crossref | Google Scholar |
Fleming PJS, Ballard G, Reid NCH, Tracey JP (2017) Invasive species and their impacts on agri-ecosystems: issues and solutions for restoring ecosystem processes. The Rangeland Journal 39, 523-535.
| Crossref | Google Scholar |
Fraser LR (1956) Phytophthora cinnamomi attacking native plants. Australian Plant Disease Recorder 8, 12.
| Google Scholar |
Garkaklis MJ, Calver MC, Wilson BA, Hardy GES (2004) Habitat alteration caused by an introduced plant disease, Phytophthora cinnamomi: a potential threat to the conservation of Australian forest fauna. In ‘Conservation of Australia’s forest fauna’. 2nd edn. (Ed. D Lunney) pp. 899–913. (Royal Zoological Society of New South Wales: Mosman)
Hardham AR (2005) Phytophthora cinnamomi. Molecular Plant Pathology 6(6), 589-604.
| Crossref | Google Scholar | PubMed |
Hill TCJ, Tippett JT, Shearer BL (1994) Invasion of Bassendean dune Banksia woodland by Phytophthora cinnamomi. Australian Journal of Botany 42, 725-738.
| Crossref | Google Scholar |
Hodges JS, Reich BJ (2010) Adding spatially-correlated errors can mess up the fixed effect you love. The American Statistician 64(4), 325-334.
| Crossref | Google Scholar |
Hüberli D, Hardy GESJ, White D, Williams N, Burgess TI (2013) Fishing for Phytophthora from Western Australia’s waterways: a distribution and diversity survey. Australasian Plant Pathology 42, 251-260.
| Crossref | Google Scholar |
Jurskis V (2005) Eucalypt decline in Australia, and a general concept of tree decline and dieback. Forest Ecology and Management 215, 1-20.
| Crossref | Google Scholar |
Kearney SG, Carwardine J, Reside AE, Fisher DO, Maron M, Doherty TS, Legge S, Silcock J, Woinarski JCZ, Garnett ST, Wintle BA, Watson JEM (2019) The threats to Australia’s imperilled species and implications for a national conservation response. Pacific Conservation Biology 25, 231-244.
| Crossref | Google Scholar |
Keighery G, Gosper CR, Barrett S, Coates D, Makinson RO (2023) The compounding impacts of disease and weeds after the 2019-20 wildfires on Australian vascular plants and communities. In ‘Australia’s megafires: Biodiversity impacts and lessons from 2019–2020’. (Eds L Rumpff, SM Legge, S van Leeuwen, BA Wintle, JCZ Woinarski) p. 512. (CSIRO Publishing: Melbourne)
Keith DA, McDougall KL, Simpson CC, Walsh JL (2012) Spatial analysis of risks posed by root rot pathogen Phytophthora cinnamomi: implications for disease management. Proceedings of the Linnean Society of NSW 134, B147-B179.
| Google Scholar |
Lambeck RJ (1997) Focal species: a multi-species umbrella for nature conservation. Conservation Biology 11, 849-856.
| Crossref | Google Scholar |
Lambers H, Ahmedi I, Berkowitz O, Dunne C, Finnegan PM, Hardy GESJ, Jost R, Laliberté E, Pearse SJ, Teste FP (2013) Phosphorus nutrition of phosphorus-sensitive Australian native plants: threats to plant communities in a global biodiversity hotspot. Conservation Physiology 1, cot010.
| Crossref | Google Scholar | PubMed |
Laurence MH, Mertin AA, Pang C, Wildman O, Daly AM, Summerell BA (2023) First report of Phytophthora plurivora in Australia. Australasian Plant Disease Notes 18, 33.
| Crossref | Google Scholar |
Lenth RV (2022) emmeans: estimated marginal means, aka least-squares means, R package version 1.7.2. Available at https://github.com/rvlenth/emmeans
Lindenmayer DB, Likens GE (2010) The science and application of ecological monitoring. Biological Conservation 143, 1317-1328.
| Crossref | Google Scholar |
Lindenmayer DB, Gibbons P, Bourke M, Burgman M, Dickman CR, Ferrier S, Fitzsimons J, Freudenberger D, Garnett ST, Groves C, Hobbs RJ, Kingsford RT, Krebs C, Legge S, Lowe AJ, McLean R, Montambault J, Possingham H, Radford J, Robinson D, Smallbone L, Thomas D, Varcoe T, Vardon M, Wardle G, Woinarski JCZ, Zerger A (2012) Improving biodiversity monitoring. Austral Ecology 37, 285-294.
| Crossref | Google Scholar |
Magurran AE, Baillie SR, Buckland ST, Dick JMP, Elston DA, Scott EM, Smith RI, Somerfield PJ, Watt AD (2010) Long-term datasets in biodiversity research and monitoring: assessing change in ecological communities through time. Trends in Ecology & Evolution 25, 574-582.
| Crossref | Google Scholar | PubMed |
McDougall KL, Liew ECY (2024) Dispersal of Phytophthora species by off-road vehicles in New South Wales. Australasian Plant Pathology 53, 63-65.
| Crossref | Google Scholar |
McDougall KL, Summerell BA (2003) The impact of Phytophthora cinnamomi on the flora and vegetation of New South Wales - a re-appraisal. In ‘Phytophthora in forests and natural ecosystems, 2nd International IUFRO Working Party 7.02.09 Meeting’, 30 September−5 October 2001, Albany, WA’. pp. 49–56. (Murdoch University: Albany, WA)
McDougall KL, Barrett S, Velzeboer R, Cahill DM, Rudman T (2024) Evaluating the risk to Australia’s flora from Phytophthora cinnamomi. Australian Journal of Botany 72, BT23086.
| Crossref | Google Scholar |
Metz MR, Varner JM, Frangioso KM, Meentemeyer RK, Rizzo DM (2013) Unexpected redwood mortality from synergies between wildfire and an emerging infectious disease. Ecology 94(10), 2152-2159.
| Crossref | Google Scholar | PubMed |
Moore N, Barrett S, Howard K, Craig MD, Bowen B, Shearer B, Hardy G (2014) Time since fire and average fire interval are the best predictors of Phytophthora cinnamomi activity in heathlands of south-western Australia. Australian Journal of Botany 62, 587-593.
| Crossref | Google Scholar |
Murphy B, Vaze J, Teng J, Tuteja NK, Gallant J, Summerell G, Young J, Wild J (2005) Modelling landscapes using terrain analysis to delineate landforms and predict soil depths - examples from catchments in NSW. In ‘Proceedings of the MODSIM International Congress on modelling and simulation’. pp. 1423–1429. (Modelling and Simulation Society of Australia and New Zealand: Canberra)
Nakova M (2010) Monitoring of Phytophthora species on fruit trees in Bulgaria. European Journal of Plant Pathology 128, 517-525.
| Crossref | Google Scholar |
Newby Z, Murphy RJ, Guest DI, Ramp D, Liew ECY (2019) Detecting symptoms of Phytophthora cinnamomi infection in Australian native vegetation using reflectance spectrometry: complex effects of water stress and species susceptibility. Australasian Plant Pathology 48, 409-424.
| Crossref | Google Scholar |
Newell GR (1998) Characterization of vegetation in an Australian open forest community affected by cinnamon fungus (shape Phytophthora cinnamomi): implications for faunal habitat quality. Plant Ecology 137, 55-70.
| Crossref | Google Scholar |
Newhook FJ, Podger FD (1972) The role of Phytophthora cinnamomi in Australian and New Zealand forests. Annual Review of Phytopathology 10, 299-326.
| Crossref | Google Scholar |
Noss RF (1990) Indicators for monitoring biodiversity: a hierarchical approach. Conservation Biology 4(4), 355-364.
| Crossref | Google Scholar |
Oberpriller J, de Souza Leite M, Pichler M (2022) Fixed or random? On the reliability of mixed-effects models for a small number of levels in grouping variables. Ecology and Evolution 12(7), e9062.
| Crossref | Google Scholar | PubMed |
O’Brien PA, Williams N, Hardy GESJ (2009) Detecting Phytophthora. Critical Reviews in Microbiology 35(3), 169-181.
| Crossref | Google Scholar | PubMed |
Petit S, Dickson CR (2005) Grass-tree (Xanthorrhoea semiplana, Liliaceae) facilitation of the endangered pink-lipped spider orchid (Caladenia syn. Arachnorchis behrii, Orchidaceae) varies in South Australia. Australian Journal of Botany 53, 455-464.
| Crossref | Google Scholar |
Podger FD (1972) Phytophthora cinnamomi, a cause of lethal disease in indigenous plant communities in Western Australia. Phytopathology 62, 972-981.
| Crossref | Google Scholar |
Pratt BH, Heather WA (1972) Method for rapid differentiation of Phytophthora cinnamomi from other Phytophthora species isolated from soil by lupin baiting. Transactions of the British Mycological Society 59(1), 87-96.
| Crossref | Google Scholar |
Pryce J, Edwards W, Gadek PA (2002) Distribution of Phytophthora cinnamomi at different spatial scales: when can a negative result be considered positively? Austral Ecology 27, 459-462.
| Crossref | Google Scholar |
Pyšek P, Hulme PE (2005) Spatio-temporal dynamics of plant invasions: linking pattern to process. Écoscience 12(3), 302-315.
| Crossref | Google Scholar |
R Core Team (2021) ‘R: a language and environment for statistical computing, 4.1.1.’ (R Foundation for Statistical Computing: Vienna, Austria) Available at https://www.R-project.org/
Regan HM, Keith DA, Regan TJ, Tozer MG, Tootell N (2011) Fire management to combat disease: turning interactions between threats into conservation management. Oecologia 167, 873-882.
| Crossref | Google Scholar | PubMed |
Robin X, Turck N, Hainard A, Tiberti N, Lisacek F, Sanchez J-C, Müller M (2011) pROC: an open-source package for R and S+ to analyze and compare ROC curves. BMC Bioinformatics 12, 77.
| Crossref | Google Scholar | PubMed |
Samuel G (2020) Independent review of the EPBC Act - Final Report. Department of Agriculture, Water and the Environment, Canberra. Available at https://epbcactreview.environment.gov.au
Schena L, Duncan JM, Cooke DEL (2008) Development and application of a PCR-based ‘molecular tool box’ for the identification of Phytophthora species damaging forests and natural ecosystems. Plant Pathology 57, 64-75.
| Crossref | Google Scholar |
Sena K, Dreaden TJ, Crocker E, Barton C (2018a) Detection of Phytophthora cinnamomi in forest soils by PCR on DNA extracted from leaf disc baits. Plant Health Progress 19, 193-200.
| Crossref | Google Scholar |
Sena K, Crocker E, Vincelli P, Barton C (2018b) Phytophthora cinnamomi as a driver of forest change: implications for conservation and management. Forest Ecology and Management 409, 799-807.
| Crossref | Google Scholar |
Shearer BL, Crane CE, Cochrane A (2004a) Quantification of the susceptibility of the native flora of the South-West Botanical Province, Western Australia, to Phytophthora cinnamomi. Australian Journal of Botany 52, 435-443.
| Crossref | Google Scholar |
Shearer BL, Crane CE, Fairman RG (2004b) Phosphite reduces disease extension of a Phytophthora cinnamomi front in Banksia woodland, even after fire. Australasian Plant Pathology 33, 249-254.
| Crossref | Google Scholar |
Shearer BL, Crane CE, Cochrane JA, Dunne CP (2013) Variation in susceptibility of threatened flora to Phytophthora cinnamomi. Australasian Plant Pathology 42, 491-502.
| Crossref | Google Scholar |
Suddaby T, Liew E (2008) Best practice management guidelines for Phytophthora cinnamomi within the Sydney Metropolitan Catchment Management Authority area. Botanic Gardens Trust, BG Trust, Sydney. Available at https://catalogue.nla.gov.au/Record/4555713
Summerell BA, Liew E (2020) Phytophthora root rot: its impact in botanic gardens and on threatened species conservation. Sibbaldia: The International Journal of Botanic Garden Horticulture 18, 89-104.
| Crossref | Google Scholar |
Thompson SE, Levin S, Rodriguez-Iturbe I (2014) Rainfall and temperatures changes have confounding impacts on Phytophthora cinnamomi occurrence risk in the southwestern USA under climate change scenarios. Global Change Biology 20, 1299-1312.
| Crossref | Google Scholar | PubMed |
Tozer MG, Turner K, Keith DA, Tindall D, Pennay C, Simpson C, MacKenzie B, Beukers P, Cox S (2010) Native vegetation of southeast NSW: a revised classification and map for the coast and eastern tablelands. Cunninghamia 11(3), 359-406.
| Google Scholar |
von Broembsen S (1984) Distribution of Phytophthora cinnamomi in rivers of the South-Western Cape Province. Phytophylactica 16, 227-229.
| Google Scholar |
Walsh JL, Keith DA, McDougall KL, Summerell BA, Whelan RJ (2006) Phytophthora Root Rot: assessing the potential threat to Australia’s oldest national park. Ecological Management & Restoration 7(1), 55-60.
| Crossref | Google Scholar |
Wan JSH, McDougall KL, Liew ECY (2019) The susceptibility of rare and threatened NSW species to the root-rot pathogen Phytophthora cinnamomi: 1. Initial testing and identification of key research questions. Australian Journal of Botany 67, 510-516.
| Crossref | Google Scholar |
Weste G (2003) The dieback cycle in Victorian forests: a 30-year study of changes caused by Phytophthora cinnamomi in Victorian open forests, woodlands and heathlands. Australasian Plant Pathology 32, 247-256.
| Crossref | Google Scholar |
Weste G, Marks GC (1987) The biology of Phytophthora cinnamomi in Australasian forests. Annual Review of Phytopathology 25, 207-229.
| Crossref | Google Scholar |
Weste G, Brown K, Kennedy J, Walshe T (2002) Phytophthora cinnamomi infestation - a 24-year study of vegetation change in forests and woodlands of the Grampians, Western Victoria. Australian Journal of Botany 50, 247-274.
| Crossref | Google Scholar |
Wilson BA, Aberton J, Cahill DM (2000) Relationships between site factors and distribution of Phytophthora cinnamomi in the Eastern Otway Ranges, Victoria. Australian Journal of Botany 48, 247-260.
| Crossref | Google Scholar |
Wilson BA, Annett K, Laidlaw WS, Cahill DM, Garkaklis MJ, Zhuang-Griffin L (2020) Long term impacts of Phytophthora cinnamomi infestation on heathy woodland in the Great Otway National Park in south-eastern Australia. Australian Journal of Botany 68, 542-556.
| Crossref | Google Scholar |
Wilson BA, Casey SP, Garkaklis MJ, Learmonth C, Wevill T (2024) Impact of Phytophthora dieback on a key heathland species Xanthorrhoea australis (Asphodelaceae) (austral grasstree) and floristic composition in the eastern Otways, Victoria. Australian Journal of Botany 72, BT23076.
| Crossref | Google Scholar |