Determining the moisture content of Acacia seeds
Geoffrey E. Burrows
A
Abstract
In a recent paper in Southern Forests, Jaganathan and co-workers indicated that the low constant-temperature drying method (LCTDM) (103°C for 17 h) is satisfactory for determining moisture content (MC) in seeds with physical dormancy (PY). Would this recommendation apply to Acacia, a large genus (1000+ species) with a seed coat so strong that seeds in the soil can maintain high viability for many decades? MC was assessed, using the LCTDM, in six Australian Acacia species by comparing intact seeds with those cut in half. Halved seeds of all species lost moisture rapidly and their mass had largely stabilised after about 2–3 h. Intact seeds had a wide variation in moisture-loss patterns. In some species, the testa was morphologically unchanged after 24 h at 103°C and this was associated with a relatively slow moisture loss. In other species, the testa had completely shattered after 8 h, leading to a rapid moisture loss. If the LCTDM is used to determine MC in Acacia species, it is advisable to employ a technique that substantially disrupts the testa.
Keywords: Acacia, lens, macrosclereid, moisture content, physical dormancy, seed coat, testa, viability.
Introduction
Moisture content (MC) is an important property of seeds (Hay et al. 2023), associated with many aspects of physiological seed quality (Grabe 1989). Consequently, techniques for determining seed MC are of considerable interest and the International Seed Testing Association (ISTA) in its publication ‘International Rules for Seed Testing 2023’ devoted Chapter 9 to ‘Determination of moisture content’. Their recommended oven-based methods for determining MC are (1) the high-temperature drying method (130°C for 1, 2, 3 or 4 h) or (2) the low constant-temperature drying method (LCTDM) (103°C for 17 h).
An interesting factor in determining MC occurs when seeds have physical dormancy (PY). This dormancy is related to a single layer of palisade sclereid cells in the testa that prevents moisture from entering the seed. In terms of MC determination, this same layer may prevent moisture from exiting the seed when being dried in an oven. The ISTA make various recommendations for allowing moisture to exit PY seeds, mainly related to cutting or grinding.
Recently, Jaganathan et al. (2022, p. 325) suggested ‘that the low constant temperature drying method can be used for species with impermeable seed coats’, without mentioning any need for seed coat disruption. They based this recommendation on trials on three tree species, namely Adenathera pavonina (Caesalpinioideae, ~250 mg/seed), Delonix regia (Faboideae, ~400 mg/seed) and Sesbania grandiflora (Caesalpinioideae, 30–60 mg/seed). Although they did not provide seed weights, information from the literature would indicate that the first two species have large seeds (1000-seed weight of >200 g, ISTA; i.e. >200 mg/seed).
Burrows et al. (2018) studied testa and lens structure in approximately 50 Australian Acacia (Mimosoideae) species, finding that almost all species had PY and a consistent testa structure (almost all species had mesophyll, palisade and cuticle layers, but with a wide range of thicknesses, plus a functional lens). Given our findings on Acacia seed coat structure, we wondered whether the recommendation of Jaganathan et al. (2022) to use the LCTDM, with no grinding or cutting, would be suitable for MC determination with Australian Acacia seeds. Acacia seed coats are so robust that seeds can remain viable in the soil for decades (i.e. the seed coat is very effective at reducing water loss from the embryo) (Cavanagh 1980). Would an Acacia seed coat also limit moisture loss at 103°C in an oven?
Materials and methods
The species studied were selected from the 51 Acacia species that had been the focus of two previous studies on lens and testa structure (Burrows et al. 2018, 2019) and seeds from the same seed lots were used. Seeds had been stored at 20°C in closed containers. Experiments were conducted during 2023 and 2024.
The species examined were A. adoxa, A. genistifolia, A. gracillima, A. granitica, A. leptoloba and A. pulchella. These species had a median collection date of 2007 (Table 1). Six replicates of 10 seeds were prepared for each species. Three replicates were controls and in three replicates each seed was cut in mid-transverse section (i.e. the cotyledons were cut in TS) with a scalpel blade immediately before the initial weighing and then transferred to the oven. The seeds were dried in an oven at 103°C for differing times. Mass was determined using a balance accurate to four decimal places. In seeds of some species, the testa began to fracture substantially or split after several hours of drying (Fig. 1f, h). The percentage of seeds with a split or fractured testa after each weighing was determined using a stereo microscope.
Species | Seed collection date | Seed mass (mg) | Total testa | Mesophyll | Palisade | Cuticle | |
---|---|---|---|---|---|---|---|
A. adoxa | 2011 | 14.9 ± 0.7 | 104 ± 13 | 75 ± 11 | 23 ± 4 | 6 ± 1 | |
A. pulchella | 2006 | 7.5 ± 0.3 | 85 ± 9 | 43 ± 6 | 29 ± 4 | 13 ± 3 | |
A. granitica | 1989 | 8.1 ± 0.3 | 139 ± 25 | 75 ± 20 | 48 ± 4 | 16 ± 4 | |
A. genistifolia | 2010 | 20.4 ± 1.2 | 158 ± 15 | 85 ± 12 | 47 ± 6 | 26 ± 5 | |
A. gracillima | 2008 | 12.0 ± 0.9 | 155 ± 10 | 108 ± 11 | 43 ± 3 | 4 ± 1 | |
A. leptoloba | 2005 | 25.9 ± 0.6 | 166 ± 14 | 84 ± 7 | 50 ± 4 | 32 ± 4 |
Seed morphology of four Acacia species. (a, b) A. pulchella; (c, d) A. adoxa; (e, f) A. leptoloba, (g, h) A. gracillima. Control seeds (a, c, e, g) and seeds after 24 h of dry heat (103°C) (b, d, f, h). Note that in A. pulchella and A. adoxa, the heat treatment had little or no effect on morphology, whereas in A. leptoloba and A. gracillima, in most seeds the testa had shattered, exposing the pale yellow embryo. Note also the numerous fine cracks in the seed coat in (f).
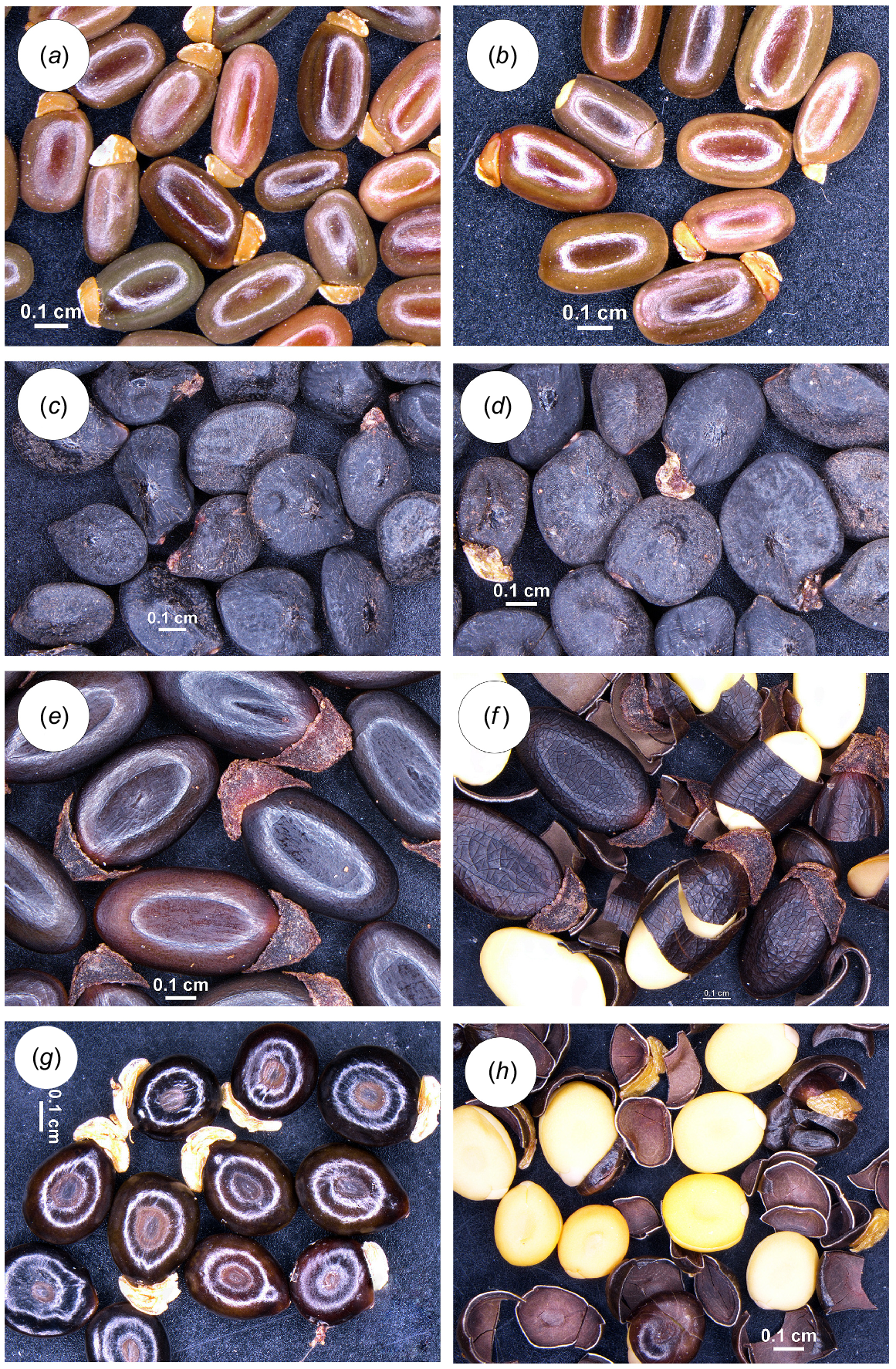
In A. adoxa and A. pulchella, the intact seeds lost moisture relatively slowly during the first 8–24 h. After 24 h, the seeds of these species were cut in TS. They were then re-weighed, to make sure no material had been lost during the cutting, before being placed back in the oven.
To assess whether the lens ‘popped’ in response to 103°C, seeds of each species were examined under a dissecting microscope to ensure that the lens had not pre-popped (Burrows et al. 2018). Ten seeds of each species were placed at 103°C for 1 min, before being re-examined to assess whether the lens had popped.
Because viable seeds might have a greater MC than non-viable seeds, viability was assessed by the triphenyl tetrazolium chloride test. Seeds were cut in longitudinal section before being soaked in the liquid (3 replicates of 10 seeds per species).
MC was calculated on a fresh weight basis (MC = (starting sample fresh weight – dry weight) / starting sample fresh weight × 100).
Results
The seeds of the six species had widely varying morphology and colour, from oblong to almost circular, from glossy to matte and from light brown to black (Fig. 1). Average seed (plus aril, where present) mass varied from 8 to 26 mg/seed (Table 1). Burrows et al. (2018, table 3) gave overall averages for many aspects of seed structure of over 50 Acacia species, but no data for individual species. Table 1 gives the average testa thickness and average cuticle, palisade and mesophyll thicknesses for the six species examined in this study, from the raw data from Burrows et al. (2018).
For all six species, when the seeds were halved they lost much of their MC after 2–3 h at 103°C (Fig. 2, Table 2). In contrast, the intact seeds showed different patterns of moisture loss. In A. leptoloba and A. gracillima, the intact seeds lost MC relatively rapidly (Fig. 2, Table 2). Morphological examination showed that A. leptoloba seeds quickly developed an extensive network of surface cracks after 1 h. This species has a thick cuticle (average 32 μm, Table 1) and thus the cracks may have been only in the wax, not the palisade cells. However, after 8 h all the testas had completely fractured, exposing the creamy-coloured embryo (Fig. 1f). At this stage, and probably at some time before, these seeds were probably losing moisture over most of their surface area. In contrast, A. gracillima had a very thin cuticle (average 4 μm, Table 1). The seeds did not develop a fine network of cracks, but the testa started cracking between 4 and 6 h along a few major fracture lines and all seeds had split after 24 h (Fig. 1h). At the other end of the moisture-loss spectrum, A. adoxa and A. pulchella had very different seed morphologies (Fig. 1a–d), but the seeds appeared unchanged after 24 h at 103°C (Fig. 1b, d). The seeds of both species lost moisture at a slow, but relatively constant rate over the first 24 h (Fig. 2). When these seeds were halved after 24 h, they quickly lost MC and were close to the final MC 1 h later (Fig. 2, Table 2). A. genistifolia and A. granitica were intermediate in their moisture-loss patterns (27–37% of seeds had split after 24 h).
Average apparent seed moisture content (%) (error bars ± s.e. of the mean), on a fresh weight basis, for Acacia adoxa and A. gracillima over 48 h. Control (whole/intact) seeds and seeds halved. The control seeds of A. adoxa were cut in half after 24 h.
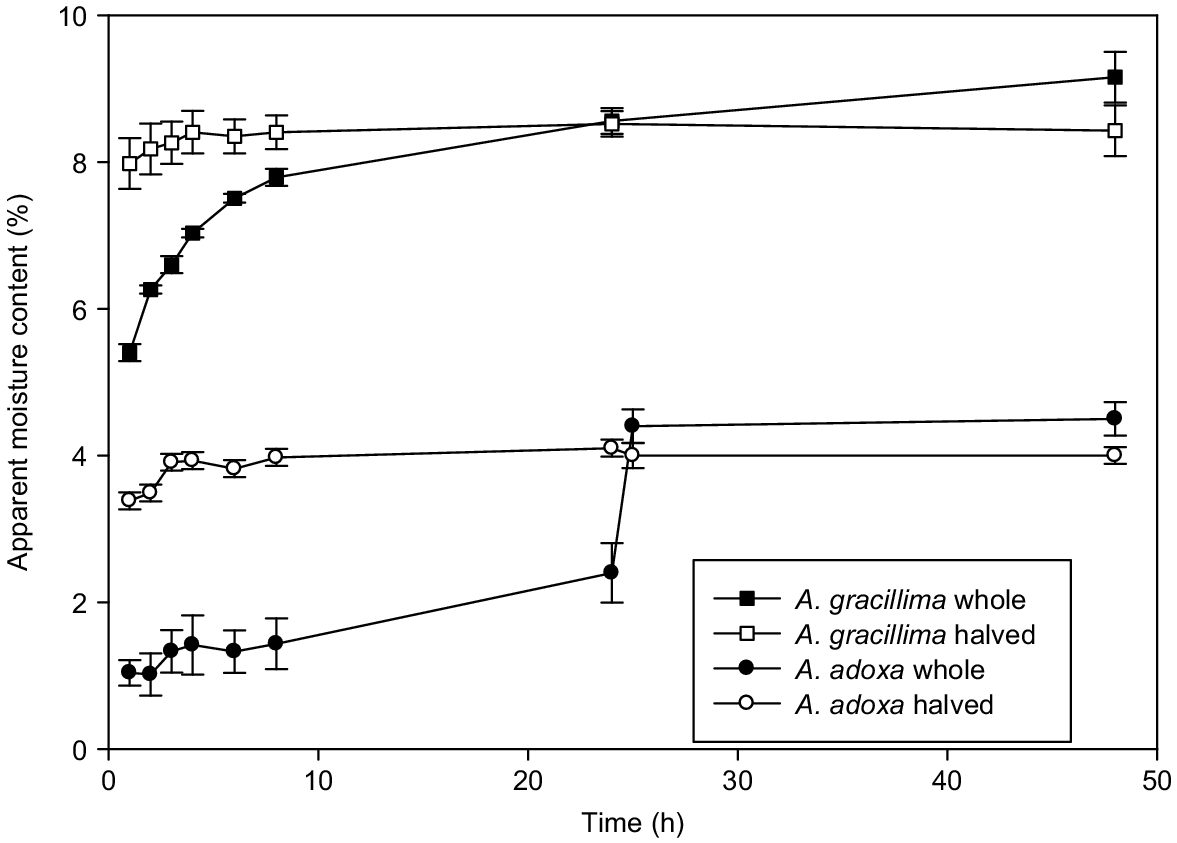
Item | 1 h | 2 h | 3 h | 4 h | 6 h | 8 h | 24 h | 25 h | 48 h | |
---|---|---|---|---|---|---|---|---|---|---|
A. adoxa | ||||||||||
Control | 1.0 ± 0.3 | 1.0 ± 0.5 | 1.3 ± 0.5 | 1.4 ± 0.7 | 1.3 ± 0.5 | 1.4 ± 0.6 | 2.4 ± 0.7 | 4.4 ± 0.4 | 4.5 ± 0.4 | |
Halved | 3.4 ± 0.2 | 3.5 ± 0.2 | 3.9 ± 0.2 | 3.9 ± 0.2 | 3.8 ± 0.2 | 4.0 ± 0.2 | 4.1 ± 0.2 | 4.0 ± 0.3 | 4.0 ± 0.2 | |
A. pulchella | ||||||||||
Control | 2.0 ± 0.7 | 2.4 ± 1.1 | 2.4 ± 0.8 | 2.7 ± 0.9 | 2.9 ± 0.8 | 2.8 ± 0.9 | 3.2 ± 1.2 | 6.1 ± 1.4 | 6.2 ± 1.7 | |
Halved | 4.2 ± 2.2 | 5.8 ± 1.2 | 5.6 ± 1.0 | 5.9 ± 0.8 | 6.1 ± 0.9 | 6.0 ± 0.8 | 6.0 ± 0.7 | 6.0 ± 0.8 | 5.8 ± 0.8 | |
A. genistifolia | ||||||||||
Control | 2.5 ± 0.3 | 2.7 ± 0.3 | 2.9 ± 0.4 | 2.9 ± 0.4 | 3.0 ± 0.4 | 3.2 ± 0.5 | 5.0 ± 0.7 | 6.2 ± 0.6 | ||
Halved | 5.7 ± 0.8 | 6.5 ± 0.4 | 6.7 ± 0.5 | 6.6 ± 0.3 | 7.0 ± 0.3 | 7.0 ± 0.4 | 7.3 ± 0.3 | 7.3 ± 0.3 | ||
A. granitica | ||||||||||
Control | 3.1 ± 0.2 | 3.5 ± 0.3 | 3.8 ± 0.3 | 4.1 ± 0.4 | 4.1 ± 0.6 | 4.3 ± 0.7 | 5.6 ± 0.7 | 8.6 ± 0.5 | ||
Halved | 7.4 ± 1.5 | 7.7 ± 1.5 | 7.8 ± 1.5 | 7.9 ± 1.6 | 7.9 ± 1.6 | 7.8 ± 1.5 | 7.8 ± 1.7 | 8.0 ± 1.7 | ||
A. leptoloba | ||||||||||
Control | 5.2 ± 0.4 | 6.3 ± 0.4 | 6.7 ± 0.5 | 7.0 ± 0.7 | 7.7 ± 0.7 | 7.7 ± 0.7 | 8.5 ± 0.7 | 8.6 ± 0.6 | ||
Halved | 6.7 ± 0.1 | 7.1 ± 0.1 | 7.5 ± 0.1 | 7.4 ± 0.1 | 7.6 ± 0.1 | 7.6 ± 0.1 | 8.1 ± 0.3 | 8.2 ± 0.3 | ||
A. gracillima | ||||||||||
Control | 5.4 ± 0.2 | 6.3 ± 0.0 | 6.6 ± 0.2 | 7.0 ± 0.1 | 7.5 ± 0.1 | 7.8 ± 0.2 | 8.6 ± 0.3 | 9.2 ± 0.6 | ||
Halved | 8.0 ± 0.6 | 8.2 ± 0.6 | 8.3 ± 0.5 | 8.4 ± 0.4 | 8.4 ± 0.4 | 8.4 ± 0.4 | 8.5 ± 0.3 | 8.4 ± 0.6 |
Species are arranged in patterns of relative moisture loss from control (intact) seeds (A. adoxa and A. pulchella – slow, A. genistifolia and A. granitica – intermediate, A. leptoloba and A. gracillima – rapid).
Average seed viability was greater than 75% in all species, except A. leptoloba (average 70%). Even where seeds were indicated to be non-viable, the embryos were plump and a healthy cream colour. In all species, over 90% of the lenses had popped after 1 min at 103°C.
Discussion
Jaganathan et al. (2022, p. 325) stated that ‘it is suggested that the low constant temperature drying method can be used for species with impermeable seed coats’. The present study showed that the LCTDM would not be suitable for intact seeds of a range of Acacia species. The work of Taylor (1987, see below) showed that the LCTDM was also not suitable for some small-seeded pasture legumes. The International Rules for Seed Testing (2023) in table 9A, part 2 (‘Details of methods for moisture determination: tree and shrub seeds’) listed approximately 70 genera, each with various comments. Ten of the genera were from the Fabaceae and all these genera were indicated to need coarse grinding. This indicates that the LCTDM, without some substantial disruption of the testa, should not be recommended as a general technique for Fabaceae species with PY. Table 9A part 2 is mainly concerned with species of commercial interest. Many seed-biology studies of Acacia have involved numerous species and small seed volumes. In these cases, seed cutting would be more convenient and accurate than grinding seeds. Ground material, with its large surface area, introduces problems such as rapid uptake of moisture from the atmosphere, decomposition from heat and the loss of material during handling (Taylor 1987; Grabe 1989).
Jaganathan et al. (2022, p. 326) also noted that ‘either the specified water-gaps, e.g., the lens, are opened, or their seed coats crack open in seconds’. In Acacia, the lenses open quickly, but this is a very small (70–80 μm internal diameter for A. melanoxylon; Burrows et al. 2009) opening for moisture to exit from the seed. In A. adoxa and A. pulchella, the lenses popped within 60 s of being placed at 103°C, but the lens is clearly not a major area for moisture to exit the seed. In A. leptoloba and A. gracillima, the seed coats did not show major cracking for several hours, although disruption of the palisade layer probably occurred sooner.
Taylor (1987) assessed the MC of seeds of three small-seeded (3–6 mg) pasture legumes (Medicago truncatula, M. polymorpha, Trifolium subterraneum). He compared naturally permeable and impermeable seeds, halved and intact seeds and time at 130°C (up to 48 h) or 105°C (up to 18 days). Like the present study, Taylor found distinct differences among the three species in their drying curves (Taylor 1987, fig. 1). In two species, the drying curves of the intact and halved impermeable seeds at 105°C were similar, whereas for Medicago polymorpha the halved seeds dried quickly, and the intact seeds needed ~15 days to reach the same MC (~4%) as the halved seeds. This is similar to the present study and shows that even small seeds can need some form of seed coat disruption to allow moisture to escape. Taylor’s (1987) overall recommendation was to dry halved seeds for 24 h at 105°C.
It is interesting to consider why the seed coats of some Acacia species were seemingly unaffected, on the basis of external morphology, by 24 h at 103°C (Fig. 1b, d), whereas in others the testa completely shattered (thus exposing the embryo) (Fig. 1f, h). Burrows et al. (2018) showed that for 49 of the 51 Australian Acacia species examined (the hard-seeded species, not the few soft-seeded species), the testa had a similar basic construction, consisting of a cuticle (15 μm average thickness, ×13 range), palisade layer (41 μm average thickness, ×7 range) and mesophyll (78 μm thickness, ×10 range). Acacia adoxa and A. pulchella had a total average testa thickness of 94–103 μm and an average palisade thickness of 23–29 μm. The other four species had averages of 146–170 μm and 43–50 μm, respectively. Thus, there appears to be a correlation between a thinner testa and palisade and resistance to testa splitting at 103°C, although the sample size was very small.
In addition, Burrows et al. (2018, fig. 8) found that the cuticle of the seeds of the 51 Acacia species reacted very differently to a 60 s exposure to very hot water. Burrows et al. (2019) found that when germinating Acacia seeds with popped lenses, there were large differences in the time taken for seeds to imbibe, both within and between species. The present study, combined with these previous studies, has indicated that although the Acacia testa has a basic uniformity of structure and function (e.g. is very effective for PY), there are some major differences among the species.
When assessing what species are present in the soil seed bank, soil samples are usually heated to mimic a fire and break PY. For example, in two Australian studies, after air-drying the soil was passed through a 4–8 mm sieve, spread to a depth of 2 cm on trays before being placed in an oven at 90°C for 1 h or 95°C for 2 h, and then placed in conditions suitable for germination (Singh et al. 2023, Duivenvoorden et al. 2024). The present study showed that the seeds of certain Acacia species will lose more moisture during these extended heat treatments; however, whether this affects viability needs to be tested.
Across the six species, MC varied from 4.3% (A. adoxa) to 8.8% (A. gracillima). Seeds of these two species were collected in 2011 and 2008, respectively. Both species had high viability percentages (>85%). Similarly, Burrows et al. (2019) found that the MC of the seeds of 11 Acacia species varied from 5.7% to 11.1% (MC assessed in halved seeds, dried at 100°C for 48 h; median collection date 2010). These species had an average of ~90% germination at the optimal tested temperature (Burrows et al. 2019, fig. 8d). These results showed that Acacia species can maintain high viability, at a wide range of MC, over long periods.
In summary, to determine the MC of Acacia seeds, the LCTDM can be effective when combined with testa disruption. If assessing large numbers of species and/or large seed numbers, it could be useful to place a small sample of seeds at 103°C for 24 h. If the testa in all the seeds cracks or fractures, exposing the embryo, then cutting the seeds of those species could be omitted.
References
Burrows GE, Virgona JM, Heady RD (2009) Effect of boiling water, seed coat structure and provenance on the germination of Acacia melanoxylon seeds. Australian Journal of Botany 57(2), 139-147.
| Crossref | Google Scholar |
Burrows GE, Alden R, Robinson WA (2018) The lens in focus – lens structure in seeds of 51 Australian Acacia species and its implications for imbibition and germination. Australian Journal of Botany 66, 398-413.
| Crossref | Google Scholar |
Burrows GE, Alden R, Robinson WA (2019) Markedly different patterns of imbibition in seeds of 48 Acacia species. Seed Science Research 29(4), 270-282.
| Crossref | Google Scholar |
Cavanagh AK (1980) A review of some aspects of the germination of acacias. Proceedings of the Royal Society of Victoria 91, 161-180.
| Google Scholar |
Duivenvoorden E, Wagner B, Nitschke CR, Kasel S (2024) Short-interval, high-severity wildfires cause declines in soil seed bank diversity in montane forests of south-eastern Australia. Forest Ecology and Management 553, 121627.
| Crossref | Google Scholar |
Hay FR, Rezaei S, Wolkis D, McGill C (2023) Determination and control of seed moisture. Seed Science and Technology 51(2), 267-285.
| Crossref | Google Scholar |
Jaganathan GK, Li J, Liu B (2022) Low constant temperature drying can be a satisfactory method to determine the moisture content of impermeable seed coated species. Southern Forests 84, 325-327.
| Crossref | Google Scholar |
Singh A, Nitschke CR, Hui FKC, Baker P, Kasel S (2023) Soil seed banks provide a storage effect in post-logging regrowth forests of southeastern Australia. Forest Ecology and Management 548, 121389.
| Crossref | Google Scholar |
Taylor GB (1987) Determination of seed moisture content in small-seeded pasture legumes. Australian Journal of Experimental Agriculture 27, 377-380.
| Crossref | Google Scholar |