Seed dormancy alleviation by warm stratification progressively widens the germination window in Mediterranean climate Rutaceae
Michael Just

A School of Molecular and Life Science, Curtin University, Kent Street Bentley, WA 6102, Australia.
B EcoHealth Network, 1330 Beacon St, Suite 355a, Brookline, MA 02446, USA.
C Kings Park Science, Department of Biodiversity, Conservation and Attractions, 2 Kattidj Close, Kings Park, WA 6005, Australia.
D School of Biological Sciences, The University of Western Australia, 35 Stirling Highway, Crawley, WA 6009, Australia.
Australian Journal of Botany 71(2) 55-66 https://doi.org/10.1071/BT22076
Submitted: 15 July 2022 Accepted: 14 February 2023 Published: 8 March 2023
© 2023 The Author(s) (or their employer(s)). Published by CSIRO Publishing. This is an open access article distributed under the Creative Commons Attribution-NonCommercial-NoDerivatives 4.0 International License (CC BY-NC-ND)
Abstract
Context: In ecosystems where rainfall is episodic or highly seasonal, plant recruitment from a soil-stored seed bank occurs during periods of elevated soil moisture conducive to germination and seedling establishment. The release of seed dormancy in response to environmental conditions has significant consequences for the temperature window over which germination occurs, and as such the timing of germination can vary between years.
Aims: We aimed to understand in seeds of two species of Rutaceae, Diplolaena dampieri and Rhadinothamnus anceps, how dormancy loss and germination timing is influenced by warm stratification.
Methods: We tested the germination response to temperatures between 5 and 30°C following increasing durations (1–12 and 4–12 weeks) of warm stratification at 20, 25, or 30°C.
Key results: Warm stratification for 1–8 weeks at 30°C progressively alleviated seed dormancy in D. dampieri and R. anceps, increasing germination proportion from ~0.1 to ~0.5 in both species. Stratification duration was optimal at 30°C for between 4 and 8 weeks depending on species. Warm stratification was not affected by water stress down to −0.8 Mpa. Application of aerosol smoke did not significantly improve germination, and heat treatments had a negative effect on final germination proportion.
Conclusions: As dormancy was progressively alleviated, the range of temperatures that support germination increased for D. dampieri and decreased for R. anceps, allowing for confirmation of type 1 and type 2 non-deep physiological dormancy (PD), respectively.
Implications: Arising from this conclusion, we suggest that in Mediterranean climates, type 1 and 2 PD dictate risk-taking and risk-avoiding ecological strategies by shifting the thermal requirements for germination towards that characteristic of the early- or mid-germination season. Classification of non-deep PD may offer a structured approach to predict how temperature requirements shift during dormancy loss, which will provide insight into seed germination response to year-to-year variation in seasonal environmental conditions.
Keywords: ecology, Mediterranean, physiological dormancy, restoration, Rutaceae, seed germination, stratification, thermal performance.
Introduction
In over 80% of angiosperms, seedling recruitment from soil seed banks is mediated by the alleviation of seed dormancy as well as the climatic and edaphic conditions that support germination (Gulden et al. 2004; Ooi 2010; Salazar et al. 2011; Baskin and Baskin 2014). Germinating seeds and newly established seedlings are vulnerable to rapid changes in environmental conditions, and thus the conditions required for seed germination, seedling emergence, and seeding establishment are usually far more restrictive than the long-term requirements for persistence of mature plants (Hardegree et al. 2018). Seed dormancy is prevalent in ecosystems with seasonally dry periods, such as within Mediterranean climate regions (Merritt et al. 2007; Baskin and Baskin 2014; Turner et al. 2022), as it ensures germination occurs following the onset of seasonal conditions favourable for seedling emergence and development (Baskin and Baskin 2014). Additionally, seed populations may exhibit bet-hedging strategies through the modulation of germination via variable seed dormancy status, spreading germination both spatially and temporally (Simons 2011). Bet-hedging reduces year-to-year risk of seedling mortality resulting from stochastic events and thus increasing the probability of seedling establishment in the medium to long term (Cross et al. 2015). How seed dormancy status fluctuates in response to climate, and how these changes influence recruitment timing, is of critical importance to better understand vegetation ecology and variability in recruitment strategy.
Physiological dormancy (PD) is the most common class of seed dormancy (Finch-Savage and Leubner-Metzger 2006). Seeds in the soil seed bank experience climate-driven seasonal variations in temperature and moisture and regulate their dormancy status in response to the varying environmental conditions to ensure germination occurs in periods when water is not limiting for seedling establishment (Merritt et al. 2007; Baskin and Baskin 2014). For seeds with PD, periods of warm to hot, dry conditions, which in many regions with Mediterranean climate typically occur over summer between the cooler and more mesic winter germination seasons (Merritt et al. 2007), can facilitate dormancy alleviation through the process of afterripening (Turner et al. 2009; Baskin and Baskin 2020). Seed dormancy alleviation can also occur under moist soil conditions (termed stratification) that occur between, in the lead up to, and during, the germination season as temperatures fluctuate in hydrated soils (Merritt et al. 2007). Stratification between temperatures of 0–10°C (cold stratification) typically alleviates dormancy in summer annuals (i.e. species germinating in early summer), and stratification at temperatures above 15°C (warm stratification) is generally associated with seed dormancy alleviation in winter annuals (i.e. species germinating in winter) (Baskin and Baskin 2014). While the conditions that alleviate physiological dormancy are generally understood, the specific regimes of temperature and moisture required for dormancy alleviation, and the effect that these conditions have on subsequent germination, remain unknown for most species.
During the alleviation of non-deep PD, there is a shift in the base or ceiling temperatures that will support germination (i.e. the lowest and highest temperatures at which seeds will germinate), often referred to as a widening of the germination window (Finch-Savage and Leubner-Metzger 2006). The direction in which the base or ceiling temperature shifts determines the type of non-deep PD present, according to six types of PD split across two sublevels that have been currently classified (Baskin and Baskin 2014; Soltani et al. 2017; Baskin and Baskin 2021). How the germination window shifts in response to dormancy alleviation is unknown in most species with PD (Walck et al. 2011), although it is likely that conditions leading up to the germination season facilitate dormancy alleviation and drive thermal requirements for germination to temperatures characteristic of the germination window. For example, in type 1 non-deep PD there is an increase in the ceiling temperature that will support germination (Baskin and Baskin 1989; Soltani et al. 2017). In environments with water availability restricted to the winter months, type 1 non-deep PD may represent a risk-taking strategy, pushing germination requirements towards warmer temperatures characteristics of the early germination window and providing an early establishment advantage, but an increased risk of mortality due to desiccation. Comparatively, type 2 non-deep PD, which is characterised by a lowering of the base temperature required for germination (Steadman and Pritchard 2004), may represent a risk-avoidance strategy, limiting seed germination to the cooler temperatures more common in the middle of the germination window when there is greater certainty of soil water stores sufficient for seedling establishment. Classification of non-deep PD type may have significant potential to predict how species recruitment will respond to year-to-year variation in the environmental conditions coinciding with the onset of the growing season.
In south-western Australia, a biodiverse region with a Mediterranean climate harbouring many species with PD, most species tend to produce seeds that germinate in late autumn through to early winter during a period where soil moisture has begun increasing but soil temperatures are still relatively warm (Turner et al. 2006; Merritt et al. 2007). Seeds of many species within this region such as Acanthocarpus preissii (Turner et al. 2006), Lomandra preissii (Merritt et al. 2007), Persoonia longifolia (Norman and Koch 2006; Chia et al. 2016), Byblis gigantea (Cross et al. 2013), and species of Hibbertia (Hidayati et al. 2012), produce seeds with PD or morphophysiological dormancy (MPD) (Hibbertia spp.) with dormancy fully or partially alleviated by warm stratification. Nevertheless, no studies to date have examined the effect of stratification on the shift in temperatures able to support germination during dormancy loss in south-west Australian species, having instead reported the effect on germination at single constant (Cross et al. 2013) or alternating incubation temperatures (Turner et al. 2006; Merritt et al. 2007; Chia et al. 2016). Also, there are few data on how species differ in their stratification duration and temperature requirements, or what the minimum threshold for hydration is before stratification ceases to occur; all of which have significant implications for the timing of dormancy loss and, therefore, the onset of the germination window and germination.
The period over which environmental conditions are conducive to seed germination and seedling establishment can vary between years (Saatkamp et al. 2019). While climatically mediated shifts in germination timing from year to year are studied infrequently in species from south-western Australia, germination timing of winter emerging species might be expected to follow similar patterns to those from regions with similarly seasonal climate. For example, Sonoran Desert annuals are known to vary germination timing in response to water availability and temperature within and between seasons, demonstrating bet-hedging strategies that spread germination events temporally to increase overall survival (Gremer et al. 2016; Huang et al. 2016; Ten Brink et al. 2020). Investigation of the effect of warm stratification on dormancy loss and subsequent germination requirements may yield insights into how south-west Australian species vary germination timing in response to varying environmental conditions between years. Further, determination of stratification optima (optimum temperature and duration of stratification) may also identify methodology able to alleviate dormancy of species that have historically proven difficult-to-germinate for restoration purposes.
One group of difficult-to-germinate species also important in restoration species mixes in Australia is the Rutaceae. Efforts to understand the conditions required for seed dormancy alleviation and seed germination in species of south-west Australian Rutaceae have yet to be successful (Dixon et al. 1995; Roche et al. 1997; Rokich et al. 2002; Norman et al. 2006; Merritt et al. 2007; Maher et al. 2008). Many Rutaceae produce PD seeds (Commander et al. 2009; Martyn et al. 2009; Baskin and Baskin 2014; Mackenzie et al. 2016; Collette and Ooi 2017; Collette and Ooi 2020), although the depth and type of PD remains unknown or assumed for many Australian Rutaceae. In eastern Australia, some species of Rutaceae germinate readily only in the presence of smoke and/or following heat shock (Mackenzie et al. 2016; Collette and Ooi 2017). Field observations of Rutaceae emerging following wildfire and smoke application in situ in Western Australian ecosystems suggest heat and/or smoke also play a significant role in seed germination (Roche et al. 1997; Norman et al. 2006). However, the lack of success reported by previous studies employing these treatments on the Rutaceae (Dixon et al. 1995; Roche et al. 1997) suggests a requirement for warm stratification and/or afterripening to alleviate physiological dormancy and increase seed sensitivity to smoke. Identification of the hydrothermal thresholds required for dormancy alleviation will also provide insights into how seedling recruitment is mediated by environmental conditions of temperature and moisture, and how best to propagate and manage species.
To implement informed species and habitat management plans, and to time seeding in variable environments, a clear understanding of conditions that mediate dormancy loss and germination is required. Detailed knowledge of species-specific requirements of dormancy loss can provide methodology able to alleviate dormancy and facilitate seed germination for the purposes of land management and restoration. Here we sought to investigate the effect of temperature, duration, and water stress during warm stratification on the germination window of two previously unstudied south-west Australian Rutaceae, Diplolaena dampieri and Rhadinothamnus anceps. The overarching hypothesis was that dormancy would be progressively alleviated over time in conditions suitable for warm stratification, thereby facilitating a widening of the germination window. It was also expected that stratification at 30°C would become less effective under increasing water stress, and that stratification below 30°C would be less effective than stratification at 30°C. Specifically, we sought to determine: (1) the class and type of seed dormancy present in study species; (2) investigate the interaction between water stress and warm stratification on seed dormancy alleviation and germination; (3) determine the effect of warm stratification temperature and duration on the temperatures over which seed germination occurs; and (4) interpret data to inform species management and restoration practice.
Materials and methods
Seed source
South-western Australia experiences a Mediterranean climate with cool wet winters and hot dry summers (Fig. 1), with most native species dispersing seed between November and February (Merritt et al. 2007). Mature seeds of D. dampieri and R. anceps were collected in late spring (November of 2020) from wild populations at various sites across south-west Western Australia. Seeds were pooled before being cleaned using a vacuum aspirator (SELECTA BV Gravity Seed Separator, The Netherlands) to separate seeds from loose plant debris, before storage under controlled conditions (15°C and 15% relative humidity) at the Western Australia Seed Centre, Kings Park and Botanic Garden, Perth, prior to experimental use.
![]() |
Seed characteristics and viability
Seed fill was determined for four replicate samples of 100 seeds using an X-ray (Autofocus X-ray cabinet, Faxitron, Tucson, USA) prior to further processing (initial seed fill) where damaged or unfilled seeds were removed (experimental seed fill). Seed weight (mg) was determined for four replicates of 25 filled seeds and the total weight divided by 25 to determine the mean individual seed weight.
To assist in classifying seed dormancy class, embryo morphology and water uptake were documented for both species using a Leica 205C camera with analysis in Leica Application Suite X (Leica Camera, Wetzlar, Germany). Sixty seeds were dissected longitudinally and embryos measured (μm) before defining embryo morphology according to Martin (1946). To assess whether seeds imbibe when exposed to water, a rudimentary assessment of testa permeability was conducted. Three replicates of 25 seeds were placed into Petri dishes lined with 84 mm germination paper (Advantec, Dublin, CA, USA) irrigated with deionised (DI) water. Each replicate was weighed prior to imbibition and again after 24 h of moist incubation, after being surface-dried using paper towels. Percentage water uptake was determined gravimetrically, based on the fresh weight of non-imbibed seeds, with the percentage increase in seed mass calculated as:

where W1 and Wd are the mass of imbibed and dry seeds, respectively (sensu Turner et al. 2009).
Response to warm stratification following the application of fire cues
To determine the response of study species to fire cues known to promote germination of related species (Mackenzie et al. 2016), unimbibed seeds were treated with a 10 min application of aerosol smoke, heat or a combination of both smoke and heat. Four replicates of 25 seeds were used for all treatments. Heat treated seeds were exposed to dry heat in foil cups placed in a pre-heated electronic oven at 90°C for 10 min (Mackenzie et al. 2016). Seeds treated with smoke were placed into a 90 L plastic propagation tent before pumping in smoke generated by combusting 100 g of oat hay in a stainless-steel bee smoker for 10 min. In heat and smoke treatments, seeds were first exposed to heat before treatment with aerosol smoke. Heat, smoke, or heat and smoke treated seeds, as well as untreated seeds used as controls, were surface sterilised in a 4% (w/v) sodium hypochlorite (NaOCI) solution supplemented with several drops of Tween 80 (Sigma-Aldrich) for 30 min under alternating vacuum (−70 kPa) (Turner et al. 2018). Seeds were then rinsed three times in sterile deionised water (DI) before plating onto 0.7% (w/v) water agar and incubation at 10°C, 15°C, 20°C or 30°C under a 12-h photoperiod. To determine the effect of duration of warm stratification on response to fire cues and germination temperature four replicates of 25 seeds were first treated with either nothing (controls), heat, smoke, or smoke and heat as previously described, and were then incubated at 30°C for 4, 8 or 12 weeks before being moved to either 10°C, 15°C or 20°C. Control seeds were maintained at 10°C, 15°C, 20°C or 30°C for the duration of the experiment. Germination was defined as emergence of the radicle to a length of >2 mm, and germination was scored once after 4 weeks to determine the proportion of primary dormancy as per Baskin and Baskin (2004), and again after 4 weeks incubation at the transferral temperature.
Identification of the optimal germination temperature following different stratification regimes
R. anceps was selected as a model species to further investigate the interactive effects of stratification and incubation temperatures on the germination response of seeds. Four replicates of 25 seeds were used for all treatments and were plated onto 0.7% (w/v) water agar under a 12-h photoperiod. Warm stratification has been defined as temperatures greater than 15°C, and an application duration of 4, 8, or 12 weeks can be used to determine the depth of physiological dormancy (Baskin and Baskin 2014). To determine if temperatures between 20°C and 30°C alleviate physiological dormancy untreated seeds were stratified at either 30°C, 25°C or 20°C for 1, 2, 4, 8 and 12 weeks before movement to either 5°C, 10°C, 15°C, 20°C or 25°C to complete the incubation process. As the germination window in south-west Australia typically proceeds with warmer drier conditions giving way to cooler, wetter conditions as the seasons move from summer through to winter (Merritt et al. 2007) seeds were only exposed to downwards shifts in temperature. Thus, seeds stratified at 30°C were moved to 5°C, 10°C, 15°C, 20°C and 25°C, those stratified at 25°C were moved to 5°C, 10°C, 15°C, 20°C and those stratified at 20°C were moved to 5°C, 10°C and 15°C. Seeds acting as controls were surface sterilised and plated as previously described before incubation at 5°C, 10°C, 15°C, 20°C, 25°C and 30°C for the entire duration of the experiment. Germination was defined as previously described and scored every 2 days for 60 days.
Interaction of warm stratification and water stress
To examine the interaction of warm stratification and water stress on seed germination, R. anceps seeds were surface sterilised as previously described. Following sterilisation seeds were dried overnight in a laminar flow cabinet before being placed onto germination papers in 90 mm Petri dishes irrigated with differing concentrations of polyethylene glycol 8000 (PEG) solution (10 mm per Petri dish) following Michel et al. (1983) to generate a range of water stresses (0 MPa, −0.1 MPa, −0.2 MPa, −0.4 MPa and −0.8 MPa). For each treatment, four replicates of 25 seeds were used. All plates were incubated at constant 30°C for 4 weeks, determined as the optimum stratification treatment for promoting dormancy alleviation from previous experiments. Following stratification, all germinated seeds were removed, with the remaining ungerminated seeds rinsed in sterile DI water and transferred to fresh germination papers irrigated with sterile DI water. Seeds were then incubated at 20°C, determined to be the optimum temperature for supporting germination in previous experiments. Germination was defined as previously described and scored every 2 days until no new germination had been observed for 25 days.
Statistical analyses
All analyses were conducted in the R statistical environment (R Core Team 2013). We used binomial generalised linear modelling (GLM) to assess the influence of three germination temperatures (10°C, 15°C and 20°C), four stratification durations (0, 4, 8, 12 weeks) and treatment applications of fire cues (heat and aerosol smoke) on germination success. The full model with interactions was fitted, followed by a stepwise reduction to simplify the final model. The final model was fitted with a logit-link function and a binomial error structure and analysed to determine the main effects of germination temperature, stratification duration and treatment on germination success following 4 weeks incubation at the final temperature. The plot_models function from the ‘sjPlot’ package (Lüdecke 2018) were used to visually represent model coefficients from the GLMs.
Germination modelling
Germination response over time for each temperature was assessed using curvilinear log-logistic germination models (Ritz et al. 2013). The ‘drc’ package (Ritz et al. 2016) was used to fit a three-parameter log-logistic function to germination data:
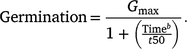
Above, Gmax is the upper limit for germination, with the lower limit for germination rate assumed to be 0, t50 is the time required for germination to reach 50% from Gmax, and b is the slope of the germination function at t50. A full model was created for the number of germinated seeds divided by the number of seeds incubated for all temperature and stratification regimes. The anova function was used to assess the explanatory power of stratification duration, germination temperature and water stress as factors influencing t50 and Gmax, versus a model without stratification duration, germination temperature and water stress. Due to poor germination proportion following stratification at temperatures below 30°C (<0.25), 20 and 25°C stratification regimes were removed from the analysis (Supplementary Fig. S1).
Thermal performance
Model fits for final germination proportion for thermal response data were estimated using Beta, Yan and Hunt and Broken-Stick thermal performance functions described by Asbury and Angilletta (2010), Yan and Hunt (1999) and Yeager and Ultsch (1989) respectively. The AIC function was used to assess the explanatory power of each model and the final model selected accordingly based upon the lowest Akaike information criterion (AIC) value (Table S1). The final model was that described by Yan and Hunt (1999):

where Gmax is the maximum germination proportion at any temperature (T), Topt is the optimum temperature for germination at the peak of the performance function, Tmax is the limit of thermal tolerance and Rmax is the asymptotic maximum germination rate at Topt.
Results
Seed characteristics and seed fill
Study species had an individual seed weight between 1.8 and 10.3 mg with seed fill >70% upon collection (Table 1). Seeds of both species possessed fully developed, linear embryos (Martin 1946) and significantly increased in mass by up to 26% after 24 h of imbibition indicating water movement into seeds (Table 1).
![]() |
Response to warm stratification following the application of fire cues
Untreated control seeds incubated at 10, 15 or 20°C germinated to a maximum proportion of 0.22 for D. dampieri and 0.12 for R. anceps. Both species showed a significant increase in germination response with increasing duration of warm stratification up to four to 8 weeks (D. dampieri; log-odds ratio = 12.89, probability ratio = 0.93, Z = 11.07, P < 0.001 and R. anceps; log-odds ratio = 9.25, probability ratio = 0.90, Z = 11.42, P < 0.001), with a three and four-fold increase in germination of D. dampieri and R. anceps respectively (Figs 2, 3). The main effect of germination temperature was also significant for both species, with decreasing germination temperature positively correlated with germination success for D. dampieri (log-odds ratio = 1.15, probability ratio = 0.54, Z = 9.44, P < 0.001) and negatively correlated with germination success for R. anceps (log-odds ratio = 0.89, probability ratio = 0.47, Z = −8.99, P < 0.001). Fire cues had no significant positive effect on seed germination for either species (Fig. 3), although heat pre-treatments had a negative effect on the outcome of germination for both species (D. dampieri; log-odds ratio = 0.52, probability ratio = 0.34, Z = −4.13, P < 0.001; R. anceps; log-odds ratio = 0.42, probability ratio = 0.73, Z = −2.13, P = 0.033).
Optimal germination temperature following variable stratification duration
Compared with unstratified control seeds, germination proportion in R. anceps seeds warm-stratified for any duration was 2- to 5-fold higher and occurred over a wider range of temperatures (Fig. 4). Increasing durations of warm stratification widened the germination window in R. anceps, allowing seeds to germinate at either 5 or 10°C, which was not seen for seeds in control groups (Fig. 4). Maximum germination was achieved at 25°C in control groups and at 25, 25, 20, 20 and 20°C after 1, 2, 4, 8 and 12 weeks of warm stratification, respectively. Germination rate increased with increasing duration of stratification and was fastest at 20°C for all treatments excluding controls, where germination speed was similarly fastest at 25 and 30°C (Fig. 4). Models applied to seeds stratified at 20–25°C were unable to converge due to poor germination (<0.25) and have been excluded from analysis (Fig. S1).
Thermal performance
The Yan and Hunt (1999) model was fitted across all temperatures tested following stratification at 30°C (Fig. 5). When fitted to maximum germination proportion the optimum temperature for germination (Topt) was 25.5 ± 1.7°C in control groups and decreased significantly (P < 0.05 in all cases) with increasing duration of stratification (Table 2) between 2 and 8 weeks, where the minimum optimum temperature for germination was estimated as 18.6 ± 1.4°C (Table 2). When compared to control seeds (0 weeks of stratification), the maximum germination proportion (Rmax) increased significantly following 1 (P = 0.048, t value = −2.02), 2 (P < 0.001, t value = −6.5158) and 4 (P < 0.001, t value = −6.5878) weeks of stratification, with maximum germination (0.48 ± 0.03) achieved following 2 and 4 weeks (Table 2). For all stratification durations the Tmax for germination was within the range 30.8–31.4°C (Table 2).
![]() |
Interaction of warm stratification and water stress
Increasing water stress during warm stratification had no effect on maximum germination proportion or time until 50% of final germination (t50) at 20°C (P = 0.81, F = 0.63). The maximum germination proportion achieved (±s.e.) was between 0.39 ± 0.03 and 0.44 ± 0.03 and the t50 between 4.3 ± 0.5 and 4.9 ± 0.4 days (Fig. 6).
Discussion
Warm stratification was found to be an effective treatment for promoting germination in two Rutaceae species growing in a Mediterranean climate, which germinated after several weeks of exposure to warm moist conditions followed by incubation at cooler temperatures. These results add to reports of warm stratification alleviating dormancy in previously difficult-to-germinate Mediterranean-type climate taxa and enhance our capacity to propagate these species under controlled conditions for use in restoration, horticulture, and amenity planting (Turner et al. 2006; Hidayati et al. 2012; Chia et al. 2016). The thermal thresholds for germination of both D. dampieri and R. anceps were seen to widen following warm stratification, while the optimum temperature for germination of R. anceps decreased significantly as dormancy was alleviated. The application of smoke, heat, or increasing water stress during stratification (to −0.8 MPa) had no effect on either dormancy alleviation by warm stratification or final germination proportion. A lack of smoke response for fresh seed of the two study species does not neatly align with previous records for Australian Rutaceae. Significant germination responses have been noted following the application of heat, smoke or karrikinolide (KAR1) (Commander et al. 2009; Mackenzie et al. 2016), or the passage of fire (Roche et al. 1997; Norman et al. 2006), and soil stored seeds may respond differently than those studied here following the effects of seasonal afterripening and stratification (Mackenzie et al. 2021). It does however demonstrate the inconsistency of smoke response between species of Rutaceae and suggests that dormancy alleviation may reduce the requirement for smoke stimulated germination in the inter-fire period. Prior to this study it was unknown how seeds of D. dampieri or R. anceps alter their requirements for germination during dormancy alleviation and the results presented here provide potential evidence for bet-hedging traits expressed in the modulation of germination of these south-west Australian species.
Fresh seeds of both study species were mainly dormant, with 0–0.14 germination proportion observed after 4 weeks of incubation in alternating light at 10°C, 15°C or 20°C. Developed linear embryos and the unrestricted uptake of water determines seeds of both species possess PD, and the positive rapid response to warm stratification indicates non-deep PD (Baskin and Baskin 2014). Notably, the shift in temperatures suitable for germination differed in these two species during dormancy alleviation. Following warm stratification, seeds of D. dampieri germinated under warmer conditions, which is typical of seeds with type 1 non-deep PD. In contrast, seeds of R. anceps exhibited a decrease in temperatures able to support germination, which is often seen in seeds with type 2 non-deep PD (Soltani et al. 2017). Seeds released into the soil seed bank in south-western Australia experience a relatively brief period of warm stratification followed by a decrease in soil temperature, prior to significant increases in soil moisture characteristic of the germination window during the cooler winter months (Merritt et al. 2007). An increase in the ceiling temperature for germination during this period of warm stratification, as seen in D. dampieri, suggests seeds are seeking to germinate while temperatures remain relatively warm during mid to late autumn, rather than delay germination until the onset of cold winter conditions. Conversely, a decrease in the temperature threshold required for germination, like that of R. anceps, indicates that seeds are delaying germination until soil temperatures decrease to a level typical of the winter germination season.
The contrasting types of non-deep PD that occur in D. dampieri and R. anceps might be alternative recruitment strategies responding to variation in the onset of the germination window. Seeds in the soil seed bank within south-west Australia are exposed to intermittent increases and decreases in moisture during autumn (specifically from April to May), and consistently high moisture conditions during winter and the beginning of spring (June to September) (Turner et al. 2006; Merritt et al. 2007). By germinating under the relatively warmer temperatures characteristic of the early germination window in autumn, D. dampieri may profit from a longer growing period, possibly yielding establishment and reproductive advantages (Donohue et al. 2010; Ten Brink et al. 2020; Miller et al. 2021) and a competitive benefit over later emerging individuals (Verdú and Traveset 2005; Ten Brink et al. 2020). However, the early stages of the germination window have a greater risk of mortality due to drought (Mercer et al. 2011; Donohue 2014; Thomson et al. 2017; Ten Brink et al. 2020) as the soil moisture conditions driving dormancy release and early germination may not be consistently present post-germination to support effective seedling establishment (Harrison et al. 2018). In contrast, by germinating in cooler temperatures more characteristic of later in the season, seedlings of R. anceps are more likely to avoid early season drought and establish in more predictable and consistent rainfall and soil moisture conditions.
In the context of Mediterranean south-west Australia, type 1 non-deep PD (D. dampieri) appears to be a ‘high-risk high-reward’ strategy while type 2 non-deep PD (R. anceps) is a ‘risk-avoidance’ strategy, though the likely trade-off is elevated seedling competition (Duncan et al. 2019; Miller et al. 2021). How species possessing different types of non-deep PD respond to variable environmental conditions is an area of seed ecology that requires further research, particularly under current predictions for climate change. Identification of non-deep dormancy type offers a structured approach to species classification that will aid management and restoration practices and provide insight into the sensitivity of species to an altered germination window under a shifting climate.
Indeed, how species possessing these alternate germination strategies will respond to changes in temperature and moisture under current climate predictions is an area that requires further investigation. While it is generally accepted that altered seasonal cycles of air temperature will change, the relationship between seasons is expected to stay the same, i.e. summer will be warmer than winter (Hajek and Knapp 2022) and therefore warm stratification will still occur if sufficient rainfall is present. Comparatively, seasonal patterns in water availability are expected to change in regionally specific ways that require nuanced assessment of ecosystem vulnerability and ecological consequence (Hajek and Knapp 2022). Predictions for future (2030–2059) mean surface soil moisture across south-western Australia suggest a decrease in volumetric water content (m3 water m−3 soil) when compared to historical records (1970–1999) (Andrys et al. 2017). Currently the seed moisture content needed to facilitate stratification in the lead up to the germination window is unknown. The mean base water potential required for germination of south-west Australian species has been estimated as 0.62 MPa (Dalziell et al. 2022), and while it was expected that dormancy loss by warm stratification would decrease as water stress approached this upper limit, our results show that warm stratification was unaffected by water stress down to −0.8 MPa. This result suggests that the base water potential required for stratification of south-west Australian species is lower than that required for germination, which makes intuitive sense considering autumn is drier than winter. Accurate estimates of dormancy alleviation in the lead up to the germination window will be required to determine how some PD species will respond to predicted reductions in soil moisture. A better understanding of the hydrothermal thresholds required for stratification in south-west Australian species is therefore a priority to ensure informed decisions are made regarding vegetation management and restoration.
Conclusion
These results have significant implications in seed ecology and practices seeking to propagate physiologically dormant species from seed. Fresh seed of both D. dampieri and R. anceps had optimum germination temperatures, which were significantly different before and after relatively short periods of warm stratification. Identification of differing optimum stratification requirements between sympatric species suggests the presence of dormancy alleviation strategies adapted to short to long periods of elevated soil moisture prior to the germination window. Wetting and drying cycles have also been shown to alleviate dormancy to proportions greater than stratification alone (Hidayati et al. 2012; Chia et al. 2016; Lewandrowski et al. 2018). In combination with stratification mediated shifts of the thermal requirements for germination, these processes highlight the ability of seeds to sense their environment and regulate germination based on past climatic cues. It is likely that the difficulty of germination associated with our study species and other members of the Rutaceae in south-west Australia is due to the requirement for species-specific cues of temperature and moisture and suggests that no single treatment application is likely to be optimal across species. The moderate (~0.5) germination proportions achieved here suggest bet-hedging strategies are being employed to spread germination events between years, and that germination proportions may be increased by further investigation into regional climate signals that occur across years. To understand the dormancy and germination requirements of Rutaceae and other physiologically dormant species several stratification durations and temperatures need to be tested to ensure protocols are optimised and seed ecological requirements are more fully captured. Implications of these findings are three-fold; firstly, warm stratification should be applied to a greater number of taxa in south-western Australia as it is likely to increase the proportion of species able to be germinated readily. Secondly, identification of the type of non-deep physiological dormancy present in Australian taxa offers additional resolution in efforts to predict germination and seedling recruitment timing and response to environmental change. Finally, understanding the hydrothermal thresholds required for dormancy alleviation by warm stratification, including identifying the lowest effective water potential for stratification, should be pursued so as to contribute to understanding the effects of climate change on germination timing in south-west WA.
Supplementary material
Supplementary material is available online.
Data availability
The data that support this study will be shared upon reasonable request to the corresponding author.
Conflicts of interest
All authors acknowledge that, regarding this manuscript, there are no conflicts of interest to declare.
Declaration of funding
This research was funded under the Australian Research Council Industrial Transformation Training Centre for Mine Site Restoration (ICI150100041). This work was supported by Australian Research Council [grant number IC150100041].
Acknowledgments
The authors acknowledge the Wadjuk people of the Noongar nation, traditional owners of the land on which this work was undertaken and pay our respects to Elders past, present and emerging. The authors are grateful to Keith Smith (Formosa Flora) and Neroli Forster (Seed Shed) for their efforts in securing the seed required for this study.
References
Andrys J, Kala J, Lyons TJ (2017) Regional climate projections of mean and extreme climate for the southwest of Western Australia (1970–1999 compared to 2030–2059). Climate Dynamics 48, 1723–1747.| Regional climate projections of mean and extreme climate for the southwest of Western Australia (1970–1999 compared to 2030–2059).Crossref | GoogleScholarGoogle Scholar |
Asbury DA, Angilletta MJ (2010) Thermodynamic effects on the evolution of performance curves. The American Naturalist 176, E40–E49.
| Thermodynamic effects on the evolution of performance curves.Crossref | GoogleScholarGoogle Scholar |
Baskin JM, Baskin CC (1989) Germination responses of buried seeds of Capsella bursa-pastoris exposed to seasonal temperature changes. Weed Research 29, 205–212.
| Germination responses of buried seeds of Capsella bursa-pastoris exposed to seasonal temperature changes.Crossref | GoogleScholarGoogle Scholar |
Baskin JM, Baskin CC (2004) A classification system for seed dormancy. Seed Science Research 14, 1–16.
| A classification system for seed dormancy.Crossref | GoogleScholarGoogle Scholar |
Baskin CC, Baskin JM (2014) ‘Seeds: ecology, biogeography, and, evolution of dormancy and germination.’ (Elsevier)
Baskin CC, Baskin JM (2020) Breaking seed dormancy during dry storage: a useful tool or major problem for successful restoration via direct seeding? Plants 9, 636
| Breaking seed dormancy during dry storage: a useful tool or major problem for successful restoration via direct seeding?Crossref | GoogleScholarGoogle Scholar |
Baskin JM, Baskin CC (2021) The great diversity in kinds of seed dormancy: a revision of the Nikolaeva–Baskin classification system for primary seed dormancy. Seed Science Research 31, 1–19.
| The great diversity in kinds of seed dormancy: a revision of the Nikolaeva–Baskin classification system for primary seed dormancy.Crossref | GoogleScholarGoogle Scholar |
Chia KA, Sadler R, Turner SR, Baskin CC (2016) Identification of the seasonal conditions required for dormancy break of Persoonia longifolia (Proteaceae), a species with a woody indehiscent endocarp. Annals of Botany 118, 331–346.
| Identification of the seasonal conditions required for dormancy break of Persoonia longifolia (Proteaceae), a species with a woody indehiscent endocarp.Crossref | GoogleScholarGoogle Scholar |
Collette JC, Ooi MKJ (2017) Germination ecology of the endangered species Asterolasia buxifolia (Rutaceae): smoke response depends on season and light. Australian Journal of Botany 65, 283–291.
| Germination ecology of the endangered species Asterolasia buxifolia (Rutaceae): smoke response depends on season and light.Crossref | GoogleScholarGoogle Scholar |
Collette JC, Ooi MKJ (2020) Evidence for physiological seed dormancy cycling in the woody shrub Asterolasia buxifolia and its ecological significance in fire-prone systems. Plant Biology 22, 745–749.
| Evidence for physiological seed dormancy cycling in the woody shrub Asterolasia buxifolia and its ecological significance in fire-prone systems.Crossref | GoogleScholarGoogle Scholar |
Commander LE, Merritt DJ, Rokich DP, Dixon KW (2009) Seed biology of Australian arid zone species: germination of 18 species used for rehabilitation. Journal of Arid Environments 73, 617–625.
Cross AT, Merritt DJ, Turner SR, Dixon KW (2013) Seed germination of the carnivorous plant Byblis gigantea (Byblidaceae) is cued by warm stratification and karrikinolide. Botanical Journal of the Linnean Society 173, 143–152.
| Seed germination of the carnivorous plant Byblis gigantea (Byblidaceae) is cued by warm stratification and karrikinolide.Crossref | GoogleScholarGoogle Scholar |
Cross AT, Turner SR, Renton M, Baskin JM, Dixon KW, Merritt DJ (2015) Seed dormancy and persistent sediment seed banks of ephemeral freshwater rock pools in the Australian monsoon tropics. Annals of Botany 115, 847–859.
| Seed dormancy and persistent sediment seed banks of ephemeral freshwater rock pools in the Australian monsoon tropics.Crossref | GoogleScholarGoogle Scholar |
Dalziell EL, Lewandrowski W, Commander LE, Elliott CP, Erickson TE, Tudor EP, Turner SR, Merritt DJ (2022) Seed traits inform the germination niche for biodiverse ecological restoration. Seed Science and Technology 50, 103–124.
| Seed traits inform the germination niche for biodiverse ecological restoration.Crossref | GoogleScholarGoogle Scholar |
Dixon KW, Roche S, Pate JS (1995) The promotive effect of smoke derived from burnt native vegetation on seed germination of Western Australian plants. Oecologia 101, 185–192.
| The promotive effect of smoke derived from burnt native vegetation on seed germination of Western Australian plants.Crossref | GoogleScholarGoogle Scholar |
Donohue K (2014) Why ontogeny matters during adaptation: developmental niche construction and pleiotorpy across the life cycle in Arabidopsis thaliana. Evolution 68, 32–47.
| Why ontogeny matters during adaptation: developmental niche construction and pleiotorpy across the life cycle in Arabidopsis thaliana.Crossref | GoogleScholarGoogle Scholar |
Donohue K, Rubio de Casas R, Burghardt L, Kovach K, Willis CG (2010) Germination, postgermination adaptation, and species ecological ranges. Annual Review of Ecology, Evolution, and Systematics 41, 293–319.
| Germination, postgermination adaptation, and species ecological ranges.Crossref | GoogleScholarGoogle Scholar |
Duncan C, Schultz NL, Good MK, Lewandrowski W, Cook S (2019) The risk-takers and -avoiders: germination sensitivity to water stress in an arid zone with unpredictable rainfall. AoB PLANTS 11, plz066
| The risk-takers and -avoiders: germination sensitivity to water stress in an arid zone with unpredictable rainfall.Crossref | GoogleScholarGoogle Scholar |
Finch-Savage WE, Leubner-Metzger G (2006) Seed dormancy and the control of germination. New Phytologist 171, 501–523.
| Seed dormancy and the control of germination.Crossref | GoogleScholarGoogle Scholar |
Gremer JR, Kimball S, Venable DL (2016) Within-and among-year germination in Sonoran Desert winter annuals: bet hedging and predictive germination in a variable environment. Ecology Letters 19, 1209–1218.
| Within-and among-year germination in Sonoran Desert winter annuals: bet hedging and predictive germination in a variable environment.Crossref | GoogleScholarGoogle Scholar |
Gulden RH, Thomas AG, Shirtliffe SJ (2004) Secondary dormancy, temperature, and burial depth regulate seedbank dynamics in canola. Weed Science 52, 382–388.
| Secondary dormancy, temperature, and burial depth regulate seedbank dynamics in canola.Crossref | GoogleScholarGoogle Scholar |
Hajek OL, Knapp AK (2022) Shifting seasonal patterns of water availability: ecosystem responses to an unappreciated dimension of climate change. New Phytologist 233, 119–125.
| Shifting seasonal patterns of water availability: ecosystem responses to an unappreciated dimension of climate change.Crossref | GoogleScholarGoogle Scholar |
Hardegree SP, Roundy BA, Walters CT, Reeves PA, Richards CM, Moffet CA, Sheley RL, Flerchinger GN (2018) Hydrothermal germination models: assessment of the wet-thermal approximation of potential field response. Crop Science 58, 2042–2049.
| Hydrothermal germination models: assessment of the wet-thermal approximation of potential field response.Crossref | GoogleScholarGoogle Scholar |
Harrison SP, LaForgia ML, Latimer AM (2018) Climate-driven diversity change in annual grasslands: drought plus deluge does not equal normal. Global Change Biology 24, 1782–1792.
| Climate-driven diversity change in annual grasslands: drought plus deluge does not equal normal.Crossref | GoogleScholarGoogle Scholar |
Hidayati SN, Walck JL, Merritt DJ, Turner SR, Turner DW, Dixon KW (2012) Sympatric species of Hibbertia (Dilleniaceae) vary in dormancy break and germination requirements: implications for classifying morphophysiological dormancy in Mediterranean biomes. Annals of botany 109, 1111–1123.
| Sympatric species of Hibbertia (Dilleniaceae) vary in dormancy break and germination requirements: implications for classifying morphophysiological dormancy in Mediterranean biomes.Crossref | GoogleScholarGoogle Scholar |
Huang Z, Liu S, Bradford KJ, Huxman TE, Venable DL (2016) The contribution of germination functional traits to population dynamics of a desert plant community. Ecology 97, 250–261.
| The contribution of germination functional traits to population dynamics of a desert plant community.Crossref | GoogleScholarGoogle Scholar |
Lewandrowski W, Erickson TE, Dalziell EL, Stevens JC (2018) Ecological niche and bet-hedging strategies for Triodia (R.Br.) seed germination. Annals of Botany 121, 367–375.
| Ecological niche and bet-hedging strategies for Triodia (R.Br.) seed germination.Crossref | GoogleScholarGoogle Scholar |
Lüdecke D (2018) sjPlot: data visualization for statistics in social science. R package version 2.6.2. Zenodo. Available at https://zenodo.org/record/2400856#.Y_GqbhNBxlM
Mackenzie BDE, Auld TD, Keith DA, Hui FKC, Ooi MKJ (2016) The effect of seasonal ambient temperatures on fire-stimulated germination of species with physiological dormancy: a case study using Boronia (Rutaceae). PLoS ONE 11, e0156142
| The effect of seasonal ambient temperatures on fire-stimulated germination of species with physiological dormancy: a case study using Boronia (Rutaceae).Crossref | GoogleScholarGoogle Scholar |
Mackenzie BDE, Auld TD, Keith DA, Ooi MKJ (2021) Fire seasonality, seasonal temperature cues, dormancy cycling, and moisture availability mediate post-fire germination of species with physiological dormancy. Frontiers in Plant Science 12, 795711
| Fire seasonality, seasonal temperature cues, dormancy cycling, and moisture availability mediate post-fire germination of species with physiological dormancy.Crossref | GoogleScholarGoogle Scholar |
Maher K, Standish R, Hallett L (2008) Restoration of Banksia Woodland after the removal of pines at Gnangara: evaluation of seedling trials. Gnangara Sustainability Strategy, Perth, WA, Australia.
Martin AC (1946) The comparative internal morphology of seeds. American Midland Naturalist 36, 513–660.
| The comparative internal morphology of seeds.Crossref | GoogleScholarGoogle Scholar |
Martyn AJ, Seed LU, Ooi MKJ, Offord CA (2009) Seed fill, viability and germination of NSW species in the family Rutaceae. Cunninghamia 11, 203–212.
Mercer KL, Alexander HM, Snow AA (2011) Selection on seedling emergence timing and size in an annual plant, Helianthus annuus (common sunflower, Asteraceae). American Journal of Botany 98, 975–985.
| Selection on seedling emergence timing and size in an annual plant, Helianthus annuus (common sunflower, Asteraceae).Crossref | GoogleScholarGoogle Scholar |
Merritt DJ, Turner SR, Clarke S, Dixon DW (2007) Seed dormancy and germination stimulation syndromes for Australian temperate species. Australian Journal of Botany 55, 336–344.
| Seed dormancy and germination stimulation syndromes for Australian temperate species.Crossref | GoogleScholarGoogle Scholar |
Michel BE, Wiggins OK Michel BE, Wiggins OK (1983) A guide to establishing water potential of aqueous two-phase solutions (polyethylene glycol plus dextran) by amendment with mannitol. Plant Physiology 72, 60–65.
| A guide to establishing water potential of aqueous two-phase solutions (polyethylene glycol plus dextran) by amendment with mannitol.Crossref | GoogleScholarGoogle Scholar |
Miller RG, Fontaine JB, Merritt DJ, Miller BP, Enright NJ (2021) Experimental seed sowing reveals seedling recruitment vulnerability to unseasonal fire. Ecological Applications 31, e02411
| Experimental seed sowing reveals seedling recruitment vulnerability to unseasonal fire.Crossref | GoogleScholarGoogle Scholar |
Norman MA, Koch JM (2006) The investigation of seed coat chipping, seed coat ageing and warm temperature stratificiation for snottygobble (Persoonia longifolia). Alcoa of Australia, Pty Ltd., Pinjarra, WA, Australia.
Norman MA, Plummer JA, Koch JM, Mullins GR (2006) Optimising smoke treatments for jarrah (Eucalyptus marginata) forest rehabilitation. Australian Journal of Botany 54, 571–581.
| Optimising smoke treatments for jarrah (Eucalyptus marginata) forest rehabilitation.Crossref | GoogleScholarGoogle Scholar |
Ooi MKJ (2010) Delayed emergence and post-fire recruitment success: effects of seasonal germination, fire season and dormancy type. Australian Journal of Botany 58, 248–256.
| Delayed emergence and post-fire recruitment success: effects of seasonal germination, fire season and dormancy type.Crossref | GoogleScholarGoogle Scholar |
R Core Team (2013) ‘R: a language and environment for statistical computing.’ (R Foundation for Statistical Computing: Vienna, Austria) https://www.R-project.org/
Ritz C, Pipper CB, Streibig JC (2013) Analysis of germination data from agricultural experiments. European Journal of Agronomy 45, 1–6.
| Analysis of germination data from agricultural experiments.Crossref | GoogleScholarGoogle Scholar |
Ritz C, Strebig JC, Ritz MC (2016) Package ‘drc’. Creative Commons, Mountain View, CA, USA. https://cran.r-project.org/web/packages/drc/drc.pdf
Roche S, Dixon KW, Pate JS (1997) Seed ageing and smoke: partner cuesin the amelioration of seed dormancyin selected Australian native species. Australian Journal of Botany 45, 783–815.
| Seed ageing and smoke: partner cuesin the amelioration of seed dormancyin selected Australian native species.Crossref | GoogleScholarGoogle Scholar |
Rokich DP, Dixon KW, Sivasithamparam K, Meney KA (2002) Smoke, mulch, and seed broadcasting effects on woodland restoration in Western Australia. Restoration Ecology 10, 185–194.
| Smoke, mulch, and seed broadcasting effects on woodland restoration in Western Australia.Crossref | GoogleScholarGoogle Scholar |
Saatkamp A, Cochrane A, Commander L, et al. (2019) A research agenda for seed-trait functional ecology. New Phytologist 221, 1764–1775.
| A research agenda for seed-trait functional ecology.Crossref | GoogleScholarGoogle Scholar |
Salazar A, Goldstein G, Franco AC, Miralles-Wilhelm F (2011) Timing of seed dispersal and dormancy, rather than persistent soil seed-banks, control seedling recruitment of woody plants in Neotropical savannas. Seed Science Research 21, 103–116.
| Timing of seed dispersal and dormancy, rather than persistent soil seed-banks, control seedling recruitment of woody plants in Neotropical savannas.Crossref | GoogleScholarGoogle Scholar |
Simons AM (2011) Modes of response to environmental change and the elusive empirical evidence for bet hedging. Proceedings of the Royal Society B: Biological Sciences 278, 1601–1609.
| Modes of response to environmental change and the elusive empirical evidence for bet hedging.Crossref | GoogleScholarGoogle Scholar |
Soltani E, Baskin CC, Baskin JM (2017) A graphical method for identifying the six types of non-deep physiological dormancy in seeds. Plant Biology 19, 673–682.
| A graphical method for identifying the six types of non-deep physiological dormancy in seeds.Crossref | GoogleScholarGoogle Scholar |
Steadman KJ, Pritchard HW (2004) Germination of Aesculus hippocastanum seeds following cold-induced dormancy loss can be described in relation to a temperature-dependent reduction in base temperature (Tb) and thermal time. New Phytologist 161, 415–425.
| Germination of Aesculus hippocastanum seeds following cold-induced dormancy loss can be described in relation to a temperature-dependent reduction in base temperature (Tb) and thermal time.Crossref | GoogleScholarGoogle Scholar |
ten Brink H, Gremer JR, Kokko H (2020) Optimal germination timing in unpredictable environments: the importance of dormancy for both among- and within-season variation. Ecology Letters 23, 620–630.
| Optimal germination timing in unpredictable environments: the importance of dormancy for both among- and within-season variation.Crossref | GoogleScholarGoogle Scholar |
Thomson DM, King RA, Schultz EL (2017) Between invaders and a risky place: exotic grasses alter demographic tradeoffs of native forb germination timing. Ecosphere 8, e01987
| Between invaders and a risky place: exotic grasses alter demographic tradeoffs of native forb germination timing.Crossref | GoogleScholarGoogle Scholar |
Turner S, Merritt D, Ridley E, Commander L, Baskin J, Baskin C, Dixon K (2006) Ecophysiology of seed dormancy in the Australian endemic species Acanthocarpus preissii (Dasypogonaceae). Annals of Botany 98, 1137–1144.
| Ecophysiology of seed dormancy in the Australian endemic species Acanthocarpus preissii (Dasypogonaceae).Crossref | GoogleScholarGoogle Scholar |
Turner S, Merritt D, Renton M, Dixon K (2009) Seed moisture content affects afterripening and smoke responsiveness in three sympatric Australian native species from fire-prone environments. Austral Ecology 34, 866–877.
| Seed moisture content affects afterripening and smoke responsiveness in three sympatric Australian native species from fire-prone environments.Crossref | GoogleScholarGoogle Scholar |
Turner SR, Lewandrowski W, Elliott CP, Merino-Martín L, Miller BP, Stevens JC, Erickson TE, Merritt DJ (2018) Seed ecology informs restoration approaches for threatened species in water-limited environments: a case study on the short-range Banded Ironstone endemic Ricinocarpos brevis (Euphorbiaceae). Australian Journal of Botany 65, 661–677.
| Seed ecology informs restoration approaches for threatened species in water-limited environments: a case study on the short-range Banded Ironstone endemic Ricinocarpos brevis (Euphorbiaceae).Crossref | GoogleScholarGoogle Scholar |
Turner SR, Cross AT, Just M, Newton V, Pedrini S, Tomlinson S, Dixon K (2022) Restoration seedbanks for mined land restoration. Restoration Ecology 30, e13667
| Restoration seedbanks for mined land restoration.Crossref | GoogleScholarGoogle Scholar |
Verdú M, Traveset A (2005) Early emergence enhances plant fitness: a phylogenetically controlled meta-analysis. Ecology 86, 1385–1394.
| Early emergence enhances plant fitness: a phylogenetically controlled meta-analysis.Crossref | GoogleScholarGoogle Scholar |
Walck JL, Hidayati SN, Dixon KW, Thompson K, Poschlod P (2011) Climate change and plant regeneration from seed. Global Change Biology 17, 2145–2161.
| Climate change and plant regeneration from seed.Crossref | GoogleScholarGoogle Scholar |
Yan W, Hunt L (1999) An equation for modelling the temperature response of plants using only the cardinal temperatures. Annals of Botany 84, 607–614.
| An equation for modelling the temperature response of plants using only the cardinal temperatures.Crossref | GoogleScholarGoogle Scholar |
Yeager DP, Ultsch GR (1989) Physiological regulation and conformation: a BASIC program for the determination of critical points. Physiological Zoology 62, 888–907.
| Physiological regulation and conformation: a BASIC program for the determination of critical points.Crossref | GoogleScholarGoogle Scholar |