Variable seed quality hampers the use of Themeda triandra (Poaceae) for seed production, agriculture, research and restoration: a review
Marne Durnin
A
B
C
D
E
Abstract
Themeda triandra (Forssk.) is a tussock grass of international importance for its keystone role in grassy ecosystems and, hence, is often a focus for seed production, research and ecological restoration. However, these applications can be challenged by its seed biology, including seed dormancy and the variability of seed traits among populations. The literature on these topics has not been well synthesised. To address this, we reviewed the relevant literature for Themeda triandra seed and found that seed quality is often poor but highly variable, and there are no current quality-assurance standards. Seed characteristics such as weight, awn length and desiccation tolerance can vary with seed ploidy (i.e. the number of chromosome sets). Germination rates of fresh Themeda triandra seed can be as low as 5%, which may be due to seed dormancy. Dormancy varies among populations and with seasonal conditions, and a period of dry storage at room temperature for 8–10 months may be needed before it will reach its full germination potential. No single treatment is effective in overcoming dormancy across all populations. The application of gibberellic acid can increase germination by ~30% in some populations. Although plant-derived smoke products can be effective, they also lack standardisation. Other treatments including the application of heat, or wet–dry cycling warrant further investigation. We recommend the standardisation of Themeda triandra seed testing and labelling, which would allow end users to assess value for money when purchasing seed commercially.
Keywords: germination protocols, grasses, indigenous species, plant biology, seed ecology, seed germination, Themeda triandra.
Introduction
Themeda triandra Forssk. is a warm-season C4 tussock grass that is widely distributed through Africa, Asia and Australia (Fig. 1). Known as kangaroo grass in Australia and red grass in Africa, it has been affected by a range of degrading factors including over-grazing, urbanisation and land clearing for agriculture. The current distribution of Themeda triandra (hereafter referred to as Themeda) is therefore significantly reduced compared with its pre-colonial distribution (Moore 1973; Hyde 1995; Prober and Thiele 1995; Snyman et al. 2013). Given its importance for grassy-ecosystem restoration (Cole and Lunt 2005; Prober et al. 2005; Cole et al. 2017), mine-site remediation (Farley et al. 2013), Indigenous cultural practices (Drake et al. 2021) and agriculture (Clarke et al. 2010; Snyman et al. 2013; Male et al. 2022), there is considerable interest in its reintroduction. However, in many ecosystems, the Themeda soil seed bank may be limited (Everson and Clarke 1987; Morgan 1998; Lunt 1990), especially with regular grazing (O’Connor and Pickett 1992) and recolonisation from extant plants may be slow (Snyman et al. 2013).
Re-establishment of Themeda from seed is possible but success is closely related to seed quality (i.e. seed purity, fill and viability). Like for many grasses (Simpson 2010), Themeda seed quality can be highly variable and often poor (Tainton and Booysen 1963; Baxter et al. 1993; Mason 2005; Clarke et al. 2010; Farley et al. 2013). The volume of Themeda seed harvested is often low, owing to asynchronous seed ripening, seed shattering, the production of sterile seed and insect attack (Woodland 1964; McDougall 1989; Sindel et al. 1993).
As we describe below, seed quality can also be affected by harvesting methods, which may lead to low seed purity owing to high levels of chaff or weed seed (Mason 2005; Male et al. 2022). Seed fill can be variable because of immature, sterile or aborted seeds (Tainton and Booysen 1963; Farley et al. 2013). Further, seed dormancy can be high at seed fall, and seeds from many populations need a period of ‘after-ripening’ before they will germinate (Groves et al. 1982; Baxter 1996; Mason 2005; Saleem et al. 2009). Given the difficulties of producing good-quality seed, it is often in low supply in Australia and prices are high in the commercial sector, ranging from ~A$900 to A$3800 kg−1 (Native Seeds Pty. 2024; Nindethana Australian Seeds 2024). High costs are a barrier to using seed (Reseigh et al. 2008), and make research, restoration, seed production and restoration using native grasses such as Themeda challenging (Sindel et al. 1993; Mason 2005; Clarke et al. 2010).
Native-seed quality assurance should mean that end-users are given quantitative information about seed purity, seed fill, seed viability and, where applicable, dormancy status (Pedrini and Dixon 2020); however, poor seed quality is common globally (Pedrini and Dixon 2020). It can have significant financial impacts for end-users, particularly restoration practitioners (Vogel 2002; Frischie et al. 2020; Pedrini and Dixon 2020), and those working in research and development (Clarke et al. 2010). For commercially significant plants, there are international standards and protocols for seed-quality testing through the International Seed Testing Association (ISTA 2023), but these are often not appropriate for wild seeds (Pedrini and Dixon 2020; Martyn Yenson et al. 2021). International principles and quality standards for wild native seeds used in restoration have recently been proposed. These would include proforma seed-quality labels covering all aspects of seed quality and viability, aimed at providing transparency and allowing purchasers to compare value for money (Pedrini and Dixon 2020; Van Moort et al. 2021).
Given its wide international distribution and importance in many grassy ecosystems, Themeda has been the subject of considerable international research over more than 70 years (Snyman et al. 2013). However, there are still many inconsistencies in the methods used to test seed-quality parameters, which need to be resolved before quality labelling for this species is possible.
Here, we review the Themeda seed-biology and seed-quality literature to provide a knowledge bank for restoration researchers and practitioners and a starting point for eventual seed-quality standards. Our overarching aim was to assist with ecosystem restoration and to underpin the development of seed-quality protocols by synthesising current knowledge and identifying research gaps. Reducing the inconsistencies in Themeda seed-quality testing and fostering the development of an international germination protocol will allow seed testing and research to be improved and become relevant at the scale required for agriculture and restoration. We started with the review by Snyman et al. (2013) and the paper by Groves et al. (1982), and followed up on the key references. Additional keyword searches using Google Scholar and Web of Science included the terms ‘Themeda triandra’ and ‘seed’, ‘seed quality’ ‘ploidy’ and ‘Australian native seed quality’. The review concludes with knowledge gaps and suggested directions for future research.
Themeda seed characteristics
Seed morphology
The Themeda dispersal unit is a ‘caryopsis enclosed by a membranous lower lemma’ (Baxter 1996, p. 24). The upper lemma extends to form the geniculate awn and the lemmas are enclosed by ‘two outer glumes; a small, tough upper glume, overlapped by a strong lower glume’ (Baxter 1996, p. 24). Viable seed is usually dark olive or dark chocolate brown in colour at maturity (McDougall 1989; Mason 2005). Hereafter, this dispersal unit is referred to as ‘seed’.
Seed purity
Pure seed does not contain any contaminants, for example, chaff, stones or weed seed (Martyn Yenson et al. 2021). It can be assessed by weighing a sample and then removing anything that is not a Themeda diaspore (Martyn Yenson et al. 2021). The sample is then weighed again to determine the percentage of the sample that is pure seed.
Themeda seed can be harvested by slashing or bailing or with a vacuum or brush harvester. Batches often include chaff, blind tillers, aborted or sterile seed and seeds of other plant species (Cole and Metcalfe 2003; Mason 2005; Male et al. 2022). Brush harvesters tend to result in higher seed purity, with one study reporting approximately 450,000 viable seeds per 15 kg wool pack compared with 15,000 viable seeds harvested through slashing and bailing (Mason 2005). Seed that includes the whole or other parts of the inflorescence is less expensive than pure seed (Nindethana Australian Seeds 2024), but contains lower proportions of pure and viable seeds (Mason 2005).
Seed fill
A filled seed is firm and contains an endosperm and embryo (Martyn Yenson et al. 2021). Themeda seed fill is critically important to seed quality and value for money, but can be difficult to assess visually because seeds that look ripe may be empty (Fig. 2). Seed fill is rarely quantified, but may be as low as ~35–50% (Tainton and Booysen 1963; Farley et al. 2013) for some populations. Reasons for this include embryo failure (Woodland 1964), insect damage (Woodland 1964; McDougall 1989) or seed abortion from stresses such as heat or drought (Farley et al. 2013).
Three tetraploid Themeda triandra seeds. The seed on the left is ripe and filled. The two seeds on the right are empty (photo: Ellen Bennett).
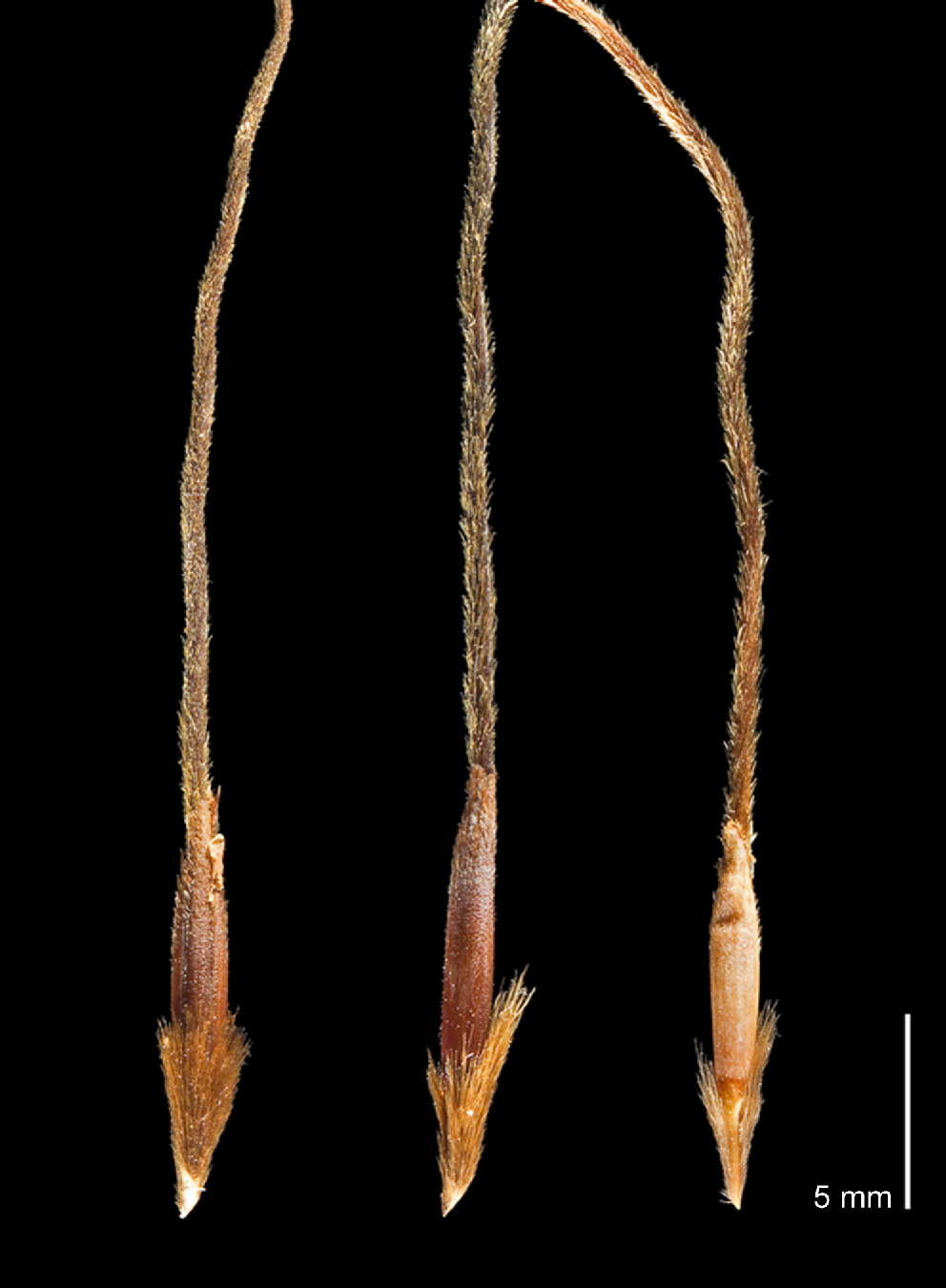
Themeda growers often use a ‘thumbnail’ test, which involves pressing the ripe seed between their thumbnail and forefinger to see whether the seed is filled. In seed laboratories and gene banks, seed fill can be tested by X-ray or by a cut test if X-ray technology is not available (Martyn Yenson et al. 2021). Seeds displaying any signs of damage including variable texture (i.e. soft spots), insect attack or reduced volume (i.e. air gaps) are not considered filled and should not be included in germination testing (Martyn Yenson et al. 2021). Seed-fill percentage can be quantified by dividing the number of filled seeds by the number of dispersal units × 100 (Martyn Yenson et al. 2021).
Seed viability
Viable seed includes both seeds that will germinate readily under the right conditions and seeds that are alive but dormant and can germinate at some future point in time if the conditions are suitable (Martyn Yenson et al. 2021). Understanding whether seed is dead or dormant is central to defining seed quality (Pedrini and Dixon 2020). Viability tests are important for calculating seeding rates (Mason 2005), for research including seed-use efficiency studies and for providing quality assurance for purchasers. However, there is a lack of standardisation in viability testing for Themeda.
A cut test can be used to check whether there is an embryo and endosperm (Saleem et al. 2009; Farley et al. 2013; Stevens et al. 2020; Martyn Yenson et al. 2021). Although cut testing was a useful tool in the field, Farley et al. (2013) found that it was not very accurate and only tetrazolium (2,3,5-triphenyl-2H-tetrazolium chloride, abbreviated as TZ) staining showed that ~50% of filled seeds were dead. Others have found that the Themeda caryopsis is small, is difficult to cut accurately and the embryo can be hard to identify without staining (Cowley et al. 2023). For these reasons, it is suggested that a TZ test (Martyn Yenson et al. 2021) is used to estimate Themeda seed viability (see Pedrini and Dixon 2020 for methods). Some studies have used TZ to determine Themeda viability (Groves et al. 1982; Baxter et al. 1994; Baxter 1996; Read and Bellairs 1999), but the concentration varied between 0.5% and 1%. Few studies have reported uncertainty in assessing seed viability, yet it is known that both cut tests and TZ tests can be difficult to interpret (Martyn Yenson et al. 2021) and require standardisation for each species (Pedrini and Dixon 2020). However, Baxter (1996) developed a detailed visual key for empirically assessing Themeda seed viability by using TZ staining, which is little known but could be useful for a national or international protocol. Under this protocol, seed is cleaned to the caryopsis, imbibed in distilled water for 24 h and then incubated for 8 h in 1% TZ. Staining patterns are then assessed using the visual key (Fig. 3).
A staining key for evaluating TZ testing of Themeda triandra seed cleaned to the caryopsis. Diagrams on the left show the surface-staining pattern of the caryopsis. Diagrams on the right show staining patterns after longitudinal cross-section. (a) Parts of the caryopsis, including radicle (r), plumule (p), scutellum (s) and endosperm (e). Red staining indicates viability. (b) A viable caryopsis: the radicle, plumule and scutellum are stained red. (c, d, e) Unviable seeds, as indicated by the lack of red staining of the radicle, plumule or scutellum. (Image from Baxter (1996) digitised to improve quality and included with the permission of Brent Baxter).
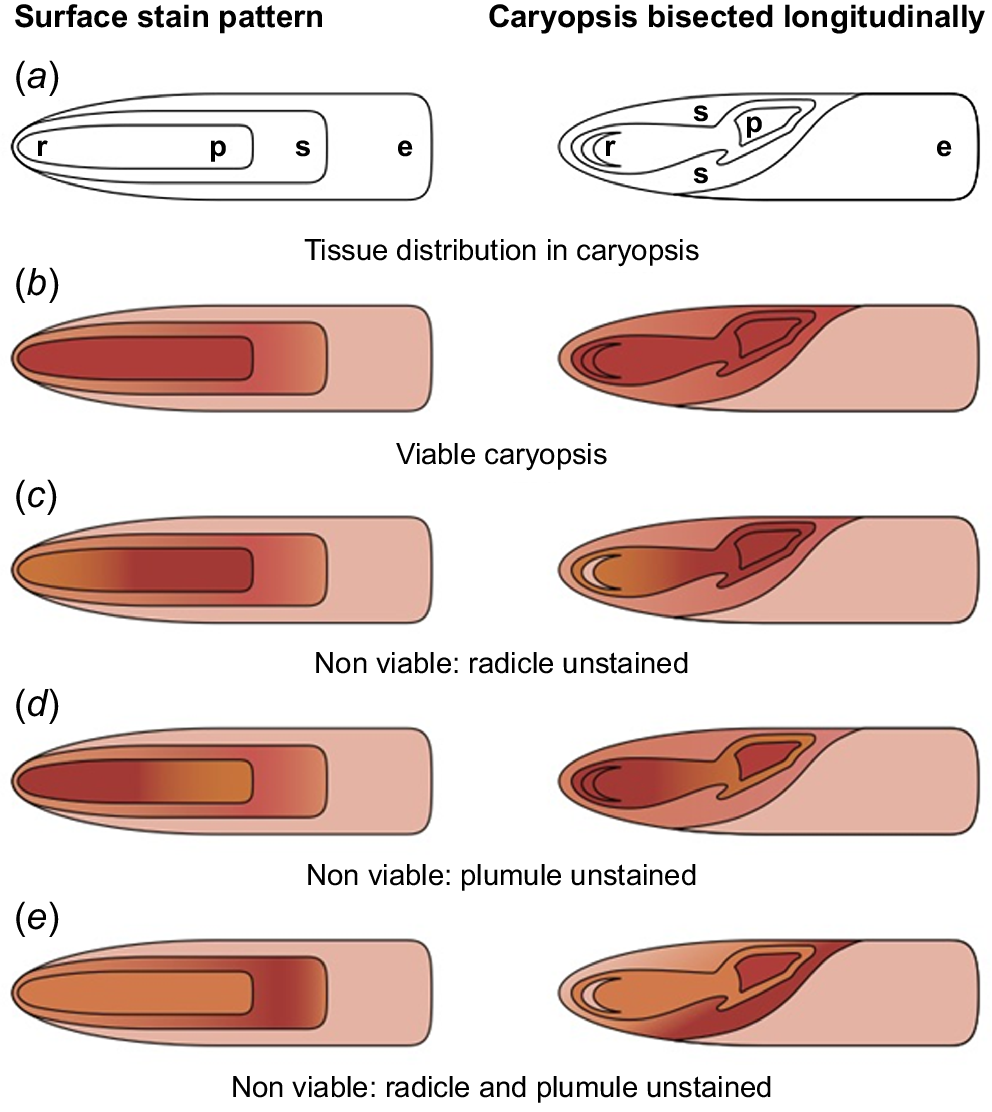
Seed viability can be affected by seed age, seed storage conditions prior to use and the environmental conditions while seeds are developing (Groves et al. 1982; Mason 2005; Saleem et al. 2009). For example, seed stored for 48 months at 25°C decreased in viability from 86% to 51% (Baxter 1996), and Mason (2005) found that seed retained its viability of 45% for 48 months, but by 60 months viability fell to just 12%. In terms of best practice, storing Themeda seeds under dry and cool/cold conditions will likely improve their lifespan in storage (Martyn Yenson et al. 2021), given that seeds are thought to be tolerant of desiccation and subzero storage (i.e. orthodox seed-storage behaviour: SER, INSR and RBGK 2023).
Seed germination
Most collections of Themeda display some level of seed dormancy (see the ‘overcoming dormancy and stimulating germination’ section below), making germination testing difficult. Inconsistencies in the methods used to germinate seed make the results difficult to compare (Table 1). In addition, there is no consistent definition of ‘germination’ for Themeda across studies. Emergence of the radicle is the common denominator, but there was no minimum radicle length defined in some studies (Martin 1975; Baxter et al. 1995; Read and Bellairs 1999). Other studies used 1 mm (Baxter et al. 1993, 1994), 2 mm (Stevens et al. 2020), 3 mm (Farley et al. 2013) or 5 mm (Mott 1978) or the emergence of the radicle or both the radicle and plumule (Groves et al. 1982).
Author/s | Seed provenance | Seed age | Substrate | Days A | Cut or TZ | Light:dark | Temperature | |
---|---|---|---|---|---|---|---|---|
Baxter et al. (1993) | Drakensburg and Zululand, Kwazulu-Natal, South Africa | ~8 weeks | Filter paper | 14 | TZ | 12 h:12 h | Tested a range of constant and alternating temperatures | |
Baxter et al. (1994) | Drakensburg and Zululand, Kwazulu-Natal, South Africa | 8 weeks | Filter paper | 10 | TZ | 24 h:0 h | (a) Dry smoke: 30°C; (b) smoke water: 15°C, 20°C and 30°C | |
Baxter et al. (1995) | Drakensburg and Zululand, Kwazulu-Natal, South Africa | 8 weeks | Filter paper | 10 | TZ | 24 h:0 h | 30°C | |
Baxter (1996) | Drakensburg and Zululand, Kwazulu-Natal, South Africa | (1) 8 weeks or (2) non-dormant | Filter paper | 14 | TZ | 24 h light or 24 h dark | 30°C | |
Clarke et al. (2000) | New England region, NSW, Austalia | 3–6 months | Sponge | 28 | TZ | 12 h:12 h | 25/15°C | |
Clarke et al. (2010) | Four populations from Walkamin, Qld, and one from Perth Hills, WA, Australia | <8 weeks | Filter paper | 28 | X-ray | (a) 12 h:12 h; (b) 24 h darkness | (a) 26/13°C; (b) 20°C | |
Cresswell and Nelson (1972) | Johannesburg region, South Africa | ~2 months | 2 filter papers | 4 | TZ | 0 h:24 h | 25°C | |
Farley et al. (2013) | Gympie, Qld, Australia | 4–8 weeks | 2 filter papers | 21 | Cut and TZ | 24 h:0 h | 25°C | |
Ghebrehiwot et al. (2012) | Trial site, Pietermaritzburg, South Africa | From soil seed bank | Soil from seed bank | 90 days | No | Natural light of greenhouse | 22°C | |
Groves et al. (1982) | Seven populations from Australia and one from PNG | >24 months | 4 filter papers | 14 | TZ | (a) 8 h:16 h; (b) and (c) heat and cold treatment: 0 h:24 h | Tested a range of constant and alternating temperatures | |
Hagon (1976) | Canberra, ACT, Australia | (1) dormant 4–8 weeks; (2) after-ripened 12–15 months | 4 filter papers | 14 | No | 8 h:16 h | 30/20°C | |
SER, INSR and RBGK (2023) | Botswana | Not stated | 1% agar | 42–105 days | Not stated | 12:12 h, 8:16 h | 33/19°C, 35/20°C | |
Martin (1975) | Near Johannesburg, South Africa | Fresh seed | 2 filter papers | 48 h | No | Darkness | 25°C | |
Mason (2005) | 10k radius of Melbourne, Vic, Australia | Fresh seed | Nutrientfree agar | 14 | Not stated | 12 h:12 h | 35/20°C | |
Read and Bellairs (1999) | Lithgow, NSW, Australia | <12 months | 2 filter papers | 42 | TZ | 12 h:12 h | 30/20°C | |
Saleem et al. (2009) | Unclear | Various | 2 filter papers | (1) 28, (2) 42 | No | Not stated | 24°C | |
Stevens et al. (2020) | Albury, Batemans Bay and Sydney, NSW, Australia | (1) 12 months (2) 117 days | Soil | 28 | Cut | (1) 12 h:12 h | (1) 25/15°C or 30/20°C, (2) 40°C and 40% RH |
It is important to maintain moist conditions for Themeda germination testing (Groves et al. 1982), because seeds can be sensitive to moisture stress (Hagon and Chan 1977; Groves et al. 1982; Stevens et al. 2020). There was variation in the substrates used for this purpose in germination tests, including filter paper (Cresswell and Nelson 1972; Hagon 1976; Groves et al. 1982; Baxter et al. 1993, 1994, 1995; Read and Bellairs 1999; Clarke et al. 2010), 1% agar (Stevens et al. 2020), soil (Saleem et al. 2009) and sponge (Clarke et al. 2000). Water added to the Petri dish has varied from 4.5 mL (Baxter et al. 1993, 1994), to a high of 15 mL (Farley et al. 2013), and some studies also used a parafilm seal (Clarke et al. 2010; Stevens et al. 2020). Most studies did not use seed surface sterilisation prior to germination assays, but some used saturated bromine water (Martin 1975) or Previcur® fungicide solution (Read and Bellairs 1999; Farley et al. 2013), seemingly without the ‘nil treatment’ controls recommended by Baskin and Baskin (2014).
The awn is often removed for germination testing, which does not diminish and may enhance seed germination (Sindel et al. 1993). Themeda seed dormancy is partially due to the mechanical pressure exerted by its tightly bound covering structures (Martin 1975; Farley et al. 2013), and their removal may increase the range of temperature and moisture conditions under which seed can germinate, as seen in other grasses (Lewandrowski et al. 2018). Many authors have reported an increase in germination of ~25–30% after removing the glumes, i.e. cleaning the seed to the caryopsis (Martin 1975; Hagon 1976; Baxter 1996; Read and Bellairs 1999; Farley et al. 2013). Whereas this may be useful in a laboratory setting, other authors have mentioned the risks of glume removal for field applications (Clarke et al. 2010). Removing the tightly bound covering structures can be difficult and expensive and risks damaging the seed (Sindel et al. 1993; Clarke et al. 2010). It may also increase seed desiccation and/or microbial infection (Lamont and Milberg 1997).
The duration of germination tests has ranged from 10 days (Mott 1978; Baxter et al. 1995) to 14 (Hagon 1976; Groves et al. 1982), 21 (Farley et al. 2013), 28 (Stevens et al. 2020) or 42 days (Read and Bellairs 1999). The calculation of germination rates is a further source of inconsistency, with some authors subtracting dead seed before calculating the percentage of germinated seed (Mott 1978; Read and Bellairs 1999).
There have been many differences in light regimes, including no light (Cresswell and Nelson 1972), and 8 h (Hagon 1976), 12 h (Baxter et al. 1993; Clarke et al. 2000; Saleem et al. 2009) or 24 h (Baxter et al. 1994) of light. For two South African populations (Baxter 1996), and one population from New South Wales, Australia (Clarke et al. 2010), light gave no increase in germination compared with ‘no light’. In contrast, Hagon (1976) found an increase from 76% to 83% germination with 8 h of light compared with no light in non-dormant seed in a population from Canberra, Australia. This suggests that the requirement for light for germination may vary among populations.
Optimal germination temperatures for Themeda have been widely investigated. Mott (1978) tested a range of constant temperatures between 19°C and 42°C on Themeda from the Northern Territory in Australia and found that 30°C was optimal for germination. Expanding to more geographically diverse populations, Groves et al. (1982) compared seven constant temperatures between 15°C and 45°C and found that germination responses were population dependent. In South Africa, Baxter (1996) compared Themeda germination of one population at six constant temperatures (5°C, 15°C, 25°C, 30°C, 35°C and 40°C). Germination rates and optimum temperatures varied depending on whether the seed was after-ripened or not. For fresh seed, germination was highest at temperatures of 30°C, 35°C and 40°C, compared with 25°C for non-dormant seed (Baxter 1996).
Even though Themeda germinates well at constant temperatures, it is thought that alternating day/night temperatures may be superior (Hagon 1976; Baxter et al. 1993). Under seven temperature regimes (35/25°C, 30/20°C, 30/15°C, 25/15°C, 25/10°C, 20/10°C, 15/5°C) used across eight geographically dispersed Australian populations, after-ripened Themeda germinated in approximately 3 days at both 35/25°C and 30/20°C, extending to nine days at 20/10°C (Groves et al. 1982). Germination percentages and optimum temperatures varied among populations, and one population from Alice Springs, Australia, required high germination temperatures (45/20°C). A comparison of two geographically diverse South Africa populations assessed six alternating temperatures (35/20°C, 30/20°C, 30/15°C, 25/15°C, 25/10°C and 20/5°C: Baxter et al. 1993). Again, results varied among populations, but optimal germination (99% of viable seed) for both populations was at 30/20°C.
In conclusion, although Themeda will germinate under a wide range of conditions, germination appears to be optimal for many populations at either 25/15°C or 30/20°C. Although light is not needed for germination, both light and optimal germination temperatures may be population dependent.
Overcoming dormancy and stimulating germination
Seed dormancy is common among Australian native plants (Martyn Yenson et al. 2021) and the grass family (Poaceae) generally, with at least 78 of the 177 grass genera having species with seed dormancy (Simpson 2010). Seed dormancy helps prevent germination when growing conditions may be hostile to survival (Hagon 1976; Baxter et al. 1993; Martyn Yenson et al. 2021) and is sometimes referred to as ‘bet hedging’, i.e. using seed dormancy to spread the risks of germination in an uncertain environment over a longer period (Lewandrowski et al. 2018). Seed dormancy has a commercial impact on growers and on end-users (Reseigh et al. 2008). Growers must choose between selling partially dormant seed or storing seed until dormancy wanes, and buyers may be purchasing seed that will not germinate for some time (Reseigh et al. 2008).
In grasses, including Themeda, there are two components to seed dormancy, namely (1) chemical inhibition of germination and (2) physical restriction of the embryo caused by the glumes tightly covering the seed, classified as non-deep physiological dormancy (PD) (Martin 1975; Baxter et al. 1993; Baxter 1996; Baskin and Baskin 2014; Baskin and Baskin 2020). For Themeda, this phenomenon has been well studied internationally (Cresswell and Nelson 1972; Martin 1975; Hagon 1976; Groves et al. 1982; Baxter et al. 1994; Baxter 1996). Breeding to reduce seed dormancy has been suggested (Male et al. 2022); however, this may risk reducing its fitness for ecological restoration, given Themeda’s high level of regional adaptation.
Physiological dormancy of fresh Themeda seed is common and can be higher than 90% at seed shed (Martin 1975; Baxter et al. 1994; Clarke et al. 2010). Physiological dormancy varies with population, region, climatic conditions and season (Groves et al. 1982; Baxter et al. 1993; Saleem et al. 2009). A period of ‘after-ripening’ (i.e. dry storage at room temperature) is usually needed before Themeda seed reaches its full germination potential (Groves et al. 1982; Saleem et al. 2009; Clarke et al. 2010; Stevens et al. 2020). During this after-ripening, resources are transferred to the embryo, increasing its osmotic potential and allowing the mechanical restraint imposed by the glumes to be overcome (Cresswell and Nelson 1972; Martin 1975).
In a comparison of fresh seed from populations across Australia, two populations of Themeda seed had little dormancy, whereas most had high dormancy that lasted for 6–10 months after seed drop (Groves et al. 1982). Dormancy increased with aridity; seed from Alice Springs, Australia, did not germinate for 2 years, compared with a gradual increase from 28% to over 80% germination after 11 months of storage for seed from the Canberra region, Australia (Hagon 1976). A South African study found that dormancy varied among populations and was significantly reduced in dry storage at room temperature after 10–14 months (Cresswell and Nelson 1972). Two-month-old South African seed had just 6.4% germination, whereas by 11 months, germination of the same seed was 89.7% (Cresswell and Nelson 1972). A ‘rule of thumb’ recommendation to minimise seed dormancy issues is therefore to store dormant Themeda seed at room temperature for 8–12 months prior to use (Cresswell and Nelson 1972; Martin 1975; Groves et al. 1982; Baxter 1996; Saleem et al. 2009), and approximately 2 years for populations from central Australia (Groves et al. 1982).
Treatments to overcome dormancy
For purposes that require on-demand germination, such as in the post-mining remediation industry and for academic research, the need for more than 8 months of seed after-ripening before use is a significant impediment. The following section reviews dormancy alleviation and germination-stimulation techniques for Themeda.
Treatment with high success but low replication
For seed collected around Canberra, Australia, exposure of fresh seed to alternating day/night temperatures of 64/24°C for 1 month overcame dormancy (i.e. ~100% germination) (Hagon 1976). The reasons for this were not known, but increased biosynthesis of gibberellin was proposed (Hagon 1976). Further testing of this method with more populations is warranted.
Treatments with variable success
The addition of GA3 commonly results in an increase in Themeda germination but this varies among studies and populations. Cresswell and Nelson (1972) tested concentrations from 0.1 mg L−1 to 100 mg L−1 and found that 100 mg L−1 GA3 was optimal, with ~70% germination of fresh seed, whereas Baxter (1996) found that optimal concentrations varied among populations, with 100 mg L−1 for one and 500 mg L−1 for another. By contrast, Groves et al. (1982) found that six of eight populations showed no response to GA3 at 100 mg L−1. The exceptions were fresh seed from Bunbury, Western Australia, which increased from 24% to 87% germination, and from Katherine, Northern Territory, germination increased from 38% to 99%. Further, cleaning the seed to the caryopsis can enhance GA3 treatment by ~30% (Hagon 1976; Mott 1978; Farley et al. 2013).
Seeds have also been treated with GA3 (100 mg L−1) prior to field sowing to determine whether this will increase germination of fresh seed. In one study, germination was not different from the control (Clarke et al. 2010). Of concern, another study found that Themeda seedlings pre-treated with GA3 grew abnormally (Baxter 1996).
Reactions to smoke compounds are species specific and may include increased germination, inhibition of germination or a neutral response, depending on the source, concentration, and form of the plant-derived smoke (Brown 1993; Dixon et al. 1995; Read and Bellairs 1999; Flematti et al. 2015). A range of products derived from burning plants have been tested for their ability to stimulate Themeda germination, including smoke, smoke water, char and karrikinolide. These products can be hard to standardise because the source materials vary among regions, making comparisons among studies and/or replications difficult. Other variables include the concentration of smoke or smoke water, smoking times, and the degree of seed imbibition.
Although not well-studied, char from native species inhibited Themeda germination from 52% to 27% (Marsden-Smedley et al. 1997), whereas ash had no effect (Baxter et al. 1994). The remainder of this section reviews the effects of smoke and smoke water on Themeda seed germination.
Themeda seed can also be treated by passing cool smoke derived from plant materials over the seed (Baxter et al. 1994; Marsden-Smedley et al. 1997), although the increase in germination from this treatment has been inconsistent. In one case, there was no change from the control (Marsden-Smedley et al. 1997), but other studies have shown benefits. A study of smoking periods (0, 5, 15, 45 and 90 min) demonstrated that soaking seeds in water for 36 h and then smoking for 15 min was optimal, and increased germination from 5% to 26% (Baxter et al. 1994). Read and Bellairs (1999) found that smoke increased germination from 75% to 87%, and the effect was greater (from 69% to 92%) when the seed was cleaned to the caryopsis before smoking. Karrikinolide, a smoke-derived compound, applied at 0.1 mg L−1, increased germination of five highly dormant populations by ~30% (Clarke et al. 2010).
Themeda’s germination response after smoking can also vary with the material used to create the smoke. When Themeda from Natal Drakensburg, South Africa, was treated with smoke derived from 27 species individually, only 18 of the 27 species significantly increased the germination of dormant seed. Further, smoke from four individual species (Aristida junciformis Trin. & Rupr., Diospyros austro-africana De Winter, Erica woodii Bolus, and Sopubia cana Harv.) was significantly better than Themeda-derived smoke (Baxter et al. 1995). Aristida junciformis smoke was most successful and increased germination from 6% to 25%.
It is difficult to compare the results of smoke-water experiments because the smoke water has been made in different ways and with different plant materials. Dixon et al. (1995) described a method for producing smoke water where smoke is bubbled for 90 min through a column of water, which is then diluted before use. Using a range of Western Australian plants, the time was modified to 60 min in the study by Read and Bellairs (1999) who diluted it with distilled water to a 10% concentration. This increased Themeda germination from 61% to 78% and cleaning the seed to the caryopsis increased germination by a further 5% (Read and Bellairs 1999). It should be noted that the control seed used in this study had low dormancy to begin with. In South Africa, Baxter et al. (1994) tested two smoke extracts. One was made by bubbling smoke from Themeda grass through 5 L of water for 45 min, the other by bubbling a mixture of dried and green fynbos plant material through 2.5 L of water for 30 min. Using seed with high dormancy (96%) and a range of dilutions, they found that a 2% dilution of the fynbos mixture gave maximum germination of 53%, whereas dilutions above 2% inhibited germination (Baxter et al. 1994). In a laboratory study using the soil seed bank from a Themeda-dominated research plot, Themeda emergence increased from five plants (control) to ~30 plants with the addition of smoke water (prepared using the Baxter et al. 1994 Themeda smoke method), whereas heat of 100°C for 15 min plus smoke water increased emergence to ~48% (Ghebrehiwot et al. 2012). Methods for preparing and standardising smoke water have recently been described (Gupta et al. 2020). Although smoke and smoke products appear to give less consistent results than GA3, for social, cultural or practical reasons (i.e. no access to laboratories or chemicals), these methods may be preferred. In this case, the material used, smoke or smoke product concentrations and treatment times will need to be determined for each population.
One study using accessions from two climatically different locations in South Africa found that boric acid at 0.5 mg L−1 improved germination, such that 2-month-old seed had a higher germination rate than the same seed batch at 14 months of age (80–85%) (Cresswell and Nelson 1972). Gibberellic acid and boric acid combined gave only slighter better results than did boric acid alone, whereas gibberellic acid alone gave poorer results than did boric acid. However, a further study by Baxter (1996) tested boric acid concentrations from 0 to 5 × 10−4 and found no increase in germination for dormant seed. Similarly, a combination of boric acid and GA3 did not increase germination.
A range of heat treatments prior to germination testing have been applied and results depended on populations (Groves et al. 1982) and seed age (Baxter 1996). Stevens et al. (2020) found that 2 months at 40°C and 40% relative humidity reduced dormancy from 55% to 10% in the most dormant population. At the same temperature, using fresh seed, 100% of viable seed germinated by 16 weeks, but viability decreased from 81% to 62% (Baxter 1996), suggesting that there is an optimum treatment length. Treatment at 70°C for 1 week increased germination of dormant seed from 13% to 48% (Baxter 1996), whereas Clarke et al. (2010) stored seed at 50% relative humidity for 4 weeks then at 45°C for 1, 2 and 3 months. Maximum germination was reached after 2 months. A lack of seed prevented testing of other combinations of relative humidity and temperature, but the authors suggested this would be worthwhile (Clarke et al. 2010). A systematic test of heat treatments would be useful further research.
Chilling Themeda seed prior to germination has given mixed results and may be temperature, chilling duration, and population specific (Table 2). Germination rates of seven populations after seed chilling did not differ from the control (Groves et al. 1982), but other populations have had a marked response (Groves et al. 1982; Baxter et al. 1993; Clarke et al. 2010). The highest increases in germination rates were for either (a) a short chilling period of 7 days (Clarke et al. 2010) or (b) for accessions from cold regions such as Tantangara, NSW, Australia where the long-term mean temperature in July is 3.9°C (Bureau of Meteorology 2024). Similarly, two South African populations from regions with different average winter temperatures showed differences in their response to chilling. The optimal chilling time for a population from Umfolozi Game Reserve (altitude 90 m/warmer winters) was 5 days, whereas the optimal time for a population from Cathedral Peak (altitude 1800 m/colder winters) was 30 days (Baxter et al. 1993).
Author/s | Provenance | Chill (°C) | Time | Germination | |
---|---|---|---|---|---|
Mott (1978) | Mundubbera, Qld, Aus | 4 | 4 or 8 weeks | Not different from control | |
Groves et al. (1982) | Tantangara, NSW, Aus | 4 | 6 weeks | Increase from ~15% to ~90% | |
Groves et al. (1982) | PNG: Lae Australia: Katherine, NT, Alice Springs, NT, Mundubbera, Qld, Adelaide Hills, SA, Bunbury, WA, Hobart, Tas | 4 | 6 weeks | Not different from control | |
Baxter et al. (1993) | 5 | 5 days | Increase from 19% to 61% | ||
Baxter et al. (1993) | 5 | 30 days | Increase from 5% to 68% | ||
Clarke et al. (2010) | 5 | 1 week | Increase from 36% to 64% |
Some treatments, including potassium nitrate, seed scarification and water imbibition prior to germination have been unsuccessful, at least on the populations tested (Table 3).
Treatment | Author/s | |
---|---|---|
Ash | Baxter et al. (1994); Marsden-Smedley et al. (1997) | |
Aqueous ash extracts | Baxter et al. (1994) | |
Cobalt (concentrations not clearly specified) | Cresswell and Nelson (1972) | |
Ethrel – wide range of concentrations | Baxter et al. (1994) | |
Ethylene – wide range of concentrations | Baxter et al. (1994) | |
Excised caryopsis, allowed to imbibe water for 1 day, refrigerated for 2 days then set on filter paper moistened with 0.1% application | Evans and Knox (1969) | |
Micronutrients at various concentrations: copper, iron, manganese, molybdenum, molybdenum plus nitrate or ammonia, zinc | Cresswell and Nelson (1972) | |
Potassium nitrate (KNO3) at (0.2%). | Hagon (1976); Mott (1978) | |
Relative humidity of 10%, 50%, 90% and 100% for 35, 70 or 105 days of storage. Groves et al. (1982) concluded that relative humidity played a minor role in reducing dormancy of fresh seed. | Groves et al. (1982) | |
Scarification | Farley et al. (2013); Martin (1975) | |
Water imbibition prior to germination | Mott (1978) |
For grasses, germination rates have been improved using wetting and drying cycles that simulate conditions found in their natural environment (Lewandrowski et al. 2018); however, to our knowledge, this has not been tested for Themeda. In a study of six Triodia (R. Br.) species, three species showed a four-fold increase in germination after eight wet–dry cycles (48 h wet then dry at 50% RH for 12 days × 8 cycles) compared with the control (Lewandrowski et al. 2018). Given that many Themeda populations are found in unpredictable environments and that Triodia and Themeda both have physiological dormancy, it may be a useful area for future Themeda research.
Seed characteristics and Themeda ploidy
Themeda is a polyploid complex (based on n = 10) with 2n, 3n, 4n, 5n, 6n, 8n, 9n and 11n cytotypes (Hayman 1960; Birari 1983; Liebenberg 1986; Godfree et al. 2017). Broadly speaking, diploids dominate in cooler/wetter areas, whereas in Australia tetraploids dominate in hotter/drier environments, and in South Africa hexaploids are found at higher-altitude environments, with tetraploids occurring between the coastal and high altitude areas (Hayman 1960; Liebenberg 1986; Godfree et al. 2017). However, recent genetic research suggests that many populations in Australia have mixed ploidy types (Ahrens et al. 2020). Until recently, it was hypothesised that tetraploids evolved from diploid populations (Hayman 1960; Godfree et al. 2017), but recent genome sequencing suggests that they colonised Australia separately (Dunning et al. 2022).
Themeda’s reproductive strategies are variable (Woodland 1964; Evans and Knox 1969). In Australia, sexual reproduction may be more common in diploids and apomixis more common in tetraploids, but races often retain both sexual and apomictic pathways regardless of ploidy (Evans and Knox 1969; Liebenberg 1990). In South Africa, diploids were found to have sexual reproduction, whereas tetraploids were either obligate or facultative apomicts (Liebenberg 1990).
Recent research is shedding light on the differences between diploid and tetraploid seed. When compared with diploids, tetraploids had seed that was ~35% larger (Stevens et al. 2020), with longer awns (Godfree et al. 2017). They were fitter than diploids in hotter, drier conditions (Godfree et al. 2017) and had both higher dormancy and higher seedling vigour (Stevens et al. 2020). Tetraploid seed was more dormant (55% dormancy) than diploid seed (35% dormancy) and germinated in a narrower range of temperatures and water potentials (Stevens et al. 2020). Although there are clear differences among cytotypes, Themeda ploidy is rarely reported in germination and dormancy studies and is an important inclusion for future studies.
Suggestions for further research and practice
Biology and ecology
We have shown that Themeda seed quality depends on region, climate, ploidy, and season. Seed purity and seed fill may be low, seed viability is highly variable, and high seed dormancy of 8–12 months is common in many populations. Given Themeda’s high variability, a national or international Themeda seed collection representing Themeda’s genetic and geographical diversity is needed for future research. The Australian Pastures Genebank is one possible home for such a collection. Future germination and dormancy studies should include populations from diverse geographical locations with both diploid and tetraploid cytotypes because responses are known to be specific to population, climate and cytotype (Groves et al. 1982; Godfree et al. 2017; Stevens et al. 2020). Dormancy-breaking treatments (e.g. heat or cold stratification) and combinations of treatments should be tested systematically. This type of research has been hindered by seed supply (Baxter 1996; Clarke et al. 2010; Stevens et al. 2020) and low access to ploidy analysis (Brian Atwell, pers. comm., BioPlatforms, Australia).
Improving germination and overcoming dormancy
Whereas dry storage after-ripening is the most reliable and economical way to overcome dormancy, for those who do not wish to wait, dormancy breaking remains a vexed area with inconsistent results. For now, cleaning the seed to the caryopsis and adding GA3 at 1 mg L−1 is the most well-studied and reliable method for breaking dormancy in a range of populations, often (but not always) increasing germination of dormant seed by ~30% (Cresswell and Nelson 1972; Hagon 1976; Mott 1978; Groves et al. 1982; Farley et al. 2013) and up to ~60% for one population (Groves et al. 1982). However, because the glumes are tightly held to the caryopsis, the caryopsis can be damaged during cleaning (Clarke et al. 2010); however, this method is too time consuming to be practical for large quantities of seed.
Plant-derived smoke products have potential to improve germination, but the variables are difficult to control, and the response is not better than that to GA3, which is cheaper and easier to obtain and standardise. However, seed smoking may be a more desirable cultural approach for some people or groups (Male et al. 2022). Research with smoke-derived products could be directed towards understanding whether responses to smoke are genetically or geographically determined (or both), and the standardisation of smoking protocols. If consistent results can be attained, pre-treatment of seed with smoke prior to sowing may also be a useful area for further research (Baxter 1996; Clarke et al. 2010).
Studies on a small number of populations have shown that 1 month storage at 64/24°C (Hagon 1976) or heat treatment at 40°C and 40% relative humidity (Stevens et al. 2020) have high potential to increase germination. Also, wet–dry cycling has increased germination in other grasses and may have applications for Themeda (Lewandrowski et al. 2017, 2018). Combinations of treatments may also help reduce the proportion of dormant seeds in a population, for example, wet–dry cycles plus smoke or GA3. Further research could be directed towards a comprehensive study of these methods across a range of accessions and both 2n and 4n cytotypes.
Standardising research protocols
We found that research on Themeda seed viability is not standardised, which makes it difficult for researchers to compare study results. Despite the high variation in methodologies and results of Themeda studies, there are enough data for the development of a national or international germination protocol. The following could now be standardised, for example: emergence of the radicle up to 2 mm length (as per Stevens et al. 2020), a 4-week germination test, two layers of Whatman No. 1 filter paper, addition of 5 mL of distilled water to the germination medium and a parafilm seal for the Petri dishes. Germination temperature could also be standardised, although it may not be optimal for all populations. Recent studies have used either 25/15°C (Mason 2005; Clarke et al. 2010) or 30/20°C (Read and Bellairs 1999; Stevens et al. 2020). Temperatures of 25/15°C could have less risk of fungal contamination (Baskin and Baskin 2014). A comparison of germination substrates would also make useful further research.
For viability testing, cut tests may have some limitations for Themeda (Farley et al. 2013) and results should be confirmed with TZ testing where possible. ISTA does not have tetrazolium-testing protocols specifically for Themeda, but studies have used a generic ISTA TZ test (Baxter et al. 1994; Read and Bellairs 1999; Clarke et al. 2010), suggesting that an ISTA protocol could be adopted along with the interpretation key developed by Baxter (1996).
Seed labelling and standardisation
In Australia, there are now strong moves towards greater standardisation of seed quality. For example, the revegetation industry is beginning to adopt a more rigorous and consistent terminology for seed quality (RIAWA 2023) and 17 organisations have formed the Australian Seedbank Partnership, which launched the Australian Virtual Seedbank in October 2023, providing a centralised database of open-access seed data (Australian Virtual Seed Bank 2023).
Calls for quality labelling of native seeds are growing (Frischie et al. 2020; Pedrini and Dixon 2020), leading seed producers in some countries to form associations and develop industry standards for seed labelling (Mainz and Wieden 2019; Pedrini and Dixon 2020). Proforma labels have been proposed and include the location and date of collection as well as percentages for seed purity, fill and viability (including any dormancy) (Pedrini and Dixon 2020). An indication of likely genetic variation (i.e. number of plants over what area) and suitability to the region where seeds will be used are also important inclusions (Martyn Yenson et al. 2021). Such labelling for Themeda is possible and should be encouraged, given its highly variable seed quality. However, labelling will increase costs and challenges for seed producers, especially small growers and/or those in regions less favourable for seed production. Seed co-ops, where seed and ploidy testing can be performed at low cost, may be one possible solution.
To conclude, this review has synthesised current knowledge about Themeda’s seed characteristics, biology and ecology. It has highlighted the challenges of using seeds from a species with a wide geographical distribution, regional adaptations, variable cytotypes and seed dormancy (see Supplementary Table S1 for a summary of studies). We have provided a baseline for the development of Themeda seed-testing protocols and eventual seed labelling, which will contribute to the use of Themeda in ecological restoration, for seed production and for research.
Data availability
Data sharing is not applicable because no new data were generated or analysed during this study.
Declaration of funding
Marne Durnin was supported by a Commonwealth Government Scholarship and Research Training Place 87029. Emma Dalziell was supported by the Australian Research Council Linkage grant LP200200680.
Acknowledgements
We acknowledge Aboriginal and Torres Strait Islander peoples as the Traditional Custodians of Country. We recognise their enduring connection to land, water, sea, culture and community and their long and continuing association with kangaroo grass. We pay our respects to all Aboriginal and Torres Strait Islander peoples, their Elders, and future leaders and acknowledge that we live and work on Kaurna, Paramangk and Ngunnawal Country. Warmest thanks go to Jiang Chee Tay, Negar Nikmanesh and Alan Humphries from the Australian Pastures Genebank, Australia, for sharing knowledge and networks. Ellen Bennett kindly provided the Themeda seed photograph.
References
Ahrens CW, James EA, Miller AD, et al. (2020) Spatial, climate and ploidy factors drive genomic diversity and resilience in the widespread grass Themeda triandra. Molecular Ecology 29, 3872-3888.
| Crossref | Google Scholar | PubMed |
Australian Virtual Seed Bank (2023) Australian Seedbank Partnership. Available at https://seedbank.ala.org.au/ [Verified 29 January 2024]
Baskin CC, Baskin JM (2020) Breaking seed dormancy during dry storage: a useful tool or major problem for successful restoration via direct seeding. Plants 9, 636.
| Crossref | Google Scholar | PubMed |
Baxter BJM, Van Staden J, Granger JE (1993) Seed germination response to temperature, in two altitudinally separate populations of the perennial grass Themeda triandra. South African Journal of Science 89, 141-144.
| Google Scholar |
Baxter BJM, Van Staden J, Granger JE, Brown NAC (1994) Plant-derived smoke and smoke extracts stimulate seed germination of the fire-climax grass Themeda triandra. Environmental and Experimental Botany 34, 217-223.
| Crossref | Google Scholar |
Baxter BJM, Granger JE, van Staden J (1995) Plant-derived smoke and seed germination: is all smoke good smoke? That is the burning question. South African Journal of Botany 61, 275-277.
| Crossref | Google Scholar |
Birari SP (1983) Chromosomal races of Indian species of Themeda triandra Forrsk. Journal of Maharashtra Argicutural Univerisity 8, 133-137.
| Google Scholar |
Brown NAC (1993) Promotion of germination of Fynbos seeds by plant-derived smoke. The New Phytologist 123, 575-583.
| Crossref | Google Scholar | PubMed |
Bureau of Meteorology (2024) Daily maximum temperature: Cabramurra SMHEA AWS. Summary statistics for all years. Available at http://www.bom.gov.au/jsp/ncc/cdio/weatherData/av?p_nccObsCode=122&p_display_type=dailyDataFile&p_startYear=&p_c=&p_stn_num=072161 [Verified on 16 April 2024]
Clarke PJ, Davison EA, Fulloon L (2000) Germination and dormancy of grassy woodland and forest species: effects of smoke, heat, darkness and cold. Australian Journal of Botany 48, 687-699.
| Crossref | Google Scholar |
Cole I, Lunt ID (2005) Restoring Kangaroo Grass (Themeda triandra) to grassland and woodland understoreys: a review of establishment requirements and restoration exercises in south-east Australia. Ecological Management & Restoration 6, 28-33.
| Crossref | Google Scholar |
Cole I, Prober S, Lunt I, Koen T (2017) Establishment of native grasses and their impact on exotic annuals in degraded box gum woodlands. Austral Ecology 42, 632-642.
| Crossref | Google Scholar |
Cowley JM, Phillips AL, Khor SF, Neumann T, Lim WL, Burton RA (2023) Carbohydrates in kangaroo grass (Themeda triandra Forssk.) grain and perspectives on its food potential. Journal of Cereal Science 111, 103670.
| Crossref | Google Scholar |
Cresswell CF, Nelson H (1972) The effect of boron on the breaking, and possible control of dormancy of seed of Themeda triandra Forsk. Annals of Botany 36, 771-780.
| Crossref | Google Scholar |
Dixon KW, Roche S, Pate JS (1995) The promotive effect of smoke derived from burnt native vegetation on seed germination of Western Australian plants. Oecologia 101, 185-192.
| Crossref | Google Scholar | PubMed |
Drake A, Keitel C, Pattison A (2021) The use of Australian native grains as a food: a review of research in a global grains context. The Rangeland Journal 43, 223-233.
| Crossref | Google Scholar |
Dunning LT, Olofsson JK, Papadopulos AST, et al. (2022) Hybridisation and chloroplast capture between distinct Themeda triandra lineages in Australia. Molecular Ecology 31(22), 5846-5860.
| Crossref | Google Scholar |
Evans LT, Knox RB (1969) Environmental control of reproduction in Themeda australis. Australian Journal of Botany 17, 375-389.
| Crossref | Google Scholar |
Everson CS, Clarke GPY (1987) A comparison of six methods of botanical analysis in the montane grasslands of Natal. Vegetation 73, 47-51.
| Crossref | Google Scholar |
Farley GJ, Bellairs SM, Adkins SW (2013) Germination of selected Australian native grass species, with potential for minesite rehabilitation. Australian Journal of Botany 61, 283-290.
| Crossref | Google Scholar |
Flematti GR, Dixon KW, Smith SM (2015) What are karrikins and how were they ‘discovered’ by plants? BMC Biology 13, 108.
| Crossref | Google Scholar |
Frischie S, Miller A, Pedrini S, Kildisheva OA (2020) Ensuring seed quality in ecological restoration: native seed cleaning and testing. Restoration Ecology 28(S3), s239-s248.
| Crossref | Google Scholar |
Ghebrehiwot HM, Kulkarni MG, Kirkman KP, Van Staden J (2012) Smoke and heat: influence on seedling emergence from the germinable soil seed bank of mesic grassland in South Africa. Plant Growth Regulation 66, 119-127.
| Crossref | Google Scholar |
Godfree RC, Marshall DJ, Young AG, Miller CH, Mathews S (2017) Empirical evidence of fixed and homeostatic patterns of polyploid advantage in a keystone grass exposed to drought and heat stress. Royal Society Open Science 4, 170934.
| Crossref | Google Scholar | PubMed |
Groves RH, Hagon MW, Ramakrishnan PS (1982) Dormancy and germination of seed of eight populations of Themeda australis. Australian Journal of Botany 30, 373-386.
| Crossref | Google Scholar |
Gupta S, Hrdlička J, Ngoroyemoto N, Nemahunguni NK, Gucký T, Novák O, Kulkarni MG, Doležal K, Van Staden J (2020) Preparation and standardisation of smoke-water for seed germination and plant growth stimulation. Journal of Plant Growth Regulation 39, 338-345.
| Crossref | Google Scholar |
Hagon MW (1976) Germination and dormancy of Themeda australis, Danthonia spp., Stipa bigeniculata and Bothriochloa macra. Australian Journal of Botany 24, 319-327.
| Crossref | Google Scholar |
Hagon MW, Chan CW (1977) The effects of moisture stress on the germination of some Australian native grass seeds. Australian Journal of Experimental Agriculture and Animal Husbandry 17, 86-89.
| Crossref | Google Scholar |
Hayman DL (1960) The distribution and cytology of the chromosome races of Themeda australis in southern Australia. Australian Journal of Botany 8, 58-68.
| Crossref | Google Scholar |
Lamont BB, Milberg P (1997) Removal of the testa during germination or establishment increases germinant mortality, decay and water loss. Seed Science Research 7, 245-252.
| Crossref | Google Scholar |
Lewandrowski W, Erickson TE, Dixon KW, Stevens JC (2017) Increasing the germination envelope under water stress improves seedling emergence in two dominant grass species across different pulse rainfall events. Journal of Applied Ecology 54, 997-1007.
| Crossref | Google Scholar |
Lewandrowski W, Erickson TE, Dalziell EL, Stevens JC (2018) Ecological niche and bet-hedging strategies for Triodia (R.Br.) seed germination. Annals of Botany 121, 367-375.
| Crossref | Google Scholar | PubMed |
Liebenberg H (1986) Cytotaxonomic studies in Themeda triandra. I. Chromosome numbers and microsporogenesis. South African Journal of Botany 52, 413-420.
| Crossref | Google Scholar |
Liebenberg H (1990) Cytotaxonomic studies in Themeda triandra Forssk.: Part III: sexual and apomictic embryo sac development in 53 collections. South African Journal of Botany 56, 554-559.
| Crossref | Google Scholar |
Lunt ID (1990) The soil seed bank of a long-grazed Themeda triandra grassland in Victoria. Proceedings of the Royal Society of Victoria 102, 53-57.
| Google Scholar |
Mainz AK, Wieden M (2019) Ten years of native seed certification in Germany – a summary. Plant Biology 21, 383-388.
| Crossref | Google Scholar | PubMed |
Male D, Hunt J, Celestina C, Morgan J, Gupta D (2022) Themeda triandra as a perennial seed crop in south-eatern Australia: what are the agronomic possibilities and constraints, and future research needs? Cogent Food & Agriculture 8, 2153964.
| Crossref | Google Scholar |
Martin CC (1975) The Role of glumes and gibberellic acid in dormancy of Themeda triandra spikelets. Physiologia Plantarum 33, 171-176.
| Crossref | Google Scholar |
Morgan JW (1998) Composition and seasonal flux of the soil seed bank of species-rich Themeda triandra grasslands in relation to burning history. Journal of Vegetation Science 9, 145-156.
| Crossref | Google Scholar |
Mott JJ (1978) Dormancy and germination in five native grass species from savannah woodland communities of the Northern Territory. Australian Journal of Botany 26, 621-631.
| Crossref | Google Scholar |
Native Seeds Pty. (2024) Themeda triandra: kangaroo grass. Available at https://nativeseeds.com.au/product/themeda-triandra/?v=6cc98ba2045f [Verified 4 January 2024]
Nindethana Australian Seeds (2024) Themeda triandra (australis) (eastern states) [reveg grade]. Available at https://www.nindethana.net.au/Product-Detail.aspx?p=3511 [Verified 30 January 2024]
O’Connor TG, Pickett GA (1992) The influence of grazing on seed production and seed banks of some African savanna grasslands. Journal of Applied Ecology 29, 247-260.
| Google Scholar |
Pedrini S, Dixon KW (2020) International principles and standards for native seeds in ecological restoration. Restoration Ecology 28, S286-S303.
| Crossref | Google Scholar |
Prober SM, Thiele KR (1995) Conservation of the grassy white box woodlands: relative contributions of size and disturbance to floristic composition and diversity of remnants. Australian Journal of Botany 43, 349-366.
| Crossref | Google Scholar |
Prober SM, Thiele KR, Lunt ID, Koen TB (2005) Restoring ecological function in temperate grassy woodlands: manipulating soil nutrients, exotic annuals and native perennial grasses through carbon supplements and spring burns. Journal of Applied Ecology 42, 1073-1085.
| Crossref | Google Scholar |
Read TR, Bellairs SM (1999) Smoke affects the germination of native grasses of New South Wales. Australian Journal of Botany 47, 563-576.
| Crossref | Google Scholar |
Revegetation Association of WA (RIAWA) (2023) Purity and viability database. Available at https://www.riawa.com.au/accreditation/purity [Verified 13 December 2024]
Saleem A, Ul Hassan F, Manaf A, Ahmedani MS (2009) Germination of Themeda triandra (kangaroo grass) as affected by different environmental conditions and storage periods. African Journal of Biotechnology 8, 4094-4099.
| Google Scholar |
Sindel BM, Davidson SJ, Kilby MJ, Groves RH (1993) Germination and establishment of Themeda triandra (kangaroo grass) as affected by soil and seed characteristics. Australian Journal of Botany 41, 105-117.
| Crossref | Google Scholar |
Snyman HA, Ingram LJ, Kirkman KP (2013) Themeda triandra: a keystone species. African Journal of Range & Forage Science 30, 99-125.
| Crossref | Google Scholar |
Society for Ecological Restoration, International Network for Seed Based Restoration and Royal Botanic Gardens Kew (SER, INSR and RBGK) (2003) Seed information database (SID). Available at https://ser-sid.org/ [Verified 29 January 2024]
Stevens AV, Nicotra AB, Godfree RC, Guja LK, Kranner I (2020) Polyploidy affects the seed, dormancy and seedling characteristics of a perennial grass, conferring an advantage in stressful climates. Plant Biology 22, 500-513.
| Crossref | Google Scholar | PubMed |
Tainton NM, Booysen PDV (1963) The effects of management on apical bud development and seeding in Themeda triandra and Tristachya hispida. South African Journal of Agricultural Science 6, 21-30.
| Google Scholar |
Vogel KP (2002) The challenge: high quality seed of native plants to ensure successful establishment. Seed Technology 24, 9-15.
| Google Scholar |
Woodland PS (1964) The floral morphology and embryology of Themeda australis (R. Br.) Stapf. Australian Journal of Botany 12, 157.
| Crossref | Google Scholar |