Severely degraded high mountain vegetation recovers under different levels of wild herbivore grazing pressure, 1991–2021
Jamie B. Kirkpatrick

A
Abstract
It is important to understand the way in which wild herbivore grazing affects decadal vegetation dynamics after cessation of unnatural disturbances, especially in a context of climate change.
We investigated the decadal effects of different grazing regimes on treeless subalpine vegetation recovery from stock grazing and burning, on sites of different environmental character and initial state.
At each of four sites, two fenced areas that excluded mammalian herbivores, two that allowed in only rabbits and two grazed control plots were monitored every 5 years between 1991 and 2021. General linear models were developed to explain variation in change over the 30 years in different cover types. The years in which peak and trough values occurred were also determined, as were the incidence and direction of differences between treatments in sites and years.
There was marked variation in change over 30 years between the sites and lifeforms. Exclusion of mammalian herbivores increased the slow rate of revegetation. There was little effect from rabbits by themselves. Unexpectedly, the cover of both short and tall herbs was not promoted by grazing exclusion. Short term climatic variation affected some cover types, with many peaks and troughs in the dry year of 2001, but it was not possible to disentangle decades scale climate change effects from the process of recovery after disturbance.
The slight increase in revegetation rates in the absence of native herbivores and rabbits does not justify culling. Restoration interventions appear to be unnecessary. The prospect of increasing fire incidence and deer numbers suggests that it is desirable to continue monitoring the plots.
Keywords: alpine ecology, alpine regeneration, climate change, conservation biology, ecology, ecosystem dynamics, herbivory.
Introduction
Decadal scale investigations of vegetation change following disturbance are desirable to determine responses to extreme weather events (Luo et al. 2011), especially as projected climate change impacts (Grose et al. 2010) become more severe in their consequences for nature (e.g. Williams and Wahren 2005; Verrall and Pickering 2020). The importance of understanding decadal-scale vegetation recovery in different environments and with different starting points is also high, with one reward being the possibility of targeting of those few situations in which expensive management interventions directed towards restoration are likely to be worthwhile.
Disturbance by stock, fire and bulldozer is widespread in the high mountains of the world, exacerbating problems caused by a warming and drying climate, such as exotic invasion (Iseli et al. 2023). The effects of these disturbances have been most extreme in places, such as Australia, New Zealand and Hawai‘i, where First Nations peoples did not graze stock in the treeless high country.
On the Australian mainland, cessation of grazing resulted in rapid recovery of tall herbs, tussock grasses and shrubs, with small bare patches being colonised by shrubs, then the shrubs by tall herbs and grasses (Carr and Turner 1959a, 1959b; Wimbush and Costin 1979a, 1979b, 1979c; Williams and Ashton 1988; Wahren et al. 1994, 2013; Scherrer and Pickering 2005). Shrub species that were not eaten by cattle (Bos taurus Linnaeus) grew more rapidly than others. In Tasmania, exclosures established in the 1960s and plots established in the 1970s indicated a recovery of bare ground at a very low rate after the exclusion of sheep (Kirkpatrick and Bridle 1999; Bridle et al. 2001; Kirkpatrick and Bridle 2013).
The present paper addresses a set of questions related to the recovery of high mountain treeless vegetation from severe human-induced damage. We used data from 30 years of half decadal monitoring of the Parks and Wildlife Service (PWS) experimental plots on the Central Plateau, Tasmania, Australia. We investigated the effects of different grazing regimes (total exclusion of mammals, rabbits only, control) on vegetation recovery, comparing sites of different environmental character and initial state. In undertaking this inquiry, we determine whether differences in rainfall and temperature between sampling years affected any of the lifeform trajectories. We expected that: grazing would slow the rate of invasion of bare ground (Bridle et al. 2001); herbs, especially tall herbs, would be favoured by grazing exclusion (Bridle and Kirkpatrick 1998, 2001); shrubs would increase then be displaced by grasses and herbs in the ungrazed treatment (Williams and Ashton 1988); sedges/rushes would increase (Kirkpatrick et al. 2002).
Methods
Study area
The study area was the treeless part of the subalpine to alpine Central Plateau of Tasmania between Great Lake and Lake Ada between 1100 m and 1300 m above sea level (Fig. 1). The environment and history of this subalpine to alpine region has been described in detail in Banks (1973). The country rocks are dolerite and basalt. A small icecap to the northwest of the study area during glacial periods ground the igneous dolerite into smaller particles, the fine sand from which forms aeolian dunes and sand sheets (Pharo and Kirkpatrick 1994). Dermosols, rudosols and organosols cover most of the regolith (Kirkpatrick et al. 2014).
Location of experimental sites and the Climate Station. Background from ListMap, Department of Natural Resources and Environment Tasmania, accessed June 2023.
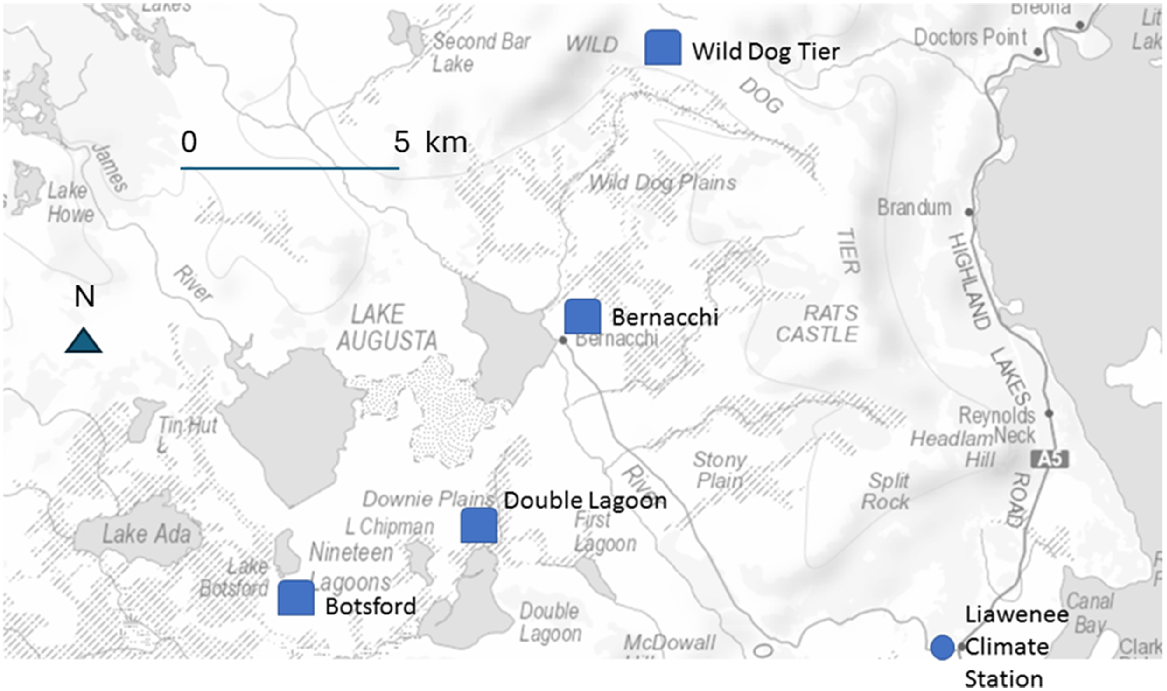
Wombats (Vombatus ursinus Shaw), wallabies (Notamacropus rufogriseus Desmerest) and rabbits (Oryctolagus cuniculatus Linnaeus) graze the vegetation all year, as snow seldom persists for long, even during winter. Over 700 fallow deer (Dama dama Linnaeus) were culled from the Central Plateau subalpine zone in 2023. Their populations are yet to be so dense that their scats are frequently observed in the study area, but there is no doubt that there are sleeper populations (Cunningham et al. 2022). There is circumstantial evidence that they eat species that are not eaten by the other mammalian herbivores (Nichols and Kirkpatrick 2019). They have extremely wide diets, including much browse (Guy et al. 2024).
Sheep (Ovies aries Linnaeus) were removed from the study area in 1989 after grazing it in summer since the early 19th century. The graziers burned to produce green pick for the sheep whenever shrubs were judged too dense. The combination of burning and stock grazing in a high mountain environment produced some of the worst sheet erosion in Australia (Jackson 1973; Shepherd 1973).
After the alpine vegetation of the Central Plateau was included in the Western Tasmanian Wilderness World Heritage Area in 1989, the Parks and Wildlife Service (PWS) established four replicated sets of grazing exclosures and controls (Fig. 1), to gauge the impact of grazing by native vertebrate herbivores and rabbits on the rate of vegetation recovery (Bridle et al. 2001), while simultaneously, and largely successfully, attempting to rehabilitate large bare areas near roads. The four sites with exclosures were all severely eroded in 1991 and represented a range of environments and vegetation types (Bridle et al. 2001).
The Wild Dog Tier site was in alpine vegetation on poorly-drained ground on dolerite. Three quarters of the site was bare ground or rock. The remaining vegetation was alpine heath dominated by Richea scoparia Hook.f. with some cushions of Abrotanella forsteroides (Hook.f.) Bentham. The site near Double Lagoon was on poorly-drained, shallow soils on basalt. Bare ground and rock constituted 65% of the cover, the remaining treeless subalpine vegetation being dominated by cordrush (Baloskion australe (R.Br.) B.G.Briggs & L.A.S.Johnson and A. forsteroides. The site at Bernacchi was on well-drained soil on dolerite, with treeless subalpine vegetation dominated by the shrub Orites acicularis (R.Br.) Roem. & Schult. and ~35% bare ground and rock. The Botsford site was similar to Bernacchi in these attributes.
Data collection
Four exclosures 6 m × 6 m in size or larger were constructed at each of the four sites in 1991, 8 years after burning and 2 years after stock grazing ceased in the study area. The fences were 1.8 m tall and the wire was buried to prevent access by digging. Two of the exclosures had a wire mesh that allowed access to rabbits but not the marsupial grazers (‘rabbits’ treatment henceforth). The other two exclosures denied access to all non-flying mammals. A 5-m long permanently marked transect was laid out in each of the exclosures and two controls at each site. Each of these transects consisted of 10 0.5 m × 0.5 m contiguous quadrats. Data were collected every 5 years between 1991 and 2021.
In each quadrat, outline overlapping covers of individual taxa of all observable vascular plants, moss as a whole, lichen as a whole and litter were estimated by reference to a 100 cell wire grid attached to the quadrat. For common species, the number of cells with more than 50% cover was used as the estimate. For rarer species the percentage covers were estimated for each of the cells in which they occurred, then added up. The percentage of ground and rock with no vascular vegetation above was also estimated.
Precipitation and temperature data by month for 1991–2021 were obtained for the Bureau of Meteorology for the Liawenee station or from climatic interpolations available on TheList (https://www.thelist.tas.gov.au/).
Data analysis
All analyses were undertaken in Minitab (ver. 16, https://www.minitab.com/).
The seasonal and annual mean annual precipitation were calculated by year. The seasons were summer (December of the previous year plus January and February), autumn (March, April, May), winter (June, July, August), spring (September, October, November). The annual means for daily maximum temperature were also calculated. A LOWESS line (segmented regression) was fitted to each of these variables against time using the default options in Minitab.
The values for the following cover types were calculated by adding up the totals of their components: bare ground and rock; cryptogams; shrubs; grasses (Poaceae), sedges and rushes (Cyperaceae, Juncaceae, Restionaceae); tall herbs (inflorescence or foliage typically 10 cm or taller), short herbs. The cover in 1991 was subtracted from the cover in 2021.
Linear models (LM) were developed for change in each of the above cover types. The normality of the residuals from each model was graphically examined to ensure that the model adequately fitted the data. Each LM consisted of location, treatment nested within location and replicate nested within treatment. Means for treatments at each site for each of the cover variables were calculated. One way analysis of variance with Tukey’s multiple range test was used to determine differences in change between treatments for each site–lifeform combination.
One way analysis of variance with Tukey’s multiple range test was used to determine differences between treatments at each site at each time. The directions of significant relationships between treatments were determined from this analysis to complement the LMs. The years of the peak and trough values for each cover type by treatment were recorded to identify short term effects of immediately preceding climatic conditions, if any, on trajectories.
Results
Climatic variability
Total precipitation and precipitation in all seasons declined between 1991 and 2021 (Fig. 2). However, two of our sampling years, 1996 and 2016, were extremely wet, whereas 2001 was extremely dry in summer (Fig. 1).
Annual, summer and winter precipitation by year at Liawenee 1990–2021 showing LOWESS lines with a smoothing of 0.5 and 2 steps and sampling years (vertical lines) (data from Bureau of Meteorology, Australia).
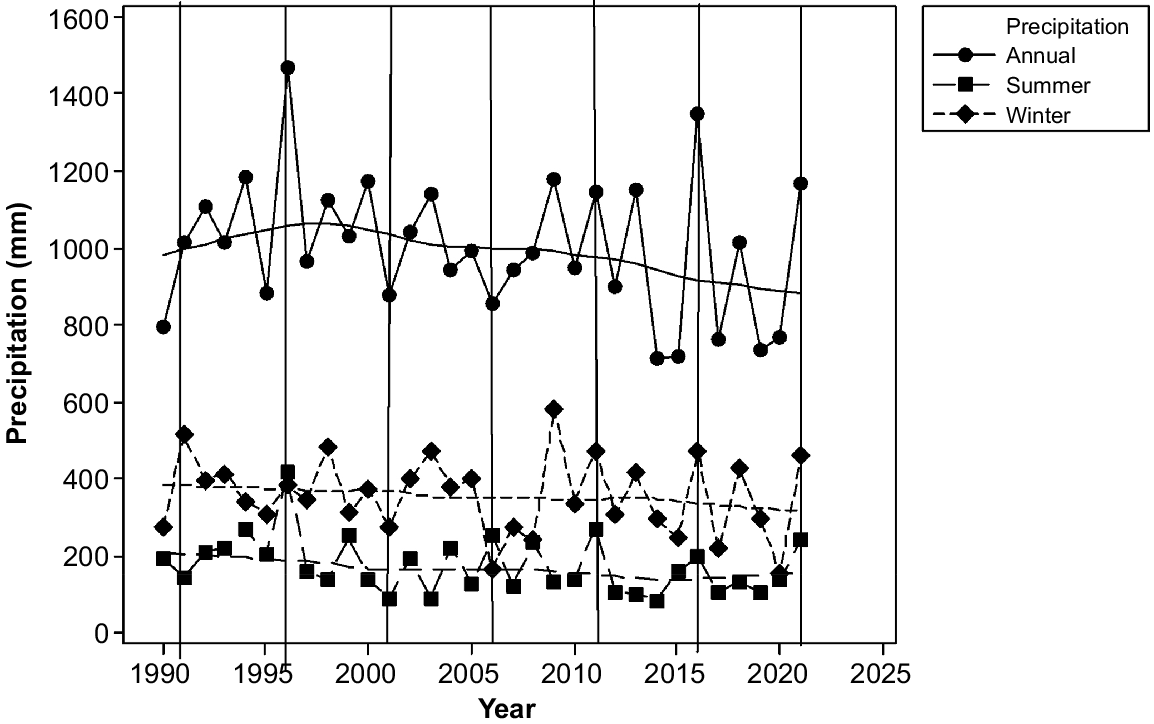
Mean daily maximum temperatures increased from ~10.4°C in the mid 1980s to ~10.8°C from 2012 to 2021 (Fig. 3). However, the temperatures in the sampling years plummeted between 1991 and 1996, increased by over a degree between 1996 and 2001, dropped by half a degree between 2001 and 2006 and changed little thereafter (Fig. 2).
Bare ground and rock
The Botsford and Wild Dog Tier sites exhibited overall mean declines in bare ground and rock cover over the 30 years (Fig. 4), whereas Bernacchi and Double Lagoon were constant (Fig. 3).
Means and 95% confidence limits for bare ground and rock cover by site and time for all treatments. DL, Double Lagoon; WDT, Wild Dog Tier.
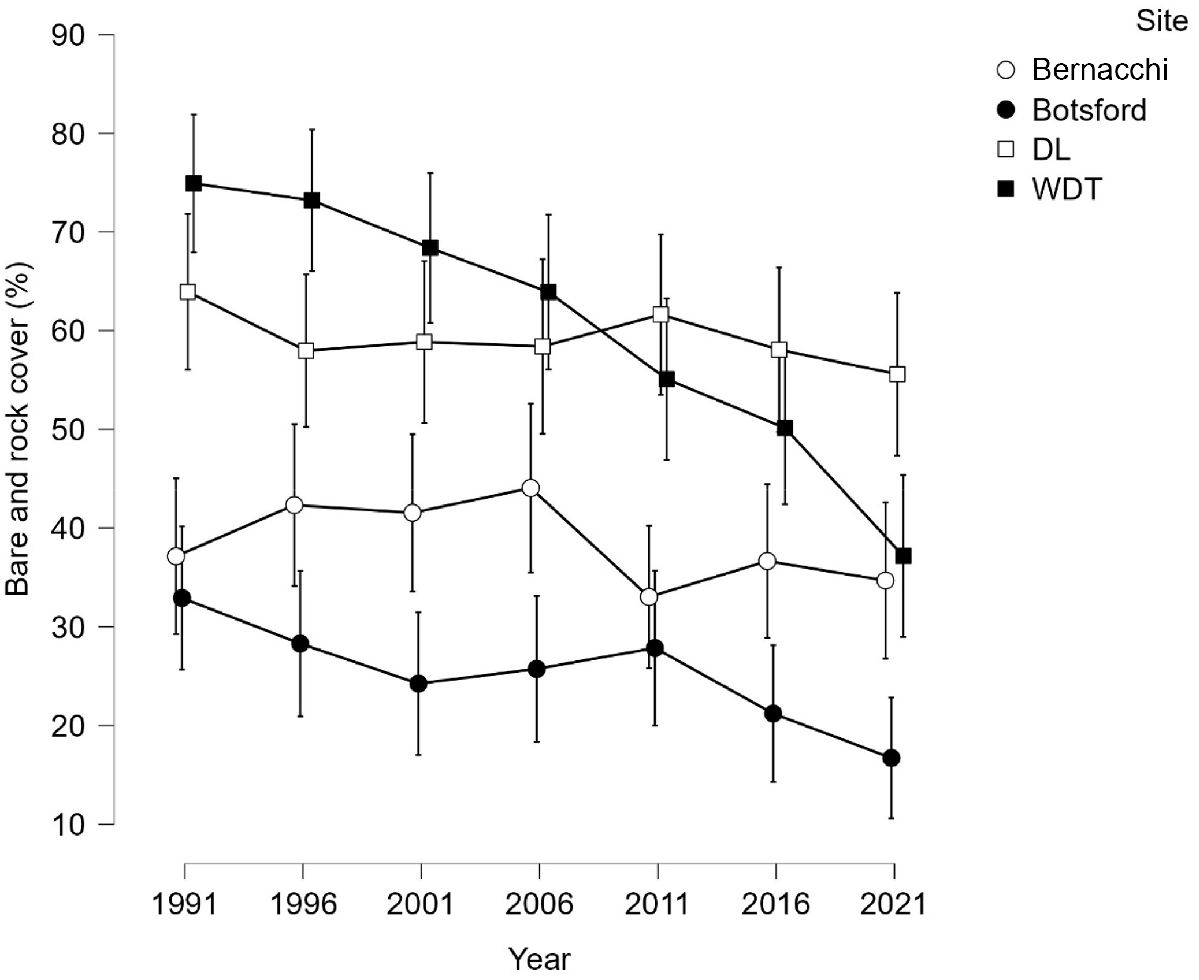
The model for change between 1991 and 2021 was significant for site, treatment and replicate (Table 1). Between 1991 and 2021 the controls went from having the lowest mean to the highest mean (Fig. 5). Bare ground and rock cover increased in the Bernacchi control but decreased in all other combinations of site and treatment. At Bernacchi, the control differed from both other treatments (Table 2). At Wild Dog Tier there was a greater reduction of bare and rock cover in the rabbit plots compared to the control plots (Table 2).
Cover type | Site | Treat | Replicate | R2 (%) | |
---|---|---|---|---|---|
Bare ground and rock | *** | ** | ** | 37 | |
Cryptogam | *** | *** | ns | 29 | |
Shrub | *** | *** | *** | 46 | |
Grass | ns | ns | ns | 14 | |
Sedge and rush | ** | ns | * | 19 | |
Tall herb | ns | ns | * | 15 | |
Short herb | *** | *** | ** | 34 |
These models have site, treatment (nested in site), and replicate (nested in treatment and site) as the explanatory variables.
Significance levels: *** P ≤ 0.001; ** P ≤ 0.01; * P ≤ 0.05; ns, not significant.
Variation in mean bare ground and rock cover by treatment and time showing 95% confidence limits.
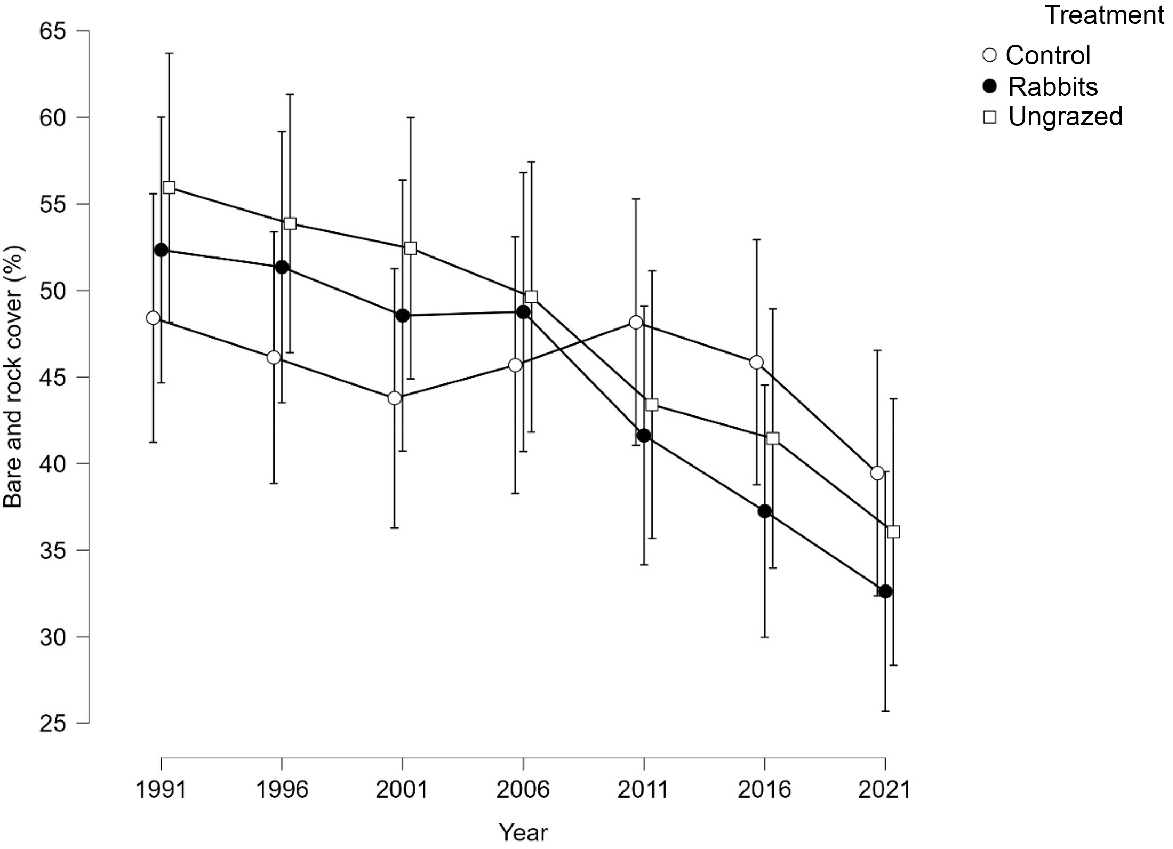
Site/treatment | Bare | Crypt | Shrub | Grass | Sedge | Tallhb | Shhb | |
---|---|---|---|---|---|---|---|---|
Bernacchi control | 13.1a | −15.8b | 6.6 | −10.1b | 0.0 | 2.4a | 0.8 | |
Bernacchi rabbits | −5.1b | 7.6a | 4.7 | −2.9ab | 0.2 | 0.9ab | 0.4 | |
Bernacchi ungrazed | −15.3b | 14.0a | 2.2 | 3.5a | −0.5 | 0.4b | −0.5 | |
Botsford control | −20.1 | 10.7 | 11.1b | 0.9 | 0.4 | 1.0 | 0.2a | |
Botsford rabbits | −17.8 | 4.6 | 39.7a | 0.0 | 0.4 | 0.4 | −5.3b | |
Botsford ungrazed | −10.6 | 4.6 | 28.0a | −4.3 | 0.6 | 1.1 | −2.1ab | |
DL control | −1.7 | 13.2ab | 0.1 | −6.0 | 1.3 | 0.0 | −1.9ab | |
DL rabbits | −11.3 | 20.1a | 14.7 | −2.1 | 0.9 | 1.3 | −5.0b | |
DL ungrazed | −11.9 | 9.8b | 0.3 | −1.6 | 0.7 | −0.5 | 1.6a | |
WDT control | −27.0b | 1.2 | 28.5b | −9.8 | 1.9ab | 0.0 | 5.5a | |
WDT rabbits | −44.6a | 0.7 | 50.6a | −4.9 | 2.5a | 0.2 | 7.1a | |
WDT ungrazed | −41.6ab | 2.0 | 60.9a | −7.0 | 0.6b | 0.3 | −2.2b |
Lowercase letters pertain to variation between treatments within cover type and site. If a letter is shared there is no difference (P > 0.05).
Bare, bare ground and rock; Crypt, cryptogams; Sedge, sedges and rushes; Tallhb, Tall herbs; Shhb, short herbs; DL, Double Lagoon, WDT, Wild Dog Tier.
Cryptogam cover
Treatment and site, but not replicate, were significant in the model for cover change between 1991 and 2021 (Table 1). By 2021 the ungrazed plots had much higher cryptogam cover than the other two treatments. The Bernacchi control decreased, whereas all other combinations of site and treatment increased (Table 2). Within Bernacchi, the control differed from the other treatments. At the Double Lagoon site, cryptogams increased more in the rabbit than in the ungrazed plots (Table 2).
Shrub cover
The vegetation at all sites except Bernacchi increased in shrub cover (Fig. 6). At Bernacchi, shrub cover decreased between 2001 and 2006, but was close to identical to 1991 by 2021 (Fig. 5). Wild Dog Tier shrub cover increased exponentially, whereas that at Botsford increased linearly (Fig. 5).
Variation in mean and 95% confidence limits for shrub cover by site and time for all treatments.
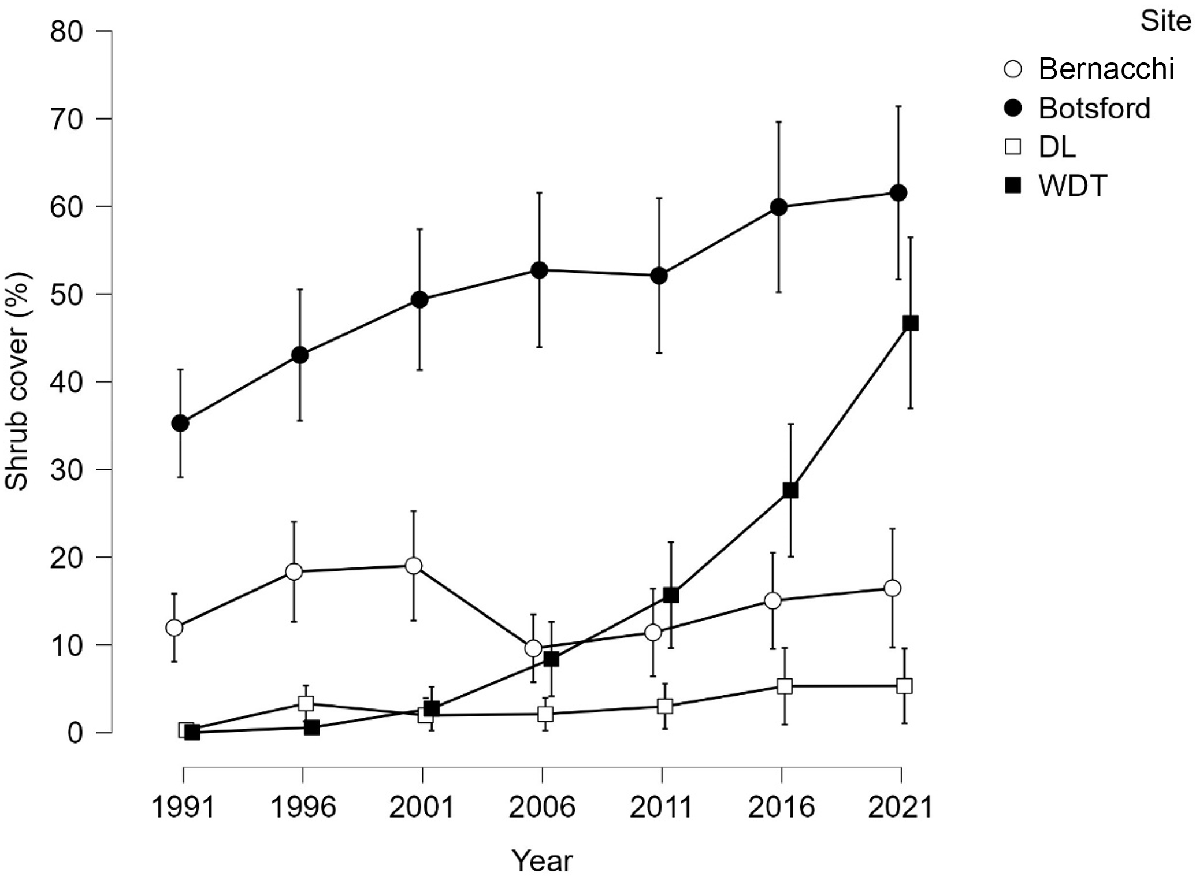
The model for change was highly significant for site, treatment and replicate (Table 2). The 1991 values were closely similar. By 2021 the control values were much less than the others (Fig. 7). Changes were positive for all combinations of site and treatment. At Botsford and Wild Dog Tier the increases were less in the controls than the other treatments (Table 2).
Grass cover
There were no significant components in the change model (Table 1). However, the controls decreased in cover, whereas the ungrazed plots increased in cover, at Bernacchi (Table 2).
Sedge and rush cover
There were site and replicate effects in the change model (Table 1). At Wild Dog Tier there was greater increase in the rabbit treatment than the ungrazed plots (Table 2).
Tall herb cover
The only significant component in the change model was replicate (Table 1). At Bernacchi, the fully grazed controls had a greater increase in tall herb cover than the ungrazed plots (Table 2).
Short herb cover
The change model for short herb cover was significant for all components (Table 1). At Botsford there was a decrease in the rabbit plots and a mild increase in the control plots (Table 2). At Double Lagoon there was an increase in the ungrazed and a decrease in the rabbit plots (Table 2). At Wild Dog Tier, there was a decrease in the ungrazed and an increase in the other two treatments (Table 2).
Overall patterns of change
Most of the peaks and troughs were in 1991 or 2021 (Table 3). However, values for these observation dates were very similar to those recorded for 2001 (Table 3). There were few peaks or troughs in 2006 or 2016 (Table 3).
1991 | 1996 | 2001 | 2006 | 2011 | 2016 | 2021 | ||
---|---|---|---|---|---|---|---|---|
Peaks lifeforms | 6 | 3 | 5 | 1 | 3 | 2 | 8 | |
Troughs lifeforms | 10 | 2 | 8 | 1 | 1 | 0 | 6 | |
Lifeform total | 16 | 5 | 13 | 2 | 4 | 2 | 14 |
Most of the significant differences between treatments in years pertained to cryptogams and short herbs (Table 4). These and tall herbs mostly had a significantly greater cover in the control plots than either of the rabbit-grazed or ungrazed plots (Table 4). There were few instances of greater or lesser cover between the ungrazed plots and the rabbit-grazed plots (Table 4). Shrubs had the opposite tendency to other lifeforms, with either ungrazed plots or rabbit-grazed plots having greater cover than the fully grazed controls (Table 4).
Cryptogams | Short herbs | Tall herbs | Shrubs | Total | ||
---|---|---|---|---|---|---|
Control > rabbit | 4 | 1 | 1 | 0 | 6 | |
Control < rabbit | 0 | 0 | 0 | 4 | 4 | |
Control > ungrazed | 6 | 10 | 1 | 0 | 17 | |
Control < ungrazed | 0 | 0 | 0 | 6 | 6 | |
Rabbit > ungrazed | 0 | 4 | 0 | 0 | 4 | |
Rabbit < ungrazed | 4 | 1 | 0 | 0 | 5 | |
Total | 14 | 16 | 2 | 10 | 42 |
There were no significant differences between treatments within any year for bare ground and rock, grass or sedges and rushes.
Discussion
Effects of grazing on recovery
Our results are consistent with the hypotheses that grazing slows invasion of bare areas, as in mainland alpine areas (Carr and Turner 1959a, 1959b; Wahren et al. 1994), and that sedges and rushes increase in cover for decades after disturbance, as in other places in Tasmania (Kirkpatrick et al. 2002).
In contrast, the lack of effect of rabbit grazing on cover was surprising. There was also unexpectedly high variation in patterns of cover change between our sites. The most unexpected finding was the lack of promotion of cover of both short and tall herbs by grazing exclusion. There was no indication in our data of the transition from shrubs to herbs and grasses that has been documented post-disturbance for mainland alpine areas (Carr and Turner 1959a, 1959b; Wimbush and Costin 1979a, 1979b, 1979c; Williams and Ashton 1988; Wahren et al. 1994, 2013; Scherrer and Pickering 2005) and Richea acerosa (Lindley) F. Muell. alpine heath in Tasmania (McPhail and Kirkpatrick 2016). It may be that this transition requires deep organic-rich alpine humus soils (dermosols), which are absent from all of our four sites because of their history of accelerated erosion (Fig. 8).
One of the two total exclosures at Wild Dog Tier in 1996 (above) and 2021 below (photos: Kerry Bridle).
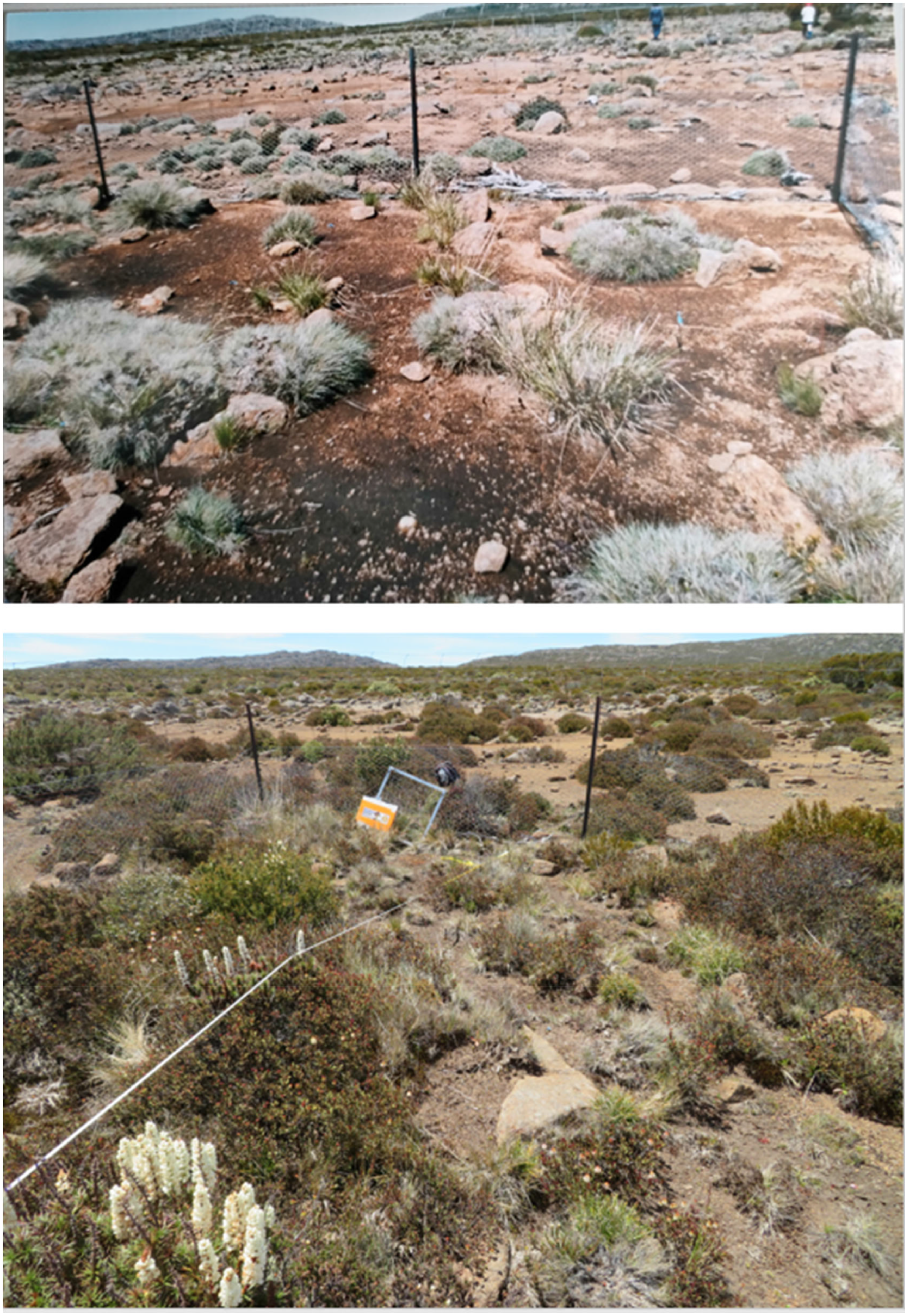
The analysis of change in bare ground and rock cover between 1991 and 2021 indicates that revegetation was faster in the rabbit and ungrazed treatments than in the controls. The controls at Bernacchi were the only ones to increase in bare ground, likely to have been caused by a loss of cryptogam cover between 1991 and 2021. At the other sites, bare ground was colonised by vegetation in the controls, but at a lesser rate than for the rabbit and ungrazed treatments. The revegetation advantage of animal population reduction may therefore be too small to suffer the animal conservation and welfare consequences of culling.
The most common treatment difference for lifeforms was a greater cover in the fully grazed control than in the ungrazed exclosure. This seemingly paradoxical difference may relate to heavier grazing of grasses and shrubs in the controls, where all species of herbivore had access. This grazing pressure could have reduced the effectiveness of grass tussocks and shrubs in suppressing shorter lifeforms.
The six instances of the rabbit exclosures having lesser cover than the fully grazed controls, which involved cryptogams, tall herbs and small herbs, may relate to the preference of rabbits for herbs (Leigh et al. 1987; Heaton et al. 2022). The rabbits may be reducing the cover of herbs in the exclosures to which they have access, as they did in the subalpine experiment of Leigh et al. (1987) in New South Wales, thereby favouring grasses, which therefore could have outcompeted the small herbs.
The six cases of ungrazed plots having greater cover than the controls all pertained to shrubs at Wild Dog Tier or Double Lagoon. The superior recovery of shrubs when freed from full grazing pressure was unexpected, although macropods are known to consume woody plants as part of their diet (Hazeldine and Kirkpatrick 2015), and mechanical breakage may occur from marsupial movement, with spreading shrubs particularly susceptible to such damage (Whinam et al. 1994).
The effects of initial state and environment on recovery
When the experiment was established, 2 years after removal of stock from the area, Wild Dog Tier and Double Lagoon had similar proportions of bare ground. Botsford and Bernacchi had similar amounts of bare ground at a lesser level. The revegetation trajectory at Wild Dog Tier has been rapid, while little change has occurred at Double Lagoon, possibly a function of shallower soils and poorer drainage. However, the lack of revegetation at Double Lagoon cannot be regarded as negative for conservation, as many rare alpine plants species that require bare ground and poor drainage are present at the site. The weak revegetation at Botsford contrasted with a lack of revegetation at Bernacchi. There may come a point at which the remaining bare ground is resistant to revegetation because of shallowness of soil and/or propensity to inundate during winter and spring. Botsford has deeper soils than Bernacchi. Grevillea australis R.Br., a spreading shrub that aids invasion of bare patches (Misiak 2007), was common at Botsford, but not Bernacchi.
The effects of climate
As an indicator of the importance of climate on the dynamics of cover, the extremely dry and warm monitoring year of 2001, which followed the extremely wet and cold monitoring year of 1996, had a strong influence on many components of the vegetation, particularly cryptogams, grasses, sedges, rushes and herbs, the lifeforms and species most immediately responsive to changing climatic conditions.
Variability in climatic conditions preceding the sampling times had no perceptible effect on the ongoing growth of shrub cover, but may have been responsible for the temporary increase in bare ground in the controls that occurred in the decade after 2001. This increase may have related to the lack of prolonged snow cover around the turn of the century (Kirkpatrick et al. 2017). Prolonged snow cover can cause high mortality among macropods (Kirkpatrick 1986).
Conclusions
Our expectation that grazing would slow the rate of reinvasion of bare ground proved to be correct for marsupials, but not rabbits, with the main effect of rabbits by themselves being on lifeform composition. Our expectations that herbs, especially tall ones, would be favoured by grazing exclusion were invalidated by our data, with the opposite effect apparent. Climatic variation between observation years had some clear effects on lifeforms and species characteristic of the ground stratum, which indicates that climate change is very likely to have effects in the longer term, although our data do not allow us to disentangle decades scale climate change effects from recovery from disturbance.
There is no indication in our data of a strong need to either restore vegetation cover or cull grazing animals. The absence of the combined effects of stock and fire has proven sufficient to ensure a natural restoration that may be close to complete at Bernacchi and Botsford and should only take a decade or two more at Wild Dog Tier. The 2019 fire near our experimental sites has dramatically reduced shrub cover but, in the absence of sheep, has resulted in little bare ground (Foulkes et al. 2021). It will be interesting to build on the early observations of vegetation change in recently burned stockless areas (Harding and Kirkpatrick 2018; Foulkes et al. 2021; Heaton et al. 2022) to compare with the ongoing succession in the controls in our experiment. It will be important to maintain monitoring of our plots as warming continues, fires become more frequent and deer populations potentially grow.
Declaration of funding
The authors thank the Australian Research Council for a grant to the senior author and PB McQuillan which covered some data collection and analysis (Australian alpine vegetation dynamics and environment-plant-invertebrate relationships 1998–2000), the predecessors of the Department of Natural Resources and Environment, Tasmania for paying for data collection by KB for several years, the University of Tasmania for paying JBK while he made his otherwise unfunded later contributions to the work and to KB for continuing the project for many years in the absence of funding.
Author contributions
Methodology, KB and JBK; formal analysis, JBK; investigation KB and JBK; data curation KB; writing – original draft preparation, JBK; writing – review and editing, JBK and KB; project administration, KB and JBK; funding acquisition, JBK and KB. Both authors have read and agreed to the published version of the manuscript.
Acknowledgements
The authors are grateful to Phil Cullen who constructed the plots and recorded the first set of data. They are grateful for assistance with data collection by Matt Appleby, Jared Parry and many others over the three decades. The authors also thank Agustina Barros for drafting some of the figures.
References
Bridle KL, Kirkpatrick JB (1998) Why do tall herbs rarely dominate Tasmanian alpine vegetation? Evidence from islands in the Ouse River system. Papers and Proceedings of the Royal Society of Tasmania 132, 9-14.
| Crossref | Google Scholar |
Bridle KL, Kirkpatrick JB (2001) Impacts of grazing by vertebrate herbivores on the flower stem production of tall alpine herbs, Eastern Central Plateau, Tasmania. Australian Journal of Botany 49, 459-470.
| Crossref | Google Scholar |
Bridle KL, Kirkpatrick JB, Cullen P, Shepherd RR (2001) Recovery in alpine heathl and grassland following burning and grazing, Eastern Central Plateau, Tasmania, Australia. Arctic, Antarctic and Alpine Research 33, 348-356.
| Crossref | Google Scholar |
Carr SGM, Turner JS (1959a) The ecology of the Bogong High Plains. I. The environmental factors and the grassland communities. Australian Journal of Botany 7, 12-33.
| Crossref | Google Scholar |
Carr SGM, Turner JS (1959b) The ecology of the Bogong High Plains. II. Fencing experiments in grassland C. Australian Journal of Botany 7, 34-63.
| Crossref | Google Scholar |
Cunningham CX, Perry GLW, Bowman DMJS, Forsyth DM, Driessen MM, Appleby M, Brook BW, Hocking G, Buettel JC, French BJ, Hamer R, Bryant SL, Taylor M, Gardiner R, Proft K, Scoleri VP, Chiu-Werner A, Travers T, Thompson L, Guy T, Johnson CN (2022) Dynamics and predicted distribution of an irrupting ‘sleeper’ population: fallow deer in Tasmania. Biological Invasions 24, 1131-1147.
| Crossref | Google Scholar |
Foulkes JA, Prior LD, Leonard SWJ, Bowman DMJS (2021) Demographic effects of severe fire in montane shrubland on Tasmania’s Central Plateau. Fire 4, 32.
| Crossref | Google Scholar |
Guy TR, Kirkpatrick JB, Cunningham CX, Berry TA, Dawkins KL, Driessen MM, Johnson CN (2024) Diet of fallow deer suggests potential for invasion of novel habitats in Tasmania. Wildlife Research 51, WR23124.
| Crossref | Google Scholar |
Harding MC, Kirkpatrick JB (2018) Short-term recovery of cushion plant communities after fire on the Central Plateau of Tasmania. Papers and Proceedings of the Royal Society of Tasmania 152, 9-16.
| Crossref | Google Scholar |
Hazeldine A, Kirkpatrick JB (2015) Practical and theoretical implications of a browsing cascade in Tasmanian forest and woodland. Australian Journal of Botany 63, 435-143.
| Crossref | Google Scholar |
Heaton DJ, McHenry MT, Kirkpatrick JB (2022) The fire and fodder reversal phenomenon: vertebrate herbivore activity in burned and unburned Tasmanian ecosystems. Fire 5, 111.
| Crossref | Google Scholar |
Iseli E, Chisholm C, Lenoir J, Haider S, Seipel T, Barros A, Hargreaves AL, Kardol P, Lembrechts JJ, McDougall K, Rashid I, Rumpf SB, Arévalo JR, Cavieres L, Daehler C, Dar PA, Endress B, Jakobs G, Jiménez A, Küffer C, Mihoc M, Milbau A, Morgan JW, Naylor BJ, Pauchard A, Ratier Backes A, Reshi ZA, Rew LJ, Righetti D, Shannon JM, Valencia G, Walsh N, Wright GT, Alexander JM (2023) Rapid upwards spread of non-native plants in mountains across continents. Nature Ecology & Evolution 7, 405-413.
| Crossref | Google Scholar | PubMed |
Kirkpatrick JB, Bridle KL (1999) Comparative effects of stock and wild vertebrate herbivore grazing on treeless subalpine vegetation, eastern Central Plateau, Tasmania. Australian Journal of Botany 47, 817-834.
| Crossref | Google Scholar |
Kirkpatrick JB, Bridle KL (2013) Natural and cultural histories of fire differ between Tasmanian and mainland Australian alpine vegetation. Australian Journal of Botany 61, 465-474.
| Crossref | Google Scholar |
Kirkpatrick JB, Bridle KL, Wild AS (2002) Succession after fire in alpine vegetation on Mount Wellington, Tasmania. Australian Journal of Botany 50, 145-154.
| Crossref | Google Scholar |
Kirkpatrick JB, Green K, Bridle KL, Venn SE (2014) Patterns of variation in Australian alpine soils and their relationships to parent material, vegetation formation, climate and topography. Catena 121, 186-194.
| Crossref | Google Scholar |
Kirkpatrick JB, Nunez M, Bridle KL, Parry J, Gibson N (2017) Causes and consequences of variation in snow incidence on the high mountains of Tasmania, 1983–2013. Australian Journal of Botany 65, 214-224.
| Crossref | Google Scholar |
Leigh JH, Wimbush DJ, Wood DH, Holgate MD, Slee AV, Stanger MG, Forrester RI (1987) Effects of rabbit grazing and fire on a sub-alpine environment. I. Herbaceous and shrubby vegetation. Australian Journal of Botany 35, 433-464.
| Crossref | Google Scholar |
Luo Y, Melillo J, Niu S, Beier C, Clark JS, Classen AET, Davidson E, Dukes JS, Evans RD, Field CB, Czimczik CI, Keller M, Kimball BA, Kueppers LM, Norby RJ, Pelini SL, Pendall E, Rastetter E, Six J, Smith M, Tjoelker MG, Torn M (2011) Coordinated approaches to quantify long-term ecosystem dynamics in response to global change. Global Change Biology 17, 843-854.
| Crossref | Google Scholar |
McPhail DC, Kirkpatrick JB (2016) Patch mosaic cyclic succession associated with the growth and senescence of an alpine shrub. Australian Journal of Botany 64, 111-119.
| Crossref | Google Scholar |
Nichols E, Kirkpatrick JB (2019) Patterns and causes of marsupial paths in subalpine Tasmania. Papers and Proceedings of the Royal Society of Tasmania 153, 53-60.
| Crossref | Google Scholar |
Pharo EJ, Kirkpatrick JB (1994) Vegetation of the alpine sand dunes at Lake Augusta, Tasmania. Australian Journal of Ecology 19, 319-327.
| Crossref | Google Scholar |
Scherrer P, Pickering CM (2005) Recovery of alpine vegetation from grazing and drought: data from long-term photoquadrats in Kosciuszko National Park, Australia. Arctic, Antarctic and Alpine Research 37, 574-584.
| Crossref | Google Scholar |
Verrall B, Pickering CM (2020) Alpine vegetation in the context of climate change: a global review of past research and future directions. Science of the Total Environment 748, 141344.
| Crossref | Google Scholar | PubMed |
Wahren C-H, Papst WA, Williams RJ (1994) Long-term vegetation change in relation to cattle grazing in sub-alpine grassland and heathland on the Bogong High-Plains: an analysis of vegetation records from 1945 to 1994. Australian Journal of Botany 42, 607-639.
| Crossref | Google Scholar |
Wahren C-H, Camac JS, Jarrad FC, Williams RJ, Papst WA, Hoffmann AA (2013) Experimental warming and long-term vegetation dynamics in an alpine heathland. Australian Journal of Botany 61, 36-51.
| Crossref | Google Scholar |
Whinam J, Cannell EJ, Kirkpatrick JB, Comfort M (1994) Studies on the potential impact of recreational horseriding on some alpine environments of the Central Plateau, Tasmania. Journal of Environmental Management 40, 103-117.
| Crossref | Google Scholar |
Williams RJ, Ashton DH (1988) Cyclical patterns of regeneration in subalpine heathland communities on the Bogong High-Plains, Victoria. Australian Journal of Botany 36, 605-619.
| Crossref | Google Scholar |
Williams RJ, Wahren CH (2005) Potential impacts of global change on vegetation in Australian alpine landscapes: Climate change, landuse, vegetation dynamics and biodiversity conservation. In ‘Global change and mountain regions: an overview of current knowledge’. (Eds UM Huber, HKM Bugmann, M Reasoner) pp. 401–408. (Springer: Dordrecht, Netherlands)
Wimbush DJ, Costin AB (1979a) Trends in vegetation at Kosciusko. 1. Grazing trials in the subalpine zone, 1957–1971. Australian Journal of Botany 27, 741-787.
| Crossref | Google Scholar |
Wimbush DJ, Costin AB (1979b) Trends in vegetation at Kosciusko. II. Subalpine range transects, 1959–1978. Australian Journal of Botany 27, 789-831.
| Crossref | Google Scholar |
Wimbush DJ, Costin AB (1979c) Trends in vegetation at Kosciusko. III. Alpine range transects, 1959–1978. Australian Journal of Botany 27, 833-871.
| Crossref | Google Scholar |